- 1Shenzhen Key Laboratory of Polymer Science and Technology, College of Materials Science and Engineering, Shenzhen University, Shenzhen. China
- 2Key Laboratory of Optoelectronic Devices and Systems of Ministry of Education and Guangdong Province, College of Physics and Optoelectronic Engineering, Shenzhen University, Shenzhen. China
- 3State Key Laboratory of Surface Physics and Department of Physics, Fudan University, Shanghai, China
- 4Jiangsu Key Laboratory for Carbon-Based Functional Materials and Devices, Soochow University, Suzhou, China
- 5Department of Electrical Engineering, City University of Hong Kong, Hong Kong, China
To meet the requirement of indoor R/G/B monochrome illumination a simplified OLEDs structure and fabrication process must occur. Herein, a design philosophy of low efficiency roll-off and simple-structure OLEDs incorporating R/G/B phosphorescent ultrathin non-doped emissive layers (EMLs) within non-exciplex forming interfaces a luminescent system by a direct charge trapping mechanism has been reported, which uses bis(2-methyldibenzo[f,h]-quinoxaline)(acetylacetonate)iridium(III) (MDQ)2Ir(acac), bis(3-phenylpyridin-e)iridium(III) (Ir(ppy)3), and bis(3,5-difluoro-2 -(2-pyridyl)phenyl-(2-carboxypyridyl) iridiumII) (Firpic) as R/G/B luminescent dyes, respectively. Although the recombination zone is narrow in the designed OLEDs, the efficiency roll-off of the designed OLEDs are unexpectedly slow, due to stable charge trapping of the emitters and are refrained from concentration quenching in relatively low current density, but the luminance meets the requirement of indoor lighting. With a low threshold voltage of 2.9/2.9/3.5 V, the designed R/G/B phosphorescent OLEDs show an efficiency roll-off as low as 7.6/3.2/4.3% for indoor luminance from 10 cd/m2 to 1,000 cd/m2, respectively. The perspective of R/G/B luminescent dyes on luminous efficiency, chromaticity coordinate drifts, efficiency roll-off, and direct charge trapping has been thoroughly studied. Therefore, our research may help to further develop ideal indoor lighting using a simplified undoped R/G/B OLEDs structure with simultaneous ultraslow efficiency roll-off, low threshold voltage, simplified fabrication process, low reagent consumption, and cost.
Introduction
As an energy conserving and environmentally friendly healthy organic semiconductor illumination apparatus, organic light emitting diodes (OLEDs) have been widely applied in health lighting sources and displays due to their excellent properties since being invented by Tang and VanSlyke (1987) and Burroughes et al. (1990) based on small molecule and conjugated polymers, respectively. However, conventional OLED products are still rather expensive for consumers (Thejokalyani and Dhoble, 2014). Hence, it is imminently required to reduce the cost of products by simplifying the OLED structure and fabrication technology. OLEDs with a doping-free ultrathin emissive layer (UEML) have aroused research interest and have been developed rapidly due to their simple structure and easily fabrication which removes the need for doping and needs fewer sensors, which therefore reduces the requirement for equipment and lowers the cost of the OLEDs (Liu et al., 2016b; Xu L. et al., 2016). Two main aspects are promoting this field development. On the one hand, OLEDs with UEML have advanced rapidly with novel UEML materials (e.g., new complexes (Zhang et al., 2012), TADF (Zhang Q. et al., 2014; Hirata et al., 2015; Zhang et al., 2015; Ahn et al., 2019), and AIE (Liu et al., 2016a; Ruan et al., 2016). Simplified doping-free white OLED (Liu et al., 2014a) and yellow-orange OLED (Liu B. et al., 2015) are achieved based on a Pt(II)-based complex emitter that achieves rather low operating voltages and high power efficiencies (Wang et al., 2014). An efficient and concentration-insensitive metal‐free thermally activated delayed fluorescence (TADF) material was reported and applied in undoped OLEDs by the Adachi group (Zhang et al., 2015). Aggregation-induced emission (AIE), color tunable, efficient undoped OLEDs and good mechanochromic properties were confirmed (Ruan et al., 2016). On the other hand, a deeper understanding of device physics, photophysics (e.g., exciplex (Park et al., 2013; Seino et al., 2014; Shin et al., 2014), electroplax (Wang et al., 2004; Yang et al., 2009; Luo et al., 2017), excimer (Chen et al., 2016), or TADF (Lin et al., 2018) property), and novel device structure with efficient exciton harvesting (Schwartz et al., 2007; Wang et al., 2009; Cui et al., 2016) also play a key role in the development of OLEDs with UEML. The Cao group fabricated a novel white OLED with a doping-free process (Liu et al., 2014b; Liu et al., 2016b). The He group designed an inverted OLED with UEML (Liu et al., 2014), which was suitable for a display driven by an active matrix transistor backplane (Lee et al., 2008). The Ma group fabricated a highly efficient and simple monochrome OLED based on UEMLs within an exciton confinement energy band structure while a deeper understanding of the exciplex formation of TCTA and TmPyPB was not clearly pointed out (Zhao et al., 2013). The Ma group also designed a novel white OLED applied with an exciplex co-host and UEML in one device (Wang et al., 2016). The Lee group managed excitons distribution in TADF (Liu et al., 2015a) and white OLEDs (Liu et al., 2015b) by an exciplex energy transfer (Cui et al., 2015). We also developed a device design philosophy of OLEDs using mixed bulk (Xu et al., 2017b; Xu et al., 2019) or an interface exciplex forming host (Xu et al., 2017c), UEMLs (Xu et al., 2018b), double UEMLs (Xu et al., 2016b), or a tandem structure (Xu et al., 2018a) as a synergistic strategy (Xu et al., 2017a) toward a simplified OLED structure (Zhang et al., 2018) and fabricated the process without sacrificing device efficiency due to improving the energy transfer process. Nowadays, the indoor conditions of photovoltaic devices under low-illuminance conditions have been extensively researched as a specialized issue (Cui et al., 2017), (Xu et al., 2016a). Meanwhile, researchers rarely pay specialized attention to indoor lighting as the energy source of indoor photovoltaics. According to the international standard of the Commission Internationale de L'Eclairage (CIE): the lighting of indoor work places (ISO 8995:2002 CIE S 008/E:2001) (Rabich et al., 2017), and the corresponding brightness of interior illumination is generally from 10 to 1,000 cd/m2.
Herein, we designed and fabricated monochrome red, green, and blue phosphorescent OLEDs based on non-doped UEMLs to meet the requirement of indoor R/G/B monochrome illumination. Particularly, it is observed that the non-doped UEML-based OLEDs generally show very slow efficiency roll-off and low threshold voltage, which is fitting for indoor lighting in low current density with a brightness from 10 cd/m2 to 1,000 cd/m2. Moreover, the maximum power efficiency reached 5.766 lm/w at 177.8 cd/m2, 15.74 lm/w at 99.27 cd/m2, and 2.277 lm/w at 252.2 cd/m2 for the R/G/B monochrome OLEDs, respectively. The perspective of R/G/B luminescent dyes on luminous efficiency, chromaticity coordinate drifts, efficiency roll-off, and charge trapping mechanism has been thoroughly studied. Therefore, our work helps to develop ideal indoor lighting using a simplified undoped R/G/B OLED structure with simultaneous slow efficiency roll-off, low threshold voltage, simplified fabrication, low reagent consumption, and cost.
Experiment Details
All OLED devices were fabricated based on the same glass substrate pattern with a conducting indium-tin-oxide (ITO) anode and a sheet resistance lower than 20 Ω/square. The substrate fabrication was followed by a routine cleaning process (Xu et al., 2016a). The basic structure of the devices is a ITO/MoO3 (1 nm)/4′-bis(carbazol-9-yl) biphenyl (CBP) (35 nm) /Ultrathin emitter (0.3 nm)/1,3,5-Tris(N -phenylbenzimidazol -2-yl)benzene (TPBi) (65 nm)/LiF (1 nm)/Al (100 nm), in which the schematic parameter diagram and energy level diagram of the device structures are shown in Figures 1A, B, including MoO3 and CBP which act as the hole injection layer (HIL) and hole transporting layer (HTL). LiF and TPBi are used as the electron injection layer (EIL) and electron transporting layer (ETL). A 1 nm thick thermally evaporated MoO3 layer was deposited on top to achieve a high work function for the hole injection into CBP. The phosphorescent dyes in this study were bis(3-phenylpyridine)iridium(III) [Ir(ppy)3] for green in device A, bis(2-methyldibenzo[f,h]-quinoxaline) (acetylacetonate) iridium(III)[(MDQ)2Ir(acac)] for red in device B, and bis(3,5-difluoro-2 -(2-pyridyl)phenyl-(2-carboxypyridyl)iridium III (FIrpic) for blue in device C. Figure 1C shows the molecular structures of Ir(ppy)3, Ir(MDQ)2(acac), Firpic, TPBi, and CBP, respectively.
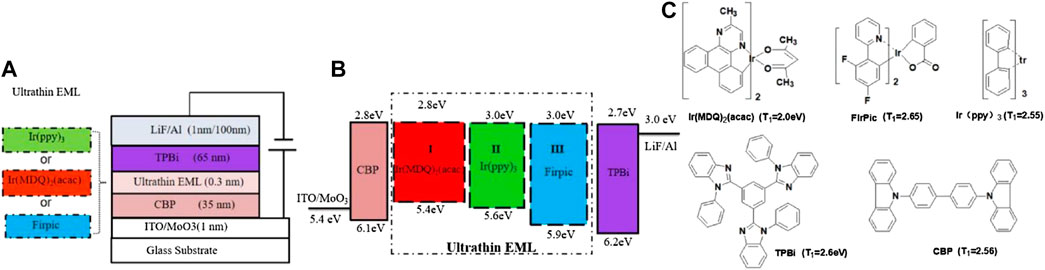
FIGURE 1. (A) Schematic device structure and (B) corresponding energy-level diagram of the devices considered in this work as well as (C) the molecular structure and triplet energies (T1) of the materials used.
Results and Discussion
Lots of researchers have proposed two primary mechanisms for the exciton process in OLEDs: host-guest energy transfer and direct charge trapping of the guest. Exciton formation occurs directly on the guest molecules, which play an important role in balancing charge injection. This direct charge trapping on the guest can be confirmed by the dependence of the drive voltage and electroluminescence spectrum on guest concentration (Holmes et al., 2003). The light emission mechanism of OLEDs with a mixed co-host emitting layer was also studied using an exciplex-type mixed co-host and an exciplex free mixed co-host (Song and Lee, 2015). The light emission process in the interface of exciplex-type OLED devices was dominated by energy transfer while the interface of non-exciplex type devices was dominated by a charge trapping emission mechanism (Wang et al., 2016). In this research, a design philosophy of low efficiency roll-off and simple-structure OLEDs incorporating R/G/B phosphorescent non-doped UEMLs within a non-exciplex forming interface luminescent system by a direct charge trapping mechanism was proposed. The device structures and the energy levels of the designed OLEDs are shown in Figure 1A,B.
In working OLEDs, firstly, electrons and holes can be easily injected from the ITO anode and aluminum cathode under driving voltage, respectively. Then, electrons and holes go through ETL/HTL and meet to form excitons. There were some differences between devices A, B, and C. Under voltage from 2.9 to 4.9 V with a corresponding luminance from 10 cd/m2 to 1,000 cd/m2, the luminance of device A was linear to the logarithmic coordinate voltage of device A as shown in Figures 2A,B, and the current efficiency roll-off of device A was as low as 7.6%. As to the chromaticity coordinate of device A, the CIE(x,y) was nearly unchanged from 2.9 to 4.9 V as shown in Figure 2C. This phenomenon is indicative of the direct charge trapping of Ir(ppy)3 as the luminescent dye of device A was quite effective in a low current density. Lastly, as the voltage increased after exceeding 5 V with a corresponding luminance of 1,000 cd/m2, the luminance of device A showed a gradual invariant with fast efficiency roll-off. There are three main reasons for this phenomenon. Firstly, the values of HOMO and LUMO of TPBi are closed to CBP as shown in Figure 1B, the energy level cannot block the leak current in a high current density, and carriers meet to form excitons in wide bandwidth HTL/ETL for inefficient lighting. Secondly, the direct charge trapping of Ir(ppy)3 as the luminescent dye of device A is saturated in high current density, while device performance is also saturated without assisting the energy transfer process (Forster energy transfer and Dexter energy transfer) in a non-exciplex system. Thirdly, the triplet state energy levels (T1) of CBP (2.55 eV) and TPBi (2.67 eV) could not be confirmed for luminescent dye Ir(ppy)3 (2.47 eV) in high voltage leading to drifting of excitons into a wide bandwidth HTL/ETL for inefficient lighting. Therefore, the chromaticity coordinate CIE(x,y) of device A was remarkably changed following the direction as the voltage increased after exceeding 5 V as shown in Figure 2C.
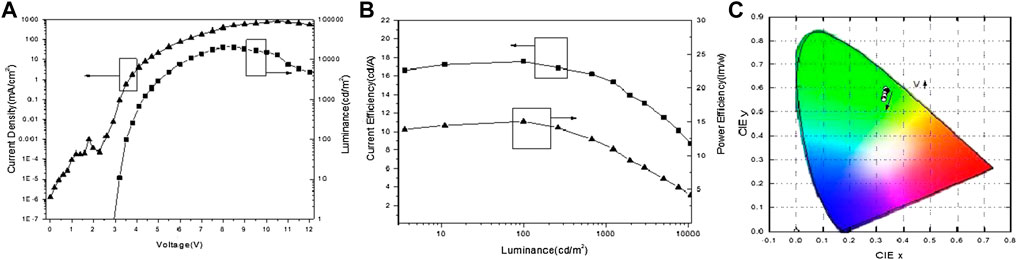
FIGURE 2. (A) Current density-voltage-luminance characteristics, (B) current efficiency-luminance-power efficiency characteristics, and (C) chromaticity coordinates of green OLEDs.
As seen in Figure 3A and Figure 4A, under low voltage with corresponding luminance from 10 to 1,000 cd/m2, the luminance of devices B and C were linear to the logarithmic coordinate voltage of devices B and C. The current efficiency roll-off of devices B and C were as low as 3.2 and 4.3% in as shown in Figure 3B and Figure 4B, respectively. The CIE(x,y) of devices B and C were nearly unchanged under low voltage while the CIE(x,y) of devices B and C changed remarkably following the direction as the voltage increased after exceeding 5–6 V as shown in Figure 3C and Figure 4c.
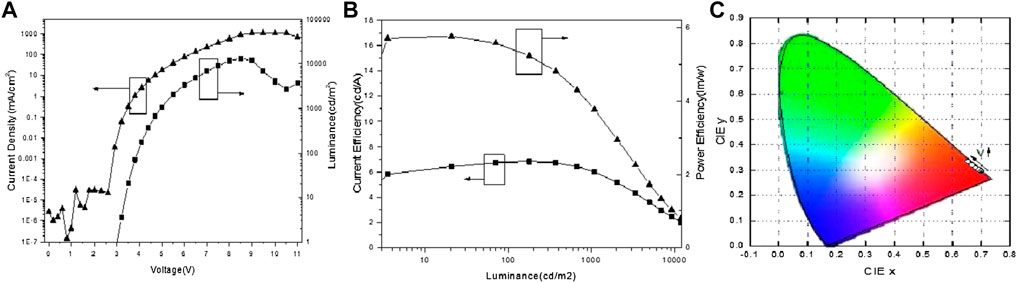
FIGURE 3. (A) Current density-voltage-luminance characteristics. (B) Current efficiency-luminance-power efficiency characteristic. (C) Chromaticity coordinate of red OLEDs.
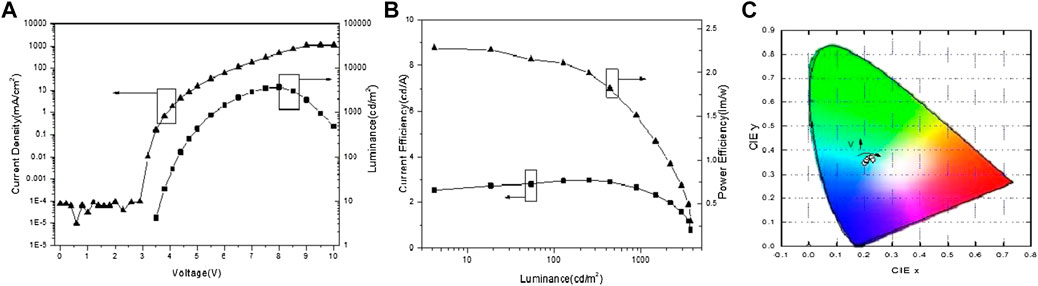
FIGURE 4. (A) Current density-voltage-luminance characteristics. (B) Current efficiency-luminance-power efficiency characteristic. (C) Chromaticity coordinate of blue OLEDs.
Thanks to the equal thickness of devices A, B, and C, the J-V-L curves of the phosphorescent dyes were different as shown in Figures 2A,B, Figures 3A,B, and Figures 4A,B. In low working voltage, there was no significant difference for J-V among devices A, B, and C implying that exciton quenching or the nonradiative decay of excitons was greatly suppressed by the direct charge trapping of this simple UEML within the non-exciplex interface TPBi/CPB. However, the efficiencies of the OLEDs with UEML were affected by the phosphorescent dyes with different T1 levels. The case of CPB or TPBi as the host with exciton formation as the guest should be similar to this OLED with non-doped UEML by direct charge trapping. The utilization of the non-doped UEML structure yields high efficiencies for the green OLED with Ir(ppy)3 as the UEML, while the efficiencies of the red and blue OLEDs with (MDQ)2 Ir(acac) and Firpic were not as high as the green dye on account of relatively low PLQY, which was similar in the host-guest doping system. The non-doped UEML was constructed by introducing a 0.3 nm thin layer of pure phosphorescent dyes between the HTL and ETL layer. The maximum current efficiency reached 15.74 lm/w at 99.27 cd/m2, 5.77 lm/w at 177.8 cd/m2, and 2.28 lm/w at 252.2 cd/m2 for the green, red, and blue monochrome OLEDs, respectively.
To further explore the working mechanism of the designed OLEDs with UEML in high current density, Supplementary Figure S1 shows the electroluminescence (EL) spectra of the non-doped OLEDs with UEML for the R/G/B phosphorescent dyes at the higher voltage of 8 V. It can be seen that device A with Ir(ppy)3 and device B with Ir(MDQ)2 (acac) show pure dye emission due to the high T1 level of CPB and TPBi confirming direct charge trapping excitation in the relatively lower T1 level of Ir(ppy)3 or Ir(MDQ)2 (acac) in high current density. In device C with FIrpic, it is interesting that the EL spectra peak around 380 nm showed the characteristics of host materials intramolecular luminescence in TPBi (Zhang T. et al., 2014) rather than in CBP (Park et al., 2011), which indicates exciton diffusion into TPBi. This may have been due to ETL occurring intensely or due to the charge carrier recombination zone in TPBi, and then radiative decay which emitted light into TPBi in a primary way. There was also a secondary peak around 450 showing the dye emission of FIrpic due to direct charge trapping of FIrpic to form exciton, but the EL of FIrpic was much weaker than the emitted light in TPBi due to the relatively low efficient exciton energy transfer from TPBi (Jang et al., 2011) to FIrpic as shown in Supplementary Figure S1. Moreover, there was a wide peak around 700 nm which shows the characteristics of inter-molecular overlay of the red-shifted excimer of phosphorescence (Kalinowski et al., 2007). Low direct charge trapping of FIrpic, exciton diffusion to ETL, and low efficient exciton energy transfer from TPBi to FIrpic led to the low efficiency of device C in high current density.
The J-V-L characteristics, the CE-L-PE characteristics, and the chromaticity coordinate of the device A are shown in Figures 2A–C, separately. Similarly, Figure 3 and Figure 4 show the corresponding performance of device B and device C. The turn-on voltages (Von) (which are defined as a luminance of 1 cd /cm2) of devices A and B were as low as 2.9 V, and device C was 3.5 V as summarized in Table 1. The maximum current and power efficiency (ηc max, Pmax) of (17.5 cd/A, 15.7 lm/W), (6.9 cd/A, 5.8 lm/W), and (2.98 cd/A, 2.3 lm/W) were obtained in devices A, B, and C, respectively. And the maximum brightness of devices A, B, and C were as high as 20,700, 13,210, and 3,740 cd/m2, respectively. The phosphorescence dyes have different capabilities to trap the charge carriers directly leading to different efficiencies in the OLEDs with UEML.
In low working voltage, the power efficiency of the blue dye OLEDs with non-doped UEML was as high as the red one. The utilization of this kind of non-doped UEML within non-exciplex forming interfaces can be appropriate for R/G/B dyes. All the monochrome R/G/B OLEDs based UEML show relatively high efficiency and ultraslow efficiency roll-off in low driving voltage, the non-doped UEML structure within a non-exciplex forming interface has broad adaptability for most phosphorescent dyes with ultraslow efficiency roll-off in low driving voltage. As there is relatively few reports on non-exciplex forming interfaces as direct charge tapping structures that have such broad adaptability for phosphorescent dyes with ultraslow efficiency roll-off in low driving voltage, our technical route offers a brief scheme to achieve indoor lighting requirements. Further, in order to clearly describe the work mechanism of this device structure, a physical model of exciton dynamics in OLED with non-doped UEML is proposed in Figure 5.
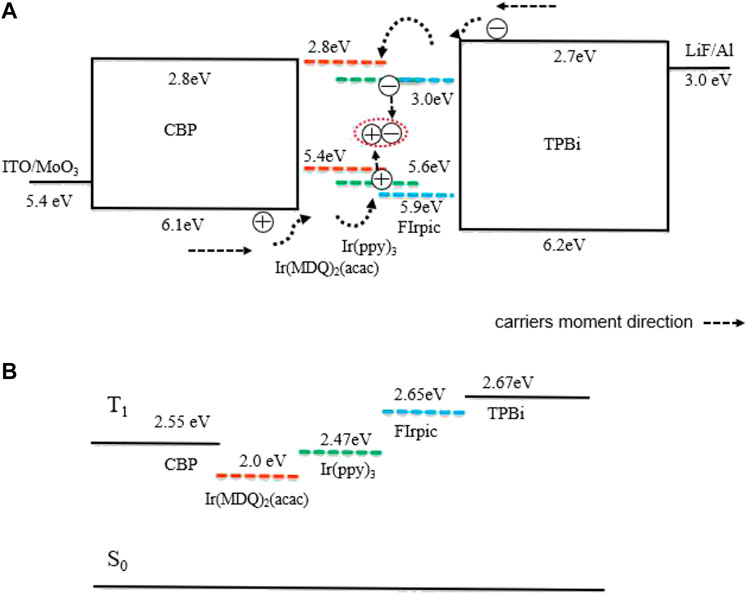
FIGURE 5. (A) Energy diagrams and carrier transfer route of proposed OLEDs. (B) Triplet energy level T1 of CBP, TPBi, Ir(MDQ)2(acac), Ir(ppy)3, and FIrpic.
The higher turn on voltage for device C suggests that the excitons from direct charge trapping are not efficient comparing with devices A and B. As depicted in Figure 5A, some of the carriers would enter into the UEMLs as deep as 3 nm (Zhao et al., 2012), and then would recombine in the organic layers, while the direct charge carrier recombination on phosphorescent dyes would generate dye emission due to the thin nature of non-doped UEML. These excitons may either transport their energy from ETL or HTL to the EML. The efficiency of OLEDs with Ir(MDQ)2 (acac) was lower than that of OLEDs with Ir(ppy)3 since the LUMO energy level of Ir(MDQ)2 (acac) was nearly equal to the LUMO energy level of CBP and TPBi. There was also no doubt that the capability of Ir(MDQ)2 (acac) to direct charge trapping was weaker than that of Ir(ppy)3 in the same thickness. From the OLED-based UEML, the energy transport process from the ETL/HTL to the EML was much more than that of exciton diffusion in device A and device B. Meanwhile, as depicted in Figure 5B, thanks to the higher T1 exciton between the transporting layers of T1 of CBP and TBPi, the excitons generated were effectively confined in the UEML for the Ir(MDQ)2 (acac) and Ir(ppy)3 dyes. As to the blue OLED with FIrpic, the efficiency of the OLEDs with FIrpic was lower than that of OLEDs with Ir(ppy)3 since the T1 energy level of FIrpic was higher than that of CBP. Although the recombination zone is narrow in the designed OLEDs, the efficiency roll-off of the designed OLEDs are unexpectedly slow mainly due to the stable charge trapping of the emitter and avoiding concentration quenching in relatively low current density for the requirements of indoor lighting. With a low threshold voltage of 2.9/2.9/3.5 V, the designed R/G/B phosphorescent OLEDs show an efficiency roll-off as low as 7.6/3.2/4.3% in the indoor luminance intensity range from 10 to 1,000 cd/m2, respectively.
Conclusion
In summary, we succeeded in applying a series of R/G/B ultrathin non-doped phosphorescent dye layers into non-exciplex forming interfaces as a cost-effective method to realize OLEDs with ultraslow efficiency roll-off and low driving voltage to satisfy indoor R/G/B illumination requirements in low driving voltage. This device structure shows great superiority in easy fabrication, low-cost, and high-efficiency when compared to compact fluorescent lamps due to the highly simplified ultrathin and undoped emitters. This technical route has high value for the future of indoor solid-state lighting applications.
Data Availability Statement
The original contributions presented in the study are included in the article/Supplementary Material, further inquiries can be directed to the corresponding author.
Author Contributions
TX conceived of the work. TX, RY, and CZ fabricated and characterized OLED. TX, and ML directed and supervised the project.
Funding
This project was funded by the China Postdoctoral Science Foundation (2019M663027). This work was also supported by the Shenzhen Peacock Plan (KQTD20170330110107046), the Science and Technology Innovation Commission of Shenzhen (JCYJ20170818085627721, JCYJ20180507182244027), the Open Project of J Key Laboratory for Carbon-Based Functional Materials & Devices, Soochow University, P. R. China, (KJS1908), and the State Key Laboratory of Surface Physics and Department of Physics, Fudan University, P. R. China (Grant No. KF2019_13). I would like to express my special gratitude to Liang-Sheng Liao and Hong Meng for their guidance in my research.
Conflict of Interest
The authors declare that the research was conducted in the absence of any commercial or financial relationships that could be construed as a potential conflict of interest.
Supplementary Material
The Supplementary Material for this article can be found online at: https://www.frontiersin.org/articles/10.3389/fchem.2020.630687/full#supplementary-material.
References
Ahn, D. H., Kim, S. W., Lee, H., Ko, I. J., Karthik, D., Lee, J. Y., et al. (2019). Highly efficient blue thermally activated delayed fluorescence emitters based on symmetrical and rigid oxygen-bridged boron acceptors. Nat. Photon. 1, 540–546. doi:10.1038/s41566-019-0415-5
Burroughes, J. H., Bradley, D. D. C., Brown, A. R., Marks, R. N., Mackay, K., Friend, R. H., et al. (1990). Light-emitting diodes based on conjugated polymers. Nature 347 (6293), 539–541. doi:10.1038/347539a0
Chen, Y.-H., Tang, K.-C., Chen, Y.-T., Shen, J.-Y., Wu, Y.-S., Liu, S.-H., et al. (2016). Insight into the mechanism and outcoupling enhancement of excimer-associated white light generation. Chem. Sci. 7 (6), 3556–3563. doi:10.1039/C5SC04902D
Cui, L. S., Kim, J. U., Nomura, H., Nakanotani, H., and Adachi, C. (2016). Benzimidazobenzothiazole‐based bipolar hosts to harvest nearly all of the excitons from blue delayed fluorescence and phosphorescent organic light‐emitting diodes. Angew. Chem. Int. Edn. 55 (24), 6864–6868. doi:10.1002/anie.201601136
Cui, L.-S., Xie, Y.-M., Wang, Y.-K., Zhong, C., Deng, Y.-L., Liu, X.-Y., et al. (2015). Pure hydrocarbon hosts for ≈100% exciton harvesting in both phosphorescent and fluorescent light-emitting devices. Adv. Mat. 27 (28), 4213–4217. doi:10.1002/adma.201501376
Cui, Y., Wang, Y., Bergqvist, J., Yao, H., Xu, Y., Gao, B., et al. (2017). Wide-gap non-fullerene acceptor enabling high-performance organic photovoltaic cells for indoor applications. Nat. Energy 4 (9), 768–775. doi:10.1038/s41560-019-0448-5
Hirata, S., Sakai, Y., Masui, K., Tanaka, H., Lee, S. Y., Nomura, H., et al. (2015). Highly efficient blue electroluminescence based on thermally activated delayed fluorescence. Nat. Mater. 14 (3), 330. doi:10.1038/nmat4154
Holmes, R., D’Andrade, B., Forrest, S., Ren, X., Li, J., and Thompson, M. (2003). Efficient, deep-blue organic electrophosphorescence by guest charge trapping. Appl. Phys. Lett. 83 (18), 3818–3820. doi:10.1063/1.1624639
Jang, J. G., Ji, H. J., Kim, H. S., and Jeong, J. C. (2011). TPBI:FIrpic organic light emitting devices with the electron transport layer of Bphen/Alq3. Curr. Appl. Phys. 11 (1), S251–S254. doi:10.1016/j.cap.2010.11.103
Kalinowski, J., Cocchi, M., Virgili, D., Fattori, V., and Williams, J. G. (2007). Mixing of excimer and exciplex emission: a new way to improve white light emitting organic electrophosphorescent diodes. Adv. Mat. 19 (22), 4000–4005. doi:10.1002/adma.200700655
Lee, Y., Kim, J., Kwon, S., Min, C.-K., Yi, Y., Kim, J., et al. (2008). Interface studies of aluminum, 8-hydroxyquinolatolithium (Liq) and Alq3 for inverted OLED application. Org. Elect. 9 (3), 407–412. doi:10.1016/j.orgel.2008.01.001
Lin, T.-C., Sarma, M., Chen, Y.-T., Liu, S.-H., Lin, K.-T., Chiang, P.-Y., et al. (2018). Probe exciplex structure of highly efficient thermally activated delayed fluorescence organic light emitting diodes. Nat. Commun. 9 (1), 3111. doi:10.1038/s41467-018-05527-4
Liu, B., Luo, D., Gao, D., Wang, X., Xu, M., Zou, J., et al. (2015). An ideal host-guest system to accomplish high-performance greenish yellow and hybrid white organic light-emitting diodes. Org. Elect. 27, 29–34. doi:10.1016/j.orgel.2015.08.030
Liu, B., Nie, H., Zhou, X., Hu, S., Luo, D., Gao, D., et al. (2016aa). Manipulation of charge and exciton distribution based on blue aggregation‐induced emission fluorophors: a novel concept to achieve high‐performance hybrid white organic light‐emitting diodes. Adv. Funct. Mat. 26 (5), 776–783. doi:10.1002/adfm.201503368
Liu, B., Tao, H., Wang, L., Gao, D., Liu, W., Zou, J., et al. (2016b). High-performance doping-free hybrid white organic light-emitting diodes: the exploitation of ultrathin emitting nanolayers (< 1 nm). Nan. Ene. 26, 26–36. doi:10.1016/j.nanoen.2016.04.054
Liu, B., Xu, M., Wang, L., Yan, X., Tao, H., Su, Y., et al. (2014a). Investigation and optimization of each organic layer: a simple but effective approach towards achieving high-efficiency hybrid white organic light-emitting diodes. Org. Elect. 15 (4), 926–936. doi:10.1016/j.orgel.2014.02.005
Liu, B., Xu, M., Wang, L., Zou, J., Tao, H., Su, Y., et al. (2014b). Regulating charges and excitons in simplified hybrid white organic light-emitting diodes: the key role of concentration in single dopant host–guest systems. Org. Elect. 15 (10), 2616–2623. doi:10.1016/j.orgel.2014.07.033
Liu, J., Shi, X., Wu, X., Wang, J., Min, Z., Wang, Y., et al. (2014). Achieving above 30% external quantum efficiency for inverted phosphorescence organic light-emitting diodes based on ultrathin emitting layer. Org. Elect. 15 (10), 2492–2498. doi:10.1016/j.orgel.2014.07.027
Liu, X.-K., Chen, Z., Qing, J., Zhang, W.-J., Wu, B., Tam, H. L., et al. (2015a). Remanagement of singlet and triplet excitons in single-emissive-layer hybrid white organic light-emitting devices using thermally activated delayed fluorescent blue exciplex. Adv. Mat. 27 (44), 7079–7085. doi:10.1002/adma.201502897
Liu, X.-K., Chen, Z., Zheng, C.-J., Liu, C.-L., Lee, C.-S., Li, F., et al. (2015b). Prediction and design of efficient exciplex emitters for high-efficiency, thermally activated delayed-fluorescence organic light-emitting diodes. Adv. Mat. 27 (14), 2378–2383. doi:10.1002/adma.201405062
Luo, D., Li, X.-L., Zhao, Y., Gao, Y., and Liu, B. (2017). High-performance blue molecular emitter-free and doping-free hybrid white organic light-emitting diodes: an alternative concept to manipulate charges and excitons based on exciplex and electroplex emission. ACS Photon. 4 (6), 1566–1575. doi:10.1021/acsphotonics.7b00364
Park, Y.-S., Jeong, W.-I., and Kim, J.-J. (2011). Energy transfer from exciplexes to dopants and its effect on efficiency of organic light-emitting diodes. J. Appl. Phys. 110 (12), 124519. doi:10.1063/1.3672836
Park, Y. S., Lee, S., Kim, K. H., Kim, S. Y., Lee, J. H., and Kim, J. J., (2013). Exciplex‐forming co‐host for organic light‐emitting diodes with ultimate efficiency. Adv. Funct. Mat. 23 (39), 4914–4920. doi:10.1002/adfm.201300547
Ruan, Z., Li, L., Wang, C., Xie, Y., Hu, Q., Peng, Q., et al. (2016). Tetraphenylcyclopentadiene derivatives: aggregation-induced emission, adjustable luminescence from green to blue, efficient undoped OLED performance and good mechanochromic properties. Small 12 (47), 6623–6632. doi:10.1002/smll.201602285
Rabich, О., Chumak, L., Meshcheriakova, I., and Laukhyna, L. (2017). “Possibility and effectiveness of led lighting of the permanent workplaces in modern construction production,” in Proceedings in memory of Starodubov, 5. Available at: http://smm.pgasa.dp.ua/article/view/103633.
Schwartz, G., Pfeiffer, M., Reineke, S., Walzer, K., and Leo, K. (2007). Harvesting triplet excitons from fluorescent blue emitters in white organic light‐emitting diodes. Adv. Mat. 19 (21), 3672–3676. doi:10.1002/adma.200700641
Seino, Y., Sasabe, H., Pu, Y. J., and Kido, J. (2014). High‐performance blue phosphorescent OLEDs using energy transfer from exciplex. Adv. Mat. 26 (10), 1612–1616. doi:10.1002/adma.201304253
Shin, H., Lee, S., Kim, K. H., Moon, C. K., Yoo, S. J., Lee, J. H., et al. (2014). Blue phosphorescent organic light‐emitting diodes using an exciplex forming co‐host with the external quantum efficiency of theoretical limit. Adv. Mat. 26 (27), 4730–4734. doi:10.1002/adma.201400955
Song, W., and Lee, J. Y. (2015). Light emission mechanism of mixed host organic light-emitting diodes. Appl. Phys. Lett. 106 (12), 123306. doi:10.1063/1.4916549
Tang, C. W., and VanSlyke, S. A. (1987). Organic electroluminescent diodes. Appl. Phys. Lett. 51 (12), 913–915. doi:10.1063/1.98799
Thejokalyani, N., and Dhoble, S. J. (2014). Importance of eco-friendly OLED lighting. Defect Diffusion Forum 357, 1–27. doi:10.4028/www.scientific.net/DDF
Wang, J., Chen, J., Qiao, X., Alshehri, S. M., Ahamad, T., and Ma, D., (2016). Simple-structured phosphorescent warm white organic light-emitting diodes with high power efficiency and low efficiency roll-off. ACS Appl. Mat. Inter 8 (16), 10093–10097. doi:10.1021/acsami.6b00861
Wang, Q., Ding, J., Ma, D., Cheng, Y., Wang, L., Jing, X., et al. (2009). Harvesting excitons via two parallel channels for efficient white organic LEDs with nearly 100% internal quantum efficiency: fabrication and emission‐mechanism analysis. Adv. Funct. Mat. 19 (1), 84–95. doi:10.1002/adfm.200800918
Wang, Q., Oswald, I. W. H., Perez, M. R., Jia, H., Shahub, A. A., Qiao, Q., et al. (2014). Doping-free organic light-emitting diodes with very high power efficiency, simple device structure, and superior spectral performance. Adv. Funct. Mat. 24 (30), 4746–4752. doi:10.1002/adfm.201400597
Wang, X., Wang, R., Zhou, D., and Yu, J. (2016). Study of organic light-emitting diodes with exciplex and non-exciplex forming interfaces consisting of an ultrathin rubrene layer. Synth. Met. 214, 50–55. doi:10.1016/j.synthmet.2016.01.022
Wang, Y. M., Teng, F., Xu, Z., Hou, Y. B., Yang, S. Y., Qian, L., et al. (2004). White emission via electroplex formation at poly (N-vinylcarbazole)/2, 9-dimethyl-4, 7-diphenyl-1, 10-phenanthroline interface. Appl. Surf. Sci. 236 (1–4), 251–255. doi:10.1016/j.apsusc.2004.04.031
Xu, L., Tang, C. W., and Rothberg, L. J., (2016). High efficiency phosphorescent white organic light-emitting diodes with an ultra-thin red and green co-doped layer and dual blue emitting layers. Org. Elect. 32, 54–58. doi:10.1016/j.orgel.2016.02.010
Xu, T., Yan, L., Miao, J., Hu, Z., Shao, S., Li, A., et al. (2016a). Unlocking the potential of diketopyrrolopyrrole-based solar cells by a pre-solvent annealing method in all-solution processing. RSC Adv. 6 (58), 53587–53595. doi:10.1039/C6RA09770G
Xu, T., Yang, M., Liu, J., Wu, X., Murtaza, I., He, G., et al. (2016b). Wide color-range tunable and low roll-off fluorescent organic light emitting devices based on double undoped ultrathin emitters. Org. Elect. 37, 93–99. doi:10.1016/j.orgel.2016.06.014
Xu, T., Zhang, Y. X., Huang, C. C., Zhou, J. G., Fung, M. K., and Meng, H. (2019). Highly simplified blue phosphorescent organic light-emitting diodes incorporating exciplex-forming co-host assisting energy transfer. J. Lumin. 206, 554–559. doi:10.1016/j.jlumin.2018.10.007
Xu, T., Zhang, Y.-X., Murtaza, I., and Meng, H. (2017a). P-170: organic light-emitting diodes incorporating a novel exciplex-forming host: a synergistic strategy to design highly simplified OLEDs for application. SID Symp. Dig. Tech. Pap. 48 (1), 1915–1918. doi:10.1002/sdtp.12004
Xu, T., Zhang, Y. X., Wang, B., Huang, C. C., Murtaza, I., Meng, H., et al. (2017b). Highly simplified reddish orange phosphorescent organic light-emitting diodes incorporating a novel carrier-and exciton-confining spiro-exciplex-forming host for reduced efficiency roll-off. ACS Appl. Mater. Inter. 9 (3), 2701–2710. doi:10.1021/acsami.6b13077
Xu, T., Zhou, J. G., Fung, M. K., and Meng, H. (2018aa). Simplified efficient warm white tandem organic light-emitting devices by ultrathin emitters using energy transfer from exciplexes. Org. Elect. 63, 369–375. doi:10.1016/j.orgel.2018.09.026
Xu, T., Zhou, J. G., Huang, C. C., Zhang, L., Fung, M. K., Murtaza, I., et al. (2017c). Highly simplified tandem organic light-emitting devices incorporating a green phosphorescence ultrathin emitter within a novel interface exciplex for high efficiency. ACS Appl. Materials Inter. 9 (12), 10955–10962. doi:10.1021/acsami.6b16094
Xu, T., Zhou, J. G., Meng, H., and Fung, M. K. (2018bb). P-164: energy transfer from interface exciplexes to ultrathin emissive layers: a path way to design simplified efficient white tandem organic light-emitting diodes for application. SID Symp. Dig. Tech. Pap. 49 (1), 1779–1781. doi:10.1002/sdtp.12394
Yang, C. C., Hsu, C. J., Chou, P. T., Cheng, H. C., Su, Y. O., and Leung, M. k. (2009). Excited state luminescence of multi-(5-phenyl-1, 3, 4-oxadiazo-2-yl) benzenes in an electron-donating matrix: exciplex or electroplex? The J. Phys. Chem. B 114 (2), 756–768. doi:10.1021/jp9093063
Zhang, B., Tan, G., Lam, C. S., Yao, B., Ho, C. L., Liu, L., et al. (2012). High‐efficiency single emissive layer white organic light‐emitting diodes based on solution‐processed dendritic host and new orange‐emitting iridium complex. Adv. Mat. 24 (14), 1873–1877. doi:10.1002/adma.201104758
Zhang, Q., Li, B., Huang, S., Nomura, H., Tanaka, H., and Adachi, C. (2014). Efficient blue organic light-emitting diodes employing thermally activated delayed fluorescence. Nat. Photon. 8 (4), 326. doi:10.1038/nphoton.2014.1
Zhang, Q., Tsang, D., Kuwabara, H., Hatae, Y., Li, B., Takahashi, T., et al. (2015). Nearly 100% internal quantum efficiency in undoped electroluminescent devices employing pure organic emitters. Adv. Mat. 27 (12), 2096–2100. doi:10.1002/adma.201405474
Zhang, T., Chu, B., Li, W., Su, Z., Peng, Q. M., and Zhao, B., (2014). Efficient triplet application in exciplex delayed-fluorescence oleds using a reverse intersystem crossing mechanism based on a δ es–t of around zero. ACS Appl. Mater. Inter. 6 (15), 11907–11914. doi:10.1021/am501164s
Zhang, W., Wang, H., Miao, J., Zhu, Y., Ali, M. U., Xu, T., et al. (2018). Revealing the influence of hole injection material's molecular orientation on OLED’s performance. Org. Elect. 59, 301–305. doi:10.1016/j.orgel.2018.05.022
Zhao, Y., Chen, J., and Ma, D. (2013). Ultrathin nondoped emissive layers for efficient and simple monochrome and white organic light-emitting diodes. ACS Appl. Materials Inter. 5 (3), 965–971. doi:10.1021/am3026097
Zhao, Y., Zhu, L., Chen, J., and Ma, D. (2012). Improving color stability of blue/orange complementary white OLEDs by using single-host double-emissive layer structure: comprehensive experimental investigation into the device working mechanism. Org. Elect. 13 (8), 1340–1348. doi:10.1016/j.orgel.2012.04.015
Keywords: indoor illumination, OLEDs, efficiency roll-off, simple structure, direct charge trapping
Citation: Xu T, Yi R, Zhu C and Lin M (2021) Simple-Structured OLEDs Incorporating Undoped Phosphorescent Emitters Within Non-Exciplex Forming Interfaces: Towards Ultraslow Efficiency Roll-Off and Low Driving Voltage for Indoor R/G/B Illumination. Front. Chem. 8:630687. doi: 10.3389/fchem.2020.630687
Received: 19 November 2020; Accepted: 30 December 2020;
Published: 15 March 2021.
Edited by:
Qifan Xue, South China University of Technology, ChinaReviewed by:
Mingjie Li, Hong Kong Polytechnic University, Hong KongJungui Zhou, Soochow University, China
Copyright © 2021 Xu, Yi, Zhu and Lin. This is an open-access article distributed under the terms of the Creative Commons Attribution License (CC BY). The use, distribution or reproduction in other forums is permitted, provided the original author(s) and the copyright owner(s) are credited and that the original publication in this journal is cited, in accordance with accepted academic practice. No use, distribution or reproduction is permitted which does not comply with these terms.
*Correspondence: Ting Xu, robin@pku.edu.cn