- Key Laboratory of Chemical Biology & Traditional Chinese Medicine Research (Ministry of Education of China), National and Local Joint Engineering Laboratory for New Petrochemical Materials and Fine Utilization of Resources, Key Laboratory of the Assembly and Application of Organic Functional Molecules of Hunan Province, Hunan Normal University, Changsha, China
Inorganic nitride nanomaterials have attracted widespread attention for applications in renewable energy due to novel electrochemical activities and high chemical stabilities. For different renewable energy applications, there are many possibilities and uncertainties about the optimal nitride phases and nanostructures, which further promotes the exploration of controllable preparation of nitride nanomaterials. Moreover, unlike conventional nitrides with bulk or ceramic structures, the synthesis of nitride nanomaterials needs more accurate control to guarantee the target nanostructure along with the phase purity, which make the whole synthesis still a challenge to achieve. In this mini review, we mainly summarize the synthesis methods for inorganic nitride nanomaterials, including chemistry vapor deposition, self-propagation high-temperature synthesis, solid state metathesis reactions, solvothermal synthesis, etc. From the perspective of nanostructure, several novel nitrides, with nanostructures like nanoporous, two-dimensional, defects, ternary structures, and quantum dots, are showing unique properties and getting extensive attentions, recently. Prospects of future research in design and synthesis of functional inorganic nitrides are also discussed.
Introduction
Increasing demands for renewable energy have stimulated the ever-growing developments in the generation of novel energy storage and conversion technologies (Chu et al., 2016; Chen et al., 2020; Vakulchuk et al., 2020). Various inorganic nitride nanomaterials have been designed, for not only conventional applications (E.g., lubricants, cutting materials, semiconductors, luminescent materials, etc.), which also attract increasing interests in renewable energy (Figure 1) and (Supplementary Table S1), such as, electrochemical hydrogen evolution reaction (WN, ZrN) (Wang et al., 2015; Zhong et al., 2016), rechargeable batteries (VN, Mo2N) (Zhang et al., 2019; Tapia-Ruiz et al., 2020), supercapacitors (TiN, NbN, Mn2N3, Ni3N) (Gray et al., 2017; Ghosh et al., 2018), solar cells (Ni3N) (Balogun et al., 2015; Prasad et al., 2018), and fuel cells (ZrN, CoN) (Dong et al., 2013; Yuan et al., 2020). In terms of element composition, functional nitrides mainly include transition metal nitrides and non-metal nitrides. For transition metal nitride nanomaterials, the bonding between metal atoms and nitrogen atoms will change/shrink the d-band structure of host metals, which fundamentally change the activity of catalytic sites and support transition metal nitrides to gain electronic structure and electrocatalytic activity like noble metals. Besides, unique electronic characteristics and strong metal-nitrogen bonds make the nitride anion (N3−) difficult to be substituted, further leading to transition metal nitride nanomaterials show properties like hardness, mechanical stiffness, and high chemical stability. For non-metal nitride nanomaterials (including BN, C3N4 and CxN), typical N configurations (like quaternary N, pyridinic N and pyrrolic N) are formed within the boron or carbon skeleton, of which quaternary N and pyridinic N could show activities for hydrogen evolution reaction (HER) and oxygen evolution reaction (OER) in water splitting, or oxygen reduction reaction (ORR) in proton exchange membrane fuel cells (PEMFCs) (Liu et al., 2015; Niu and Yang, 2018). Moreover, physical and chemical modifications could furtherly support BN obtain tunable band gap or high porosity, which enable those modified BN nanomaterials with various energy storage applications (Han et al., 2020). Above all, inorganic nitride nanomaterials show various possibilities for renewable energy applications.
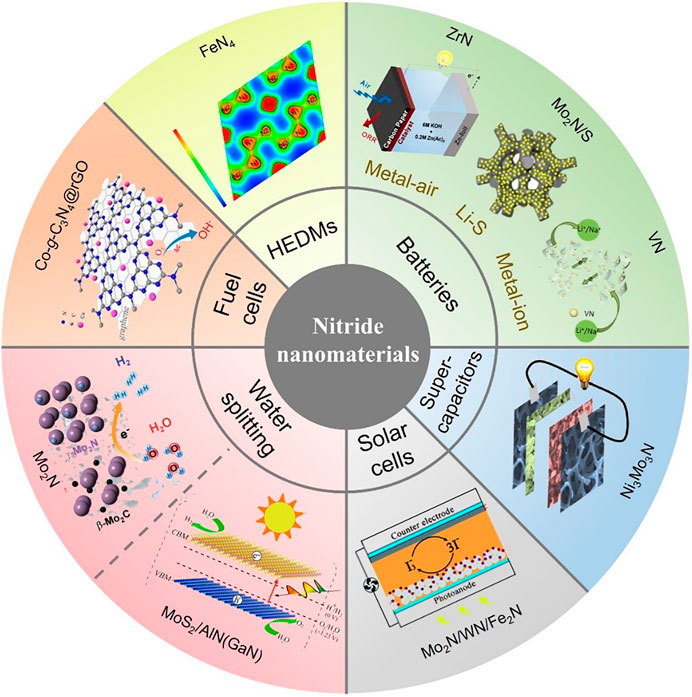
FIGURE 1. Overview of inorganic nitride nanomaterials for renewable energy applications, including ZrN for zinc-air batteries, mesoporpus Mo2N/S for Li-S batteries, hybrid 2D–0D Graphene–VN quantum dots for lithium and sodium ion batteries, Ni3Mo3N3 nanorods for supercapacitors, Mo2N or WN or Fe2N for solar cells solar cells, heterostructural MoS2/AlN(GaN) for photocatalytic water splitting, 3D porous Mo2N for electrocatalytic hydrogen evolution, 2D Co-g-C3N4 bulk for fuel cells and FeN4 as high energy density materials. The inserted graphics are adapted with permission from Li et al. (2011), Chen et al. (2013), Liu and Zhang (2013), Liao et al. (2014), Wang et al. (2016), Bykov et al. (2018), Jiang et al. (2018), Kumar et al. (2020), Yuan et al. (2020).
Over the last decades, the applications of functional nitrides grow with the development of new synthetic routes and analysis technologies (Horvath-Bordon et al., 2006; Mazumder et al., 2008; Zhong et al., 2016; Hong et al., 2020; Li Y. et al., 2020; Wu et al., 2020). Although the fabrication of inorganic nitrides has made significant progress, and many new nitride phases or new nanomaterials or new applications have been produced, the synthesis process for nitrides is always complex and has a large thermodynamic barrier to overcome (945 kJ mol−1 for the formation/break of N≡N bonds, compared to 498 kJ mol−1 for that of O=O bonds) (Balogun et al., 2015). Moreover, for the synthesis of nitride nanomaterials, it is a much more difficult challenge to realize the multi-parameter control of its structure, morphology, size, doping, and defects. In this review, we summarized the mostly used synthesis methods of inorganic nitride bulk-materials or nanomaterials and their corresponding development trends. Some nitride nanomaterials with various morphologies have been presented along with their fabrication operations and the applications as renewable energy. Besides, several unique nanostructures are specially discussed: nanoporous, defects, two-dimensional (2D), ternary nitrides, and quantum dots. Lastly, we conclude with the challenges and chances of precise functionalized inorganic nitrides.
Synthesis Methods for Nitrides
The electrochemical activity and chemical stability of nitride nanomaterials are highly dependent on the structures of related nitride nanomaterials. Therefore, the design and fabrication of high-quality nitride nanomaterials is the initial and key steps to realize their applications for renewable energy. Traditionally, nitrides are generally synthesized under high temperatures (800–1500°C) or high pressure, which makes it easier to obtain nitride materials with bulk or ceramic structures. In order to obtain nanostructured nitrides, active precursors or halide raw-materials (E.g., TiAlNx, SiCl4, BBr3) would be selected, and additives or active intermediates (E.g., S, I2, H2O, NaBH4, NaN3) are always introduced. (Gillan and Kaner, 1996; Spatz et al., 1999; Sardar et al., 2005; Mazumder and Hector, 2009; Yang et al., 2010; Chen et al., 2012; Xu et al., 2012; Zhou et al., 2017). In this work, classic synthesis methods of nitrides are summarized into a few categories (Figure 2).
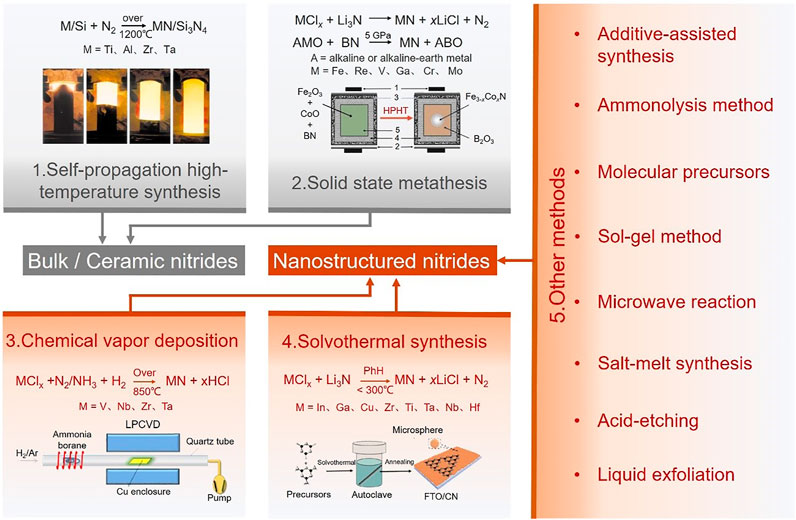
FIGURE 2. Schematic illustration of various synthetic methods to inorganic nitrides, where methods 1 and 2 are mostly used to produce bulk or ceramic nitride materials (marked in grey), and methods 3, 4 and 5 are mostly used to prepare various nitride nanomaterials (marked in red). Method 1 of self-propagation high-temperature synthesis reproduced with the permission from Amiour et al. (2016). Method 2 of solid state metathesis reactions reproduced with the permission from Lei and Zhang (2018). Method 3 of chemistry vapor deposition reproduced with the permission from Song et al. (2015). Method 4 of solvothermal synthesis reproduced with the permission from Xie et al. (2016). Recently, some new synthesis methods for nitride nanomaterials are derived from the above four methods, including additive-assisted synthesis, ammonolysis, molecular precursors method, sol-gel, microwave reaction, salt-melt synthesis, acid-etching and liquid exfoliation.
Self-Propagation High-Temperature Synthesis
The SHS method (or named as combustion synthesis) is used to describe a process in which initial reagents spontaneously transform into products due to the exothermic heat of reaction, once ignited (Subrahmanyam and Vijayakumar, 1992). Due to the fast-release of heat from the exothermic reaction, the time to meet the combustion temperature is short and the conversion rate to products is high (Merzhanov, 2004). For example, a divalent europium-doped nitride phosphors Ca1-xEuxAlSiN3 (x = 0–0.2) were prepared by heating Ca1-xEuxAlSi alloy powders under continuous N2 flow at 1050°C (Piao et al., 2007). Wang’ groups reported the selective synthesis of four types of BN nanotubes by using a porous precursor through the SHS method (Wang et al., 2011). SHS is a practical method for large-scale preparation of nitride powders, but it is difficult to achieve the controllable preparation of nitride nanomaterials.
Solid State Metathesis Reactions
Solid state metathesis has been widely used to produce bulk or nano-sized metal nitrides. The core design of SSM is the replacement reactions between metal halides and alkali (or alkaline earth) nitrides (E.g., Li3N and NaN3), yielding corresponding metal nitrides (Wiley and Kaner, 1992; Zhu et al., 2011; Karaballi et al., 2019), as described in Eq. 1. When producing low thermal stability nitrides (E.g., GaN, InN, Zn3N2, Ta3N5), the synthesis temperature need to be controlled (Gillan and Kaner, 1994).
A high-pressure solid-state metathesis (HPSSM) reaction was reported between boron nitride (BN) and ternary metal oxide AxMyOz (A = alkaline or alkaline-earth metal and M = main group or transition metal), as presented in Eq. 2. Then, a series of well crystallized metal nitrides (Fe3N, Re3N, VN, GaN, CrN, and WxN) were produced (Lei et al., 2013). In subsequent reports, high pressure was confirmed in SSM to benefit the contact diffusion and ion-exchange of the solid reactions (Lei and Zhang, 2018). Well-crystallized WxN nanomaterials were synthesized via the HPSSM, where solid-state ion exchanges and a nitrogen pressure of 5 GPa were involved (Wang et al., 2012). According to DFT calculations of thermodynamic investigations, high pressure could reduce the reaction enthalpy △H.
Moreover, kinetically controlled SSM reactions provide an avenue toward the synthesis of nitride materials (Rognerud et al., 2019). For example, Mn3N2 micro-/nano-crystals were prepared by a kinetically controlled SSM reactions. The further developments of SSM for inorganic nitride nanomaterials would be focused on lower pressure and the kinetic control, where slightly mild and mild kinetics are helpful for the controllable preparation of nanostructures in a exothermic reaction.
Chemistry Vapor Deposition
Chemistry Vapor Deposition (CVD) method is widely favored by chemists, physicists, and material scientists, by which nitride nanomaterials (mainly thin layers, geometric nano-sheets, nano-column, or nano-flowers, etc.) were fabricated by heating volatile metal halides under flow gas (E.g., N2+H2 or NH3+H2, etc.) at high temperatures (above 850°C) (Fix et al., 1993; Zerr et al., 2003), as illustrated in Eq. 3. The replacement of metal chlorides by metal-organic salts could reduce the reaction temperatures to 200–450°C (Fix et al., 1996).
Recent reports focused on the kinetic control and the nano-structural evolutions during the CVD processes. For CVD method, the substrate places an important role for the growth of nitride crystals in terms of the phase and the crystal morphology. In a vapor-liquid-solid case, high-quality GaN nanowires were synthesized via metal-initiated metalorganic CVD (Kuykendall et al., 2003). For another example, high-quality h-BN mono-layer was prepared by using a folded Cu-foil enclosure (Song et al., 2015). Smooth Cu surface effectively reduced the precursor feeding rate, leading to a drastic decrease in the nucleation density. Density functional theory (DFT) calculations demonstrated the crystallographic orientation of the Cu substrate strongly correlated with the orientation of h-BN. Similar phenomena appear in the silicon-assisted growth of centimeter-scale MoSi2N4 monolayers (Hong et al., 2020). It can be predicted that more and more nitrides of the 2D thin-layered family will be synthesized by CVD.
Solvothermal Synthesis
Solvothermal reactions are carried out in organic solvents within an autoclave under a certain temperature and autogenous pressure, where the solvents dissipate the enthalpy of the exothermic metathetical reactions and reduce the diffusion barriers between reactants (Demazeau, 2007). Thus, the solvothermal reactions could be occurred at a relative low temperature and provide better control over the size and morphology of the particles (compared to solid state reactions like SHS or SSM).
In early reports, solvothermal synthesis of nitride nanomaterials mainly explored the regulation of metastable phases, different solvents, and nano morphologies. For example, the reaction between GaCl3 and Li3N in benzene could yield hexagonal/rocksalt mixed-phase GaN nano-crystallites at 280°C (Xie et al., 1996). InN could be synthesized by reactions between InCl3 and Li3N in xylene at 250°C (Demazeau et al., 2002). Later, CrN, ZrN, TiN and NbN were obtained from reactions of chlorides with NaN3 in benzene (Mazumder and Hector, 2009). Similarly, solvothermal methods have been applied to synthesize group 5, 6 metal nitrides in different organic solvents (Mazumder et al., 2008; Bugaris and Ibers, 2010). In recent years, many nitride nanomaterials were fabricated and studied for various renewable energy applications. A Cu3N nanocubes were fabricated in a mixed solvent of ODA and OAm, which exhibited room-temperature ferromagnetism and excellent electrocatalytic activity for ORR and nitrobenzene reduction (Xi et al., 2014). Oxygen-doped g-C3N4 hollow nanospheres were synthesized from acetonitrile solvothermal method at 180°C, which showed remarkably photocatalytic activity for environment pollutant purification and splitting water for HER (Wang et al., 2017). Compared to other methods, solvothermal synthesis is energy-efficient and easy-control. Besides, the reaction is carried out in a closed system, which can effectively prevent the oxidation and uneven growth of nanocrystals, then becoming popular for the synthesis of nitride nanostructures.
Other Synthetic Methods
Besides four traditional synthetic methods, we summarized some other novel methods for inorganic nitride nanomaterials. Generally, thermodynamics driven nanocrystals are easily obtained from additive assisted synthesis; ammonolysis, molecular precursors, sol-gel method and salt-melt synthesis are based on the precursor design with similar principles; microwave synthesis uses the high energy of microwaves for a quickly synthesis; spin-steaming, acid-etching, liquid-exploration, MOFs-assisted synthesis are designed to prepare nitrides with certain characteristic nanostructures (Figure 2).
Additive-Assisted Synthesis
Inspired by SSM and solvothermal reactions, the key to efficient synthesis of nitride crystals is to select thermodynamically spontaneous reactions and raw materials with high activity. In our previous works, a series of nitride (E.g., TiN, BN, AlN, MgSiN2, VN) nanocrystals were prepared from metal oxides with the assistant pre-reactions of metallic Mg (or Na) with H2O (or S) at a low temperature. In detail, TiN, ZrN, BN, and AlN were prepared by using the corresponding elements (Ti, Zr, B, Al), NaN3 and sulfur as starting materials at 250°C (Yang et al., 2010). The exothermic reaction of additives (Na and S) was taken to prepare TiN, BN, AlN, MgSiN2, VN nanocrystals, and the raw materials were metal oxides and NaN3. Due to the additives, the reaction temperature was lowered at 150°C (Chen et al., 2012). And a metal-hydrolysis-assisted synthesis (MHAS) strategy was used to synthesis nitrides (VN, AlN, CrN, MgSiN2etc.) from related metal oxides in a low temperature range (120–180°C) (Zhou et al., 2017). Additive-assisted synthesis is very efficient, but the controllability of nanostructures needs further optimization.
Ammonolysis
Temperature programmed ammonolysis was developed, which involves heating the metal oxide in flowing NH3 and slowly raising the temperature (Hausler and Schnick, 2018). Crystallographic orientations, particle size, and geometry are retained from the reactant solid. For example, S. Imran U. reported an ammonolysis reaction of MoCl5 and [Mo (NMe2)4] to form two different polymeric precursors, where the cubic Mo2N from Mo(NMe2)4 exhibited lattice distortions and significant redox properties as the supercapacitor electrode, and hexagonal Mo2N (observed from the MoCl5) with higher surface areas a had a high capacitance of 275 F g−1 (Shah et al., 2014). Also, binary Ni2Mo3N nanorods were synthesized similarly, which showed excellent electrochemical performance for supercapacitors with a specific capacity of 264 F g−1 at 0.5 A g−1 and 81.4% capacity retention after 1000 cycles (Kumar et al., 2020). Moreover, through this Ammonolysis method, ultrathin 2D GaN (∼2 nm thick) and InN (∼1.3 nm thick) were synthesized from liquid metal (Syed et al., 2019).
Molecular Precursors Method
In this way, nitrogenous precursors or intermediate products were firstly synthesized, then the precursors/intermediates would follow by a low-temperature decomposition and a high-temperature calcination, through which the size and morphology of the nanocrystals can be controlled by adjusting the temperature and the heating time. For example, nitride (ZrN, TiN, NbN) nanomaterials are pyrolyzed by reactions of Zr4+, Ti4+ and Nb5+ dialkylamines with liquid ammonia to produce insoluble precipitates (Brown and Maya, 1988). Also, group 13 nitrides could be obtained by refluxing urea derivatized AlCl3, InCl3 and GaCl3 precursors in TOA, then hexagonal GaN and cubic AlN, InN are generated (Sardar et al., 2005). It is worth learning that Lee used a urea precursor to prepare mesoporous carbon nitride nanostructures with silica nanospheres as a hard template (Lee et al., 2012).
Sol-Gel Processing
Sol-gel methods are wildly used for producing nitride nanomaterials, such as nano-powders or nanoporous structures (Giordano and Antonietti, 2011). During the whole process, ions-organics molecular precursors are formed in solution, then heated the reacted to yield a gel, and finally fired to the target nitrides. For example, TiN nanoparticles (NPs) were coated on carbon fibers via a sol-gel method based on self-condensation of titanium alkyl amide species. In this work, the capacity of the coated fibers was increased with significant redox capacitance contributions (Zhang et al., 2018). In another example, ZrN NPs have been prepared by heating the ZrCl4-Urea sol-gel under an argon flow, which showed better ORR activity than commercial Pt/C catalysts in an alkaline environment (Yuan et al., 2020). In general, the size, morphology, and porosity of the sol-gel prepared nitrides can be controlled by adjusting the organic content, reaction conditions and templates or supports.
Salt-Melt Synthesis
Salt-melt synthesis (SMS) is a special synthesis method in which the reaction happen in the liquid molten salts and products will be obtained by washing the cooled salts when the reaction is completed. There are two main advantages of SMS. For the first, SMS can lower the synthesis temperature and shorten the reaction time. For example, by using the SMS, the size and morphology of the nitride nanoparticles can be well controlled even under lower synthesis temperature (Liu et al., 2013). And secondly, SMS is a convenient and efficient way to fabricate 2D films or 3D porous nanostructures. For example, 3D porous N-doped graphene (HNG) was synthesized by SMS method, which display super bifunctional catalytic activity toward both ORR and OER (Cui et al., 2018). Also, a family of 2D layered transition metal nitrides (TMNs, such as MoN1.2, WN1.5, and Mo0.7W0.3N1.2etc.) could be produced under an atmospheric pressure and showed superior performance in HER (Jin et al., 2020). Lastly, the choice of salts is important in SMS, especially for the large-scale (over decagram) synthesis with High purity requirements.
Microwave Synthesis
Microwave synthesis is a modern synthesis technique under the condition of microwave, which has advantages of rapid heating, homogeneity, and selectivity (Tompsett et al., 2006). Since microwaves can penetrate deep into the substance, it only takes one-tenth to one-hundredth of the time of conventional methods to complete the entire heating process. The size of the nitride products is small and its distribution is uniform. For example, Graphitic carbon nitride (g-C3N4) sub-microspheres were prepared via a facile microwave synthesis through polymerization reaction between C3N3Cl3 and NaN3 in acetonitrile solvent (Dai et al., 2013). Guo et al. produced a g-C3N4 material after optimizing the microwave reaction time which can effectively generate H2 under visible-light irradiation (Guo et al., 2016). In this work, the highest H2 evolution rate achieved was 40.5 mmol h−1, which was two times higher than that of g-C3N4 products prepared by other methods.
In addition to the above methods, two novel synthesis methods of nitride materials have been developed recently. One is acid-etching, E.g., multilayered Ti2N sheets were achieved by the selective etching of ternary layered Ti2AlN with a mixture of potassium fluoride and hydrochloric acid, which showed excellent SERs efficiency (Soundiraraju and George, 2017). Another method named as liquid exfoliation, E.g., atomically-thin MoN nanosheets were obtained by liquid exfoliation of MoN precursors in N methyl-pyrrolidone (NMP) after ultrasound treatment for about 8 h, which had wonderful HER performance (Xie et al., 2014). It is still quite difficult to prepare high-quality nitrides by traditional methods, because the obtained nitrides always contain a certain number of nitrogen vacancies, and the miscibility of products is common. These problems are the same important as the control of nanostructures. In addition, it is undeniable that the high-pressure effect can effectively improve the high temperature thermal stability of nitrides and inhibit the dissipation of nitrogen atoms. In conclusion, the selection of suitable precursors and substrates, and the control of high-pressure reaction path are still the keys for the synthesis of nitride nanomaterials with high-purity.
Controllable Preparation of Specific Nanostructures
Due to the crystalline variety, the obtained morphologies of various nitride crystals are diverse, including nanopyramids, core-shell, nanoporous sheets, nanoflowers, quantum dots, nanotubes, nanoflowers, nanocubes, nanorods, etc. (Supplementary Figure S1) Driven by growth dynamics, BN or CxN with layered structures are generally to form flake-, tubular-, film-, or porous-like nanostructures, while metal nitrides with cubic or tetragonal structures are preferred to form particles, rods, wires, or dendrites. In this section, nitrides with several specific nanostructures (E.g., nanoporous, 2D, defects, ternary, and quantum dots) are specially illustrated in detail (Supplementary Figure S2).
Porous Nitrides
Nanostructured nitrides with a large surface area and pore volume are much expected because the confined-space effects inside the pore-structures would improve their chemical and physical performance to meet the demands of electrode/catalysis materials for renewable energy (Wan et al., 2006) As we known, the soft-templating approach is effective and most wildly used to fabricate ordered micro-/meso-porous metal oxides, metal sulfides, metal phosphates, silicates, and zeolites. However, it is difficult to apply soft-templating approach for the preparation of porous metal nitrides due to the lack of proper raw materials for the sol-gel or molecular precursor processes (Yang and Huang, 2005). Present studies mainly adopted the hard-templating method for the development of nitride porous nanostructures (Wan and Zhao, 2007). For example, GaN, VN, and TiN nanoparticles have been synthesized inside the confinement space of porous templet materials (E.g., ordered mesoporous: MCM-41 and SBA-15; disordered mesoporous CxN), and nitride products with porous composites or isolated nanoparticles would be left after the removal of mesoporous hard templates (Yang and Zhao, 2005; Kogiso et al., 2007; Uedono et al., 2007). Also, self-supported ordered mesoporous metal nitrides (CrN) have been reported (Shi et al., 2008)
Nitrides With Defects
Nitrides with defects have shown many unique functions, where defects in nitrides are detrimental to the electrode or catalytic reactions by working as a recombination center of charge carriers (Van de Walle and Neugebauer, 2004; Wang et al., 2018; Takamura et al., 2020). Reasonable control of defects in nitrides is the key to the excellent performance of these nitrides. Li’ groups proposed a method to control the defects of carbon nitrates, which can effectively influence the defects of PHI-type CN nanocrystals (Li H. et al., 2020). Defects in h-BN are promising single-electron emitter materials with the advantages of high brightness and stability under room temperatures (Abidi et al., 2019). In the process of molten-salt polymerization with melamine as the precursor, KOH were added to realize the nitrogen defect regulation of carbon nitride. For the case of CVD, backside boron gettering technology is used to control the diffusion of B into Cu, thus controlling the defect growth in h-BN (Abidi et al., 2019). In addition, ion implantation can also be used to introduce the boron-vacancy, like ion beams (E.g. nitrogen, xenon, and argon) (Kianinia et al., 2020).
2D Materials
2D nitride materials (E.g., graphene, BN, MoN, and MXene, etc.) have received widespread attentions, because the electron migration and heat diffusion are confined to nano-sized two-dimensional planes, further leading to excellent electrical, optical, and mechanical properties (Naguib et al., 2014; Anasori et al., 2017). For example, 2D transition metal nitrides (MXene) have great application prospects in energy storage, like lithium and sodium ion batteries (Urbankowski et al., 2017). And 2D BN nanomaterials show highly stable structure and unique photoelectric characteristics as graphene, which are mainly prepared by exfoliation, CVD, and vapor phase epitaxy (Zhang et al., 2017). MoN nanosheets was prepared via a liquid exfoliation of annealed and can be used as effective HER electrocatalyst (Xie et al., 2014). Nitride-based MXene was prepared by etching the A atomic layer of the layered precursor MAX phase by HF. For example, 2D Ti4N3 is obtained by etching the Al atomic layer in Ti4AlN3 with molten fluoride under a condition of high temperatures (Soundiraraju and George, 2017). Many 2D nitride nanomaterials show great application potentials in energy storage (Xiao et al., 2019).
Ternary Nitrides
Ternary nitrides are usually formed by combining alkali metals or alkaline earth metals and transition metals with nitrogen elements. In such combinations, the cations can stabilize the high oxidation state N3− anions (Balbarin et al., 1996). No more than 400 kinds of ternary metal nitrides have been synthesized (Sun et al., 2019). Compared with binary nitrides, ternary nitrides exhibit more extensive functions and adjustable plasmonic performance (Ran et al., 2019). As the ammonia generated by ammonolysis can damage the test instrument during characterization process. The common synthetic methods for synthesizing ternary nitrides are: HPSSM, solvothermal, ammonolysis, sol-gel, etc. For example, the Ti0.5Cr0.5N was synthesized by ammonizing a solid metal oxide (ZnMO, M = Cr, Ti). By using the HPHT method, a series of ternary materials (Mo0.23Nb0.77N0.66 and Mo0.66Nb0.34N0.60) were synthesized (Tareen et al., 2019). And a template reaction method was reported to prepare continuously adjustable metal composition ternary nitride nanoparticles (Al-Ga-N and Ti-V-N) by using mesoporous graphite carbon nitride as the nanoreactor and reactant (Fischer et al., 2008). It seems that sol-gel method is preferred for the synthesis of ternary nitrides.
Carbon Nitride Quantum Dots
Graphite phase carbon nitride (g-C3N4) with few nanometers can be named as g-C3N4 quantum dots (g-CNQDs), and they always exhibit enhanced photo-absorption and photo-response comparing with the bulk g-C3N4 materials due to the quantum confinement effects (Albolkany et al., 2020). Because of these properties, g-CNQDs showed wide range of applications that include photocatalysis, fluorescence probes, drug delivery, bioimaging, and security Ink, etc. (Zhang et al., 2014; Yin et al., 2017; Dong et al., 2018; Patir and Gogoi, 2018) Basically, g-CNQDs could be prepared utilizing two methods: the top-to-down way, or the bottom-to-up way. The top-to-down strategy might involve the fragmentation of the bulk g-C3N4 by chemical exfoliation, thermal treatment or ultrasonication into g-CNQDs (Song et al., 2016); and the bottom-to-up strategy is usually based on thermal treatment of nitrogen-rich organic precursors into well dispersed g-CNQDs (Barman and Sadhukhan, 2012; Tang et al., 2014). It can be expected that g-CNQDs will receive growing attentions in the field of functional nanomaterials.
Conclusions and Outlook
Inorganic nitride nanomaterials have excellent corrosion resistance, special semiconductor structures, and abundant active sites, which make inorganic nitride nanomaterials show great potentials for various applications in renewable energy. In this review, we summarize several important synthesis methods of nitrides (E.g., SHS, SSM, CVD, solvothermal, additive-assisted synthesis, sol-gel, molecular precursor, etc.) and some unique structures (E.g., porous, 2D, defects, ternary nitrides, and CxN quantum dots). Also, we briefly analyzed the advantages and disadvantages of some synthesis methods in the preparation of nitride nanomaterials, and discussed the relationship between nanostructures and characteristics for renewable energy applications.
Based on the above analysis, we think that the synthesis of nitride nanomaterials for renewable energy will present the following three aspects in the future research. (Ⅰ) As thermally stable nitrides are always fabricated via above routes from related compounds, it’s still a big challenge to avoid trace impurities and build perfect triple bonds in dinitrogen during the nitride synthesis. Thus, some researchers will focus more on the surface nitridation, surficial atomic composition, and surface activity of functional nitride nanomaterials. (Ⅱ) The synthesis and electrode process of nitride nanomaterials are complex heterogeneous reactions, most of which are reversible or irreversible redox reactions. Therefore, with the help of cryo-electron microscopy, thermal ablation corrected technology, in-situ characterization technologies, we can more closely understand the growth truth of nitride nanomaterials and the origins of their activity/failures during the applications. (Ⅲ) The physical and chemical properties of nitride materials are mainly affected by their crystal structures and electronic structures. And DFT calculations may greatly support the design and preparation of complex nitrides under more precise conditions with higher degree of controllability.
Author Contributions
HZ and LY supervised the implementation of the project. LY and YM conceived the idea. YM, LX, YL, WZ, YY, LM, and LY analysed the data. YM, LY, LX, and YL wrote the manuscript.
Funding
This work was supported by Joint Funds of the National Natural Science Foundation of China (U20A20280), National Natural Science Foundation of China (21805083, 52074119, 21878074), Scientific Research Fund of Hunan Provincial Education Department (19K058) and High-Tech Leading Plan of Hunan Province (2020GK2072).
Conflict of Interest
The authors declare that the research was conducted in the absence of any commercial or financial relationships that could be construed as a potential conflict of interest.
Supplementary Material
The Supplementary Material for this article can be found online at: https://www.frontiersin.org/articles/10.3389/fchem.2021.638216/full#supplementary-material
References
Abidi, I. H., Mendelson, N., Tran, T. T., Tyagi, A., Zhuang, M., Weng, L. T., et al. (2019). Selective Defect Formation in Hexagonal boron Nitride. Adv. Opt. Mater. 7, 1900397–1900405. doi:10.1002/adom.201900397
Albolkany, M. K., Wang, Y., Li, W., Arooj, S., Chen, C. H., Wu, N., et al. (2020). Dual‐Function HKUST‐1: Templating and Catalyzing Formation of Graphitic Carbon Nitride Quantum Dots under Mild Conditions. Angew. Chem. Int. Ed. 59, 21499–21504. doi:10.1002/anie.202009710
Amiour, Y., Zemmour, K., and Vrel, D. (2016). Microstructural and Mechanical Properties of Cu-Based alloy Manufactured by Self-Propagating High-Temperature Synthesis Method. J. Powder. Metall. Min. 05, 1000144. doi:10.4172/2168-9806.1000144
Anasori, B., Lukatskaya, M. R., and Gogotsi, Y. (2017). 2D Metal Carbides and Nitrides (MXenes) for Energy Storage. Nat. Rev. Mater. 2, 16098. doi:10.1038/natrevmats.2016.98
Balbarin, V., Van Dover, R. B., and Disalvo, F. J. (1996). The High Temperature Preparation and Property Measurements of CaTaN2: A Ternary Superconducting Nitride. J. Phys. Chem. Sol. 57, 1919–1927. doi:10.1016/S0022-3697(96)00066-2
Balogun, M. S., Qiu, W., Wang, W., Fang, P., Lu, X., and Tong, Y. (2015). Recent Advances in Metal Nitrides as High-Performance Electrode Materials for Energy Storage Devices. J. Mater. Chem. A. 3, 1364–1387. doi:10.1039/c4ta05565a
Barman, S., and Sadhukhan, M. (2012). Facile Bulk Production of Highly Blue Fluorescent Graphitic Carbon Nitride Quantum Dots and Their Application as Highly Selective and Sensitive Sensors for the Detection of Mercuric and Iodide Ions in Aqueous media. J. Mater. Chem. 22, 21832–21837. doi:10.1039/c2jm35501a
Brown, G. M., and Maya, L. (1988). Ammonolysis Products of the Dialkylamides of Titanium, Zirconium, and Niobium as Precursors to Metal Nitrides. J. Am. Ceram. Soc. 71, 78–82. doi:10.1002/chin.19881436610.1111/j.1151-2916.1988.tb05763.x
Bugaris, D. E., and Ibers, J. A. (2010). Syntheses and Characterization of Some Solid-State Actinide (Th, U, Np) Compounds. Dalton Trans. 39, 5949–5964. doi:10.1039/b927026d
Bykov, M., Bykova, E., Aprilis, G., Glazyrin, K., Koemets, E., Chuvashova, I., et al. (2018). Fe-N System at High Pressure Reveals a Compound Featuring Polymeric Nitrogen Chains. Nat. Commun. 9, 2756. doi:10.1038/s41467-018-05143-2
Chen, B., Yang, L., Heng, H., Chen, J., Zhang, L., Xu, L., et al. (2012). Additive-assisted Synthesis of Boride, Carbide, and Nitride Micro/nanocrystals. J. Solid State Chem. 194, 219–224. doi:10.1016/j.jssc.2012.05.032
Chen, W. F., Iyer, S., Iyer, S., Sasaki, K., Wang, C. H., Zhu, Y., et al. (2013). Biomass-derived Electrocatalytic Composites for Hydrogen Evolution. Energy Environ. Sci. 6, 1818–1826. doi:10.1039/c3ee40596f
Chen, G., Li, Y., Bick, M., and Chen, J. (2020). Smart Textiles for Electricity Generation. Chem. Rev. 120, 3668–3720. doi:10.1021/acs.chemrev.9b00821
Chu, S., Cui, Y., and Liu, N. (2016). The Path towards Sustainable Energy. Nat. Mater 16, 16–22. doi:10.1038/nmat4834
Cui, H., Jiao, M., Chen, Y. N., Guo, Y., Yang, L., Xie, Z., et al. (2018). Molten-salt-assisted Synthesis of 3D Holey N-Doped Graphene as Bifunctional Electrocatalysts for Rechargeable Zn-Air Batteries. Small Methods 2, 1800144. doi:10.1002/smtd.201800144
Dai, H., Gao, X., Liu, E., Yang, Y., Hou, W., Kang, L., et al. (2013). Synthesis and Characterization of Graphitic Carbon Nitride Sub-microspheres Using Microwave Method under Mild Condition. Diamond Relat. Mater. 38, 109–117. doi:10.1016/j.diamond.2013.06.012
Demazeau, G., Goglio, G., Denis, A., and Largeteau, A. (2002). Solvothermal Synthesis: A New Route for Preparing Nitrides. J. Phys. Condens. Matter 14, 11085–11088. doi:10.1088/0953-8984/14/44/430
Demazeau, G. (2007). Solvothermal Reactions: an Original Route for the Synthesis of Novel Materials. J. Mater. Sci. 43, 2104–2114. doi:10.1007/s10853-007-2024-9
Dong, S., Chen, X., Zhang, X., and Cui, G. (2013). Nanostructured Transition Metal Nitrides for Energy Storage and Fuel Cells. Coord. Chem. Rev. 257, 1946–1956. doi:10.1016/j.ccr.2012.12.012
Dong, J., Zhao, Y., Wang, K., Chen, H., Liu, L., Sun, B., et al. (2018). Fabrication of Graphitic Carbon Nitride Quantum Dots and Their Application for Simultaneous Fluorescence Imaging and pH-Responsive Drug Release. Chemistry Select 3, 12696–12703. doi:10.1002/slct.201802492
Fischer, A., Müller, J. O., Antonietti, M., and Thomas, A. (2008). Synthesis of Ternary Metal Nitride Nanoparticles Using Mesoporous Carbon Nitride as Reactive Template. ACS. Nano. 2, 2489–2496. doi:10.1021/nn800503a
Fix, R., Gordon, R. G., and Hoffman, D. M. (1993). Chemical Vapor Deposition of Vanadium, Niobium, and Tantalum Nitride Thin Films. Chem. Mater. 5, 614–619. doi:10.1021/cm00029a007
Fix, R., Gordon, R. G., and Hoffman, D. M. (1996). Low-temperature Atmospheric-Pressure Metal-Organic Chemical Vapor Deposition of Molybdenum Nitride Thin Films. Thin Solid Films 288, 116–119. doi:10.1016/S0040-6090(96)08867-0
Ghosh, S., Jeong, S. M., and Polaki, S. R. (2018). A Review on Metal Nitrides/oxynitrides as an Emerging Supercapacitor Electrode beyond Oxide. Korean J. Chem. Eng. 35, 1389–1408. doi:10.1007/s11814-018-0089-6
Gillan, E. G., and Kaner, R. B. (1994). Rapid Solid-State Synthesis of Refractory Nitrides. Inorg. Chem. 33, 5693–5700. doi:10.1021/ic00103a015
Gillan, E. G., and Kaner, R. B. (1996). Synthesis of Refractory Ceramics via Rapid Metathesis Reactions between Solid-State Precursors. Chem. Mater. 8, 333–343. doi:10.1021/cm950232a
Giordano, C., and Antonietti, M. (2011). Synthesis of Crystalline Metal Nitride and Metal Carbide Nanostructures by Sol-Gel Chemistry. Nano Today 6, 366–380. doi:10.1016/j.nantod.2011.06.002
Gray, B. M., Hector, A. L., Jura, M., Owen, J. R., and Whittam, J. (2017). Effect of Oxidative Surface Treatments on Charge Storage at Titanium Nitride Surfaces for Supercapacitor Applications. J. Mater. Chem. A. 5, 4550–4559. doi:10.1039/c6ta08308k
Guo, Y., Li, J., Yuan, Y., Li, L., Zhang, M., Zhou, C., et al. (2016). A Rapid Microwave-Assisted Thermolysis Route to Highly Crystalline Carbon Nitrides for Efficient Hydrogen Generation. Angew. Chem. Int. Ed. 55, 14693–14697. doi:10.1002/anie.201608453
Han, R., Liu, F., Wang, X., Huang, M., Li, W., Yamauchi, Y., et al. (2020). Functionalised Hexagonal boron Nitride for Energy Conversion and Storage. J. Mater. Chem. A. 8, 14384–14399. doi:10.1039/d0ta05008c
Häusler, J., and Schnick, W. (2018). Ammonothermal Synthesis of Nitrides: Recent Developments and Future Perspectives. Chem. Eur. J. 24, 11864–11879. doi:10.1002/chem.201800115
Hong, Y. L., Liu, Z., Wang, L., Zhou, T., Ma, W., Xu, C., et al. (2020). Chemical Vapor Deposition of Layered Two-Dimensional MoSi2N4 Materials. Science 369, 670–674. doi:10.1126/science.abb7023
Horvath-Bordon, E., Riedel, R., Zerr, A., McMillan, P. F., Auffermann, G., Prots, Y., et al. (2006). High-pressure Chemistry of Nitride-Based Materials. Chem. Soc. Rev. 35, 987–1014. doi:10.1039/b517778m
Jiang, G., Xu, F., Yang, S., Wu, J., Wei, B., and Wang, H. (2018). Mesoporous, Conductive Molybdenum Nitride as Efficient Sulfur Hosts for High-Performance Lithium-Sulfur Batteries. J. Power Sourc. 395, 77–84. doi:10.1016/j.jpowsour.2018.05.061
Jin, H., Gu, Q., Chen, B., Tang, C., Zheng, Y., Zhang, H., et al. (2020). Molten Salt-Directed Catalytic Synthesis of 2D Layered Transition-Metal Nitrides for Efficient Hydrogen Evolution. Chem. 6, 2382–2394. doi:10.1016/j.chempr.2020.06.037
Karaballi, R. A., Humagain, G., Fleischman, B. R. A., and Dasog, M. (2019). Synthesis of Plasmonic Group‐4 Nitride Nanocrystals by Solid‐State Metathesis. Angew. Chem. Int. Ed. 58, 3147–3150. doi:10.1002/anie.201813134
Kianinia, M., White, S., Fröch, J. E., Bradac, C., and Aharonovich, I. (2020). Generation of Spin Defects in Hexagonal boron Nitride. ACS. Photon. 7, 2147–2152. doi:10.1021/acsphotonics.0c00614
Kogiso, M., Zhou, Y., and Shimizu, T. (2007). Instant Preparation of Self-Assembled Metal-Complexed Lipid Nanotubes that Act as Templates to Produce Metal-Oxide Nanotubes. Adv. Mater. 19, 242–246. doi:10.1002/adma.200601117
Kumar, R., Bhuvana, T., and Sharma, A. (2020). Ammonolysis Synthesis of Nickel Molybdenum Nitride Nanostructures for High-Performance Asymmetric Supercapacitors. New J. Chem. 44, 14067–14074. doi:10.1039/d0nj01693d
Kuykendall, T., Pauzauskie, P., Lee, S., Zhang, Y., Goldberger, J., and Yang, P. (2003). Metalorganic Chemical Vapor Deposition Route to GaN Nanowires with Triangular Cross Sections. Nano Lett. 3, 1063–1066. doi:10.1021/nl034422t
Lee, S. C., Lintang, H. O., and Yuliati, L. (2012). A Urea Precursor to Synthesize Carbon Nitride with Mesoporosity for Enhanced Activity in the Photocatalytic Removal of Phenol. Chem. Asian J. 7, 2139–2144. doi:10.1002/asia.201200383
Lei, L., and Zhang, L. (2018). Recent advance in High-Pressure Solid-State Metathesis Reactions. Matter Radiat. Extremes 3, 95–103. doi:10.1016/j.mre.2017.12.003
Lei, L., Yin, W., Jiang, X., Lin, S., and He, D. (2013). Synthetic Route to Metal Nitrides: High-Pressure Solid-State Metathesis Reaction. Inorg. Chem. 52, 13356–13362. doi:10.1021/ic4014834
Li, G. R., Song, J., Pan, G. L., and Gao, X. P. (2011). Highly Pt-like Electrocatalytic Activity of Transition Metal Nitrides for Dye-Sensitized Solar Cells. Energ. Environ. Sci. 4, 1680–1683. doi:10.1039/c1ee01105g
Li, H., Zhu, B., Cao, S., and Yu, J. (2020). Controlling Defects in Crystalline Carbon Nitride to Optimize Photocatalytic CO2 Reduction. Chem. Commun. 56, 5641–5644. doi:10.1039/d0cc01338b
Li, Y., Xiao, K., Huang, C., Wang, J., Gao, M., Hu, A., et al. (2020). Enhanced Potassium-Ion Storage of the 3D Carbon Superstructure by Manipulating the Nitrogen-Doped Species and Morphology. Nano-micro Lett. 13 (1), 1–14. doi:10.1007/s40820-020-00525-y
Liao, J., Sa, B., Zhou, J., Ahuja, R., and Sun, Z. (2014). Design of High-Efficiency Visible-Light Photocatalysts for Water Splitting: MoS2/AlN(GaN) Heterostructures. J. Phys. Chem. C 118, 17594–17599. doi:10.1021/jp5038014
Liu, Q., and Zhang, J. (2013). Graphene Supported Co-g-C3N4 as a Novel Metal-Macrocyclic Electrocatalyst for the Oxygen Reduction Reaction in Fuel Cells. Langmuir 29, 3821–3828. doi:10.1021/la400003h
Liu, X., Fechler, N., and Antonietti, M. (2013). Salt Melt Synthesis of Ceramics, Semiconductors and Carbon Nanostructures. Chem. Soc. Rev. 42, 8237–8265. doi:10.1039/c3cs60159e
Liu, J., Liu, Y., Liu, N., Han, Y., Zhang, X., Huang, H., et al. (2015). Metal-free Efficient Photocatalyst for Stable Visible Water Splitting via a Two-Electron Pathway. Science 347, 970–974. doi:10.1126/science.aaa3145
Mazumder, B., and Hector, A. L. (2009). Synthesis and Applications of Nanocrystalline Nitride Materials. J. Mater. Chem. 19, 4673–4686. doi:10.1039/b817407e
Mazumder, B., Chirico, P., and Hector, A. L. (2008). Direct Solvothermal Synthesis of Early Transition Metal Nitrides. Inorg. Chem. 47, 9684–9690. doi:10.1021/ic800767m
Merzhanov, A. G. (2004). The Chemistry of Self-Propagating High-Temperature Synthesis. J. Mater. Chem. 14, 1779–1786. doi:10.1039/b401358c
Naguib, M., Mochalin, V. N., Barsoum, M. W., and Gogotsi, Y. (2014). 25th Anniversary Article: MXenes: a New Family of Two-Dimensional Materials. Adv. Mater. 26, 992–1005. doi:10.1002/adma.201304138
Niu, W., and Yang, Y. (2018). Graphitic Carbon Nitride for Electrochemical Energy Conversion and Storage. ACS Energ. Lett. 3, 2796–2815. doi:10.1021/acsenergylett.8b01594
Patir, K., and Gogoi, S. K. (2018). Facile Synthesis of Photoluminescent Graphitic Carbon Nitride Quantum Dots for Hg2+ Detection and Room Temperature Phosphorescence. ACS Sustain. Chem. Eng. 6, 1732–1743. doi:10.1021/acssuschemeng.7b03008
Piao, X., Machida, K. I., Horikawa, T., Hanzawa, H., Shimomura, Y., and Kijima, N. (2007). Preparation of CaAlSiN3:Eu2+ Phosphors by the Self-Propagating High-Temperature Synthesis and Their Luminescent Properties. Chem. Mater. 19, 4592–4599. doi:10.1021/cm070623c
Prasad, S., Durai, G., Devaraj, D., AlSalhi, M. S., Theerthagiri, J., Arunachalam, P., et al. (2018). 3D Nanorhombus Nickel Nitride as Stable and Cost-Effective Counter Electrodes for Dye-Sensitized Solar Cells and Supercapacitor Applications. RSC Adv. 8, 8828–8835. doi:10.1039/c8ra00347e
Ran, Y., Lu, H., Zhao, S., Jia, L., Guo, Q., Gao, C., et al. (2019). Structural and Plasmonic Properties of TixZr1−xNy Ternary Nitride Thin Films. Appl. Surf. Sci. 476, 560–568. doi:10.1016/j.apsusc.2019.01.108
Rognerud, E. G., Rom, C. L., Todd, P. K., Singstock, N. R., Bartel, C. J., Holder, A. M., et al. (2019). Kinetically Controlled Low-Temperature Solid-State Metathesis of Manganese Nitride Mn3N2. Chem. Mater. 31, 7248–7254. doi:10.1021/acs.chemmater.9b01565
Sardar, K., Dan, M., Schwenzer, B., and Rao, C. N. R. (2005). A Simple Single-Source Precursor Route to the Nanostructures of AlN, GaN and InN. J. Mater. Chem. 15, 2175–2177. doi:10.1039/b502887f
Shah, S. I. U., Hector, A. L., and Owen, J. R. (2014). Redox Supercapacitor Performance of Nanocrystalline Molybdenum Nitrides Obtained by Ammonolysis of Chloride- and Amide-Derived Precursors. J. Power Sourc. 266, 456–463. doi:10.1016/j.jpowsour.2014.05.045
Shi, Y., Wan, Y., Zhang, R., and Zhao, D. (2008). Synthesis of Self-Supported Ordered Mesoporous Cobalt and Chromium Nitrides. Adv. Funct. Mater. 18, 2436–2443. doi:10.1002/adfm.200800488
Song, X., Gao, J., Nie, Y., Gao, T., Sun, J., Ma, D., et al. (2015). Chemical Vapor Deposition Growth of Large-Scale Hexagonal boron Nitride with Controllable Orientation. Nano Res. 8, 3164–3176. doi:10.1007/s12274-015-0816-9
Song, Z., Lin, T., Lin, L., Lin, S., Fu, F., Wang, X., et al. (2016). Invisible Security Ink Based on Water-Soluble Graphitic Carbon Nitride Quantum Dots. Angew. Chem. Int. Ed. 55, 2773–2777. doi:10.1002/anie.201510945
Soundiraraju, B., and George, B. K. (2017). Two-Dimensional Titanium Nitride (Ti2N) MXene: Synthesis, Characterization, and Potential Application as Surface-Enhanced Raman Scattering Substrate. ACS Nano 11, 8892–8900. doi:10.1021/acsnano.7b03129
Spatz, J. P., Herzog, T., Mößmer, S., Ziemann, P., and Möller, M. (1999). Micellar Inorganic-Polymer Hybrid Systems-A Tool for Nanolithography. Adv. Mater. 11, 149–153. doi:10.1002/(SICI)1521-4095(199902)11:2
Subrahmanyam, J., and Vijayakumar, M. (1992). Self-propagating High-Temperature Synthesis. J. Mater. Sci. 27, 6249–6273. doi:10.1007/BF00576271
Sun, W., Bartel, C. J., Arca, E., Bauers, S. R., Matthews, B., Orvañanos, B., et al. (2019). A Map of the Inorganic Ternary Metal Nitrides. Nat. Mater. 18, 732–739. doi:10.1038/s41563-019-0396-2
Syed, N., Zavabeti, A., Messalea, K. A., Della Gaspera, E., Elbourne, A., Jannat, A., et al. (2019). Wafer-sized Ultrathin Gallium and Indium Nitride Nanosheets through the Ammonolysis of Liquid Metal Derived Oxides. J. Am. Chem. Soc. 141, 104–108. doi:10.1021/jacs.8b11483
Takamura, T., Harada, T., Furuta, T., Ikariya, T., and Kuwata, S. (2020). Front Cover: Half‐Sandwich Iridium Complexes Bearing a Diprotic Glyoxime Ligand: Structural Diversity Induced by Reversible Deprotonation (Chem. Asian J. 1/2020). Chem. Asian J. 15, 1. doi:10.1002/asia.201901486
Tang, Y., Su, Y., Yang, N., Zhang, L., and Lv, Y. (2014). Carbon Nitride Quantum Dots: a Novel Chemiluminescence System for Selective Detection of Free Chlorine in Water. Anal. Chem. 86, 4528–4535. doi:10.1021/ac5005162
Tapia-Ruiz, N., Gordon, A. G., Jewell, C. M., Edwards, H. K., Dunnill, C. W., Blackman, J. M., et al. (2020). Low Dimensional Nanostructures of Fast Ion Conducting Lithium Nitride. Nat. Commun. 11, 4492. doi:10.1038/s41467-020-17951-6
Tareen, A. K., Priyanga, G. S., Behara, S., Thomas, T., and Yang, M. (2019). Mixed Ternary Transition Metal Nitrides: a Comprehensive Review of Synthesis, Electronic Structure, and Properties of Engineering Relevance. Prog. Solid State. Chem. 53, 1–26. doi:10.1016/j.progsolidstchem.2018.11.001
Tompsett, G. A., Conner, W. C., and Yngvesson, K. S. (2006). Microwave Synthesis of Nanoporous Materials. Chem. Eur. J. Chem. Phys. 7, 296–319. doi:10.1002/cphc.200500449
Uedono, A., Ishibashi, S., Chichibu, S. F., and Akimoto, K. (2011). Point Defects in GaN and Related Group-III Nitrides Studied by Means of Positron Annihilation. SPIE, 7939. doi:10.1117/12.871611
Urbankowski, P., Anasori, B., Hantanasirisakul, K., Yang, L., Zhang, L., Haines, B., et al. (2017). 2D Molybdenum and Vanadium Nitrides Synthesized by Ammoniation of 2D Transition Metal Carbides (MXenes). Nanoscale 9, 17722–17730. doi:10.1039/c7nr06721f
Vakulchuk, R., Overland, I., and Scholten, D. (2020). Renewable Energy and Geopolitics: A Review. Renew. Sustain. Energ. Rev. 122, 109547. doi:10.1016/j.rser.2019.109547
Van de Walle, C. G., and Neugebauer, J. (2004). First-principles Calculations for Defects and Impurities: Applications to III-Nitrides. J. Appl. Phys. 95, 3851–3879. doi:10.1063/1.1682673
Wan, Y., and Zhao, D. (2007). On the Controllable Soft-Templating Approach to Mesoporous Silicates. Chem. Rev. 107, 2821–2860. doi:10.1021/cr068020s
Wan, Y., Yang, H., and Zhao, D. (2006). "Host−Guest" Chemistry in the Synthesis of Ordered Nonsiliceous Mesoporous Materials. Acc. Chem. Res. 39, 423–432. doi:10.1021/ar050091a
Wang, J., Zhang, L., Zhao, G., Gu, Y., Zhang, Z., Zhang, F., et al. (2011). Selective Synthesis of boron Nitride Nanotubes by Self-Propagation High-Temperature Synthesis and Annealing Process. J. Solid State. Chem. 184, 2478–2484. doi:10.1016/j.jssc.2011.07.026
Wang, S., Yu, X., Lin, Z., Zhang, R., He, D., Qin, J., et al. (2012). Synthesis, crystal Structure, and Elastic Properties of Novel Tungsten Nitrides. Chem. Mater. 24, 3023–3028. doi:10.1021/cm301516w
Wang, S., Ge, H., Sun, S., Zhang, J., Liu, F., Wen, X., et al. (2015). A New Molybdenum Nitride Catalyst with Rhombohedral MoS2 Structure for Hydrogenation Applications. J. Am. Chem. Soc. 137, 4815–4822. doi:10.1021/jacs.5b01446
Wang, L., Sun, J., Song, R., Yang, S., and Song, H. (2016). Hybrid 2D-0D Graphene-VN Quantum Dots for Superior Lithium and Sodium Storage. Adv. Energ. Mater. 6, 1502067. doi:10.1002/aenm.201502067
Wang, Y., Wang, H., Chen, F., Cao, F., Zhao, X., Meng, S., et al. (2017). Facile Synthesis of Oxygen Doped Carbon Nitride Hollow Microsphere for Photocatalysis. Appl. Catal. B: Environ. 206, 417–425. doi:10.1016/j.apcatb.2017.01.041
Wang, Q., Zhang, Q., Zhao, X., Luo, X., Wong, C. P. Y., Wang, J., et al. (2018). Photoluminescence Upconversion by Defects in Hexagonal boron Nitride. Nano Lett. 18, 6898–6905. doi:10.1021/acs.nanolett.8b02804
Wiley, J. B., and Kaner, R. B. (1992). Rapid Solid-State Precursor Synthesis of Materials. Science 255, 1093–1097. doi:10.1126/science.255.5048.1093
Wu, L., Li, Y., Fu, Z., and Su, B. L. (2020). Hierarchically Structured Porous Materials: Synthesis Strategies and Applications in Energy Storage. Natl. Sci. Rev. 7, 1667–1701. doi:10.1093/nsr/nwaa183
Xi, P., Xu, Z., Gao, D., Chen, F., Xue, D., Tao, C. L., et al. (2014). Solvothermal Synthesis of Magnetic Copper Nitride Nanocubes with Highly Electrocatalytic Reduction Properties. RSC Adv. 4, 14206–14209. doi:10.1039/c4ra01307g
Xiao, X., Wang, H., Bao, W., Urbankowski, P., Yang, L., Yang, Y., et al. (2019). Two‐Dimensional Arrays of Transition Metal Nitride Nanocrystals. Adv. Mater. 31, 1902393. doi:10.1002/adma.201902393
Xie, J., Li, S., Zhang, X., Zhang, J., Wang, R., Zhang, H., et al. (2014). Atomically-thin Molybdenum Nitride Nanosheets with Exposed Active Surface Sites for Efficient Hydrogen Evolution. Chem. Sci. 5, 4615–4620. doi:10.1039/c4sc02019g
Xie, X., Fan, X., Huang, X., Wang, T., and He, J. (2016). In Situ growth of Graphitic Carbon Nitride Films on Transparent Conducting Substrates via a Solvothermal Route for Photoelectrochemical Performance. RSC Adv. 6, 9916–9922. doi:10.1039/c5ra21228f
Xie, Y., Qian, Y., Wang, W., Zhang, S., and Zhang, Y. (1996). A benzene-thermal synthetic route to nanocrystalline GaN. Science 272, 1926. doi:10.1126/science.272.5270.1926
Xu, L., Li, S., Zhang, Y., and Zhai, Y. (2012). Synthesis, Properties and Applications of Nanoscale Nitrides, Borides and Carbides. Nanoscale 4, 4900. doi:10.1039/c2nr30598d
Yang, C. T., and Huang, M. H. (2005). Formation of Arrays of Gallium Nitride Nanorods within Mesoporous Silica SBA-15. J. Phys. Chem. B 109, 17842–17847. doi:10.1021/jp052228k
Yang, H., and Zhao, D. (2005). Synthesis of Replica Mesostructures by the Nanocasting Strategy. J. Mater. Chem. 15, 1217–1231. doi:10.1039/b414402c
Yang, L., Yu, H., Xu, L., Ma, Q., and Qian, Y. (2010). Sulfur-assisted Synthesis of Nitride Nanocrystals. Dalton Trans. 39, 2855–2860. doi:10.1039/b920429f
Yin, Y., Zhang, Y., Gao, T., Yao, T., Han, J., Han, Z., et al. (2017). One-pot Evaporation-Condensation Strategy for green Synthesis of Carbon Nitride Quantum Dots: An Efficient Fluorescent Probe for Ion Detection and Bioimaging. Mater. Chem. Phys. 194, 293–301. doi:10.1016/j.matchemphys.2017.03.054
Yuan, Y., Wang, J., Adimi, S., Shen, H., Thomas, T., Ma, R., et al. (2020). Zirconium Nitride Catalysts Surpass Platinum for Oxygen Reduction. Nat. Mater. 19, 282–286. doi:10.1038/s41563-019-0535-9
Zerr, A., Miehe, G., and Riedel, R. (2003). Synthesis of Cubic Zirconium and Hafnium Nitride Having Th3P4 Structure. Nat. Mater. 2, 185–189. doi:10.1038/nmat836
Zhang, S., Li, J., Zeng, M., Xu, J., Wang, X., and Hu, W. (2014). Polymer Nanodots of Graphitic Carbon Nitride as Effective Fluorescent Probes for the Detection of Fe3+ and Cu2+ Ions. Nanoscale 6, 4157–4162. doi:10.1039/c3nr06744k
Zhang, K., Feng, Y., Wang, F., Yang, Z., and Wang, J. (2017). Two Dimensional Hexagonal boron Nitride (2D-hBN): Synthesis, Properties and Applications. J. Mater. Chem. C 5, 11992–12022. doi:10.1039/c7tc04300g
Zhang, J., Hector, A. L., Soulé, S., Zhang, Q., and Zhao, X. (2018). Effects of Ammonolysis and of Sol-Gel Titanium Oxide Nitride Coating on Carbon Fibres for Use in Flexible Supercapacitors. J. Mater. Chem. A. 6, 5208–5216. doi:10.1039/c7ta11142h
Zhang, H., Tian, D., Zhao, Z., Liu, X., Hou, Y. N., Tang, Y., et al. (2019). Cobalt Nitride Nanoparticles Embedded in Porous Carbon Nanosheet Arrays Propelling Polysulfides Conversion for Highly Stable Lithium-Sulfur Batteries. Energ. Storage Mater. 21, 210–218. doi:10.1016/j.ensm.2018.12.005
Zhong, Y., Xia, X., Shi, F., Zhan, J., Tu, J., and Fan, H. J. (2016). Transition Metal Carbides and Nitrides in Energy Storage and Conversion. Adv. Sci. 3, 1500286. doi:10.1002/advs.201500286
Zhou, L., Yang, L., Shao, L., Chen, B., Meng, F., Qian, Y., et al. (2017). General Fabrication of Boride, Carbide, and Nitride Nanocrystals via a Metal-Hydrolysis-Assisted Process. Inorg. Chem. 56, 2440–2447. doi:10.1021/acs.inorgchem.6b02501
Keywords: nitride nanomaterials, controllable synthesis, energy storage, two-dimensional materials, defects
Citation: Ma Y, Xiong L, Lu Y, Zhu W, Zhao H, Yang Y, Mao L and Yang L (2021) Advanced Inorganic Nitride Nanomaterials for Renewable Energy: A Mini Review of Synthesis Methods. Front. Chem. 9:638216. doi: 10.3389/fchem.2021.638216
Received: 05 December 2020; Accepted: 12 May 2021;
Published: 09 July 2021.
Edited by:
Winnie Kwai-Wah Wong-Ng, National Institute of Standards and Technology, United StatesReviewed by:
Karthik Ramasamy, UbiQD, Inc., United StatesQiyuan He, City University of Hong Kong, China
Copyright © 2021 Ma, Xiong, Lu, Zhu, Zhao, Yang, Mao and Yang. This is an open-access article distributed under the terms of the Creative Commons Attribution License (CC BY). The use, distribution or reproduction in other forums is permitted, provided the original author(s) and the copyright owner(s) are credited and that the original publication in this journal is cited, in accordance with accepted academic practice. No use, distribution or reproduction is permitted which does not comply with these terms.
*Correspondence: Haihong Zhao, emhoXzE5OThAMTYzLmNvbQ==; Lishan Yang, bHN5YW5nLmNoZW1pc3RyeUBnbWFpbC5jb20=