- 1State Environmental Protection Key Laboratory of Soil Environmental Management and Pollution Control, Nanjing Institute of Environmental Sciences, Ministry of Ecology and Environment, Nanjing, China
- 2College of Environment, Hohai University, Nanjing, China
Although pesticide intermediates are a kind of typical toxic pollutant in contaminated sites, the remediation of these contaminants in groundwater and soils is of limited concern. In the present study we investigated the performance of a novel heterogeneous oxidation system, biochar supported nanoscaled-zero valent iron (nZVI/BC) activated persulfate (PS), in the oxidative degradation of nitrochlorobenzene (NCB), a typical pesticide intermediate. Peanut shell based nZVI/BC was prepared and used as the PS activator. The degradation kinetics of m-, p-, and o-NCB isomers in the aqueous phase were investigated. The effects of BC/nZVI composition (Fe/BC mass ratio), the amount of BC/nZVI and PS, and initial contaminant concentration on NCB removal were also examined. Results suggest that over 90% removals of three NCB isomers could be obtained by the nZVI/BC activated PS system at initial NCB concentration of 10 mg L−1. The combination of nZVI/BC composite and PS showed superior performance to PS alone. The optimal treatment condition was supposed as the Fe: BC ratio of 1:1, Fe amount of 6 mmol L−1, and the mole ratio of Fe to perfulfate of 1:1.
1 Introduction
Nitrochlorobenzene (NCB) is a kind of chlorine-containing nitro aromatic hydrocarbon compound, which is widely used as a basic chemical in dyeing, explosives, pesticides, and chemical synthesis industries (Li and Zhu, 2014; Wu et al., 2014; Vahid et al., 2015; Zhang et al., 2019). The world's annual discharge of NCB compounds into the environment is about 30, 000 tons. China is one of the countries with the largest production of ortho and para-nitrochlorobenzene in the world (Chen et al., 2018). NCB has strong carcinogenic and mutagenicity, a very stable structure, and it is not easily decomposed and transformed in the environment. NCB can enter the human body and animals through inhalation, ingestion, and skin absorption, causing tissue lesions in the lungs, breast, liver, kidneys and other organs, accompanied by clinical symptoms such as mental disorders and coma (Le et al., 2011). Therefore, NCB has been listed as the environmental priority control pollutants by the United States EPA and China SEPA.
Varied physical, chemical, and biological technologies have been explored to treat NCB in water (Le et al., 2011; Li and Zhu, 2014; Huang et al., 2016; Liu et al., 2016; Chen et al., 2018; Song et al., 2020). Among which advanced oxidation technology (AOT) is more reliable due to its high degradation efficacy and lower secondary pollution. Persulfate oxidation, as a new and green AOT, has been increasingly investigated and widely used for in situ chemical oxidation (ISCO) (Liu et al., 2015; Devi et al., 2016; Fang et al., 2017; Zhu et al., 2017; Park et al., 2019; Khashij et al., 2020; Lee, et al., 2020). Persulfate is stable, and has a much longer lifetime in the subsurface than hydrogen peroxide. To promote the performance of persulfate, various activation methods are usually applied to activate persulfate to generate reactive radical species, mainly sulfate radicals and hydroxyl radicals, which can oxidize most recalcitrant organic contaminants (Fang et al., 2017; Ghanbari and Moradi, 2017; Zhu et al., 2017). Heat-activation, alkali activation, and transition metals activation are most frequently used.
Very recently a novel activation method, persulfate activated by biochar supported nano zero valent iron (nZVI/BC), was investigated, and exciting results were found in the treatment of chlorinated organics (Yan et al., 2015; Yan et al., 2017; Xu et al., 2018; Hayat et al., 2019; Luo et al., 2019). Yan et al. (2015) synthesized nZVI/BC composite as an activator for persulfate to enhance the removal of trichloroethylene (TCE) in aqueous solutions. Reliable treatment performance was obtained, much higher than that of nZVI/BC or persulfate alone. Similar results were reported for the degradation of nonylphenol, imidacloprid and diatrizoate (Hussain et al., 2017; Xu et al., 2018; Luo et al., 2019). As demonstrated by the following process in Eqs 1–3, the enhanced formation of the SO−4. was detected and supposed as the main mechanism for the accelerated degradation of pollutants. However, the application of the above promising persulfate activation technique in the treatment of NCB has not been mentioned.
The present study aimed at exploring the feasibility of treating three NCB isomers by nZVI/BC activated PS. Various parameters including the BC-Fe ratio, the dosage of nZVI/BC and PS, as well as the initial NCB concentration were examined. Both degradation percentage and kinetics of NCB were analyzed and compared to obtain the optimal reaction conditions.
2 Materials and Methods
2.1 Materials
Ferrous sulfate heptahydrate was purchased from Sinopharm Chemical Reagent Co., Ltd., China. Sodium borohydride was purchased from J&K Chemicals. Sodium persulfate (>98%) was from Shanghai Lingfeng Chemical Reagent Co. Ltd. O-NCB (>99%, GC grade), p-NCB (>99.5%, GC grade) and m-NCB (>98%, chemical pure) were all provided by Aladdin (Shanghai) Reagent Co. Ltd. Absolute ethanol and hexane were both chromatographically pure and obtained from Merk. The experimental ultrapure water was provided by Millipore pure water system. Other reagents are of analytical grade.
2.2 Preparation of nZVI/BC Composite
The preparation of peanut shell-derived biochar followed the procedure that was mentioned before (Wang et al., 2019). In brief, peanut shell was cleaned by water, dried (70°C, 24 h) and broken into about 2 mm strips, then pyrolyzed in a muffle at 500°C under limited oxygen condition for 2 h, then ground and passed through a 0.25 mm sieve. The nZVI/BC composite was then prepared using the liquid phase reduction method. To begin with, 5 g of ferrous sulfate heptahydrate was weighted and dissolved in 250 ml of 30% ethanol solution (volume fraction) in a three-necked flask. A certain amount of biochar (2 g) was added to the three-necked flask and mixed with ferrous sulfate heptahydrate solution, and nitrogen was then purged into the mixed solution to exclude the oxygen during the whole preparation procedure. Following, 100 ml of sodium borohydride solution at 0.395 mol L−1 was added dropwise through a constant pressure funnel, maintained at 1–2 drop s−1. With strong stirring by an electric stirring device, nZVI deposited on the surface of BC. After the completion of sodium borohydride addition, the solution was further stirred for 30 min with the nitrogen purging. The obtained nZVI/BC particles were settled and separated from the liquid by a positive pressure filter under an atmosphere of nitrogen, then washed with deionized water and anhydrous ethanol at least three times, then transferred to a screw-top reagent bottle, and dried in the oven at 70°C under protection of nitrogen, and stored under anaerobic conditions for use.
2.3 Batch Treatment Experiments
Batch aqueous tests were performed in 40 ml stoppered glass tubes with Teflon-lined screw caps. To begin with, 15 ml of NCB water solution (with initial concentration of each NCB was 5, 10, or 20 mg L−1) and 15 ml of sodium persulfate (with the amount of 3, 6, or 12 mmol) was added sequentially to the tubes and mixed with certain amount nZVI/BC particles (with Fe amount of 3 or 6 mmol). The tubes were placed in a rotary shaker (25 ± 1°C, 150 rpm). At regular time intervals, three tubes were sampled and sacrificed for analysis. The mixture was centrifugated at 3,000 rpm for 5 min to separate the solution from solid particles. Then 10 ml of solution phase was transferred and extracted with 10 ml of hexane. The composite particles were mixed with 2 ml ethanol and 8 ml hexane and extracted in a rotary shaker for 2 h, centrifugated and filtered. The organic phase was subjected for analysis. Triplicate samples were prepared for each test.
2.4 Analysis Method
The organic extractant was subjected for OCPs analysis on an Agilent 6890N gas chromatograph analyzer equipped with an electron capture detector and a HP-5 capillary column (30.0 mm × 0.32 mm × 0.25 µm). The injector and detector temperatures were 280 and 300°C, respectively, and the injection volume was 1.0 µl. The carrier gas flow rate (99.999% nitrogen) was 1.0 ml min−1. The heating procedure was as follows: the oven was heated to 90°C, held for 0.5 min and then heated from 90 to 110°C at a rate of 10°C min−1, and from 110 to 150 at a rate of 10°C min−1. Finally, the oven was heated to 270°C at a rate of 20°C min−1 and then held for 1 min.
2.5 Statistical Analysis
All data come from the average value of three repeated samples, statistically analyzed by SPSS 13.0 software. Data were analyzed by two way analysis of variance. The differences identified were pinpointed by an unpaired Student’s t-test. Mean values were compared by least significant difference at probability level≤5%. All charts were made by Microsoft Excel 2013 and Origin 2018 software.
3 Results and Discussion
3.1 Characteristics of BC and nZVI/BC
The composition of BC was analyzed on a Vario EL III Elemental Analyzer (German elementary company). The contents of C, H, O, and N were 61.3%, 2.69, 12.0, and 1.66%, respectively. The specific surface area of nZVI and nZVI/BC obtained from ASAP 2010N specific surface area analyzer were 34.2 and 6.3 m2 g-1, respectively.
Scanning electron microscope (SEM) was performed to examine the morphologies of BC, nZVI, and nZVI/BC on a JSM-7600F scanning electron microscope at an accelerating voltage of 10 kV. It can be observed from the SEM characterization image (Figure 1A) that the peanut-derived BC was of laminated structure with rough and porous surface morphologies. The nZVI was composed of fine spherical particles, and was severely agglomerated (Figure 1B). For the nZVI/BC composites, the nZVI particles were homogeneously distributed across the entire BC surface, forming a chain-like structure (Figure 1C). In addition, the diameters of spherical nZVI particles were about 30–40 nm.
3.2 NCB Degradation by Varied Materials
3.2.1 PS Activation by Different nZVI/BC Materials
The effect of nZVI/BC composition on the activation of PS was examined. Two different nZVI/BC particles, with mass ratio of nZVI to BC of 1:1 and 1:4, were tested. The amount of nZVI/BC was 0.01 g, and the reaction time was 2 h. As demonstrated by Figure 2, the system with a higher Fe/BC ratio of 1:1 had statistically higher removal rates for all three NCB isomers compared to that of 1:4. Yan et al. (2015) reported a desired nZVI to BC mass ratio of 1:5, wherein the degradation of TCE increased as the nZVI/BC ratio increasing from 1:1 to 1:5, and decreased when the ratio further increased to 1:7. In another study by Hussain et al. (2017), the optimal nZVI/BC ratio for activation PS was found as 1:3, the degradation of nonylphenol at this ratio was superior to 1:1, 1:2, and 1:4. The activation capacity of nZVI/BC composite was suggested to be associated with their surface area that provided more reactive sites of Fe to activate PS. However, excessive BC may block the reactive sites of the iron surface and cause the aggregation of BC sheets (Hussain et al., 2017). It could be inferred that the optimal composition of nZVI and BC relates with the different characteristics of both BC (peanut shell-derived or rice straw-derived) and target contaminants.
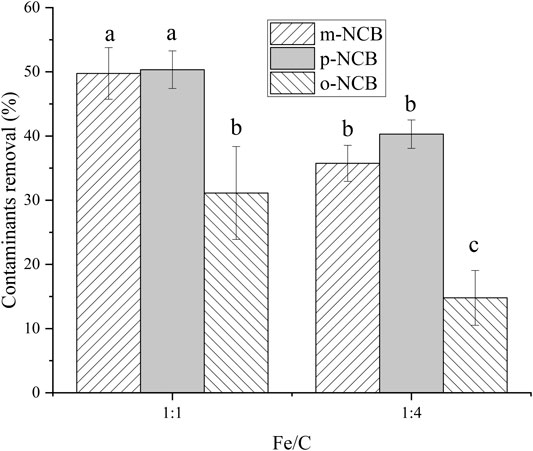
FIGURE 2. Effect of Fe/C in composite on NCB degradation. The nZVI/BC amount 0.01 g, Fe/PS molar ratio 1:1, the initial NCB isomer concentration 10 mg L−1, and the reaction time 2 h.
3.2.2 Effect of nZVI/BC and PS Dosage
The effect of nZVI/BC and PS amounts on NCB degradation was further investigated. 0.005–0.02 g of composite were used, and the Fe to PS molar ratio was kept as 1:1. As showed in Figure 3, no reliable removal of NCB was observed when 0.005 g of composite was added, especially for m- and o-NCB, wherein lower than 10% of removal were obtained. With the amount of nZVI/BC increasing to 0.01 and 0.02 g, the contaminants removal increased significantly. For instance, when 0.02 g of nZVI/BC (6 mmol L−1 of Fe) was added, the degradation rate of m-, p- and o-NCB reached 82.4%, 78.8%, and 73.3%, respectively. Higher activator and PS dosage meant more oxidation free radicals being formed and therefore, higher degradation efficiencies for contaminants (Luo et al., 2019). It is indicated that to achieve a desirable contaminant degradation rate, an amount of nZVI/BC of 0.02 g was required.
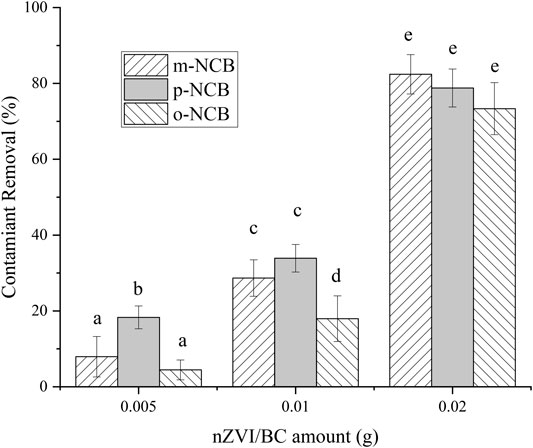
FIGURE 3. NCB degradation by different amount of nZVI/BC and PS. The Fe/C ratio 1:1, Fe/PS molar ratio 1:1, the initial NCB isomer concentration 10 mg L−1, and the reaction time 1 h.
3.2.3 NCB Degradation by Combined and Individual Systems
Figure 4 reveals different removal performances of NCB in varied treatment systems, i.e., nZVI/BC, PS, and nZVI/BC activated PS. For all of the three treatments, robust contaminant removals were evidenced. It is suggested that the combination of nZVI/BC and PS exhibited superior removal efficacy to the system of PS alone. The promotive effect of nZVI/BC on PS oxidation has been verified in other studies (Yan et al., 2015; Luo et al., 2019). The promoted formation of SO4·-, which was detected as the primary active group, was supposed responsible for the accelerated degradation of NCB (Kang et al., 2019). Furthermore, both the Fe(II)/Fe(III) redox reaction induced by nZVI and the electron-transfer mediator of the BC oxygen functional groups were suggested to be related with promoting the generation of SO4·- in the combined nZVI/BC-PS system (Luo et al., 2019). Figure 2 suggests that no significant difference in NCB removal was obtained between nZVI/BC-PS and nZVI/BC anole system. Nevertheless, it should be noted that the oxidation reaction induced by nZVI/BC-PS could degrade the contaminants more thoroughly, which was comparatively superior to the reductive process by zero valent iron (Li et al., 2020). Herein, in the nZVI/BC-PS system, NCB could be reduced by nZVI to chloroaniline, and then oxidized by sulfate radicals to benzoquinone and finally been mineralized as carbon dioxide and water (Kang et al., 2019).
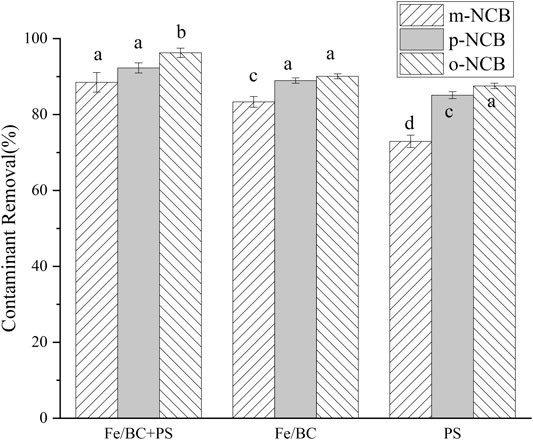
FIGURE 4. NCB degradation in different systems. The nZVI/BC amount 0.02 g, Fe/PS molar ratio 1:1, the initial NCB isomer concentration 10 mg L−1, and the reaction time 2 h.
3.3 Effect of nZVI/BC Amount on NCB Degradation
Figure 5 depicts the degradation kinetics of three NCB isomers by addition of different amount of PS activator (0.01 g vs. 0.02 g of nZVI/BC, corresponding to 3 and 6 mmol L−1 of Fe). It can be seen from Figure 5 that for all six groups tests, the degradation efficacies of NCB increased with the reaction time prolonging in the range of 0–240 min. Approximately 50% of m- and p-NCB were removed with 240 min when 0.01 g of nZVI/BC was used to activate PS. In comparison, significantly (p < 0.05) higher removal was obtained when nZVI/BC dosage was 0.02 g, wherein over 90% of NCB were degraded. The results were consistent with those in Section 3.2.2 and reported in other studies (Yan et al., 2015; Hussain et al., 2017). An increased dosage of nZVI/BC contributed to more active sites for PS decomposition, producing more SO4·- and increasing the efficiency of NCB degradation (Kang et al., 2019).
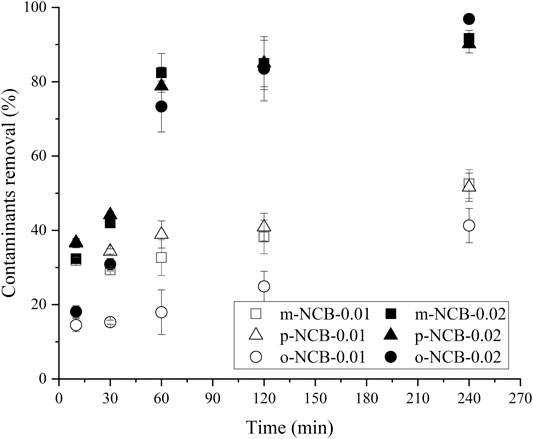
FIGURE 5. Effect of activator dosage on NCB degradation. The Fe/PS molar ratio 1:1, the initial NCB isomer concentration 10 mg L−1.
The much more rapid degradation of NCB at higher activator dosage can be also verified by the larger degradation first order kinetic constant (kobs), as showed in Table 1. In general, the kobs at 0.02 g of Fe/BC were 5–11.75 times of those at 0.01 g, depending on the types of NCB isomers.
3.4 Effect of Fe:PS Ratio on NCB Degradation
The influence of Fe: PS molar ratio on NCB degradation was further investigated. The amount of nZVI/BC was kept as 0.02 g, i.e., the dosage of Fe was 6 mmol/L. Generally, for either p- or o-NCB, insignificant difference in contaminant degradation was attained among three Fe:PS ratio values (p > 0.05, Figure 5). However, the lower Fe/PS of 0.5 showed reduced degradation of m-NCB relative to Fe/PS of 2 and 1. For instance, the 4 h-removal of m-NCB for 1:1 Fe/PS group was 86.3%, while that for 1:2 Fe/PS group was 71.2%. It should be mentioned that herein the amount of Fe was constant, a lower Fe/PS value meant more or excess PS was initially present in the system, which might lead to the excessive scavenging of PS by itself, unfavorable to the oxidation of the contaminant (Hussain et al., 2017; Hayat et al., 2019). Yan et al. (2015) also reported a decreased TCE removal at lower nZVI/BC concentration beyond 4.5 mmol/L. The possible scavenging between SO4·- species themselves was proposed, as established in Eqs 4, 5. In another study, lower degradation of imidacloprid over higher nZVI dosage (above 3 g/L) or higher PS concentration (above 5 mmol/L) was evidenced, the self-scavenging caused by excess SO4·-−PS decomposition was investigated by detection of the concentration of PS anion during degradation experiments over applied concentrations of PS (Hayat et al., 2019).
3.5 Effect of NCB Initial Concentration
The influence of initial NCB concentration on their degradation was further investigated. The amount of nZVI/BC and PS was kept as 0.02 g and 6 mmol L−1, respectively. It can be seen from Figure 6 that the removal performance of NCB was significantly affected when the initial NCB concentration increased to 20 mg L−1 (p < 0.05). In particular, the removal rate for p-NCB was less than 30% within 480 min, while those were over 88% at initial concentrations of 5 and 10 mg L−1. Similar results have also been reported Liu et al. (2016), wherein insignificant concentration effect on the degradation of o-NCB at low o-NCB concentrations was found. As the formation of Fe2+ and SO4·- are initially rapid in the nZVI/PS system, the degradation over lower concentrations of NCB could be very fast. However, the concentration of racial became lower over higher NCB concentrations, thereby inducing a slower degradation rate.
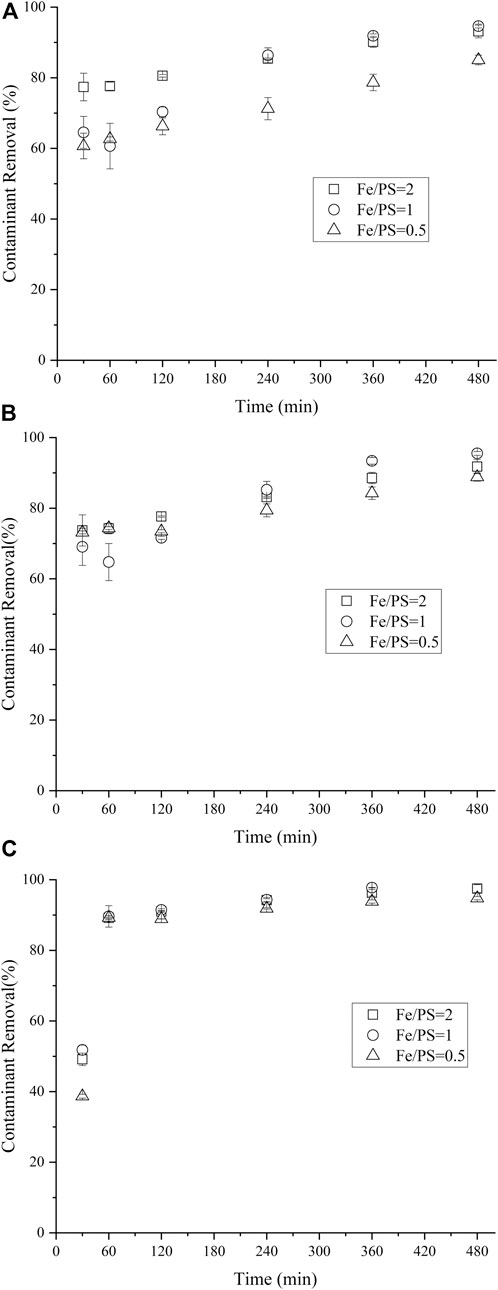
FIGURE 6. Effect of mole ratio of Fe/PS on m-NCB (A), P-NCB (B) and o-NCB (C) degradation. The nZVI/BC amount 0.02 g, the initial NCB isomer concentration 10 mg L−1.
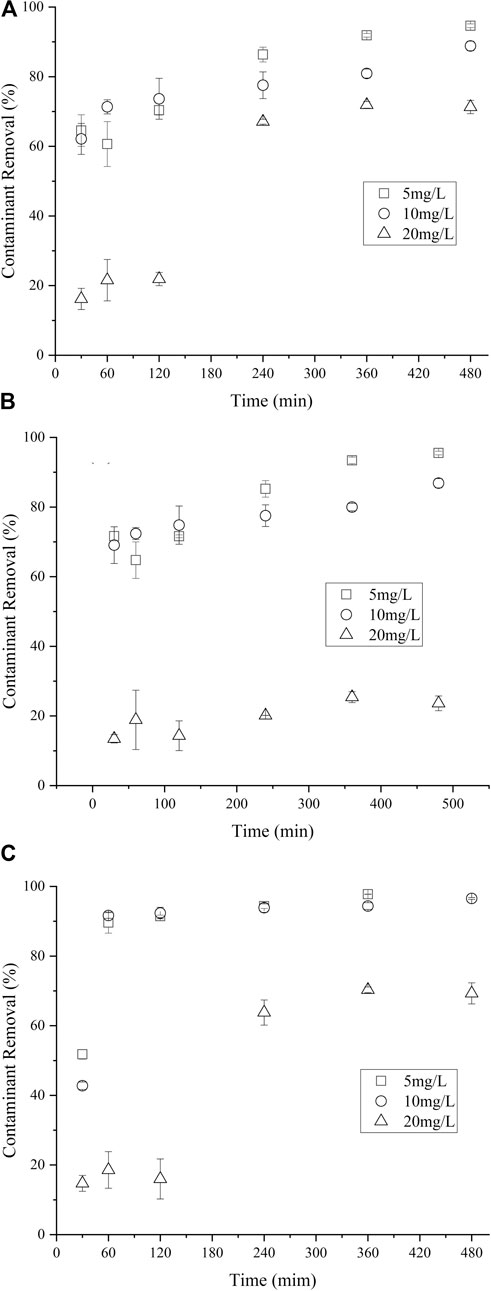
FIGURE 7. Effect of m-NCB (A), P-NCB (B) and o-NCB (C) initial concentration on their degradation. The nZVI/BC amount 0.02 g, Fe/PS molar ratio 1:1.
4 Conclusion
In this study nZVI/BC was synthesized and used as an effective activator for PS oxidation to degrade NCB, a typical and toxic pesticide intermediate. The combination of composite and PS showed superior performance to PS alone. To some extent, a higher amount of nZVI/BC or PS exhibited larger NCB removal, while further increasing the dosage of the two agents led to decreasing oxidation effect, owing to the excessive scavenging of PS by itself. The optimal mole ratio of nZVI/BC to PS was found as 1:1. The initial concentration of the target contaminant had an insignificant influence in NCB removal, except for an initial concentration as high as 20 mg L−1. Our investigation suggests that nZVI/BC activated PS oxidation is an efficient method for NCB treatment in aqueous systems.
Data Availability Statement
The original contributions presented in the study are included in the article/Supplementary Material, further inquiries can be directed to the corresponding authors.
Author Contributions
WX designed and did some of the experiments, and writed the Experiment and introduction of this paper; DRF and SWB did the main experiment works; HJB and LQ processed some of the data; ZY processed the main data and figures; WJZ designed the experiments and writed the results and dissucusion section; LT and ZST helped to improve the paper including writing.
Funding
Funding was received from the National Key Research and Development Project of China (No.2019YFC1805705) and the National Natural Science Foundation of China (No. 41571316).
Conflict of Interest
The authors declare that the research was conducted in the absence of any commercial or financial relationships that could be construed as a potential conflict of interest.
References
Chen, F., Zeng, S. Y., Ma, J., Zhu, Q. L., and Zhang, S. L. (2018). Degradation of para-nitrochlorobenzene by the combination of zero-valent iron reduction and persulfate oxidation in soil. Water Air Soil Pollut. 229 (10), 333. doi:10.1007/s11270-018-3989-5
Devi, P., Das, U., and Dalai, A. K. (2016). In-situ chemical oxidation: principle and applications of peroxide and persulfate treatments in wastewater systems. Sci. Total Environ. 571, 643–657. doi:10.1016/j.scitotenv.2016.07.032 |
Fang, G., Wu, W., Liu, C., Dionysiou, D. D., Deng, Y., and Zhou, D. (2017). Activation of persulfate with vanadium species for PCBs degradation: a mechanistic study. Appl. Catal. B Environ. 202, 1–11. doi:10.1016/j.apcatb.2016.09.006
Ghanbari, F., and Moradi, M. (2017). Application of peroxymonosulfate and its activation methods for degradation of environmental organic pollutants: review. Chem. Eng. J. 310, 41–62. doi:10.1016/j.cej.2016.10.064
Hayat, W., Zhang, Y. Q., Hussain, I., Du, X. D., Du, M. M., Yao, C. H., et al. (2019). Efficient degradation of imidacloprid in water through iron activated sodium persulfate. Chem. Eng. J. 370, 1169–1180. doi:10.1016/j.cej.2019.03.261
Huang, B. B., Qian, W. T., Yu, C. X., Wang, T., Zeng, G. M., and Lei, C. (2016). Effective catalytic hydrodechlorination of o-, p- and m-chloronitrobenzene over Ni/Fe nanoparticles: effects of experimental parameter and molecule structure on the reduction kinetics and mechanisms. Chem. Eng. J. 306, 607–618. doi:10.1016/j.cej.2016.07.109
Hussain, I., Li, M. Y., Zhang, Y. Q., Li, Y. C., Huang, S. B., Du, X. D., et al. (2017). Insights into the mechanism of persulfate activation with nZVI/BC nanocomposite for the degradation of nonylphenol. Chem. Eng. J. 311, 163–172. doi:10.1016/j.cej.2016.11.085
Kang, J., Wu, W. C., Liu, W. X., Li, J. H., and Dong, C. X. (2019). Zero-valent iron (ZVI) activation of persulfate (PS) for degradation of para-chloronitrobenzene in soil. Bull. Environ. Contam. Toxicol. 103, 140–146. doi:10.1007/s00128-018-2511-5 |
Khashij, M., Mehralian, M., and Chegini, Z. G. (2020). Degradation of acetaminophen (ACT) by ozone/persulfate oxidation process: experimental and degradation pathways. Pigment Resin Technol. 49 (5), 363–368. doi:10.1108/PRT-11-2019-0107
Le, C., Liang, J. Q., Wu, J. H., Li, P., Wang, X. D., Zhu, N. W., et al. (2011). Effective degradation of para-chloronitrobenzene through a sequential treatment using zero-valent iron reduction and Fenton oxidation. Water Sci. Technol. 64 (10), 2126–2131. doi:10.2166/wst.2011.803 |
Lee, J., Gunten, U., and Kim, J. H. (2020). Persulfate-based advanced oxidation: critical assessment of opportunities and roadblocks. Environ. Sci. Technol. 54 (6), 3086–3081. doi:10.1021/acs.est.9b07082
Li, B., and Zhu, J. (2014). Removal of p-chloronitrobenzene from groundwater: effectiveness and degradation mechanism of a heterogeneous nanoparticulate zero-valent iron (NZVI)-induced Fenton process. Chem. Eng. J. 255, 225–232. doi:10.1016/j.cej.2014.06.013
Li, X., Jia, Y., Zhou, M. H., Su, X. F., and Sun, J. H. (2020). High-efficiency degradation of organic pollutants with Fe, N co-doped biochar catalysts via persulfate activation. J. Hazard Mater. 397, 11. doi:10.1016/j.jhazmat.2020.122764
Liu, J., Lu, W., Zhang, F., Su, X., Zhu, Y., Li, R., et al. (2015). Kinetics study of activated persulfate oxidation of phenol in ground water. China Environ. Sci. 35 (9), 2677–2681. doi:10.3969/j.issn.1000-6923.2015.09.017
Liu, Z. H., Guo, W. L., Han, X. M., Li, X. H., Zhang, K., and Qiao, Z. M. (2016). In situ remediation of ortho-nitrochlorobenzene in soil by dual oxidants (hydrogen peroxide/persulfate). Environ. Sci. Pollut. Res. 23 (19), 19707–19712. doi:10.1007/s11356-016-7188-x |
Luo, H. Y., Lin, Q. T., Zhang, X. F., Huang, Z. F., Liu, S. S., Jiang, J. R., et al. (2019). New insights into the formation and transformation of active species in nZVI/BC activated persulfate in alkaline solutions. Chem. Eng. J. 359, 1215–1223. doi:10.1016/j.cej.2018.11.056
Park, J. H., Wang, J. J., Tafti, N., and Delaune, R. D. (2019). Removal of eriochrome black T by sulfate radical generated from Fe-impregnated biochar/persulfate in Fenton-like reaction. J. Ind. Eng. Chem. 71, 201–209. doi:10.1016/j.jiec.2018.11.026
Song, T. S., Zhou, B., Wang, H., Huang, Q., and Xie, J. (2020). Bioaugmentation of p-chloronitrobenzene in bioelectrochemical systems with Pseudomonas fluorescens. J. Chem. Technol. Biotechnol. 95 (1), 274–280. doi:10.1002/jctb.6233
Vahid, B., Mousanejad, T., and Khataee, A. (2015). Sonocatalytic ozonation, with nano-TiO2 as catalyst, for degradation of 4-chloronitrobenzene in aqueous solution. Res. Chem. Intermed. 41 (10), 7029–7042. doi:10.1007/s11164-014-1796-1
Wang, X., Deng, S. P., Li, C., and Wan, J. Z. (2019). Optimization of preparation conditions for biochar-loaded nano-zero-valent iron with chlorinated hydrocarbons. J. Ecol. Rural Environ.-China 35 (12), 1626–1632. doi:10.19741/j.issn.1673-4831.2019.0554
Wu, Y., Luo, H., and Wang, H. (2014). Removal of para-nitrochlorobenzene from aqueous solution on surfactant-modified nanoscale zero-valent iron/graphene nanocomposites. Environ. Technol. 35 (21), 2698–2707. doi:10.1080/09593330.2014.919032 |
Xu, J., Zhang, X. L., Sun, C., He, H., Dai, Y. X., Yang, S. G., et al. (2018). Catalytic degradation of diatrizoate by persulfate activation with peanut shell biochar-supported nano zero-valent iron in aqueous solution. Int. J. Environ. Res. Publ. Health 15 (9), 18. doi:10.3390/ijerph15091937
Yan, J. C., Han, L., Gao, W. G., Xue, S., and Chen, M. F. (2015). Biochar supported nanoscale zerovalent iron composite used as persulfate activator for removing trichloroethylene. Bioresour. Technol. 175, 269–274. doi:10.1016/j.biortech.2014.10.103 |
Yan, J. C., Qian, L. B., Gao, W. G., Chen, Y., Ouyang, D., and Chen, M. F. (2017). Enhanced Fenton-like degradation of trichloroethylene by hydrogen peroxide activated with nanoscale zero valent iron loaded on biochar. Sci. Rep. 7, 9–16. doi:10.1038/srep43051 |
Zhang, Y., Xu, X., Pan, Y., Xu, L., and Zhou, M. (2019). Pre-magnetized Fe0 activated persulphate for the degradation of nitrobenzene in groundwater. Separ. Purif. Technol. 212, 555–562. doi:10.1016/j.seppur.2018.11.074
Keywords: persulfate, biochar, zero valent iron, nitrochlorobenzen, kinetics
Citation: Wang X, Deng R, Shen W, Huang J, Li Q, Zhao Y, Wan J, Zhou Y, Long T and Zhang S (2021) Rapid Degradation of Nitrochlorobenzene by Activated Persulfate Oxidation With Biochar Supported Nanoscaled Zero Valent Iron. Front. Chem. 9:615694. doi: 10.3389/fchem.2021.615694
Received: 09 October 2020; Accepted: 11 January 2021;
Published: 10 March 2021.
Edited by:
Manoj B Gawande, Palacky University Olomouc, CzechiaReviewed by:
Mattia Bartoli, Politecnico di Torino, ItalyChinnarajesh Ummadisetti, Purdue University, United States
Copyright © 2021 Wang, Deng, Shen, Huang, Li, Zhao, Wan, Zhou, Long and Zhang. This is an open-access article distributed under the terms of the Creative Commons Attribution License (CC BY). The use, distribution or reproduction in other forums is permitted, provided the original author(s) and the copyright owner(s) are credited and that the original publication in this journal is cited, in accordance with accepted academic practice. No use, distribution or reproduction is permitted which does not comply with these terms.
*Correspondence: Jinzhong Wan, wjz@nies.org; Yan Zhou, zhouyan@nies.org