- 1Neuroscience Research Center, Kerman University of Medical Sciences, Kerman, Iran
- 2Leishmaniasis Research Center, Kerman University of Medical Sciences, Kerman, Iran
- 3Research Center for Tropical and Infectious Diseases, Kerman University of Medical Sciences, Kerman, Iran
- 4Environment Department, Institute of Science and High Technology and Environmental Sciences, Graduate University of Advanced Technology, Kerman, Iran
- 5Department of Materials Science and Engineering, Research Institute of Advanced Materials, Seoul National University, Seoul, South Korea
- 6Institute of Research and Development, Duy Tan University, Da Nang, Vietnam
- 7Innovative Enterprise Cooperation Center, Korea Institute of Science and Technology, Seoul, South Korea
- 8College of Forestry, Henan Agricultural University, Zhengzhou, China
- 9School of Automotive Engineering, Huanghe Jiaotong University, Jiaozuo, China
In this paper, graphene (Gr)/Co3O4 nanocomposite was synthesized and utilized for the development of a novel electrochemical sensor to detect tramadol. Tramadol determination was examined by linear sweep voltammetry, differential pulse voltammetry, cyclic voltammetry, and chronoamperometry on Gr/Co3O4 nanocomposite-modified screen-printed electrode (Gr/Co3O4/SPE) in phosphate-buffered saline (PBS). Under the optimized condition, the detection limit of tramadol is 0.03 μM (S/N = 3) in the linear ranges of 0.1–500.0 μM. Furthermore, Gr/Co3O4/SPE was satisfactorily utilized to detect tramadol in tramadol tablet and urine specimens.
Introduction
Tramadol is a synthetic analog of codeine that acts as a central analgesic. In terms of structure, there is a relationship between tramadol and codeine and morphine. Moreover, it acts as one of the opioid agonists in the body (Abu-Shawish et al., 2010). In addition, it is possible to use tramadol alone or combined with additional non-steroidal anti-inflammatory drugs (NSAIDs) when dealing with patients who have serious chronic pain, depression, spinal cord injuries, low back pain, and post-operative pain (Tetsunaga et al., 2015). Furthermore, it is increasingly being abused by opioid addicts. However, usual dosing requirements for oral consumption of the drug ranges between 50 and 100 mg per 4–6 h, with the maximum dose being 400 mg a day (Thévenin et al., 2008). Overdosing on tramadol can cause vomiting, problems in the central nervous system (CNS) and respiratory system, depression, dizziness, nausea, coma, tachycardia, and seizure (Scott and Perry, 2000). Tramadol is rapidly and nearly completely absorbed in oral usage, but excretion of roughly 10–30% of the parent drug happens un-metabolized in urine (Mohammadpour et al., 2019). Thus, for reasons related to treatment and the drug, it is of special significance to propose a sensitive technique to measure specimens containing of tramadol. At present, many methods have been employed for measuring tramadol quantitatively, such as high-performance liquid chromatography (Kmetec and Roškar, 2003; Saccomanni et al., 2010), gas chromatography (Ghasemi, 2012), gas chromatography/mass spectrometry (El-Sayed et al., 2013), electrochemiluminescence (Ding et al., 2006), spectrophotometry (Glavanović et al., 2016), capillary electrophoresis (Cunha et al., 2017), and electrochemistry (Ghorbani-Bidkorbeh et al., 2010; Babaei et al., 2011; Chitravathi and Munichandraiah, 2016; Madrakian et al., 2017; Hassannezhad et al., 2019; Hassanvand and Jalali, 2019; Rokhsefid and Shishehbore, 2019).
Nevertheless, some of these methods are limited due to complex sample procurement stages and the utilization of organic solvents. Electrochemical techniques have been proposed instead when analyzing drug and biological specimens because of the simplification, speed, inexpensive instruments, higher sensitivity, and precise analytical devices (Mahmoudi-Moghaddam et al., 2014; Prasad and Sreedhar, 2018; Elobeid and Elbashir, 2019; Tan et al., 2020; Xia et al., 2020). Considering the above features to detect diverse significant samples, researchers utilized many procedures for improving the electrode modification in order to enhance selectivity and sensitivity (Beitollahi and Sheikhshoaie, 2012; Soltani et al., 2014; Zhang et al., 2015; Deshmukh et al., 2018; Ghodsi et al., 2018; Mohammadi et al., 2018; Rabiee et al., 2018).
Nanomaterials are defined as substances possessing at least one dimension with ~100 nanometers. They are very interesting materials because of their unique electrical, magnetic, and optical, properties. These emergent features provide the potential for great impacts in medicine, electronics, and other fields (Zhu et al., 2015; Abdussalam-Mohammed, 2019; Elumalai et al., 2019). Now, interest has grown in using nanomaterials for modifying the electrode surface. However, owing to nanomaterials' very good catalytic features and conductivity, we can use them for enhancing the electron transfer between the electrode surface and target analyte. Moreover, they can be used as the catalyst for increasing the electrochemical reaction (Beitollahi et al., 2008, 2018; Li et al., 2010, 2016; Yang et al., 2014; Moghaddam et al., 2015; Zheng et al., 2018).
In this regard, Gr is an ideal nanomaterial for electrochemical processes owing to its larger surface area, reasonable electrical conductivity, and inexpensiveness (Shao et al., 2010). In the fabrication of the electrochemical sensors and biosensors, the Gr-based electrodes have been considered interesting films for the sensing platforms as they cause synergy of the electrocatalytic activities and augment the sensor sensitivity (Zhang et al., 2011). In fact, the Gr sheets are very good host materials to make nanocomposites for high performance electrochemical utilizations (Kim et al., 2009; Zhou et al., 2010; Vinodhkumar et al., 2018). Gr nanocomposites display acceptable benefits as one of the sensing platforms in electrochemical sensors (Luo et al., 2012; Thanh et al., 2016; Shahid et al., 2019). Combining the advantages of the unique features of Gr together with those ofCo3O4 (the increased absorption capability, larger active site, and wide availability), the Gr/Co3O4 nanocomposite provides stable and sensitive platforms for electroanalysis (Yavuz et al., 2013a,b; Feng et al., 2014). Therefore, we have used Gr/Co3O4 as one of the nanocomposite materials because it has the most reasonable features.
According to studies, the screen-printing technology used for microelectronics has a significant utilization for fabricating electrodes for disposable electrochemical sensors and biosensors (Tangkuaram et al., 2007; Gevaerd et al., 2020). In fact, an SPE enjoys simplified operation, versatility, inexpensiveness, portability, reliability, and lower sizes, and has the capability for mass production. Hence, it has widespread application in the field of electroanalytical chemistry (Nicholas et al., 2018; Khalilzadeh et al., 2020). Moreover, an SPE would avoid the cleaning procedure in contrast to the traditional electrodes, like a glassy carbon electrode (Renedo et al., 2007). SPE resolves the shortcomings of the traditional electrode systems, which require repeated recalibration and are not stable and suitable for on-site analyses, because their completion lasts several hours. Additionally, they require the use of professionals, as they need multiple isolation and washing phases. Therefore, the mentioned disadvantages of the traditional electrode systems resulted in their lower capability in comparison to the SPEs.
We aimed at providing a technique with higher simplification, sensitivity, and speed to electrochemically detect tramadol. Therefore, a Gr/Co3O4/SPE was fabricated by dropping Gr/Co3O4 over SPE to make a voltametric sensor as well as evaluating tramadol voltammetric behaviors. According to our analysis, Gr/Co3O4/SPE exhibited more robust electrochemical oxidation for tramadol with a more negative potential. The present method was tested and verified using tramadol tablet and urine specimens, showing reasonable precision and recovery. To the best of our knowledge, the detection of tramadol by using Gr/Co3O4/SPE has not been reported yet.
Experimental
Apparatus and Chemicals
Autolab potentiostat/galvanostat (PGSTAT 302N, Eco Chemie, the Netherlands) was used to measure electrochemicals. The use of General Purpose Electrochemical System (GPES) software aimed at controlling the experimental conditions. Moreover, SPE (DropSens; DRP-110: Spain) possessed three typical graphite counters, unmodified graphite working, and silver pseudo-reference electrodes. Metrohm 710 pH meter was utilized for pH measurements.
Tramadol and other remaining reagents represented an analytical grade. Their preparation was carried out through Merck (Darmstadt, Germany). Orthophosphoric acid and the associated salts with a pH ranging higher than 2.0–9.0 have been utilized to prepare the buffer solutions.
Synthesizing the Gr/Co3O4 Nanocomposite
The synthesis of the Gr/Co3O4 composite was done via the chemical deposition of Co3O4 particles over the graphene oxide (GO), which was accompanied by reducing GO to Gr in NaBH4 solution. It is notable that Li et al. fixed synthesis and composition of the Gr/Co3O4 composite (Li et al., 2011). Therefore, we poured the graphite oxide (0.1 g) into 200 mL of ultra-high purity water and placed it in an ultrasonic bath for 2 h in order to establish a GO suspension. Then, an aqueous solution (10 mL) of CoCl2 (1.4 g CoCl2.6H2O) was added in this suspension. The mixture was shaken for two hours for completion of the ion exchange. The next stage concerned the drop wise addition of an aqueous solution (10 mL) of NaOH (1 equivalent) to the obtained mix by shaking for another hour. Afterwards, we achieved the solid using centrifugation via the ultrahigh purity water. Next, the product was distributed into ultra-high pure water (25 mL). Hydrogen peroxide (30%, 1.5 mL) was added to the obtained mix. This mix has been sealed into a 50 mL Teflon-lined stainless-steel autoclave. Then, heating was performed to 100°C and it was kept at the mentioned temperature for four hours. Notably, we collected the solid product through centrifugation that had been accompanied by a suspension in the ultra-high purity water (25 mL). In addition, we added NaBH4 (0.25 g) into the obtained suspension, and transported it to the autoclave heated to 120°C. Finally, we kept it at the above temperature for four hours. Finally, we collected the obtained solid and used ultra-high purity water to wash it. The solid was dried to achieve the Gr/Co3O4 composite. The composite was gained as black powder.
Preparation of Electrode
The bare SPE was coated by a Gr/Co3O4 nanocomposite. To prepare the Gr/Co3O4 nanocomposite stock solution in 1 mL of aqueous solution, the Gr/Co3O4 nanocomposite (1 mg) was distributed by 30-min ultra-sonication, whereas the Gr/Co3O4 nanocomposite suspension aliquots (5 μl) were cast on working electrodes. Then it was kept until the solvent was evaporated in room temperature.
Preparation of Real Sample
Five 50 mg tramadol pills (Amin Co., Iran) were used to prepare a solution by dissolving the pills (250 mg) in water (25 ml) in exposure to ultra-sonication. Then, different dilutions were poured in 25 ml volumetric flasks and reached final volume with PBS at pH of 7.0. The standard addition method was used to measure the tramadol concentrations.
10 ml of directly refrigerated urine samples were centrifuged at 2,000 rpm for 15 min, followed by filtering the supernatant using a 0.45 μm filter. Then, different volumes of solution were distributed into a 25 ml volumetric flask and diluted with PBS (pH = 7.0) until the mark, which were anesthetized by various doses of tramadol. The standard addition method was used to determine the tramadol concentrations.
Result and Discussion
Characterization of Nanostructures
The energy-dispersive X-ray spectroscopy (EDX) elemental mapping has been analyzed for the Gr/Co3O4 nanocomposite for the control of distributing the elements found in the nanocomposite (Figure 1). In order to have a clear result, Figures 1A–C shows the independent elemental distribution of C, O, and Co. Figure 1A represents the existence of GO as the carbon materials whereas Figure 1B (O) and Figure 1C (Co) show a big area coverage because of the densely packaged Co3O4 nanoparticles. The presence of all elements (oxygen, carbon, and cobalt) in the Gr/Co3O4 nanocomposite was confirmed by EDS analysis (Figure 2). We applied the scanning electron microscope (SEM) for characterizing the morphology of the as-synthesized Gr/Co3O4 nanocomposite. A SEM image of the Gr/Co3O4 nanocomposite is given in Figure 3.
Electrochemical Behavior of Tramadol on the Gr/Co3O4/SPE
To study the electrochemical behaviors of tramadol, which was supposed to show dependence on pH, obtaining an optimum pH-value to reach acceptable outcomes is important. Thus, the modified electrode was employed in running the experiments with different pH values varying between 2.0 and 9.0. Eventually, the most desirable results were considered for electro-oxidation of tramadol at a pH of 7.0 (Figure 4).
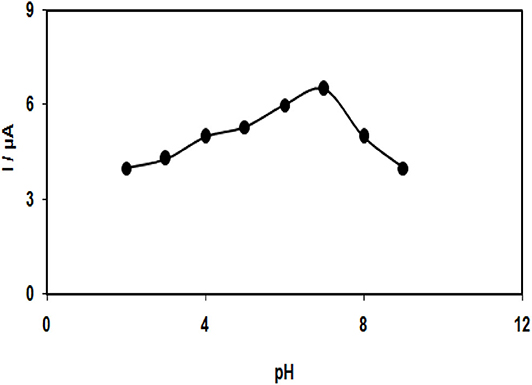
Figure 4. Plot of Ip vs. pHs of buffer solution (2.0, 3.0, 4.0, 5.0, 6.0, 7.0, 8.0, and 9.0) in the presence of 100.0 μM tramadol.
Figure 5 represents cyclic voltammograms in the presence of 100.0 μM tramadol with the bare SPE (Curve a) and Gr/Co3O4/SPE (Curve b). Based on the CV outputs, the greatest oxidation of tramadol on the Gr/Co3O4/SPE occurred at 700 mV, which was ~140 mV more negative than the bare SPE.
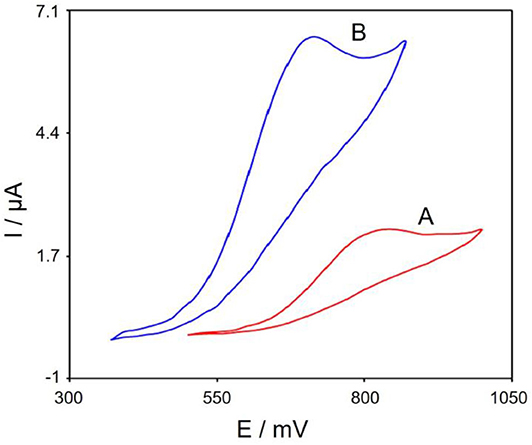
Figure 5. The CVs of (A) bare SPE, (B) Gr/Co3O4/SPE in 0.1 M PBS at pH of 7.0 when 100.0 μM tramadol is present at 50 mVs−1 scan rate.
The Scan Rate Effects on the Results
The association between peak current and scan rate would supply helpful information considering the electrochemical mechanisms. Therefore, the scan rate effects on the peak current of tramadol were examined using LSV, at a range of 10–300 mVs−1 in PBS (0.1 M, pH 7), according to Figure 6. The electrode response of tramadol was a diffusion-controlled procedure, as the oxidation peak current corresponded to the square root of the scan rate (Figure 6 inset).
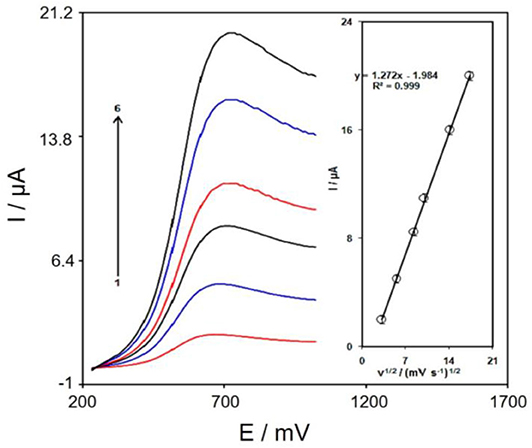
Figure 6. LSVs of Gr/Co3O4/SPE in 0.1 M PBS at pH of 7.0 including 150.0 μM tramadol at distinct scan rates; 1-6 respective to 10, 30, 70, 100, 200, and 300 mV s−1. Inset; variations in the anodic peak currents vs. ν1/2.
Chronoamperometric Analysis
Chronoamperometric study was used to calculate the diffusion coefficient (D) of tramadol at the surface Gr/Co3O4/SPE at an optimum condition. Figure 7 displays the chronoamperometric outputs of the tramadol sample at different concentrations (PBS at pH of 7.0). In addition, Cottrell equation was recommended to perform electroactive moiety chronoamperometric analyses according to the mass transfer restricted conditions (Bard and Faulkner, 2001):
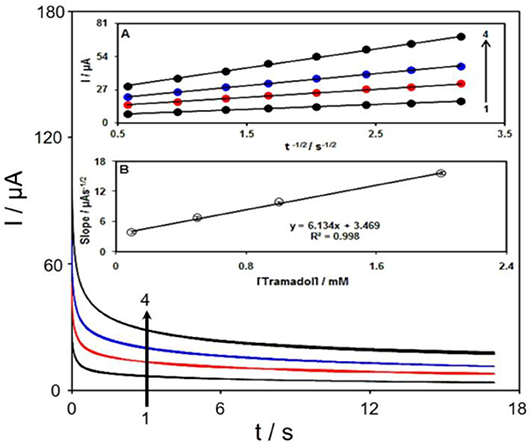
Figure 7. The chronoamperograms obtained at Gr/Co3O4/SPE in 0.1 M PBS at pH of 7.0 for tramadol at various concentrations. Accordingly, 1–4 relate to 0.1, 0.5, 1.0, and 2.0 mM of tramadol. Inset (A) the I plot vs. t−1/2 observed using chronoamperograms 1–5. (B) The straight-line slope plot vs. tramadol concentration.
Figure 7A indicates experimental findings regarding I vs. t−1/2, which shows the most acceptable fit for tramadol distinct concentrations. Then, the ultimate slopes relative to the straight lines in Figure 7A could be depicted vs. tramadol concentrations (Figure 7B). Thus, D mean-value equaled to 1.3 × 10−5 cm2/s with regard to Cottrell equation and resultant slopes.
Calibration Curve
The DPV method explored the association of the peak current with tramadol at different concentrations. As shown in Figure 8, the DPVs of Gr/Co3O4/SPE in the presence of different concentrations of tramadol was recorded in the concentration ranging from 00.5 to 500.0 μM. The detection limit 30.0 nM was established for analysis of tramadol at the surface of Gr/Co3O4/SPE. The response time was ~13 s.
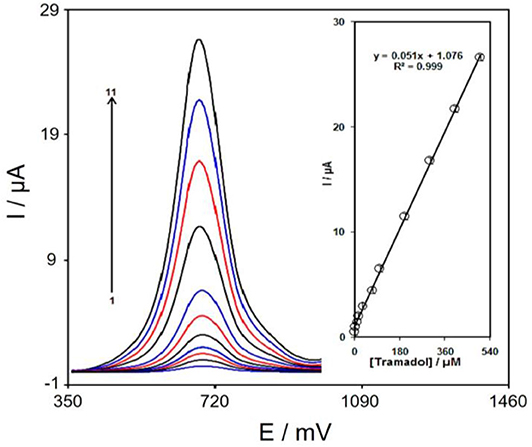
Figure 8. DPVs of Gr/Co3O4/SPE in 0.1 M PBS (pH 7.0) including tramadol at varying concentrations. 1–11 indicate 0.1, 2.0, 7.5, 15.0, 30.0, 70.0, 100.0, 200.0, 300.0, 400.0, and 500.0 μM of tramadol, respectively. The inset indicates the peak current plot as a function of the tramadol concentrations ranging from 0.1–500.0 μM.
The comparison study of the results for the determination of tramadol with different modified electrodes in the literature are listed in Table 1. The novel sensor presented in this study exhibits outstanding analytical performance for the determination of tramadol. The comparative data showed excellent efficiency of the proposed sensor over some earlier reported sensors. Thus, the fabricated electrode was appropriate for the determination of tramadol with the advantages of facile fabrication, ease of use, and low cost.
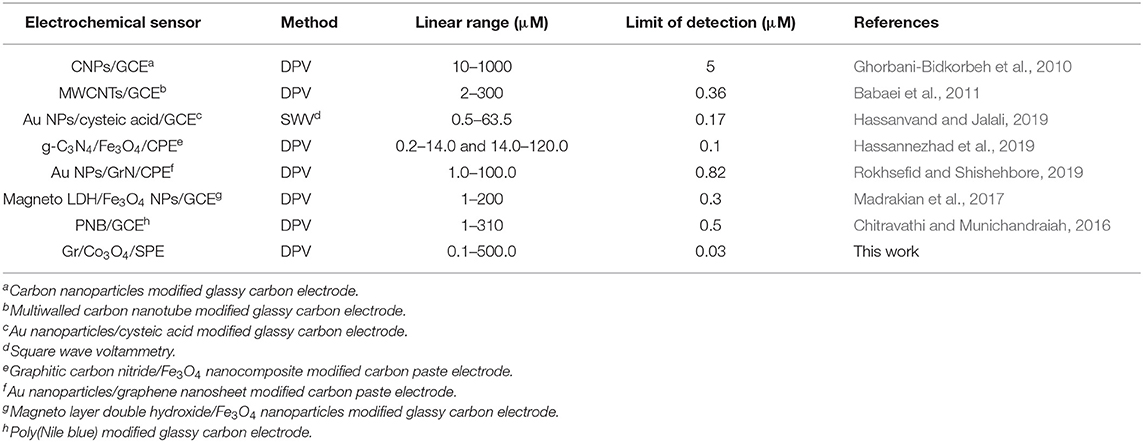
Table 1. Comparing performances of the purposed electrochemical sensor with others for determination of tramadol.
Stability and Repeatability of Gr/Co3O4/SPE
The stability of Gr/Co3O4/SPE was evaluated using records of the oxidation signal of 40.0 μM tramadol over 2 weeks. A 2.5% deviation was identified with compression of the first oxidation signal of tramadol after 2 weeks, indicating good stability of Gr/Co3O4/SPE as a voltammetric sensor.
Examination of the modified SPE anti-fouling features regarding tramadol oxidation and the corresponding products carried out through CV for the modified SPE prior and subsequent to application when tramadol was present. CVs were recorded when tramadol was present following cycling the potential 15 times at a 50 mV s−1. The currents were reduced by more than 2.3 %, while the peak potential faced no alterations.
Analyzing the Real Samples
Finally, Gr/Co3O4/SPE performance as a new electrochemical sensor used to analyze tramadol in tramadol tablet and urine samples was evaluated. Table 2 indicates the collected data and, according to the recovery data, Gr/Co3O4/SPE could be regarded as a sensitive sensor to analyze tramadol in actual samples.
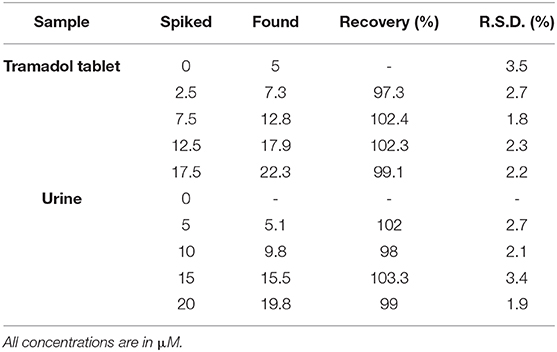
Table 2. The application of Gr/Co3O4/SPE for determination of tramadol in tramadol tablet and urine samples (n = 5).
Conclusions
We used a simplified procedure to synthesize Gr/Co3O4 and characterized it by EDX and SEM. Then, it was utilized for the electrochemical detection of tramadol. The modified electrode exhibited good electrocatalytic activity and sensitivity. Linear response of its peak current on tramadol concentrations ranged between 0.1 and 500.0 μM, and LOD was 0.03 μM. Finally, the modified electrode was substantially used for tramadol analysis in the real specimens. The proposed method offers a sensitive approach to detect tramadol in drug and biological formulations.
Data Availability Statement
The raw data supporting the conclusions of this article will be made available by the authors, without undue reservation.
Author Contributions
All authors have contributed to the scientific discussion, investigation, analysis, and manuscript writing and editing.
Funding
This research was supported by Kerman University of Medical Sciences, Kerman, Iran and by the Future Material Discovery Program (2016M3D1A1027666), the Basic Science Research Program (2017R1A2B3009135) through the National Research Foundation of Korea. Support from the Vietnam National Foundation for Science and Technology Development (NAFOSTED) under grant number of 104.05-2020.15 is also appreciated.
Conflict of Interest
The authors declare that the research was conducted in the absence of any commercial or financial relationships that could be construed as a potential conflict of interest.
References
Abdussalam-Mohammed, W. (2019). Review of the rapeutic applications of nanotechnology in medicine field and its side effects. J. Chem. Rev. 1, 243–251. doi: 10.33945/SAMI/JCR.2019.3.5
Abu-Shawish, H. M., Ghalwa, N. A., Zaggout, F. R., Saadeh, S. M., Al-Dalou, A. R., and Abou Assi, A. A. (2010). Improved determination of tramadol hydrochloride in biological fluids and pharmaceutical preparations utilizing a modified carbon paste electrode. Biochem. Eng. J. 48, 237–245. doi: 10.1016/j.bej.2009.10.019
Babaei, A., Taheri, A. R., and Afrasiabi, M. (2011). A multi-walled carbon nanotube-modified glassy carbon electrode as a new sensor for the sensitive simultaneous determination of paracetamol and tramadol in pharmaceutical preparations and biological fluids. J. Brazil. Chem. Soc. 22, 1549–1558. doi: 10.1590/S0103-50532011000800020
Bard, A. J., and Faulkner, L. R. (2001). Electrochemical Methods: Fundamentals and Applications. 2nd ed. New York, NY: Wiley.
Beitollahi, H., Dourandish, Z., Tajik, S., Ganjali, M. R., Norouzi, P., and Faridbod, F. (2018). Application of graphite screen printed electrode modified with dysprosium tungstate nanoparticles in voltammetric determination of epinephrine in the presence of acetylcholine. J. Rare Earth. 36, 750–757. doi: 10.1016/j.jre.2018.01.010
Beitollahi, H., Karimi-Maleh, H., and Khabazzadeh, H. (2008). Nanomolar and selective determination of epinephrine in the presence of norepinephrine using carbon paste electrode modified with carbon nanotubes and novel 2-(4-oxo-3-phenyl-3, 4-dihydro-quinazolinyl)-N′-phenyl-hydrazinecarbothioamide. Anal. Chem. 80, 9848–9851. doi: 10.1021/ac801854j
Beitollahi, H., and Sheikhshoaie, I. (2012). Novel nanostructure-based electrochemical sensor for simultaneous determination of dopamine and acetaminophen. Mater. Sci. Eng. C 32, 375–380. doi: 10.1016/j.msec.2011.11.009
Chitravathi, S., and Munichandraiah, N. (2016). Voltammetric determination of paracetamol, tramadol and caffeine using poly (Nile blue) modified glassy carbon electrode. J. Electroanal. Chem. 764, 93–103. doi: 10.1016/j.jelechem.2016.01.021
Cunha, R. R., Ribeiro, M. M., Muñoz, R. A., and Richter, E. M. (2017). Fast determination of codeine, orphenadrine, promethazine, scopolamine, tramadol, and paracetamol in pharmaceutical formulations by capillary electrophoresis. J. Sep. Sci. 40, 1815–1823. doi: 10.1002/jssc.201601275
Deshmukh, M. A., Celiesiute, R., Ramanaviciene, A., Shirsat, M. D., and Ramanavicius, A. (2018). EDTA_PANI/SWCNTs nanocomposite modified electrode for electrochemical determination of copper (II), lead (II) and mercury (II) ions. Electrochim. Acta 259, 930–938. doi: 10.1016/j.electacta.2017.10.131
Ding, S. N., Xu, J. J., Zhang, W. J., and Chen, H. Y. (2006). Tris (2,2′-bipyridyl) ruthenium (II)–Zirconia–Nafion composite modified electrode applied as solid-state electrochemiluminescence detector on electrophoretic microchip for detection of pharmaceuticals of tramadol, lidocaine and ofloxacin. Talanta 70, 572–577. doi: 10.1016/j.talanta.2006.01.017
Elobeid, W. H., and Elbashir, A. A. (2019). Development of chemically modified pencil graphite electrode based on benzo-18-crown-6 and multi-walled CNTs for determination of lead in water samples. Prog. Chem. Biochem. Res. 2, 24–33. doi: 10.33945/SAMI/PCBR.2019.2.2433
El-Sayed, A. A. Y., Mohamed, K. M., Nasser, A. Y., Button, J., and Holt, D. W. (2013). Simultaneous determination of tramadol, O-desmethyltramadol and N-desmethyltramadol in human urine by gas chromatography–mass spectrometry. J. Chromatogr. B 926, 9–15. doi: 10.1016/j.jchromb.2013.02.019
Elumalai, D., Sathiyaraj, M., Vimalkumar, E., Kaleena, P. K., Hemavathi, M., and Venkatesh, P. (2019). Bio fabricated of silver nanoparticles using Ocimum basilicum and its efficacy of antimicrobial and antioxidant activity. Asian J. Green Chem. 3, 103–124. doi: 10.22034/ajgc.2018.67295
Feng, Z. L., Yao, Y. Y., Xu, J. K., Zhang, L., Wang, Z. F., and Wen, Y. P. (2014). One-step co-electrodeposition of graphene oxide doped poly (hydroxymethylated-3,4-ethylenedioxythiophene) film and its electrochemical studies of indole-3-acetic acid. Chinese Chem. Lett. 25, 511–516. doi: 10.1016/j.cclet.2014.01.004
Gevaerd, A., Banks, C. E., Bergamini, M. F., and Marcolino-Junior, L. H. (2020). Nanomodified Screen-Printed Electrode for direct determination of Aflatoxin B1 in malted barley samples. Sens. Actuat. B Chem. 307:127547. doi: 10.1016/j.snb.2019.127547
Ghasemi, E. (2012). Optimization of solvent bar microextraction combined with gas chromatography mass spectrometry for preconcentration and determination of tramadol in biological samples. J. Chromatogr. A 1251, 48–53. doi: 10.1016/j.chroma.2012.06.060
Ghodsi, J., Rafati, A. A., and Shoja, Y. (2018). Determination of acetaminophen using a glassy carbon electrode modified by horseradish peroxidase trapped in MWCNTs/silica sol-gel matrix. Adv. J. Chem. A 1, 39–55. doi: 10.29088/SAMI/AJCA.2018.5.3955
Ghorbani-Bidkorbeh, F., Shahrokhian, S., Mohammadi, A., and Dinarvand, R. (2010). Simultaneous voltammetric determination of tramadol and acetaminophen using carbon nanoparticles modified glassy carbon electrode. Electrochim. Acta 55, 2752–2759. doi: 10.1016/j.electacta.2009.12.052
Glavanović, S., Glavanović, M., and Tomišić, V. (2016). Simultaneous quantitative determination of paracetamol and tramadol in tablet formulation using UV spectrophotometry and chemometric methods. Spectrochim. Acta A Mol. Biomol. Spectrosc. 157, 258–264. doi: 10.1016/j.saa.2015.12.020
Hassannezhad, M., Hosseini, M., Ganjali, M. R., and Arvand, M. (2019). A graphitic carbon nitride (gC3N4/Fe3O4) nanocomposite: an efficient electrode material for the electrochemical determination of tramadol in human biological fluids. Anal. Methods 11, 2064–2071. doi: 10.1039/C9AY00146H
Hassanvand, Z., and Jalali, F. (2019). Gold nanoparticles/cysteic acid modified electrode for simultaneous electrochemical determination of tramadol and paracetamol. Anal. Bioanal. Chem. Res. 6, 393–404. doi: 10.22036/abcr.2019.170247.1305
Khalilzadeh, M. A., Tajik, S., Beitollahi, H., and Venditti, R. A. (2020). Green synthesis of magnetic nanocomposite with iron oxide deposited on cellulose nanocrystals with copper (Fe3O4@CNC/Cu): investigation of catalytic activity for the development of a venlafaxine electrochemical sensor. Ind. Eng. Chem. Res. 59, 4219–4228. doi: 10.1021/acs.iecr.9b06214
Kim, K. S., Zhao, Y., Jang, H., Lee, S. Y., Kim, J. M., Kim, K. S., et al. (2009). Large-scale pattern growth of graphene films for stretchable transparent electrodes. Nature 457, 706–710. doi: 10.1038/nature07719
Kmetec, V., and Roškar, R. (2003). HPLC determination of tramadol in human breast milk. J. Pharm. Biomed. Anal. 32, 1061–1066. doi: 10.1016/S0731-7085(03)00209-7
Li, B., Cao, H., Shao, J., Li, G., Qu, M., and Yin, G. (2011). Co3O4@graphene composites as anode materials for high-performance lithium ion batteries. Inorg. Chem. 50, 1628–1632. doi: 10.1021/ic1023086
Li, D. W., Li, Y. T., Song, W., and Long, Y. T. (2010). Simultaneous determination of dihydroxybenzene isomers using disposable screen-printed electrode modified by multiwalled carbon nanotubes and gold nanoparticles. Anal. Methods 2, 837–843. doi: 10.1039/c0ay00076k
Li, Y., Zhai, X., Liu, X., Wang, L., Liu, H., and Wang, H. (2016). Electrochemical determination of bisphenol A at ordered mesoporous carbon modified nano-carbon ionic liquid paste electrode. Talanta 148, 362–369. doi: 10.1016/j.talanta.2015.11.010
Luo, L., Zhu, L., and Wang, Z. (2012). Nonenzymatic amperometric determination of glucose by CuO nanocubes–graphene nanocomposite modified electrode. Bioelectrochemistry 88, 156–163. doi: 10.1016/j.bioelechem.2012.03.006
Madrakian, T., Alizadeh, S., Bahram, M., and Afkhami, A. (2017). A novel electrochemical sensor based on magneto LDH/Fe3O4 nanoparticles@glassy carbon electrode for voltammetric determination of tramadol in real samples. Ionics 23, 1005–1015. doi: 10.1007/s11581-016-1871-2
Mahmoudi-Moghaddam, H., Beitollahi, H., Tajik, S., Malakootian, M., and Karimi-Maleh, H. (2014). Simultaneous determination of hydroxylamine and phenol using a nanostructure-based electrochemical sensor. Environ. Monit. Assess. 186, 7431–7441. doi: 10.1007/s10661-014-3938-8
Moghaddam, H. M., Beitollahi, H., Tajik, S., Sheikhshoaie, I., and Biparva, P. (2015). Fabrication of novel TiO2 nanoparticles/Mn (III) salen doped carbon paste electrode: application as electrochemical sensor for the determination of hydrazine in the presence of phenol. Environ. Monit. Assess. 187, 1–12. doi: 10.1007/s10661-015-4629-9
Mohammadi, S., Taheri, A., and Rezayati-Zad, Z. (2018). Ultrasensitive and selective non-enzymatic glucose detection based on pt electrode modified by carbon nanotubes@graphene oxide/nickel hydroxide-Nafion hybrid composite in alkaline media. Prog. Chem. Biochem. Res. 1, 1–10. doi: 10.29088/SAMI/PCBR.2018.1.110
Mohammadpour, A., Ashkezari, M. D., Farahmand, B., and Shokrzadeh, M. (2019). Demographic characteristics and functional performance of the kidneys and hearts of patients with acute tramadol toxicity. Drug Res. 69, 207–210. doi: 10.1055/a-0646-3918
Nicholas, P., Pittson, R., and Hart, J. P. (2018). Development of a simple, low cost chronoamperometric assay for fructose based on a commercial graphite-nanoparticle modified screen-printed carbon electrode. Food Chem. 241, 122–126. doi: 10.1016/j.foodchem.2017.08.077
Prasad, P., and Sreedhar, N. Y. (2018). Effective SWCNTs/Nafion electrochemical sensor for detection of dicapthon pesticide in water and agricultural food samples. Chem. Methodol. 2, 277–290. doi: 10.22034/chemm.2018.63835
Rabiee, N., Safarkhani, M., and Rabiee, M. (2018). Ultra-sensitive electrochemical on-line determination of Clarithromycin based on Poly (L-Aspartic acid)/graphite oxide/pristine graphene/glassy carbon electrode. Asian J. Nanosci. Mater. 1, 63–73. doi: 10.26655/ajnanomat.2018.3.3
Renedo, O. D., Alonso-Lomillo, M. A., and Martinez, M. A. (2007). Recent developments in the field of screen-printed electrodes and their related applications. Talanta 73, 202–219. doi: 10.1016/j.talanta.2007.03.050
Rokhsefid, N., and Shishehbore, M. R. (2019). Synthesis and characterization of an Au nanoparticles/graphene nanosheet nanocomposite and its application for the simultaneous determination of tramadol and acetaminophen. Anal. Methods 11, 5150–5159. doi: 10.1039/C9AY01497G
Saccomanni, G., Del Carlo, S., Giorgi, M., Manera, C., Saba, A., and Macchia, M. (2010). Determination of tramadol and metabolites by HPLC-FL and HPLC–MS/MS in urine of dogs. J. Pharm. Biomed. Anal. 53, 194–199. doi: 10.1016/j.jpba.2010.03.016
Scott, L. J., and Perry, C. M. (2000). Tramadol. Drugs 60, 139–176. doi: 10.2165/00003495-200060010-00008
Shahid, M. M., Rameshkumar, P., Numan, A., Shahabuddin, S., Alizadeh, M., Khiew, P. S., et al. (2019). A cobalt oxide nanocubes interleaved reduced graphene oxide nanocomposite modified glassy carbon electrode for amperometric detection of serotonin. Mater. Sci. Eng. C 100, 388–395. doi: 10.1016/j.msec.2019.02.107
Shao, Y., Wang, J., Wu, H., Liu, J., Aksay, I. A., and Lin, Y. (2010). Graphene based electrochemical sensors and biosensors: a review. Electroanalysis 22, 1027–1036. doi: 10.1002/elan.200900571
Soltani, H., Beitollahi, H., Hatefi-Mehrjardi, A. H., Tajik, S., and Torkzadeh-Mahani, M. (2014). Voltammetric determination of glutathione using a modified single walled carbon nanotubes paste electrode. Anal. Bioanal. Electrochem. 6, 67–79.
Tan, C., Zhao, J., Sun, P., Zheng, W., and Cui, G. (2020). Gold nanoparticle decorated polypyrrole/graphene oxide nanosheets as a modified electrode for simultaneous determination of ascorbic acid, dopamine and uric acid. New J. Chem. 44, 4916–4926. doi: 10.1039/D0NJ00166J
Tangkuaram, T., Ponchio, C., Kangkasomboon, T., Katikawong, P., and Veerasai, W. (2007). Design and development of a highly stable hydrogen peroxide biosensor on screen printed carbon electrode based on horseradish peroxidase bound with gold nanoparticles in the matrix of chitosan. Biosens. Bioelectron. 22, 2071–2078. doi: 10.1016/j.bios.2006.09.011
Tetsunaga, T., Tetsunaga, T., Tanaka, M., and Ozaki, T. (2015). Efficacy of tramadol–acetaminophen tablets in low back pain patients with depression. J. Orthop. Sci. 20, 281–286. doi: 10.1007/s00776-014-0674-4
Thanh, T. D., Balamurugan, J., Lee, S. H., Kim, N. H., and Lee, J. H. (2016). Effective seed-assisted synthesis of gold nanoparticles anchored nitrogen-doped graphene for electrochemical detection of glucose and dopamine. Biosens. Bioelectron. 81, 259–267. doi: 10.1016/j.bios.2016.02.070
Thévenin, A., Beloeil, H., Blanie, A., Benhamou, D., and Mazoit, J. X. (2008). The limited efficacy of tramadol in postoperative patients: a study of ED80 using the continual reassessment method. Anesth. Analg. 106, 622–627. doi: 10.1213/ane.0b013e31816053aa
Vinodhkumar, G., Ramya, R., Vimalan, M., Potheher, I., and Cyrac Peter, A. (2018). Reduced graphene oxide based on simultaneous detection of neurotransmitters. Prog. Chem. Biochem. Res. 1, 40–49. doi: 10.29088/SAMI/PCBR.2018.1.4049
Xia, Y., Zhao, F., and Zeng, B. (2020). A molecularly imprinted copolymer based electrochemical sensor for the highly sensitive detection of L-Tryptophan. Talanta 206, 120245. doi: 10.1016/j.talanta.2019.120245
Yang, Y., Asiri, A. M., Du, D., and Lin, Y. (2014). Acetylcholinesterase biosensor based on a gold nanoparticle-polypyrrole-reduced graphene oxide nanocomposite modified electrode for the amperometric detection of organophosphorus pesticides. Analyst 139, 3055–3060. doi: 10.1039/c4an00068d
Yavuz, E., Tokalioglu, S., Sahan, H., and Patat, S. (2013a). A graphene/Co3O4 nanocomposite as a new adsorbent for solid phase extraction of Pb (II), Cu (II) and Fe (III) ions in various samples. Rsc Adv. 3, 24650–24657. doi: 10.1039/c3ra45111a
Yavuz, E., Tokalioglu, S., Sahan, H., and Patat, S. (2013b). Ultralayered Co3O4 as a new adsorbent for preconcentration of Pb (II) from water, food, sediment and tobacco samples. Talanta 115, 724–729. doi: 10.1016/j.talanta.2013.06.042
Zhang, Y., Sun, X., Zhu, L., Shen, H., and Jia, N. (2011). Electrochemical sensing based on graphene oxide/Prussian blue hybrid film modified electrode. Electrochim. Acta 56, 1239–1245. doi: 10.1016/j.electacta.2010.11.011
Zhang, Y., Zeng, G. M., Tang, L., Chen, J., Zhu, Y., He, X. X., et al. (2015). Electrochemical sensor based on electrodeposited graphene-Au modified electrode and nanoAu carrier amplified signal strategy for attomolar mercury detection. Anal. Chem. 87, 989–996. doi: 10.1021/ac503472p
Zheng, W., Wu, H., Jiang, Y., Xu, J., Li, X., Zhang, W., et al. (2018). A molecularly-imprinted-electrochemical-sensor modified with nano-carbon-dots with high sensitivity and selectivity for rapid determination of glucose. Anal. Biochem. 555, 42–49. doi: 10.1016/j.ab.2018.06.004
Zhou, Y. G., Chen, J. J., Wang, F. B., Sheng, Z. H., and Xia, X. H. (2010). A facile approach to the synthesis of highly electroactive Pt nanoparticles on graphene as an anode catalyst for direct methanol fuel cells. Chem. Commun. 46, 5951–5953. doi: 10.1039/c0cc00394h
Keywords: electrochemical sensor, tramadol, graphene/Co3O4 nanocomposite, screen printed electrode, nanomaterials
Citation: Aflatoonian MR, Tajik S, Aflatoonian B, Beitollahi H, Zhang K, Le QV, Cha JH, Jang HW, Shokouhimehr M and Peng W (2020) A Screen-Printed Electrode Modified With Graphene/Co3O4 Nanocomposite for Electrochemical Detection of Tramadol. Front. Chem. 8:562308. doi: 10.3389/fchem.2020.562308
Received: 15 May 2020; Accepted: 14 October 2020;
Published: 30 November 2020.
Edited by:
Yao He, Soochow University, ChinaCopyright © 2020 Aflatoonian, Tajik, Aflatoonian, Beitollahi, Zhang, Le, Cha, Jang, Shokouhimehr and Peng. This is an open-access article distributed under the terms of the Creative Commons Attribution License (CC BY). The use, distribution or reproduction in other forums is permitted, provided the original author(s) and the copyright owner(s) are credited and that the original publication in this journal is cited, in accordance with accepted academic practice. No use, distribution or reproduction is permitted which does not comply with these terms.
*Correspondence: Somayeh Tajik, dGFqaWtfczEzNjVAeWFob28uY29t; Quyet Van Le, bGV2YW5xdXlldEBkdHUuZWR1LnZu; Mohammadreza Shokouhimehr, bXJzaDJAc251LmFjLmty; Wanxi Peng, cGVuZ3dhbnhpQDE2My5jb20=