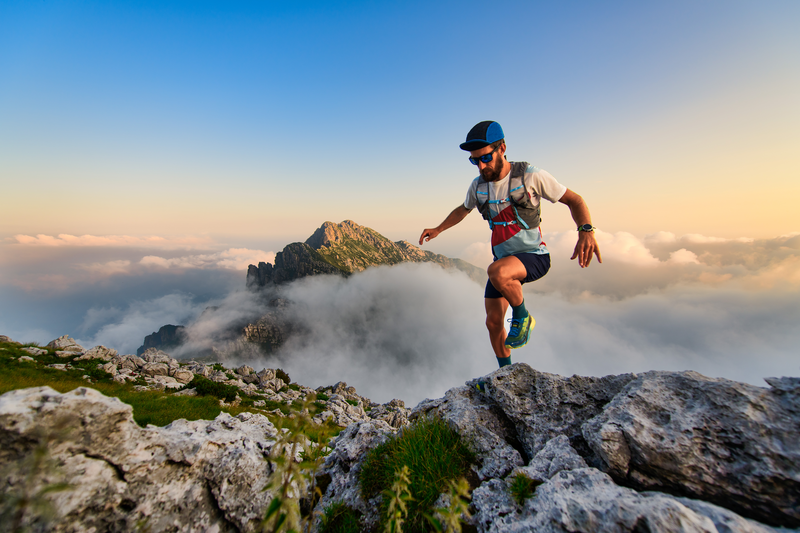
95% of researchers rate our articles as excellent or good
Learn more about the work of our research integrity team to safeguard the quality of each article we publish.
Find out more
ORIGINAL RESEARCH article
Front. Chem. , 15 March 2021
Sec. Catalysis and Photocatalysis
Volume 9 - 2021 | https://doi.org/10.3389/fchem.2021.652762
The development of graphite-carbon nitride (g-C3N4) photocatalyst is of great significance for various visible utilization applications. Control the nanostructures of g-C3N4 can tailor its photocatalytic performance. In this paper, one-dimensional chain-like g-C3N4 was successfully synthesized by heat-induced polymerization of melamine which was saturated in ethylene glycol. The photocatalytic hydrogen production rate (HER) of the prepared g-C3N4 chain enhanced about 3 times than that of bulk g-C3N4, increasing from 9.6 μmolh−1 to 28.7 μmolh−1. The improved photocatalytic activity of the g-C3N4 chain was attributed to the advantages of porosity and nanostructure. The extraordinary nanopores result in an enlarged specific surface area for adsorption and the production of abundantly available channels for charge transfer. The one-dimensional chain-like structure can facilitate the exposure of internal/external active sites as many as possible, and induce the directional migration of charge carriers.
With the rapid pace of industrialization and urbanization growing in the past few decades, global crises related to environmental degradation and energy shortage have become the most critical topics to the world and threaten the survival environment of humankind (Yu et al., 2017; Yu et al., 2019). Photocatalytic water splitting for hydrogen evolution has been considered as a sustainable strategy to convert and store plentiful solar energy for future energy requirements (Wang et al., 2009; Wang et al., 2012; Ong et al., 2016). In the last several decades, graphitic carbon nitride (g-C3N4) the organic semiconductor showed a specific graphite-like sp2-bonded C–N structure that exhibits significant potential in the field of CO2 conversion, water splitting, and environmental remediation on account of its inexpensive preparation, brilliant visible light response, thermal stability, and well-developed electronic band structure (Ding et al., 2018; He et al., 2019; Rong et al., 2020; Shu et al., 2020; Zhang D. et al., 2020). However, g-C3N4 accompanied by the defaults such as poor quantum efficiency, low specific surface area, and rapid charge recombination have adversely affected the photocatalytic application of g-C3N4.
A large number of strategies acting to resolve these problems and reinforce the photocatalytic performances of g-C3N4, involving non-metal element doping [S (Cao et al., 2018), O (Jiang et al., 2019), V (Ding et al., 2013), B (Yan et al., 2010), etc.], noble metals decoration [Pd (Wang et al., 2011) Pt (Maeda et al., 2009) and Au (Li et al., 2012)], heterojunction designing (Liang et al., 2019; Shi et al., 2020a; Shi et al., 2020b) and coupling with graphene (Li et al., 2013; Han et al., 2017). Expect for the capacity of g-C3N4 to collaborate with other materials, layers structure configuration is deemed as a prospective method to compound g-C3N4 as they can accelerate the diffusion of reactant, strengthen the light harvesting, expand the exposed surface areas, and promote the charge delivery.
Generally speaking, microstructural g-C3N4 photocatalysts with controllable morphology and structure can be divided into six categories: mesoporous (Zhao et al., 2018; Chen et al., 2019), nanosheets (Yang et al., 2017; Gao et al., 2018), nanorods (Cui et al., 2012; Bai et al., 2013), nanotubes (Gao et al., 2012), and nanospheres (Zheng et al., 2015). Among them, one-dimensional (1D) nanostructured photocatalyst, especially fabricating 1D photocatalyst with large surface area, is especially interested as the charges can vectorially transfer along with the 1D structure (Tahir et al., 2014).
After discovering the carbon nanotubes, 1D nanostructures (lines, rods, tubes, strips, fibers, etc) have attracted extensive attention from researchers. 1D nanostructures have greatly satisfied the increasing demand for microelectronics and optoelectronic devices such as optical waveguides, field-effect transistors, and photodetectors in recent years. 1D nanostructures showed brilliant phonon, gas sensitivity, field emission, photoconductivity, and electron transport performance due to their higher surface volume ratio and more active position. Furthermore, the growth of 1D nanostructures has an immense effect on improving the mechanical energy, thermal and electrical capabilities of materials. Because nanoparticles in 1D nanostructures are interconnected in three dimensions, an extremely fast interparticle, vectorially transport of photogenerated charge carriers (electrons and holes) is likely to emerge through the grain boundaries. This represents that the redox reaction sites are far away related to the photoexcitation sites, which seems to be responsible for the high activities of photocurrent generation and hydrogen production.
In the present work, 1D g-C3N4 chain nanostructures were obtained by regulating the saturation of melamine and ethylene glycol solution for photocatalytic hydrogen evolution. The enhanced photocatalytic properties are known to be caused by an extension of the life of the photoinduced charge carrier. In addition, increasing specific surface area is also a vital factor in promising photocatalytic performance. Owing to the composite method is convenient, environmentally friendly, and low-cost, it is suitable for an expanded range of practical applications.
The one-dimensional g-C3N4 chain nanostructures were prepared by a controllable approach. Firstly, excessive amounts of melamine powders (3.0 g) were dissolved in 60 ml of ethylene glycol to form a saturated solution at room temperature. Subsequently, add 1 ml of concentrated nitric acid solution to 59 ml of water, drop by drop add the solution to 20 ml of supernatant and stir continuously until a white flocculent precipitate is obtained. The white flocculent precipitate was bleached with ethanol five times to remove nitric acid and ethylene glycol. Lastly, the collected sample was transferred into the muffle furnace for heating 2 h at 550°C with a heating rate of 20°C·min−1. Meanwhile, as the contrast sample, the bulk g-C3N4 was obtained by the heating process at 550°C for 2 h with a heating rate of 20°C·min−1 of the melamine powders.
The crystal structure was characterized by X-ray diffraction (XRD, D/max2600, Rigaku, Japan) using the Cu Ka radiation (k = 1.5418 Å). The morphologies of one-dimensional g-C3N4 chain nanostructures were characterized by scan electron microscopy (SEM, SU70, Hitachi, Japan). And specific surface areas of the one-dimensional g-C3N4 chain nanostructures were measured by a Micromeritics ASAP 2010 instrument and analyzed by the Brunauer–Emmett–Teller (BET) method. Photoluminescence (PL) spectra of photocatalysts were performed on a Jobin Yvon HR800 micro-Raman spectrometer including a 325 nm line from a He-Cd laser. UV-Vis diffuse reflectance spectra (DRS) of the samples are obtained by using a UV-Vis-IR spectrometer (Perkin-Elmer, Lambda 850). Photocurrent measurements were characterized by CHI 660 E electrochemical workstation (Chenhua, Shanghai) by applying a three-electrode cell accompanied by a visible light source. A platinum filament, Ag/AgCl electrode, and 0.2 M of Na2SO4 were acted as the counter electrode, reference electrode, and an electrolyte solution, separately. The as-fabricated sample mixed a certain amount of Nafion solution was painted on FTO glass as a working electrode (the effective area was 1 cm × 1 cm).
Photocatalytic H2 evolution was injected into a 250 ml of quartz reactor with a visible-light source irradiation. As a typical synthesis experiment, 0.1 g of photocatalyst with a certain Pt cocatalyst (1 wt%) was dispersed in a mixed solution of aqueous solution (90 ml) and methanol (10 ml). The amount of H2 evolution was measured in a gas chromatograph (GC-2014C Shimadzu Corp., N2 as carrier gas). The time interval of sampling was performed at 40 min during the water splitting process.
The morphology of the as-fabricated products was scrutinized by scanning electron microscope (SEM). Figure 1A depicts the SEM image of the as-prepared bulk g-C3N4, in which the sample possesses a hierarchical component with a particle size of greater than 20 μm. However, in Figure 1B, we found that the morphology of g-C3N4 changed greatly compared with the bulk structure, and a new one-dimensional chain structure appeared. It can be illustrated from Figure 1B that the lengths of these irregular-oriented chains g-C3N4 one-dimensional structure could reach dozens of micrometers, and the diameters of those fibers range from 1 to 2 µm. Each one-dimensional structure was separated from each other, and further secondary structures could be found. Through the observation of the morphology characteristics of the two structures, we can infer that the chain g-C3N4 material will possess a vast specific surface area, which is more conducive to enhance the photocatalytic performance. The transmission electron micrographs of chain g-C3N4 are shown in Figures 1C,D. We can see the chain g-C3N4 presents a similar sponge hole that exists on the surface of the chain g-C3N4, the morphology can effectively improve the capacity in the course of the photocatalytic reaction area. Meanwhile, the product can shorten the time and distance of the charge transfer, and promote the charge separation order to improve the photocatalytic activity of the material. As we all known, in the pyrolysis period of supramolecular precursor, the by-products were formed with varieties of gases gradually released, giving rise to the nitrogen defects obtained in the framework of g-C3N4.
FIGURE 1. SEM images of (A) bulky g-C3N4 and (B) chain g-C3N4 at low and high magnification, and (C, D) TEM image of chain g-C3N4 at low and high magnification; (E) XRD and (F) FT-IR patterns of bulky g-C3N4 and pearl-chain g-C3N4.
X-ray diffraction (XRD) patterns for chain g-C3N4 and bulky g-C3N4 are expressed in Figure 1E. Obviously, both patterns contain two diffraction peaks, which are located at 13.2° and 27.6°, respectively. The former peak at 13.2° could be indexed as (100) lattice plane, which is associated with interlayer stacking. The corresponding interlayer spacing value has been calculated to be 0.676 nm. And the later peak at 27.6° is a feature interlayer stacking peak of aromatic systems, which could be indexed as (002) lattice plane. The calculated interplanar distance of aromatic units is 0.326 nm (Li et al., 2020a; Zhang et al., 2020b).
Figure 1F shows the Fourier transform infrared (FT-IR) spectra of the as-prepared samples. The FT-IR spectra of the synthesized chain g-C3N4 were compared to the bulky g-C3N4 exhibit with similar characteristics. The FT-IR the bands located at 1,240, 1,321, 1,415 cm−1, and 1,570 cm−1 are mainly from the typical stretching modes of C-N heterocycles. And the band at 810 cm−1 is attributed to out-of-plane bending modes of C-N heterocycles. The C-N stretching mode has IR band at 1,635 cm−1. And the broadband near 3,200 cm−1 corresponds to the stretching modes of terminal NH2 or NH groups at the defect sites of the aromatic ring (Li et al., 2020b; Li et al., 2020c).
The exposed surface area and pores distribution of chain g-C3N4 and bulky g-C3N4 were further researched. The adsorption-desorption isotherm curve of chain g-C3N4 and bulky g-C3N4 demonstrate the type IV curve, as shown in Figure 2, indicating the presence of uniform mesoporous with high specific surface area and large total pore volume. The specific surface area of chain g-C3N4 was counted to be 47.85 m2g−1 through the Brunauer-Emmett-Teller (BET), which is approximately three times larger than pure bulky g-C3N4 (16.15 m2g−1). The inset in Figure 2 exhibits the pore-size distribution of the chain g-C3N4 and bulky g-C3N4. The pore distribution for chain g-C3N4 is mainly located at 2.46 nm, and the pore volume is 0.233 cm3g−1. But for the bulky g-C3N4, the pore volume is just 0.097 cm3g−1. The mesoporous structure and large surface area of the chain g-C3N4 are conducive to the absorption of more active substances and reactants on the surface, enhancing the photocatalytic reaction. Therefore, we can assume that the chain g-C3N4 material can have superior photocatalytic activity.
The UV-vis diffuse reflectance spectroscopy illustrated by Figure 3. from the (αhv)1/2 vs. photon energy (hv) plot (Hou et al., 2020; Li et al., 2020d; Wang et al., 2020a; Zhang et al., 2020a; Zhang Y. et al., 2020), the optical bandgap of chain g-C3N4 and bulk g-C3N4 was calculated to be approximate 2.88 and 2.93 eV, respectively. When the particle size descends to a certain value, the electron energy level near the Fermi energy level of the metal changes from quasi-continuous to discrete energy level (Miao et al., 2019; Huang et al., 2020b; Wu et al., 2020; Zhang S. et al., 2020), and the highest occupied molecular orbital (HOMO) and lowest unoccupied molecular orbital energy level (LUMO) of the nano-semiconductor particles have discontinuous energy gap, which leads to the blue shift of the chain g-C3N4.
According to mentioned above, the prepared chain g-C3N4 has a one-dimensional superstructure with a wide surface area, making it a more suitable candidate material for photocatalytic H2 production. To evaluate the photocatalytic performance of chain g-C3N4 photocatalyst with the visible light irradiation, the H2 production performance was tested and compared with that of bulky g-C3N4 photocatalytic performance was compared.
As the result shown in Figure 4A, the H2 evolution rate of the bare bulky g-C3N4 sample was measured to be 9.6 μmolh−1, separately. However, the photocatalytic properties of chain g-C3N4 were markedly improved, and the H2 generation rate increased as high as 28.7 μmolh−1. The chain g-C3N4 sample shows rather superior photocatalytic activity for H2 evolution, which could be ascribed to its vaster surface area (Figure 2). Figure 4B shows the stability of the photocatalytic H2 production system using chain g-C3N4 as the photocatalyst under visible light irradiation. Therefore, we studied the chemical stability of chain g-C3N4, as described in Figure 4B. After four consecutive cycle experiments, we found that the evolution rate of the chain g-C3N4 did not decline significantly, indicating that the material has good chemical stability.
FIGURE 4. (A) The amount of hydrogen evolution over the bulky g-C3N4 and chain g-C3N4, and (B) cycling test of photocatalytic hydrogen evolution under visible light.
The key factor of photocatalytic reaction is the effective generation and rapid separation of photoexcited carriers (Huang et al., 2020a; Li X. et al., 2020; Wang L. et al., 2020; Wang et al., 2020b; Zhang et al., 2020c). The optical performances of the samples were measured by photoluminescence (PL). The intensity of PL spectra can state the extent of the recombination of photo-generated charges. In Figure 5A, it illustrates bulky g-C3N4 emerge severe charge recombination, while the PL spectrum of chain g-C3N4 is intense quenched (Figure 5A). The reorganizing of the photo-generated e-h pairs of chain g-C3N4 can be restrained. The restraining of the e-h pair recombination is powerfully verified by the increased photocurrents for chain g-C3N4, as shown in Figure 5B. Apparently, the chain g-C3N4 was provided with a better separation efficiency of e-h pairs.
To further confirm the ability of several samples to separate and transfer charges and to respond to light (Cheng et al., 2019; Li et al., 2021), we performed photocurrent tests on them, as shown in Figure 5B six cycles were tested under λ > 420 nm xenon lamp. As can be seen from the diagram, two working electrode transient photocurrent response of bulky g-C3N4 and chain g-C3N4 were obtained through these six cycles. It can be seen that the transient photocurrent spectrum of the chain g-C3N4 electrode was much better than the bulky g-C3N4. Test results indicate that chain g-C3N4 greatly improves the separation of interface carriers. The photocurrent research consequences coincide with the impedance and photoluminescence research consequences, demonstrating that the chain g-C3N4 material will have better photocatalytic performance.
In summary, we proposed an annealing method to achieve bulky g-C3N4 and chain g-C3N4 utilizing melamine as reactant materials. The chain g-C3N4 nanostructures illustrated improvement on the photocatalytic H2 production under visible light irradiation owing to unique inimitable one-dimensional structure, high specific surface area, excellent light-harvesting properties, and low recombination rate of electron-hole pairs. This simple preparation and facile composition of one-dimensional g-C3N4 nanostructures demonstrate a promising candidate for exploring more actual applications of carbon nitride.
The original contributions presented in the study are included in the article/Supplementary Material, further inquiries can be directed to the corresponding author.
All authors listed have made a substantial, direct, and intellectual contribution to the work and approved it for publication.
This work was supported by the National Natural Science Foundation of China (51872068), Heilongjiang Natural Science Foundation (E2018051).
The authors declare that the research was conducted in the absence of any commercial or financial relationships that could be construed as a potential conflict of interest.
Bai, X., Wang, L., Zong, R., and Zhu, Y. (2013). Photocatalytic activity enhanced via g-C3N4 nanoplates to nanorods. J. Phys. Chem. C. 117, 9952–9961. doi:10.1021/jp402062d
Cao, S., Fan, B., Feng, Y., Chen, H., Jiang, F., and Wang, X. (2018). Sulfur-doped g-C3N4 nanosheets with carbon vacancies: general synthesis and improved activity for simulated solar-light photocatalytic nitrogen fixation. Chem. Eng. Technol. 353, 147–156. doi:10.1016/j.cej.2018.07.116
Chen, X., Shi, R., Chen, Q., Zhang, Z., Jiang, W., Zhu, Y., et al. (2019). Three-dimensional porous g-C3N4 for highly efficient photocatalytic overall water splitting. Nano Energy 59, 644–650. doi:10.1016/j.nanoen.2019.03.010
Cheng, J., Hu, Z., Li, Q., Li, X., Fang, S., Wu, X., et al. (2019). Fabrication of high photoreactive carbon nitride nanosheets by polymerization of amidinourea for hydrogen production. Appl. Catal. B-Environ. 245, 197–206. doi:10.1016/j.apcatb.2018.12.044
Cui, Y., Ding, Z., Fu, X., and Wang, X. (2012). Construction of conjugated carbon nitride nanoarchitectures in solution at low temperatures for photoredox catalysis. Angew. Chem. Int. Ed. Engl. 51, 11814–11818. doi:10.1002/anie.201206534
Ding, G., Wang, W., Jiang, T., Han, B., Fan, H., and Yang, G. (2013). Highly selective synthesis of phenol from benzene over a vanadium-doped graphitic carbon nitride catalyst. ChemCatChem 5, 192–200. doi:10.1002/cctc.201200502
Ding, J., Xu, W., Wan, H., Yuan, D., Chen, C., Wang, L., et al. (2018). Nitrogen vacancy engineered graphitic C3N4-based polymers for photocatalytic oxidation of aromatic alcohols to aldehydes. Appl. Catal. B-Environ. 221, 626–634. doi:10.1016/j.apcatb.2017.09.048
Gao, M., Feng, J., Zhang, Z., Gu, M., Wang, J., Zeng, W., et al. (2018). Wrinkled ultrathin graphitic g-C3N4 nanosheets for photocatalytic degradation of prganic wastewater. ACS Appl. Nano Mater. 1, 6733–6741. doi:10.1021/acsanm.8b01528
Gao, J., Zhou, Y., Li, Z., Yan, S., Wang, N., and Zou, Z. (2012). High-yield synthesis of millimetre-long, semiconducting carbon nitride nanotubes with intense photoluminescence emission and reproducible photoconductivity. Nanoscale 4, 3687–3692. doi:10.1039/c2nr30777d
Han, Q., Cheng, Z., Gao, J., Zhao, Y., Zhang, Z., Dai, L., et al. (2017). Mesh-on-mesh graphitic-C3N4@graphene for highly efficient hydrogen evolution. Adv. Funct. Mater. 27, 1606352. doi:10.1002/adfm.201606352
He, S., Xiao, K., Chen, X.-Z., Li, T., Ouyang, T., Wang, Z., et al. (2019). Enhanced photoelectrocatalytic activity of direct Z-scheme porous amorphous carbon nitride/manganese dioxide nanorod arrays. J. Colloid Interface Sci. 557, 644–654. doi:10.1016/j.jcis.2019.09.035
Hou, R., Zhang, S., Zhang, P., Zhang, Y., Zhang, X., Li, N., et al. (2020). Ti3C2 MXene as an “energy band bridge” to regulate the heterointerface mass transfer and electron reversible exchange process for Li-S batteries. J. Mater. Chem. A 8, 25255. doi:10.1039/d0ta06695h
Huang, J., Du, J., Du, H., Xu, G., and Yuan, Y. (2020a). Control of nitrogen vacancy in g-C3N4 by heat treatment in an ammonia atmosphere for enhanced photocatalytic hydrogen generation. Acta. Phys. Chim. Sin. 36, 1905056. doi:10.3866/pku.whxb201905056
Huang, J., Liu, T., Wang, R., Zhang, M., Wang, L., She, H., et al. (2020b). Facile loading of cobalt oxide on bismuth vanadate: proved construction of p-n junction for efficient photoelectrochemical water oxidation. J. Colloid Interface Sci. 570, 89–98. doi:10.1016/j.jcis.2020.02.109
Jiang, Y., Sun, Z., Tang, C., Zhou, Y., Zeng, L., and Huang, L. (2019). Enhancement of photocatalytic hydrogen evolution activity of porous oxygen doped g-C3N4 with nitrogen defects induced by changing electron transition. Appl. Catal. B-Environ. 240, 30–38. doi:10.1016/j.apcatb.2018.08.059
Li, X.-H., Wang, X., and Antonietti, M. (2012). Mesoporous g-C3N4 nanorods as multifunctional supports of ultrafine metal nanoparticles: hydrogen generation from water and reduction of nitrophenol with tandem catalysis in one step. Chem. Sci. 3, 2170–2174. doi:10.1039/c2sc20289a
Li, Y., Zhang, H., Liu, P., Wang, D., Li, Y., and Zhao, H. (2013). Cross-linked g-C3 N4 /rGO nanocomposites with tunable band structure and enhanced visible light photocatalytic activity. Small 9, 3336–44. doi:10.1002/smll.201203135
Li, X., Wang, B., Wang, B., Yin, W., Di, J., Xia, J., et al. (2020). Cu2+ modified g-C3N4 photocatalysts for visible light photocatalytic properties. Acta Phys. Chim. Sin. 36, 1902001. doi:10.3866/pku.whxb201902001
Li, Y., Gu, M., Shi, T., Cui, W., Zhang, X., Dong, F., et al. (2020a). Carbon vacancy in C3N4 nanotube: electronic structure, photocatalysis mechanism and highly enhanced activity. Appl. Catal. B-Environ. 262, 118281. doi:10.1016/j.apcatb.2019.118281
Li, Y., Gu, M., Zhang, M., Zhang, X., Lv, K., Liu, Y., et al. (2020b). C3N4 with engineered three coordinated (N3C) nitrogen vacancy boosts the production of 1O2 for Efficient and stable NO photo-oxidation. Chem. Eng. J. 389, 124421. doi:10.1016/j.cej.2020.124421
Li, Y., Gu, M., Zhang, X., Fan, J., Lv, K., Carabineiro, S. A. C., et al. (2020c). 2D g-C3N4 for advancement of photo-generated carrier dynamics: status and challenges. Mater. Today 41, 270–303. doi:10.1016/j.mattod.2020.09.004
Li, Y., Zhang, P., Wan, D., Xue, C., Zhao, J., and Shao, G. (2020d). Direct evidence of 2D/1D heterojunction enhancement on photocatalytic activity through assembling MoS2 nanosheets onto super-long TiO2 nanofibers. Appl. Surf. Sci. 504, 144361. doi:10.1016/j.apsusc.2019.144361
Li, K. N., Zhang, M. X., Ou, X. Y., Li, R. N., Li, Q., Fan, J. J., et al. (2021). Strategies for the fabrication of 2D carbon nitride nanosheets. Acta. Phys. Chim. Sin. 37, 2008010. doi:10.3866/PKU.WHXB202008010
Liang, S., Zhang, D., Pu, X., Yao, X., Han, R., Yin, J., and Ren, X. (2019). A novel Ag2O/g-C3N4 p-n heterojunction photocatalysts with enhanced visible and near-infrared light activity. Sep. Purif. Technol. 210, 786–797. doi:10.1016/j.seppur.2018.09.008
Maeda, K., Wang, X., Nishihara, Y., Lu, D., Antonietti, M., and Domen, K. (2009). Photocatalytic activities of graphitic carbon nitride powder for water reduction and oxidation under visible light. J. Phys. Chem. C. 113, 4940–4947. doi:10.1021/jp809119m
Miao, F., Lu, N., Zhang, P., Zhang, Z., and Shao, G. (2019). Multidimension‐controllable synthesis of ant nest‐structural electrode materials with unique 3D hierarchical porous features toward electrochemical applications. Adv. Funct. Mater. 29, 1808994. doi:10.1002/adfm.201808994
Ong, W.-J., Tan, L.-L., Ng, Y. H., Yong, S.-T., and Chai, S.-P. (2016). Graphitic carbon nitride (g-C3N4)-based photocatalysts for artificial photosynthesis and environmental remediation: are we a step closer to achieving sustainability? Chem. Rev. 116, 7159–7329. doi:10.1021/acs.chemrev.6b00075
Rong, X., Liu, S., Xie, M., Liu, Z., Wu, Z., Zhou, X., et al. (2020). N2 photofixation by Z-scheme single-layer g-C3N4/ZnFe2O4 for cleaner ammonia production. Mater. Res. Bull. 127, 110853. doi:10.1016/j.materresbull.2020.110853
Shi, W., Li, M., Huang, X., Ren, H., Yan, C., and Guo, F. (2020a). Facile synthesis of 2D/2D Co3(PO4)2/g-C3N4 heterojunction for highly photocatalytic overall water splitting under visible light. Chem. Eng. J. 382, 122960. doi:10.1016/j.cej.2019.122960
Shi, W., Liu, C., Li, M., Lin, X., Guo, F., and Shi, J. (2020b). Fabrication of ternary Ag3PO4/Co3(PO4)2/g-C3N4 heterostructure with following Type II and Z-scheme dual pathways for enhanced visible-light photocatalytic activity. J. Hazard. Mater. 389, 121907. doi:10.1016/j.jhazmat.2019.121907
Shu, K., Chen, F., Shi, W., Guo, F., Tang, Y., Ren, H., et al. (2020). Construction of DyVO4/nitrogen deficient g-C3N4 composite for enhanced visible-light photocatalytic activity for tetracycline degradation. Mater. Res. Bull. 124, 110766. doi:10.1016/j.materresbull.2020.110766
Tahir, M., Cao, C., Mahmood, N., Butt, F. K., Mahmood, A., Idrees, F., et al. (2014). Multifunctional g-C3N4 Nanofibers: a template-free fabrication and enhanced optical, electrochemical, and photocatalyst properties. ACS Appl. Mater. Interfaces 6, 1258–1265. doi:10.1021/am405076b
Wang, X., Maeda, K., Thomas, A., Takanabe, K., Xin, G., Carlsson, J. M., et al. (2009). A metal-free polymeric photocatalyst for hydrogen production from water under visible light. Nature Mater. 8, 76–80. doi:10.1038/nmat2317
Wang, Y., Yao, J., Li, H., Su, D., and Antonietti, M. (2011). Highly selective hydrogenation of phenol and derivatives over a Pd@carbon nitride catalyst in aqueous media. J. Am. Chem. Soc. 133, 2362–2365. doi:10.1021/ja109856y
Wang, Y., Wang, X., and Antonietti, M. (2012). Polymeric graphitic carbon nitride as a heterogeneous organocatalyst: from photochemistry to multipurpose catalysis to sustainable chemistry. Angew. Chem. Int. Ed. Engl. 51, 68–89. doi:10.1002/anie.201101182
Wang, L., Zhu, C., Yin, L., and Huang, W. (2020). Construction of Pt-M (M = Co, Ni, Fe)/g-C3N4 composites for highly efficient photocatalytic H2 generation. Acta Phys. Chim. Sin. 36, 1907001. doi:10.3866/pku.whxb201907001
Wang, Y., Liu, J., Wang, Y., and Zhang, M. (2020a). CO2 photoreduction to CO/CH4 over Bi2W0.5Mo0.5O6 solid solution nanotubes under visible light. RSC Adv. 10, 8821–8824. doi:10.1039/d0ra00672f
Wang, Y., Shen, S. H., and Shen, S. (2020b). Progress and prospects of non-metal doped graphitic carbon nitride for improved photocatalytic performances. Acta Phys. Chim. Sin. 36, 1905080. doi:10.3866/pku.whxb201905080
Wu, J. Q., Hua, W. M., Yue, Y. H., and Gao, Z. (2020). Swelling characteristics of g-C3N4 as base catalyst in liquid-phase reaction. Acta Phys. Chim. Sin. 36, 1904066. doi:10.3866/pku.whxb201912023
Yan, S. C., Li, Z. S., and Zou, Z. G. (2010). Photodegradation of rhodamine B and methyl orange over boron-doped g-C3N4 under visible light irradiation. Langmuir 26, 3894–3901. doi:10.1021/la904023j
Yang, Y., Chen, J., Mao, Z., An, N., Wang, D., and Fahlman, B. D. (2017). Ultrathin g-C3N4 nanosheets with an extended visible-light-responsive range for significant enhancement of photocatalysis. RSC Adv. 7, 2333–2341. doi:10.1039/c6ra26172h
Yu, Z., Li, F., Yang, Q., Shi, H., Chen, Q., and Xu, M. (2017). Nature-mimic method to fabricate polydopamine/graphitic carbon nitride for enhancing photocatalytic degradation performance. ACS Sustainable Chem. Eng. 5, 7840–7850. doi:10.1021/acssuschemeng.7b01313
Yu, C., He, H., Liu, X., Zeng, J., and Liu, Z. (2019). Novel SiO2 nanoparticle-decorated BiOCl nanosheets exhibiting high photocatalytic performances for the removal of organic pollutants. Chinese J. Catal. 40, 1212–1221. doi:10.1016/s1872-2067(19)63359-0
Zhang, D., Tan, G., Wang, M., Li, B., Dang, M., Ren, H., et al. (2020). The modulation of g-C3N4 energy band structure by excitons capture and dissociation. Mater. Res. Bull. 122, 110685. doi:10.1016/j.materresbull.2019.110685
Zhang, P., Li, Y., Zhang, Y., Hou, R., Zhang, X., Xue, C., et al. (2020a). Photogenerated electron transfer process in heterojunctions: in situ irradiation XPS. Small Methods 4, 2000214. doi:10.1002/smtd.202000214
Zhang, P., Tong, Y., Liu, Y., Vequizo, J. J. M., Sun, H., Yang, C., et al. (2020b). Heteroatom dopants promote two-electron O2 reduction for photocatalytic production of H2 O2 on polymeric carbon nitride. Angew. Chem. Int. Ed. Engl. 59, 16209–16217. doi:10.1002/anie.202006747
Zhang, P., Zhang, S., Wan, D., Zhang, P., Zhang, Z., and Shao, G. (2020c). Multilevel polarization-fields enhanced capture and photocatalytic conversion of particulate matter over flexible schottky-junction nanofiber membranes. J. Hazard. Mater. 395, 122639. doi:10.1016/j.jhazmat.2020.122639
Zhang, S., Zhang, P., Hou, R., Li, B., Zhang, Y., Liu, K., et al. (2020). In situ sulfur-doped graphene nanofiber network as efficient metal-free electrocatalyst for polysulfides redox reactions in lithium-sulfur batteries. J. Energy Chem. 47, 281–290. doi:10.1016/j.jechem.2020.01.033
Zhang, Y., Zhang, P., Li, B., Zhang, S., Liu, K., Hou, R., et al. (2020). Vertically aligned graphene nanosheets on multi-yolk/shell structured TiC@C nanofibers for stable Li-S batteries. Energy Storage Mater. 27, 159–168. doi:10.1016/j.ensm.2020.01.029
Zhao, S., Zhang, Y., Zhou, Y., Wang, Y., Qiu, K., Zhang, C., et al. (2018). Facile one-step synthesis of hollow mesoporous g-C3N4 spheres with ultrathin nanosheets for photoredox water splitting. Carbon 126, 247–256. doi:10.1016/j.carbon.2017.10.033
Keywords: g-C3N4, one-dimensional (1D), photocatalytic, template-free, hydrogen evolution
Citation: Zhang M, Sun Y, Chang X and Zhang P (2021) Template-Free Synthesis of One-Dimensional g-C3N4 Chain Nanostructures for Efficient Photocatalytic Hydrogen Evolution. Front. Chem. 9:652762. doi: 10.3389/fchem.2021.652762
Received: 13 January 2021; Accepted: 04 February 2021;
Published: 15 March 2021.
Edited by:
Kangle Lv, South-Central University for Nationalities, ChinaReviewed by:
Kezhen Qi, Shenyang Normal University, ChinaCopyright © 2021 Zhang, Sun, Chang and Zhang. This is an open-access article distributed under the terms of the Creative Commons Attribution License (CC BY). The use, distribution or reproduction in other forums is permitted, provided the original author(s) and the copyright owner(s) are credited and that the original publication in this journal is cited, in accordance with accepted academic practice. No use, distribution or reproduction is permitted which does not comply with these terms.
*Correspondence: Peng Zhang, emhhbmdwQHp6dS5lZHUuY24=
Disclaimer: All claims expressed in this article are solely those of the authors and do not necessarily represent those of their affiliated organizations, or those of the publisher, the editors and the reviewers. Any product that may be evaluated in this article or claim that may be made by its manufacturer is not guaranteed or endorsed by the publisher.
Research integrity at Frontiers
Learn more about the work of our research integrity team to safeguard the quality of each article we publish.