- 1Key Laboratory of High Efficiency and Clean Mechanical Manufacture, Ministry of Education, School of Mechanical Engineering, Shandong University, Jinan, China
- 2National Demonstration Center for Experimental Mechanical Engineering Education, Shandong University, Jinan, China
- 3Shandong Key Laboratory of Water Pollution Control and Resource Reuse, School of Environmental Science and Engineering, Shandong University, Qingdao, China
- 4Department of Mechanical Engineering, Hanyang University, Ansan, South Korea
- 5Department of Process Engineering and Chemical Technology, Faculty of Chemistry, Gdańsk University of Technology, Gdańsk, Poland
The rise of CO2 in the atmosphere, which results in severe climate change and temperature increase, is known as the major reason for the greenhouse effect. Reducing CO2 to value-added products is an attractive solution to this severe problem, along with addressing the energy crisis, to which the catalysts being employed are of vital importance. Due to their high porosity and tunable compositions, metal-organic frameworks (MOFs) show great potential in energy conversion systems. By thermal or chemical treatment methods, the MOFs are easily turned into MOF-derived carbon nanomaterials. The much higher level of conductivity enables MOF-derived carbon nanomaterials to be employed in CO2 conversion processes. The present review, discusses the state of the art of MOF-derived carbon nanomaterials in CO2 electrochemical, photocatalytic, and thermal reduction applications. The corresponding reaction mechanisms and influence of various factors on catalyst performance are elaborated. Finally, the deficiencies and recommendations are provided for future progress.
Introduction
The catalytic reduction of carbon dioxide (CO2) to value-added products is an effective way of alleviating the severe environmental problem of global warming (Ran et al., 2018; Li et al., 2019). Great efforts have been devoted to developing advanced CO2 conversion systems, including CO2 electrochemical (Tripkovic et al., 2013; Ma et al., 2017; Wang et al., 2017), photocatalytic (Crake et al., 2017; Pipelzadeh et al., 2017; Cardoso et al., 2018; Li et al., 2019), and thermal catalytic reduction systems (Chaemchuen et al., 2019; Lin et al., 2019; Zeng et al., 2020). For these CO2 conversion systems, the key factor which impacts the efficiency and conversion rate is the CO2 reduction catalyst (Samanta et al., 2012; Zheng et al., 2017; Yaashikaa et al., 2019).
Metal-organic frameworks (MOFs), as an emerging category of porous materials, have attracted great interest due to their unique physicochemical properties, such as their highly specific surface area, tunable porosity, and controllable functionality (Liu et al., 2010; Aiyappa et al., 2019; Bhadra et al., 2019). Therefore, MOFs have been widely applied in various energy conversion and storage systems, such as gas separation and storage (Furukawa and Yaghi, 2009; Salehi and Anbia, 2017), drug delivery (Cai et al., 2019a,b; Safaei et al., 2019), biosensors (Zhang et al., 2016; Qiu et al., 2019), heterogeneous catalysis, and CO2/N2 conversion (An et al., 2017; Luo et al., 2019). However, because of their unsatisfactory electrical conductivity and stability, it greatly hinders their application in CO2 reduction processes. By thermal or chemical treatment, the pristine MOF composites can be converted into MOF-derived carbon materials with embedded metal nanoparticles or metal oxides (Qian et al., 2017; Bhadra et al., 2019; Shi et al., 2019). These MOF-derived carbon materials generally combine the advantageous physicochemical properties of the pristine MOF (porosity and tunable chemical compositions) and carbon/metal (high conductivity, active metal sites) which offers more possibilities for catalysis. The strategies of design of carbon materials derived from MOFs can not only improve the charge transportation abilities of materials and shorten the CO2 molecules diffusion path, but also create more active sites on the materials.
In this mini review, we highlight recent advances in the application of MOF-derived carbon materials for CO2 reduction processes, including electrochemical, photocatalytic, and thermal reductions. Recent progress and development of MOF-derived carbon materials for CO2 conversion were also discussed. Additionally, at the end of this review, we give a brief perspective of MOF-derived carbon materials in CO2 conversion.
Fabrication of MOF-Derived Carbon Materials
MOFs can be assembled by metal ions/clusters and organic linkers through coordination bonds by hydrothermal (Chen et al., 2020), microwave (The Ky et al., 2020), electrochemical (Vehrenberg et al., 2020), ultrasonic (Zhao et al., 2020), or hydrodynamic cavitation methods (Aiyappa et al., 2019; Kim et al., 2020; Sun et al., 2020a,b). Generally, the MOFs possess special properties, including porous structures and tunable chemical compositions which enable the desirable design of MOF-derived composites with high catalytic activity. In the first report of MOF-derived carbon materials (Liu et al., 2008), carbon materials were synthesized by thermal transformation of pristine MOFs. Due to the special structure of MOFs (a topology in which metal atoms are connected by ligands), metal oxides, metal phosphides, metal chalcogenides, and metal carbides can be synthesized in-situ in the carbon matrix. Researchers have studied the impact of synthesis conditions on the physicochemical properties of MOF-derived carbon materials. The results show that under the high-temperature conditions of pyrolysis treatment, the structure of pristine MOFs tended to collapse, and their porosities were damaged, leading to the aggregation of metal atoms in the carbon matrix. In addition, recent studies (Khalid et al., 2018; Bhadra et al., 2019) indicated that by choosing appropriate pristine MOFs and controlling the synthetic conditions subtly, the morphologies of pristine MOFs can be reserved after the pyrolysis process. The high-temperature treatment of pristine MOFs offers an effective way of precisely controlling the shape, size, and structure and at the same time maintains the materials' functionalities in one step. Generally, the most used pyrolysis synthetic methods of MOF-derived carbon materials can be categorized into two types: self-templating and external templating methods. In the self-templating method, only pristine MOF is pyrolyzed, while in the external templating method, pristine MOF, as well as some external templates (including metal nanoparticles, graphene, silica, and metal oxides) are pyrolyzed. A typical scheme of the self-templating method and external-templating method is shown in Figure 1. Besides the above-mentioned synthetic methods, the in-situ growth of MOF crystals on carbon materials is also an effective and easily performed method to synthesis MOF-derived carbon materials.
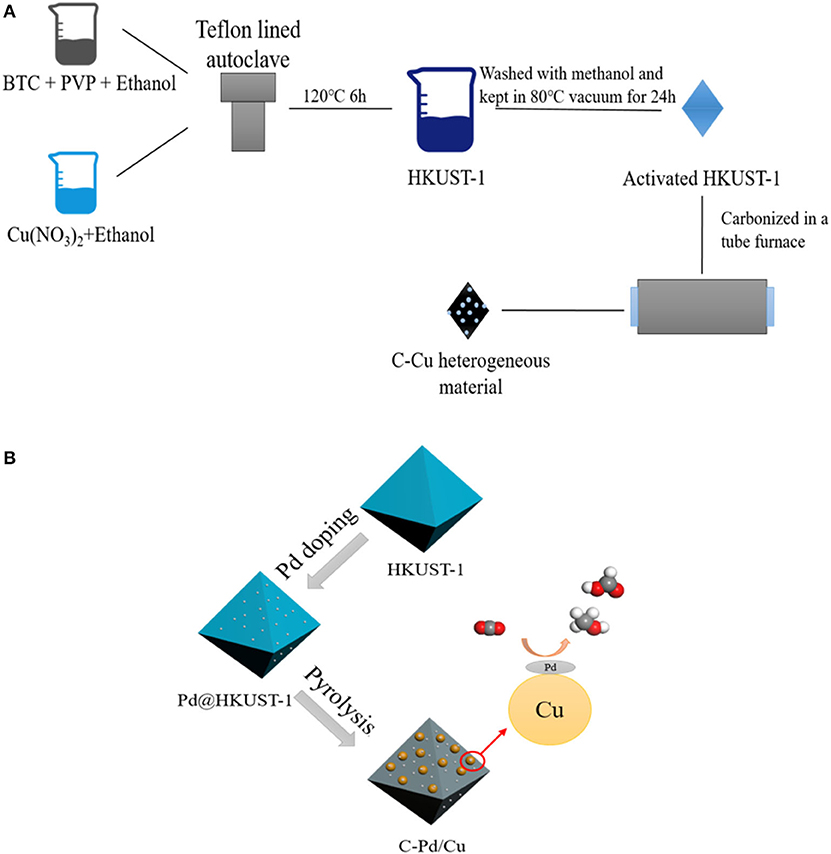
Figure 1. Typical synthetic process of MOF-derived carbon materials (HKUST-1 as the pristine MOF): self-templating method (A) and external-templating method (B). (BTC, 1,3,5-Benzenetricarboxylicacid; PVP, polyvinyl pyrrolidone; HKUST-1, a kind of Cu MOF).
MOF-Derived Carbon Materials in CO2 Electrochemical Reduction
As a promising approach to produce value-added products, the electrochemical reduction of CO2 has attracted many researchers since it was first reported by Hori et al. (1985). Double-cell reactors, with a Nafion membrane used to separate the cells, are widely used for this process (Figure 2A).
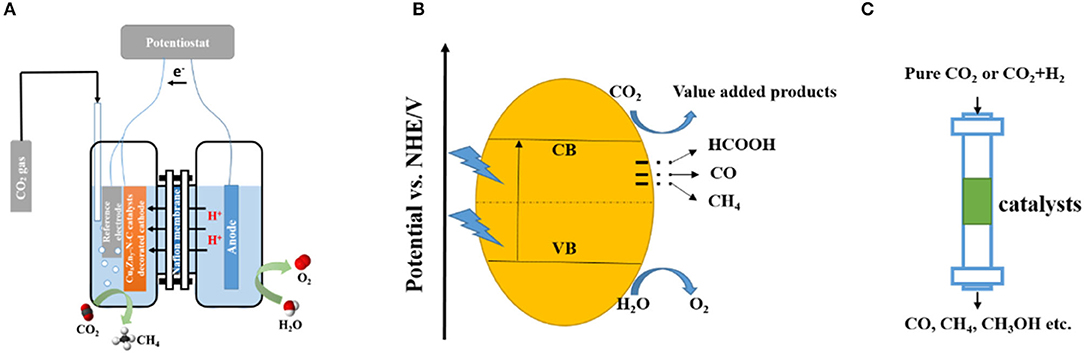
Figure 2. Scheme of CO2 reduction systems: electrochemical reduction system (A), photocatalytic reduction system (B), and thermal catalytic reduction system (C).
Typically, the oxidation reaction occurs on the anode of the system, water is oxidized to produce oxygen and protons, the proton then goes through the Nafion membrane to take part in the CO2 reduction reaction which happens on the cathode. Generally, the electrochemical reduction of CO2 involves different reaction pathways, which lead to various products (Equations 1–6). By tuning the compositions and functionalities of the electrochemical reduction catalysts, the selectivity and CO2 conversion rate can be largely improved.
As MOF-derived carbon materials possess the properties of high conductivity, large porosities, and highly active reaction centers, they are excellent catalysts for high-efficiency CO2 reduction.
Copper is reported to have high activity and selectivity in CO2 electrochemical reduction. Fabricating copper or copper oxides containing carbon materials based on MOFs offers a facile method to synthesize highly active CO2 electrochemical reduction catalysts (Xuan et al., 2019, 2020). The most used pristine Cu MOF in the fabrication of CO2 electrochemical catalysts is HKUST-1. Oxide-derived Cu/carbon (OD Cu/C) catalysts were synthesized by the carbonization of HKUST-1. Zhao et al. fabricated OD Cu/C catalysts with temperatures of 900, 1,000, and 1,100°C. The obtained materials exhibited high selectivity in CO2 reduction to alcohol products, and the highest selective CO2 reduction to ethanol was achieved on OD Cu/C-1000 at an overpotential of 190 mV. The electrochemical catalytic activity of this catalyst was attributed to the synergistic effect between the highly dispersed copper and the matrix of the porous carbon (Zhao et al., 2017). Cheng et al. used the external template method to synthesize a Pd nanoparticle-doped carbonized HKUST-1 catalyst (C-Pd/Cu). The noble metal-copper embedded in the carbon matrix structure enabled the fast transportation and easy adsorption of CO2 molecules, facilitating CO2 hydrogenation (Cheng et al., 2019b).
Due to its special surface charge density distribution which leads to high CO2 adsorption ability, metal-N sites containing MOF-derived carbon materials have recently attracted attention (Cheng et al., 2019a). Bao's group has devoted large efforts to this area. Fe-N and Ni-N active sites containing ZIF-derived carbon materials were successfully synthesized by direct carbonization of pristine Fe/Ni-doped ZIF. Ammonia treatment was also employed on the Fe-N-containing catalyst to improve the specific area and mesopore areas, thus effectively boosting CO2 reduction (Yan et al., 2018b). A coordinatively unsaturated Ni-N active site containing a ZnNi ZIF-derived porous carbon catalyst was also fabricated and employed in CO2 electrochemical reduction. The highest CO Faradaic efficiency of 98% was achieved. Density functional theory (DFT) calculations revealed that the CO2 reduction reaction was favored more than the hydrogen evolution reaction over Ni-N sites embedded in the porous carbon structure (Yan et al., 2018a).
MOF-Derived Carbon Materials in CO2 Photocatalytic Reduction
Photocatalytic CO2 reduction is a process in which the catalyst absorbs sunlight radiation and creates electron-hole pairs to evoke CO2 molecules, generating value-added products (Figure 2B). In this energy converting process, three fundamental steps are needed: (1) sunlight absorption by the catalyst to create electro-hole pairs; (2) generation and migration of redox equivalents; and (3) reduction and oxidation reactions with the redox equivalents at the catalytic active centers (Zhang and Lin, 2014).
Due to their unique structures, tunable compositions, and porosities, MOF-derived carbon materials can contain photosensitizers and catalytic active centers in a single solid. A number of recent studies have demonstrated that MOF-derived carbon materials show high catalytic activity in CO2 photocatalytic reduction. Hu et al. reported an HKUST-1-derived hollow C-Cu2−xS nanotube/g-C3N4 composite for CO2 photoreduction with H2O vapor. During the CO2 photocatalytic reduction process, the carbon coat in the catalyst acted as an electron reservoir, which facilitated electron-hole pair separation. The optimized C-Cu2−xS@g-C3N4 acted as a photocatalyst. The reactivity and selectivity were boosted to 1062.6 μmol g−1 and 97% respectively, which were much higher than those when bare Cu2S or g-C3N4 was applied under the same conditions (Hu et al., 2019).
In-situ growth of MOF crystals on carbon materials is an effective method to synthesize MOF-derived carbon materials that possess both the properties of porous MOF and carbon materials. Liu et al. fabricated a g-C3N4/ZIF-8 composite by a simple in-situ heterogeneous deposition method. The designed hybrid photocatalyst not only inherited the broadened optical properties of g-C3N4, but also had a high CO2 adsorption capacity due to the porous structures of ZIF-8. Herein, the g-C3N4/ZIF-8 photocatalyst exhibited much higher activity for CO2 photocatalytic reduction, reaching 0.75 μmol h−1g−1 under 365 nm light radiation. This study provided a promising method for the easy synthesis of MOF-derived carbon materials (Liu et al., 2017).
MOF-derived carbon materials offer the possibility of tuning the size of active sites in the photocatalyst. Mu et al. successfully synthesized a series of C-BMZIF with a rhombic dodecahedral structure. The Zn and reduced Co presented in the carbon matrix could prevent Co active sites from aggregation. They came up with the idea that the size of Co active sites could be regulated by adjusting the Zn/Co ratio, thus influencing the compositions of the resulting products (Mu et al., 2018).
MOF-Derived Carbon Materials in CO2 Thermal Catalytic Reduction
CO2 thermal catalytic reduction is an approach in which high temperature and pressure are applied to realize the conversion of CO2 into value-added products (Figure 2C). Compared with the above-mentioned methods to convert CO2 into value-added products, the CO2 thermal catalytic reduction method has a longer history. Nowadays, various approaches to CO2 thermal conversion have been widely used in industrial-scale applications. Generally, industrial-scale CO2 thermal catalytic reduction approaches can be divided into two types: pure CO2 decomposition and CO2 conversion with a co-reactant. As the CO2 thermal catalytic reduction process involves high temperature and high pressure, therefore, catalysts with high stability are required. The carbon matrix structure enables MOF-derived carbon material to endure the high temperature in CO2 thermal catalytic reduction and at the same time offers more anchoring dots for the CO2 molecules.
A robust and easily collected pyrolyzed bimetallic Zn/Co ZIF catalyst was successfully synthesized by Chaemchuen et al., and it showed high catalytic activity for the cycloaddition of CO2 into epoxides. The metal dispersion and catalytic properties of the catalyst were improved by the Co species hybrid with N species in the carbon matrix wall (Chaemchuen et al., 2019).
Lu et al. pyrolyzed ZIF-67 at a temperature of 700°C to fabricate a cobalt-based nonprecious metal catalyst for CO2 hydrogenation. The Mott-Schottky effect at the metal-support interface in the catalyst caused an electron transfer over the Schottky barrier. The first-principle mechanistic study revealed that the Co embedded in the carbon matrix could increase CO2 activation during the reaction (Lu et al., 2019). Lin et al. presented a facile synthesis of hierarchical Ni@C spheres with Ni nanoparticles confined in carbon shells for CO2 methanation under relatively low temperatures. The hollow and porous structures of the catalyst afforded a high surface area and isolated more active sites for CO2 methanation, therefore, resulting in high activity and superior selectivity in the CO2 thermal catalytic reduction (Lin et al., 2019).
Conclusions and Perspectives
In this mini review, the application of MOF-derived carbon materials in CO2 reduction was summarized. MOF-derived carbon materials inherit the properties of high specific surface area, porous structures, and tunable compositions from pristine MOF. Furthermore, their charge transportation ability, electron-hole separation ability, and stability are improved comparing with pristine MOF. Though MOF-derived carbon materials have been widely used in the field of CO2 reduction, there still remain problems to be solved:
(1) The reaction mechanisms of the CO2 electrochemical reduction in the aqueous phase electrolyte on MOF-derived carbon materials are still not clear. In-situ detecting methods should be utilized to further clarify the reaction pathways of CO2 reduction for various products.
(2) The porous structure of the carbon matrix in MOF-derived carbon materials is unstable and prone to collapse during the pyrolysis and reduction reaction process. Substituting the internal carbon matrix with external carbon templates and building more robust MOF-derived carbon materials could be a solution for this issue.
(3) The cost of MOF-derived carbon materials is high compared with traditional catalysts. To satisfy the need of MOF-derived carbon material applications in the industrial scale CO2 reduction process, large scale MOF-derived carbon material synthetic methods should be developed to lower the cost and improve the efficiency.
Author Contributions
XS, JY, and SC contributed the conception of the study. XX produced and wrote the article. XS, GB, and SZ edited the article. All authors contributed to the article and approved the submitted version.
Funding
This work was supported by the National Natural Science Foundation of China (51906125); China Postdoctoral Science Foundation (2020T130364 and 2019M650162); Youth Interdisciplinary Science and Innovative Research Groups of Shandong University (2020QNQT014); Young Scholars Program of Shandong University; Fundamental Research Funds of Shandong University (2019HW027 and 2020GN050); Key Laboratory of High efficiency and Clean Mechanical Manufacture at Shandong University, Ministry of Education; and Ocean Industry Leading Talent Team of Yantai's Double Hundred Plan.
Conflict of Interest
The authors declare that the research was conducted in the absence of any commercial or financial relationships that could be construed as a potential conflict of interest.
References
Aiyappa, H. B., Masa, J., Andronescu, C., Muhler, M., Fischer, R. A., and Schuhmann, W. (2019). MOFs for electrocatalysis: from serendipity to design strategies. Small Methods 3:415. doi: 10.1002/smtd.201800415
An, B., Zhang, J. Z., Cheng, K., Ji, P. F., Wang, C., and Lin, W. B. (2017). Confinement of ultrasmall Cu/ZnOx nanoparticles in metal-organic frameworks for selective methanol synthesis from catalytic hydrogenation of CO2. J. Am. Chem. Soc. 139, 3834–3840. doi: 10.1021/jacs.7b00058
Bhadra, B. N., Vinu, A., Serre, C., and Jhung, S. H. (2019). MOF-derived carbonaceous materials enriched with nitrogen: preparation and applications in adsorption and catalysis. Mater. Today 25, 88–111. doi: 10.1016/j.mattod.2018.10.016
Cai, W., Wang, J., Chu, C., Chen, W., Wu, C., and Liu, G. (2019a). Metal organic framework-based stimuli-responsive systems for drug delivery. Adv. Sci. 6:1526. doi: 10.1002/advs.201801526
Cai, X., Deng, X., Xie, Z., Shi, Y., Pang, M., and Lin, J. (2019b). Controllable synthesis of highly monodispersed nanoscale Fe-soc-MOF and the construction of Fe-soc-MOF@polypyrrole core-shell nanohybrids for cancer therapy. Chem. Eng. J. 358, 369–378. doi: 10.1016/j.cej.2018.10.044
Cardoso, J.C., Stulp, S., de Brito, J.F., Flor, J.B.S., Frem, R.C.G., and Zanoni, M.V.B. (2018). MOFs based on ZIF-8 deposited on TiO2 nanotubes increase the surface adsorption of CO2 and its photoelectrocatalytic reduction to alcohols in aqueous media. Appl. Catal. B-Environ. 225, 563–573. doi: 10.1016/j.apcatb.2017.12.013
Chaemchuen, S., Xiao, X., Ghadamyari, M., Mousavi, B., Klomkliang, N., Yuan, Y., et al. (2019). Robust and efficient catalyst derived from bimetallic Zn/Co zeolitic imidazolate frameworks for CO2 conversion. J. Catalysis 370, 38–45. doi: 10.1016/j.jcat.2018.11.027
Chen, X., Li, Q., Li, J., Chen, J., and Jia, H. (2020). Modulating charge separation via in situ hydrothermal assembly of low content Bi2S3 into UiO-66 for efficient photothermocatalytic CO2 reduction. Appl. Catal. B-Environ. 270:118915. doi: 10.1016/j.apcatb.2020.118915
Cheng, J., Xuan, X., Yang, X., Zhou, J., and Cen, K. (2019a). Enhanced photoelectrochemical hydrogenation of green-house gas CO2 to high-order solar fuel on coordinatively unsaturated metal-N sites containing carbonized Zn/Co ZIFs. Int. J. Hydrog. Energy 44, 21597–21606. doi: 10.1016/j.ijhydene.2019.06.102
Cheng, J., Xuan, X., Yang, X., Zhou, J., and Cen, K. (2019b). Selective reduction of CO2 to alcohol products on octahedral catalyst of carbonized Cu(BTC) doped with Pd nanoparticles in a photoelectrochemical cell. Chem. Eng. J. 358, 860–868. doi: 10.1016/j.cej.2018.10.091
Crake, A., Christoforidis, K. C., Kafizas, A., Zafeiratos, S., and Petit, C. (2017). CO2 capture and photocatalytic reduction using bifunctional TiO2/MOF nanocomposites under UV-vis irradiation. Appl. Catal. B-Environ. 210, 131–140. doi: 10.1016/j.apcatb.2017.03.039
Furukawa, H., and Yaghi, O. M. (2009). Storage of hydrogen, methane, and carbon dioxide in highly porous covalent organic frameworks for clean energy applications. J. Am. Chem. Soc. 131:8875. doi: 10.1021/ja9015765
Hori, Y., Kikuchi, K., and Suzuki, S. (1985). Production of CO and CH4 in electrochemical reduction of CO2 at metal electrodes in aqueous hydrogencarbonate solution. Chem. Lett. 14, 1695–1698. doi: 10.1246/cl.1985.1695
Hu, C.-Y., Zhou, J., Sun, C.-Y., Chen, M.-m., Wang, X.-L., and Su, Z.-M. (2019). HKUST-1 derived hollow C-Cu2-xS nanotube/g-C3N4 composites for visible-light CO2 photoreduction with H2O vapor. Chem. Eur. J. 25, 379–385. doi: 10.1002/chem.201804925
Khalid, M., Honorato, A. M. B., Varela, H., and Dai, L. (2018). Multifunctional electrocatalysts derived from conducting polymer and metal organic framework complexes. Nano Energy 45, 127–135. doi: 10.1016/j.nanoen.2017.12.045
Kim, H., Koo, B., Sun, X., and Yoon, J. Y. (2020). Investigation of sludge disintegration using rotor-stator type hydrodynamic cavitation reactor. Separation Purification Technol. 240:116636. doi: 10.1016/j.seppur.2020.116636
Li, X., Yu, J., Jaroniec, M., and Chen, X. (2019). Cocatalysts for selective photoreduction of CO2 into solar fuels. Chem. Rev. 119, 3962–4179. doi: 10.1021/acs.chemrev.8b00400
Lin, X., Wang, S., Tu, W., Hu, Z., Ding, Z., Hou, Y., et al. (2019). MOF-derived hierarchical hollow spheres composed of carbon-confined Ni nanoparticles for efficient CO2 methanation. Catal. Sci. Technol. 9, 731–738. doi: 10.1039/C8CY02329H
Liu, B., Shioyama, H., Akita, T., and Xu, Q. (2008). Metal-organic framework as a template for porous carbon synthesis. J. Am. Chem. Soc. 130, 5390–5391. doi: 10.1021/ja7106146
Liu, B., Shioyama, H., Jiang, H., Zhang, X., and Xu, Q. (2010). Metal–organic framework (MOF) as a template for syntheses of nanoporous carbons as electrode materials for supercapacitor. Carbon 48, 456–463. doi: 10.1016/j.carbon.2009.09.061
Liu, S., Chen, F., Li, S., Peng, X., and Xiong, Y. (2017). Enhanced photocatalytic conversion of greenhouse gas 2 into solar fuels over g-C3N4 nanotubes with decorated transparent ZIF-8 nanoclusters. Appl. Catal. B-Environ. 211, 1–10. doi: 10.1016/j.apcatb.2017.04.009
Lu, X., Liu, Y., He, Y., Kuhn, A. N., P.-,Shih, C., C.-,Sun, J., et al. (2019). Cobalt-based nonprecious metal catalysts derived from metal-organic frameworks for high-rate hydrogenation of carbon dioxide. ACS Appl. Mater. Interfaces 11, 27717–27726. doi: 10.1021/acsami.9b05645
Luo, S., Li, X., Zhang, B., Luo, Z., and Luo, M. (2019). MOF-derived Co3O4@NC with core-shell structures for N-2 electrochemical reduction under ambient conditions. ACS Appl. Mater. Interfaces 11, 26891–26897. doi: 10.1021/acsami.9b07100
Ma, M., Hansen, H. A., Valenti, M., Wang, Z., Cao, A., Dong, M., et al. (2017). Electrochemical reduction of CO2 on compositionally variant Au-Pt bimetallic thin films. Nano Energy 42, 51–57. doi: 10.1016/j.nanoen.2017.09.043
Mu, Q., Zhu, W., Yan, G., Lian, Y., Yao, Y., Li, Q., et al. (2018). Activity and selectivity regulation through varying the size of cobalt active sites in photocatalytic CO2 reduction. J. Mater. Chem. A 6, 21110–21119. doi: 10.1039/C8TA06151C
Pipelzadeh, E., Rudolph, V., Hanson, G., Noble, C., and Wang, L. (2017). Photoreduction of CO2 on ZIF-8/TiO2 nanocomposites in a gaseous photoreactor under pressure swing. Appl. Catal. B Environ. 218, 672–678. doi: 10.1016/j.apcatb.2017.06.054
Qian, Y., Khan, I. A., and Zhao, D. (2017). Electrocatalysts derived from metal-organic frameworks for oxygen reduction and evolution reactions in aqueous media. Small 13:1143. doi: 10.1002/smll.201701143
Qiu, Q., Chen, H., Wang, Y., and Ying, Y. (2019). Recent advances in the rational synthesis and sensing applications of metal-organic framework biocomposites. Coordination Chem. Rev. 387, 60–78. doi: 10.1016/j.ccr.2019.02.009
Ran, J., Jaroniec, M., and Qiao, S-Z. (2018). Cocatalysts in semiconductor-based photocatalytic CO2 reduction: achievements, challenges, and opportunities. Adv. Mater. 30:4649. doi: 10.1002/adma.201704649
Safaei, M., Foroughi, M. M., Ebrahimpoor, N., Jahani, S., Omidi, A., and Khatami, M. (2019). A review on metal-organic frameworks: synthesis and applications. Trac-Trends Anal. Chem. 118, 401–425. doi: 10.1016/j.trac.2019.06.007
Salehi, S., and Anbia, M. (2017). High CO2 adsorption capacity and CO2/CH4 selectivity by nanocomposites of MOF-199. Energy Fuels 31, 5376–5384. doi: 10.1021/acs.energyfuels.6b03347
Samanta, A., Zhao, A., Shimizu, G. K. H, Sarkar, P., and Gupta, R. (2012). Post-combustion CO2 capture using solid sorbents: a review. Ind. Eng. Chem. Res. 51:1438. doi: 10.1021/ie200686q
Shi, K., Yang, H., Lian, Y., and Peng, Y. (2019). Hierarchical structure N-CoFe-C@NF derived from MOF for rechargeable zinc-air battery. Chin. Sci. Bull. Chinese 64, 3378–3384. doi: 10.1360/TB-2019-0539
Sun, X., Jia, X., Liu, J., Wang, G., Zhao, S., Ji, L. J, and Yong Yoon Chen, S. (2020a). Investigation on the characteristics of an advanced rotational hydrodynamic cavitation reactor for water treatment. Separation Purification Technol. 251:117252. doi: 10.1016/j.seppur.2020.117252
Sun, X., Liu, J., Ji, L., Wang, G., Zhao, S., Yoon, J. Y., and Chen, S. (2020b). A review on hydrodynamic cavitation disinfection: the current state of knowledge. Sci. Total Environ. 737:139606. doi: 10.1016/j.scitotenv.2020.139606
The Ky, V. L, Van Nhieu, N., Van Cuong Song, M., Kim, D., and Yoo, K. S. (2020). Microwave-assisted continuous-flow synthesis of mixed-ligand UiO-66(Zr) frameworks and their application to toluene adsorption. J. Indus. Eng. Chem. 86, 178–185. doi: 10.1016/j.jiec.2020.03.001
Tripkovic, V., Vanin, M., Karamad, M. M. E, Björketun Jacobsen, K. W., Thygesen, K. S., et al. (2013). Electrochemical CO2 and CO reduction on metal-functionalized porphyrin-like graphene. J. Phys. Chem. C 117, 9187–9195. doi: 10.1021/jp306172k
Vehrenberg, J., Vepsalainen, M., Macedo, D. S. M, Rubio-Martinez Webster, N. A. S., et al. (2020). Steady-state electrochemical synthesis of HKUST-1 with polarity reversal. Micropor. Mesopor. Mater. 303:110218. doi: 10.1016/j.micromeso.2020.110218
Wang, Y., Hou, P., Wang, Z., and Kang, P. (2017). Zinc imidazolate metal-organic frameworks (ZIF-8) for electrochemical reduction of CO2 to CO. Chemphyschem 18, 3142–3147. doi: 10.1002/cphc.201700716
Xuan, X., Cheng, J., Yang, X., and Zhou, J. (2020). Highly selective electrochemical reduction of CO2 to CH4 over vacancy-metal-nitrogen sites in an artificial photosynthetic cell. ACS Sustain. Chem. Eng. 8, 1679–1686. doi: 10.1021/acssuschemeng.9b07258
Xuan, X., Cheng, J., Yang, X., Zhou, J., and Cen, K. (2019). Solar driven reduction of CO2 using Pt-Cu/C as a catalyst in a photoelectrochemical cell: experiment and mechanism study. RSC Adv. 9, 10635–10644. doi: 10.1039/C9RA00176J
Yaashikaa, P. R. P, Senthil Kumar Varjani, S. J., and Saravanan, A. (2019). A review on photochemical, biochemical and electrochemical transformation of CO2 into value-added products. J. CO2 Util. 33, 131–147. doi: 10.1016/j.jcou.2019.05.017
Yan, C., Li, H., Ye, Y., Wu, H., Cai, F., Si, R., et al. (2018a). Coordinatively unsaturated nickel-nitrogen sites towards selective and high-rate CO2 electroreduction. Energy Environ. Sci 11, 1204–1210. doi: 10.1039/C8EE00133B
Yan, C., Ye, Y., Lin, L., Wu, H., Jiang, Q., Wang, G., and Bao, X. (2018b). Improving CO2 electroreduction over ZIF-derived carbon doped with Fe-N sites by an additional ammonia treatment. Catal. Today. 330, 252–258. doi: 10.1016/j.cattod.2018.03.062
Zeng, L., Wang, Y., Li, Z., Song, Y., Zhang, J., Wang, J., et al. (2020). Highly dispersed Ni catalyst on metal-organic framework-derived porous hydrous zirconia for CO2 methanation. ACS Appl. Mater. Interfaces 12, 17436–17442. doi: 10.1021/acsami.9b23277
Zhang, E., Xie, Y., Ci, S., Jia, J., and Wen, Z. (2016). Porous Co3O4 hollow nanododecahedra for nonenzymatic glucose biosensor and biofuel cell. Biosens. Bioelectr. 81, 46–53. doi: 10.1016/j.bios.2016.02.027
Zhang, T., and Lin, W. (2014). Metal–organic frameworks for artificial photosynthesis and photocatalysis. Chem. Soc. Rev. 43, 5982–5993. doi: 10.1039/C4CS00103F
Zhao, K., Liu, Y., Quan, X., Chen, S., and Yu, H. (2017). CO2 electroreduction at low overpotential on oxide-derived Cu/Carbons fabricated from metal organic framework. ACS Appl. Mater. Interfaces 9, 5302–5311. doi: 10.1021/acsami.6b15402
Zhao, L., Zhao, Z., Li, Y., Chu, X., Li, Z., Qu, Y., et al. (2020). The synthesis of interface-modulated ultrathin Ni(ii) MOF/g-C3N4 heterojunctions as efficient photocatalysts for CO2 reduction. Nanoscale 12, 10010–10018. doi: 10.1039/D0NR02551H
Keywords: carbon dioxide CO2 reduction, nanomaterials, MOFs, green chemistry, carbon catalysts
Citation: Xuan X, Chen S, Zhao S, Yoon JY, Boczkaj G and Sun X (2020) Carbon Nanomaterials From Metal-Organic Frameworks: A New Material Horizon for CO2 Reduction. Front. Chem. 8:573797. doi: 10.3389/fchem.2020.573797
Received: 18 June 2020; Accepted: 17 August 2020;
Published: 14 October 2020.
Edited by:
Xingtao Xu, National Institute for Materials Science, JapanReviewed by:
Leiqing Hu, University at Buffalo, United StatesWei Xia, Nanjing University of Aeronautics and Astronautics, China
Copyright © 2020 Xuan, Chen, Zhao, Yoon, Boczkaj and Sun. This is an open-access article distributed under the terms of the Creative Commons Attribution License (CC BY). The use, distribution or reproduction in other forums is permitted, provided the original author(s) and the copyright owner(s) are credited and that the original publication in this journal is cited, in accordance with accepted academic practice. No use, distribution or reproduction is permitted which does not comply with these terms.
*Correspondence: Xun Sun, eHVuc3VuJiN4MDAwNDA7c2R1LmVkdS5jbg==