- 1Key Laboratory of Molecular Target and Clinical Pharmacology, State Key Laboratory of Respiratory Disease, School of Pharmaceutical Sciences & Fifth Affiliated Hospital, Guangzhou Medical University, Guangzhou, China
- 2Asia-Pacific Institute of Aging Studies, Lingnan University, Hong Kong, China
Traditional Chinese medicine (TCM) has been used to treat disorders in China for ~1,000 years. Growing evidence has shown that the active ingredients from TCM have antibacterial, antiproliferative, antioxidant, and apoptosis-inducing features. However, poor solubility and low bioavailability limit clinical application of active compounds from TCM. “Nanoformulations” (NFs) are novel and advanced drug-delivery systems. They show promise for improving the solubility and bioavailability of drugs. In particular, “smart responsive NFs” can respond to the special external and internal stimuli in targeted sites to release loaded drugs, which enables them to control the release of drug within target tissues. Recent studies have demonstrated that smart responsive NFs can achieve targeted release of active compounds from TCM at disease sites to increase their concentrations in diseased tissues and reduce the number of adverse effects. Here, we review “internal stimulus–responsive NFs” (based on pH and redox status) and “external stimulus–responsive NFs” (based on light and magnetic fields) and focus on their application for active compounds from TCM against tumors and infectious diseases, to further boost the development of TCM in modern medicine.
Introduction
Traditional Chinese medicine (TCM) as an important approach to treat disorders has been used widely in China for ~1,000 years. Accumulating evidence has shown that TCM exhibits excellent effects on tumors (Luo et al., 2019), bacteria (Kim et al., 2008; Moloney, 2016; Wang Z. et al., 2018b), and viruses (Li et al., 2018a; Yao et al., 2018; Yang et al., 2020) because of their active compounds. Some active compounds, such as artemisinin, curcumin, and epigallocatechin gallate, emodin, and celastrol, show prominent efficacy against tumors (Wang et al., 2014; Shanmugam et al., 2017). Flavonoids, polyphenols, alkaloids, and terpenoids are considered to be efficacious against antibiotic-resistant bacteria (Zhao et al., 2019b). Also, TCM shows multitarget features against viruses in direct or indirect ways (Ai et al., 2018; Li et al., 2018a).
However, the poor solubility and low bioavailability of the active compounds from TCM limit their clinical application. To address these shortcomings, novel drug-delivery nanoformulations (NFs) are being employed to increase solubility, improve the efficiency of targeted delivery, and reduce the number of adverse effects. Recently, liposomes, nanoparticles, vesicles, mesoporous silica nanoparticles, and micelles as potential drug-delivery NFs have shown great promise in TCM (Ma et al., 2019).
“Smart responsive NFs” are new types of targeted NFs that deliver drugs specifically to target tissues or organs via an “intelligent response” in target sites. Usually, smart responsive NFs are classified into “internal stimuli–responsive NFs” and “external stimuli–responsive NFs” according to different stimuli conditions as shown in Figure 1. Internal stimuli–responsive NFs are mainly from the microenvironment of the targeted site and include pH, enzymes, redox status, and receptors. External stimuli–responsive NFs are mainly from magnets, heat, light, or ultrasound.
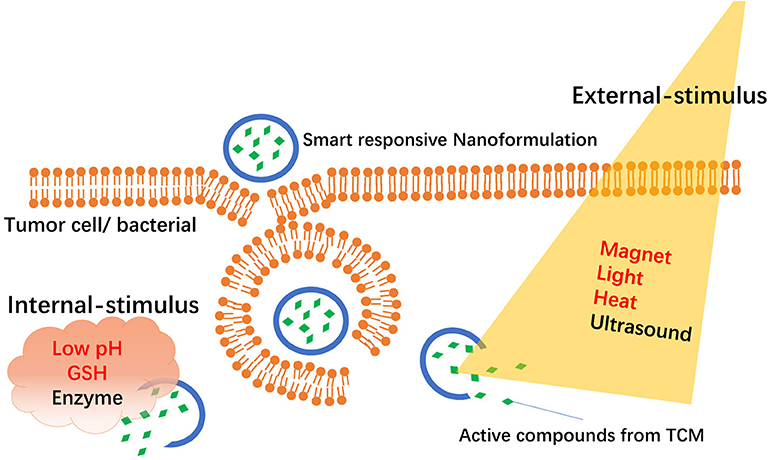
Figure 1. Schematic representation of smart responsive nanoformulations-mediated delivery of active compounds from TCM.
Here, we reviewed internal stimulus–responsive NFs and external stimulus–responsive NFs. We focused on the application of active compounds from TCM against tumors and infectious diseases, to further boost the development of TCM concepts in modern medicine.
Internal Stimulus–Responsive NFs
pH-Responsive NFs for Delivery of Active Compounds From TCM
The metabolism of tumor cells involves aerobic glycolysis to obtain energy for overgrowth and proliferation (Ganapathy-Kanniappan and Geschwind, 2013). Aerobic glycolysis is based on the conversion of glucose to pyruvate, which results in fermentation and lactate production (Shi et al., 2018), and the resulting acidosis is a ubiquitous characteristic of cancer (Tang et al., 2018). It has been demonstrated that the tumor tissues' acidic environment is about pH 6.5 (Som et al., 2016), whereas the pH of normal tissue is 7.4 (Liu et al., 2014). The microenvironment of tissues infected by bacteria is also acidic (Tao et al., 2019a). As shown in Table 1, based on the characteristics of an acidic microenvironment, pH-responsive NFs have been developed for targeted delivery of active compounds from TCM.
Delivery of Artemisinin and Dihydroartemisinin
Artemisinin and dihydroartemisinin are active agents from the Chinese herb Artemisia annua. They are used as antimalarial drugs in China (Tu, 2016). They are also potential anticancer agents when interacting with iron ions to produce reactive oxygen species (ROS), which can kill malignant cancer cells (Efferth, 2017). Chen et al. developed Fe3O4 nanoparticles as a pH-sensitive NF, for treating cervical cancer (Chen et al., 2014). In this NF, artemisinin was stored in the outer mesoporous shells. Fe2+ was liberated from the core of the Fe3O4 nanosphere in acidic organelles and cleaved the endoperoxide bridges of artemisinin to generate free radicals to kill HeLa cells. Artemisinin-hollow mesoporous silica-Fe3O4 nanoparticles have also been used to treat breast cancer via pH-responsive Fe3O4 nanoparticles (Bhaw-Luximon and Jhurry, 2017). Sustained release of artemisinin occurred in the lysosomal compartment (pH 3.8–5.0), and Fe3O4 was metabolized to free Fe2+ to generate many free radicals through reaction with the released artemisinin. In addition, an Fe3O4@MnSiO3-folate nanosphere was developed for the treatment of lung cancer (Chen et al., 2015). Folate was introduced to increase the enhanced permeability and retention (EPR) effect. Meanwhile, Mn2+ was released from the silicate shells and interacted with artemisinin in an acidic tumor environment and organelles of A549 cells.
Liu et al. (2015) prepared dihydroartemisinin–graphene oxide–transferrin nanoparticles. The Fe (III) conjugated on transferrin was released in lysosomes and was reduced to Fe (II) by ferric reductase. Fe (II) reacted with dihydroartemisinin on graphene oxide to yield ROS and oxygen/carbon-centered radicals, which then induced the death of EMT6 cells.
Delivery of Baicalein
Baicalein is a flavonoid from Scutellaria baicalensis Georgi. which has antioxidant, antivirus, antibacterial, anti-inflammatory, and antiallergic properties (Bie et al., 2017). Baicalein can inhibit the cell cycle; scavenge oxygen radicals; attenuate the activity of mitogen-activated protein kinase, protein kinase B, or mammalian target of rapamycin signaling pathways; and induce apoptosis by activating caspase-9/-3 (Liu et al., 2016). Recently, baicalein was delivered by a pH-responsive cleaved hydrazone bond in transferrin decorated docetaxel baicalein co-loaded solid lipid nanoparticles (Li et al., 2017). A modification based on polyethylene glycol (PEG) provided this system a long circulation in the body. Also, transferrin could guide delivery of nanoparticles into tumor cells by transferrin receptors. At an acidic condition, the hydrazone bond ruptured, resulting in shedding of the PEG layer from nanoparticles and the targeted release of baicalein.
Delivery of Cinnamaldehyde
Cinnamon is used not only as a spice, but also in TCM. Cinnamaldehyde is an active compound in cinnamon that can induce the apoptosis of tumor cells (Sadeghi et al., 2019). Yoo et al. (2018) used acetal linkages to couple cinnamaldehyde and maltodextrin to fabricate a cinnamaldehyde-maltodextrin nanoparticle. The acetal linkage dissociated in the acidic environment, and cinnamaldehyde and maltodextrin could return to their native states. Zhao et al. (2019a) also used acetal linkages to couple cinnamaldehyde and dextran in 10-hydroxy camptothecin-cinnamaldehyde nanoparticles. The acetal linkage of cinnamaldehyde was hydrolyzed from dextran at an acidic pH, which broke the nanoparticles into water-soluble fragments, and resulted in rapid dissociation. Ferrocene-loaded poly[(3-phenylprop-2-ene-1,1-diyl)bis(oxy)bis(ethane-2,1-diyl)diacrylate]-co-4,4′(trimethylene dipiperidine)-copoly(ethylene glycol) micelles have been designed to release ferrocene and cinnamaldehyde rapidly at the site of bacterial infections (which are characterized by a low pH) (Park et al., 2016). Cinnamaldehyde induced the generation of hydrogen peroxide (H2O2) and iron in ferrocene and then converted H2O2 into highly toxic hydroxyl radicals.
Delivery of Curcumin
Curcumin is a polyphenol extracted from the herb turmeric. It has gained attention worldwide because of its antioxidant, anti-inflammatory, antimicrobial, and antiviral activities (Giordano, 2019). NF development can circumvent the poor bioavailability of curcumin to improve treatment outcome (Adiwidjaja and McLachlan, 2017).
Poly(β-amino ester) (PAE) is a typical pH-responsive biodegradable polymer. PAE has outstanding characteristics, such as positive charges, readily degradable linkages, high biodegradability, and high biocompatibility (Cordeiro et al., 2019). Yu et al. developed poly(ethylene glycol)-poly(lactide)-PAE micelles to deliver curcumin to the breast cancer cell line MCF-7. PAE is insoluble at pH 7.4 and maintains a large micelle structure with a hydrophobic PLA/PAE core (Yu and Zhang, 2014). However, PAE is soluble at pH 6.8 (thanks to protonation of tertiary amino groups) and shrinks the micelle structure to a hydrophobic PLA core. D-α-Tocopheryl PEG 1000-block-PAE (TPGSPAE) nanoparticles used in treatment of hepatocellular carcinoma have a similar mechanism of action (Zhang et al., 2017). The tertiary diamine moieties of the PAE core are protonated at low pH so that the hydrophobic curcumin is released from the hydrophilic core-shell structure. In curcumin-pluronic P123-PAE (Cai et al., 2016), P123 is constituted by poly(ethylene oxide)-poly(phenylene oxide)-poly(ethylene oxide) (PEO-PPO-PEO). The PPO/PAE core offers a local hydrophobic microenvironment for curcumin loading, and the hydrophilic PEO shell maintains a large micelle structure at pH 7.4. PAE is protonated and dissolves at weakly acidic pH, making the nanocarrier shrink to be a small micelle structure and release curcumin.
pH-sensitive linkages have also been used for curcumin delivery. At pH 4.5, chains of β-acrylic acid undergo transition to the protonated state. This action lowers their solubility abruptly and isolates them from the aqueous phase and results in rearrangements of the bilayer membrane and curcumin leakage. Fang et al. (2016) coupled a poloxamer (F68) and curcumin by a cis-aconitic anhydride linker. In acidic environments, the pH-sensitive cis-aconitic anhydride linker of F68-cis- curcumin conjugates were cleaved to release curcumin. Chen et al. (2016a) reported a menthone 1,2-glycerol ketal (MGK), which was used in Cur-hyaluronan-histidine-MGK. The ketal moieties of MGK could be degraded in a tumor microenvironment. Also, the imidazole group of histidine, which linked oligomeric hyaluronic acid (oHA) and MGK, could destroy lysosomal membranes to prevent the drug being degraded in cells. Luan et al. found that an acidic condition (pH < 6.5) could cause breakage of internal linkages in aliphatic amines grafted konjac glucomannan (KGM-g-AH) between a primary amine of octylamine and an aldehyde group and release curcumin. The responsive agent in KGM-g-AH8 micelles was KGM (Luan et al., 2017). Similarly, pH-sensitive hydrazone bonds have been used to deliver curcumin. Polymer oHA-hydrazone bond-folic acid biotin nanomicelles consisting of folic acid, biotin, and cluster of differentiation (CD)44 receptors can mediate the targeting of tumor tissue and cancer stem cells, from which the icariin and curcumin are released into the tumor microenvironment, depending on the pH-sensitive hydrazone bond (Liu et al., 2019).
Eucalyptus from Eucalyptus robusta Smith has been developed as a transdermal delivery vehicle for curcumin (Liu and Chang, 2011). Sahu et al. reported a 5-fluorouracil double-walled nanogel (FDWNL) to load eucalyptus with pH-sensitive chitosan. The hydrophilic pendant groups of chitosan (-OH and -NHCOCH3) and strong ionic attraction between the ionized nanogel and hydrated counter ions caused rapid swelling of the FDWNL to release curcumin at acidic pH (Sahu et al., 2017).
Among metal nanoparticles, ZnO nanoparticles are pH-sensitive nanoplatforms used for curcumin delivery. The nontoxic ZnO nanoparticles are stable at pH ~7, but dissolve and open up the polymer linkages of polymethyl methacrylate-acrylic acid ZnO nanoparticles to release curcumin together with toxic Zn2+ at a low pH (~5.4) (Dhivya et al., 2018). Curcumin was also connected to one side of the disulfide-condensed menthone 1,2 glycerol ketal as a redox response prodrug material. The other side of menthone 1,2 glycerol ketal was connected to hyaluronic acid with an ester bond that made pH/redox response. The pH-responsive calcium phosphate shell made this NF broken at pH 5.5 to release curcumin to kill cancer (Chen et al., 2017). Wu et al. also used calcium phosphate as a pH-responsive shell to wrap the curcumin-adsorbed sodium caseinate micelles to improve the stability of curcumin. In the study, Wu et al. found that the calcium phosphate shell covering the sodium caseinate micelles was broken in the acidic stroma outside the tumor cells (Wu et al., 2020).
Delivery of Glycyrrhetinic Acid
Glycyrrhetinic acid (GA) is extracted from licorice and is modified for targeting of liver cells (Zhu et al., 2019, 2020; Li et al., 2020). In doxorubicin-loaded chitosan/poly(ethylene glycol)-GA nanoparticles, GA increases the affinity for liver cancer cells (Tian et al., 2010). Moreover, protonation of the amino groups of chitosan at an acidic condition and the acid-soluble doxorubicin hydrochloride cause the swelling of nanoparticles and release doxorubicin. In GA-modified chitosan-polyethyleneimine-4-hydrazinobenzoic acid–doxorubicin nanoparticles, GA improves the liver-targeting ability, and the hydrazone bond between doxorubicin and GA-CS-PEI-HBA is broken to release the “payload” in the intracellular environment (pH 4.5–6.5) of tumor cells (Yan et al., 2018).
Delivery of Triptolide and Celastrol
Triptolide and celastrol are contained in Tripterygium wilfordii. Triptolide has excellent inhibitory effects in the treatment of pancreatic cancer (Kim et al., 2018). Kong et al. (2019) enhanced the efficacy of triptolide by developing triptolide prodrug-loaded ultra–pH-sensitive micelles (T-UPSMs). After internalization by the endocytic organelles of cancer cells, the pH reached the apparent acid dissociation constant of T-UPSMs due to lysosomal acidification. The tertiary amine groups of T-UPSMs absorbed protons and induced micelle dissociation to elicit immediate drug release as a pH buffer in endosomes/lysosomes. Ding et al. (2017) found that triptolide or celastrol was weakly adsorbed or bonded to silk fibroin nanoparticles. At a low pH, silk loses its overall acidic surface properties and negative net charge, and the balance of the negative charge is shielded at an acidic pH, which destroys aggregates. Ling et al. (2014) developed nanoformulated triptolide nanoparticles for treatment of hepatocellular carcinoma. Internally packed imidazole was employed for pH-sensitive ionization and dispersion. During endosomal maturation, the decrease in pH could lead to the collapse of nanoparticles and triptolide release.
Delivery of Ursolic Acid
Ursolic acid (UA) is present in Prunella vulgaris L. but is also abundant in most fruits and vegetables (Yin et al., 2016). UA use has been reported in treatment of cancer (Jaman, 2018; Manayi et al., 2018; Mlala et al., 2019) and some infections (Tohmé et al., 2019). UA has been encapsulated in nanoparticles or liposomes and found to inhibit progression of cervical cancer (Wang M. et al., 2017; Wang S. et al., 2017). Jiang et al. (2017) prepared UA-loaded mesoporous silica nanoparticles and folic acid conjugated chitosan (UA@M-CS-FA) nanoparticles in which the acid-labile amide bond between CS-FA and mesoporous silica nanoparticles was broken in acidic conditions. This action made the chitosan chains swell and opened the mesopores of UA@M-CS-FA nanoparticles, resulting in UA release.
Delivery of Berberine
Berberine is isolated from Coptis chinensis and has antibacterial effects. Berberine was shown to markedly increase the survival rate of mice challenged with the bacterial endotoxin lipopolysaccharide (2 EU/mL) (Chu et al., 2014). Chitosan and heparin are ionized and form polyelectrolyte complexes via electrostatic interactions at pH 1.2–6.0 to protect berberine from destruction by gastric acids. At pH 7.0, chitosan is deprotonated, and berberine-loaded fucose-conjugated nanoparticles are broken apart to release berberine (Lin et al., 2015). Moreover, berberine as a hydrophobic part was linked to chitosan oligosaccharides with a dithiodipropionic acid linker, and 3,4-dihydroxyphenylpropionic acid as a hydrophilic part was also linked to another end of the chitosan oligosaccharides to form a polymer monomer. The positively charged 3,4-dihydroxyphenylpropionic acid attracted the negatively charged hyaluronic acid to form an oHA-3-carboxyphenylboronic acid shell and encapsulate curcumin. In the acidic microenvironment of tumor, the exposed hyaluronic acid specifically binds to the CD44 receptor, and the micelles are endocytosed by the cells and undergo charge reversal. The high concentration of glutathione (GSH) in tumor cells hydrolyzes the disulfide bonds of dithiodipropionic acid; the micelles collapse and release berberine and curcumin (Fang et al., 2019b).
Delivery of Physcion
Usually, zeolitic imidazolate framework-8 (ZIF-8) is fabricated using zinc ions and 2-methylimidazolate. ZIF-8 has high porosity, good structural regularity and tunability, adjustable surface functionality, and intrinsic pH-induced biodegradability (Gao et al., 2019b). Soomro et al. (2019) developed physcion@ZIF-8 to deliver the physcion present in rhubarb. In acidic conditions, the coordination linkage between zinc and imidazolate was broken, whereas the imidazolate was protonated, resulting in physcion release from ZIF-8.
Delivery of Quercetin
Quercetin from Hypericum ascyron L. has been reported to be beneficial for cardiovascular disease (Patel et al., 2018). In recent years, the nanotechnology transformation of quercetin has revealed that quercetin has considerable effects against tumors (Vinayak, 2019). Bertleff-Zieschang et al. reported quercetin/Fe (III) nanoparticles as potential antibacterial NFs. In that work, formation of a quercetin/Fe (III) network was pH-dependent based on coordination between a flavonoid ligand and Fe (III). At an acidic pH, quercetin/Fe (III) capsules were disassembled readily (Bertleff-Zieschang et al., 2017). Furthermore, amphiphilic pH-responsive 8-arm- and 12-arm-dendritic polyacrylic acid block copolymers were synthesized for quercetin loading. In vitro release experiments showed that both of these new materials achieved complete release faster at pH 5.0 than at pH 7.4. But the 12-arm dendritic polyacrylic acid polymer blocks copolymer release quercetin faster than the 8-arm-one (Sedghi et al., 2013). In a self-assembled micelle of N-acetyl-histidine-phosphocholine-chitosan conjugate, histidine was a pH-responsive molecule because of its imidazole group. At pH ≤ 6.0, the micelles swelled and ruptured to release the encaspsulated quercetin due to the protonation of the imidazole group (Wu et al., 2016).
Redox-Responsive NFs
GSH depletion promotes cancer cell death through processes such as apoptosis, necroptosis, autophagy, and ferroptosis (Lv et al., 2019). GSH deficiency, or a decrease in the ratio of GSH and glutathione disulfide (GSSG), leads to an increased susceptibility to oxidative stress implicated in the progression of cancer cells (Traverso et al., 2013). The GSH:GSSG ratio, as the major pool of thiol groups, is a key factor in the antioxidative capacity of cells. GSH is an ideal and omnipresent internal stimulus for rapid degradation of disulfide linkages (Zhang et al., 2018b). As shown in Table 2, there are many applications of disulfide bonds in redox-responsive delivery of TCM agents.
Wang B et al. (2019) designed quercetin–dithiodipropionic acid–oHA–mannose–ferulic acid “nano-dandelions” for synchronous delivery of curcumin and baicalein. This was achieved by using the nano-dandelions as reduction-sensitive amphiphilic carriers. The coated oHA targeted CD44 receptors and could facilitate uptake of nano-dandelions in tumor locations. Mannose-targeting CD206 receptors could be engulfed readily by tumor-associated macrophages. The S-S linkage in 3,30-dithiodipropionic acid connecting the hydrophobic and hydrophilic parts could be broken by the high concentration of GSH within tumor cells, which facilitated release of curcumin and baicalein.
Wang K. et al. (2018) developed distearoyl phosphatidyl ethanolamine derivatized PEG-modified nano-echinus materials. Dithiodipropionic acid–curcumin (S-S-Cur) was chemically conjugated onto the side chain of the conjugated GA-oHA (GA-HA) to generate an amphiphilic polymeric prodrug of curcumin (GA-HA-S-S-Cur). HA targeted CD44 receptors on the surface of tumor cells, and the breaking of disulfide bonds by a high concentration of GSH in a tumor environment led to disassembly of nano-echinus materials, and then released curcumin. Tian et al. (2018) developed HA-S-S-CUR micelles for glioma treatment, in which the disulfide bond of cystamine linked HA (hydrophilic group) and curcumin (hydrophobic group). Reductive cleavage of the disulfide bond by GSH in glioma cells caused instability of the hydrophobic core, resulting in micelle degradation and curcumin release.
In alendronate-oHA-S-S-curcumin micelles (Dong et al., 2018), a disulfide bond combines hydrophobic curcumin with hydrophilic oHA and alendronate to form micelles in water. These micelles fracture under a reducing environment to release curcumin. Cleavage of the disulfide bonds of PEGylated prodrug nanomicelles (Zhang et al., 2019) can transfer these nanomicelles into hydrophilic curcumin-mercapto. This action accelerates hydrolysis of the adjoining ester bond and releases curcumin from PEGylated prodrug nanomicelles.
Recently, synthetically designed polymers containing disulfide bonds have been used to deliver TCM-active compounds. Homoharringtonine (HHT)–loaded poly(lactic-co-glycolic acid)-SS-PEG (Zhang et al., 2020a) is an epidermal growth factor receptor (EGFR) aptamer-modified PLGA targeted to the EGFR (which shows high expression in lung cancer cells). The drug is delivered into the cytoplasm via receptor-mediated endocytosis. The disulfide bonds of PLGA are broken by GSH in lung cancer cells.
Additionally, ester bonds have also been used in the development of redox-responsive NFs. In the podophyllotoxin (POD) delivery, PODPEG nanoparticles (Ou et al., 2019) are formed by PEG and POD with oxalate ester bond bridges. The latter are cleaved in the presence of H2O2 to release the drug.
External Stimulus–Responsive NFs
External stimuli–responsive NFs for delivery of active compounds from TCM are mainly including magnetic, light, and thermal response as shown in Table 3.
Magnetic-Responsive NFs for Delivery of Active Compounds From TCM
Magnetic-responsive NFs can be used to monitor tumors in vivo by noninvasive magnetic resonance imaging (MRI) (Kang et al., 2017). In this way, they can deliver chemotherapy drugs, small-molecule agents, photosensitizers, and small interfering RNA molecules (Zhu et al., 2017). Iron oxide nanoparticles are essential in magnetic-responsive NFs (Vangijzegem et al., 2019).
Gharib et al. created artemisinin- and transferrin-loaded magnetic nanoliposomes coated with iron oxide. The external magnet achieved high concentrations of artemisinin and transferrin in tumors to enhance the antitumor effect of artemisinin in BALB/c mice (Gharib et al., 2015). HHT- Fe3O4 magnetic nanoparticles were designed to deliver the HHT to tumor sites. HHT inhibited the synthesis of the short-lived protein Mcl-1 by targeting the A-site cleft of eukaryotic ribosomes (Chen et al., 2016b).
Dihydroartemisinin-MLP nanoliposomes were created using Fe3O4 nanoparticles. The negative charge of dihydroartemisinin-MLPs nanoliposomes under the action of a magnetic field delivered dihydroartemisinin with nonspecific binding to plasma proteins (Li et al., 2019a). Tetrandrine–Fe3O4-PLGA nanoparticles were used to direct tetrandrine to specific sites upon manipulation by an external magnetic field to increase the tetrandrine concentration. Tetrandrine is derived from Stephania tetrandra and is an active compound of a TCM called “Fangji” (Wang K et al., 2019).
In the design of magnetic-responsive nanoliposomes to deliver quercetin, quercetin was not only loaded as an antitumor flavonoid drug and also has strong antioxidant properties to prevent the nanomagnetic liposomes from being oxidized. However, the incorporation of quercetin might weaken the magnetic properties of the nanoliposomes, and it was necessary to precisely control the ratio of quercetin to ensure that the superparamagnetism of the magnetic liposomes was maintained (Cruz Dos Santos et al., 2019).
Light-Responsive NFs for Delivery of Active Compounds From TCM
NFs can be activated by light (visible, ultraviolet, infrared) for using in photodynamic imaging, photodynamic therapy (PDT) or photothermal therapy (PTT) (Rkein, 2014; Chen and Zhao, 2018). Light-responsive agents (“photosensitizers”) play an important part in such light-responsive NFs (Reeßing and Szymanski, 2017).
Traditional photosensitizers and TCM agents can be co-encapsulated, or a TCM agent can be encapsulated in light-responsive inorganic/organic nanoparticles. Moreover, some active compounds of TCM can be used as photosensitizers to carry out PDT or PTT after light activation.
An artesunate-modified PEGylated nanographene oxide (nGO-PEG-ARS) (Pang et al., 2017) delivered artesunate to liver cancer with the light-responsive agent. The light responsive agent was a covalent bond between carboxylic groups and amino groups of nGO-PEG. Near-infrared irradiation triggered the artesunate loaded on nGO-PEG to produce nitric oxide and peroxynitrite groups to achieve a synergistic chemophotothermal anticancer effect.
In Fe–gallic acid–PEG coordination polymer-based nanoparticles, ultrasmall (5 nm) Fe–gallic acid nanoparticles were formed by mixing FeCl3 solution and gallic acid solution (Jin et al., 2017). Surface modification of PEG increased its passive accumulation in tumor cells. Also, the temperature was increased by 20°C after laser irradiation for 5 min (808-nm laser at 0.8 W cm2).
In the treatment of gastric cancer, Li et al. (2016) used aloe emodin to fabricate aloe emodin nanoliposomes for transfection of the r-caspase-3 gene and PDT. Moreover, hypericin is an excellent photosensitizer in hypericin–low-density lipoprotein–dextran complexes for glioma treatment. In these complexes, dextran is modified on the surface of low-density lipoprotein particles, which reduces the interaction of low-density lipoprotein with other serum constituents to prevent hypericin redistribution to other free lipoproteins (Huntosova et al., 2012).
Also, single-walled carbon nanohorn-hypericin has been shown to improve the water solubility, photostability, and therapeutic effects of hypericin and to protect it from light degradation. Single-walled carbon nanohorn-hypericin can simultaneously generate sufficient ROS and hyperthermia upon light irradiation at 590 and 808 nm (Gao et al., 2019a).
Thermal-Responsive NFs for Delivery of Active Compounds From TCM
In thermal-responsive chitosan-g-poly(N-isopropylacrylamide) co-polymeric nanoparticles, the polymer–polymer interaction of poly(N-isopropylacrylamide) is greater than the polymer-curcumin interaction, whereas the hydrogen bond is weakened at a lower critical solution temperature, so curcumin molecules can escape from the entrapped polymer matrices (Rejinold et al., 2011). Honokiol hydrogel (Fang et al., 2009) loads honokiol as a free-flowing sol at room temperature or below the critical gelation temperature. Honokiol can become a gel at body temperature and remain in situ for a long time to reduce the burst release of honokiol.
Multiple-Responsive NFs for Delivery of Active Compounds From TCM
Multiple-responsive NFs usually have two or more different responsive functions and modes. The combination of multiple responsive modes can overcome the shortcomings of a single responsive mode to achieve targeted delivery of drugs. The main multiple-responsive NFs are shown in Table 4.
Dual Internal–Responsive NFs
For targeted delivery of cinnamaldehyde, quinone methide-cinnamaldehyde (Noh et al., 2015) was prepared as a dual-responsive NF combining a redox response and pH response. The H2O2-sensitive boronate linkage and acid-sensitive acetal linkage were oxidized rapidly to release quinone methide to abrogate GSH. Also, the acetal linkage degraded in acidic tumor environments to release ROS-generating cinnamaldehyde, which killed DU145 and SW620 cells efficiently in vitro and in vivo. The same type of response mode was used in tumor-specific enhanced oxidative stress polymer conjugate (Ma et al., 2020) delivery of cinnamaldehyde on CT26 and 4T1 cells. The acidic condition accelerated hydrolysis of acetal linkages in phenylboronic acid containing cinnamaldehyde derivatives, and H2O2 facilitated detachment of boric acid groups and intramolecular rearrangement to produce quinone methide and further accelerated the hydrolysis of acetal linkages and cinnamaldehyde release.
Celastrol was delivered to desmoplastic melanoma cells by aminoethylanisamide–polymer–disulfide bond nanoparticles (Liu et al., 2018). In an acidic tumor microenvironment, tertiary amines of mitoxantrone and celastrol copolymer were protonated and reversed the zeta potential from a negative charge to a positive charge, thereby attracting negatively charged cell membranes. Also, the disulfide bond was broken in the presence of GSH, resulting in an increased particle size.
Disulfide bonds and β-thiopropionate linkages endowed a redox response and pH response of T7-peptide-S-S-nanoparticles (Li et al., 2019b) for POD delivery. Disulfide bonds were disassociated by a high concentration of GSH, and the β-thiopropionate linkage was broken by an acidic environment, and then POD was released rapidly. Massaro et al. replaced the keto group of curcumin with a pH-responsive imine group and used a carbon chain with a disulfide bond to connect the silicon on halloysite nanotubes to prepare a pH/redox responsive curcumin prodrug. In the different pH and 10 mM GSH environment, it showed a good release behavior of curcumin (Massaro et al., 2016).
External/Internal–Responsive NFs
Multiple-responsive NFs with both external and internal responses are conducive to targeted release of TCM agents at lesion sites. As stated above, often magnetically responsive delivery is combined with other responsive modes for higher efficiency of drug delivery.
A multiple-responsive NF called Prussian blue-iron carboxylate dual-metal organic frameworks [PB@MIL-100(Fe)dual-MOFs] can deliver artemisinin to HeLa cells through integrated magnetic, light and pH responses (Wang et al., 2016b). The inner Prussian blue MOFs and outer MIL-100(Fe) MOFs serve as MRI contrast agents. Under the illumination of an 808-nm near-infrared laser, the inner Prussian blue MOF induces hyperthermia by converting light energy to heat. The MIL-100(Fe) MOF can collapse in acidic environments to release artemisinin.
Dihydroartemisinin was delivered using a strategy combining a magnetic response and pH response. Fe3O4@C@MIL-100(Fe) nanoparticles were designed by Wang and colleagues (Wang et al., 2016a). Fe3O4@C nanoparticles were magnetic targeting, and carbon dots were two-photon fluorescence imaging agents. MIL-100(Fe) MOFs degraded and released dihydroartemisinin and Fe (III) ions in acidic conditions. When the pH within an endosome decreased, Fe3+ reduced to Fe2+ by ferric reductase and other reductive molecules within cells.
Magnetic field–induced endocytosis and pH-responsive drug release of berberine-loaded Fe3O4-mSiO2 nanoparticles were exhibited in hepatocellular-carcinoma treatment because the pH-responsive carboxylate functional group was modified on the surface and pores of silica rods and the magnetic-responsive Fe3O4 head (Wang Z. et al., 2017). In stevioside (STE)-MNP, STE reduced the size of Fe3O4 nanoparticles to control magnetic properties and aligned rapidly with the external magnetic field (Gupta and Sharma, 2019). The carbohydrate nature of STE enhanced the interaction between STE-MNP and C6 cells. STE-MNP aggregated as cluster-like formations in the glioma site to enhance hyperthermia.
The magnetic-responsive agents, superparamagnetic iron oxide nanoparticles (SPIONs), and a light-responsive agent, indocyanine green (ICG), were combined in SPIONs-GA-ICG (Ghorbani et al., 2018). The SPIONs produced hyperthermia; gallic acid was oxidized and bonded to ICG by free-radical production, and gallic acid also acted as a biological coating for ICG-loaded SPIONs.
A thermal- and pH-responsive NF was used as a cinnamaldehyde-delivery platform with glycine and a pluronic polymer. Cinnamaldehyde tagged Fe3O4 nanoparticles capped with glycine and pluronic polymer nanoparticles increased the temperature to 41.6°C within 1 min after radiofrequency pulses of 20 MHz (Wani et al., 2014). Also, the ionic interaction between the free aldehyde group of cinnamaldehyde and the liberated protons under acidic conditions delivered cinnamaldehyde rapidly from the nanoparticles.
Summary
Most of the active compounds extracted from TCM are small molecules. They have unfavorable pharmacokinetics and suboptimal biodistribution (e.g., prominent accumulation in multiple healthy organs) (Golombek et al., 2018). Through nanotechnology modification, drug molecules have a better EPR effect to increase the accumulation of drugs at lesion sites (Maeda, 2017). Thus, several types of nanodelivery system have been employed to improve the clinical outcome of the active compounds in TCM formulations: nanoparticles, liposomes, micelles, nanocapsules, and nanoemulsions (Ma et al., 2019).
Stimuli-responsive NFs can release drugs under internal and external stimuli. We reviewed the applications of stimuli-responsive NFs used commonly in the delivery of active compounds from TCM.
Four types of pH-responsive agents can be classified. The first type is inorganic material. Iron-containing nanoparticles play a key part in the pH-responsive delivery of artemisinin. The endoperoxide linkages (R-O-O-R′) in artemisinin can be cleaved with intracellular Fe2+ to generate toxic radicals to kill cancer cells and treat malaria (Asano and Iwahashi, 2017; Ding et al., 2018). Fe3O4 dissociates rapidly at low pH in lysosomes and transforms to Fe2+ (Bhaw-Luximon and Jhurry, 2017). Simultaneously, Fe3O4 is also a magnetic agent that can induce a magnetic response. In Fe3O4@MnSiO3-FA nanospheres, Mn2+ has the same function as Fe2+ (Chen et al., 2015). ZIF-8 is a well-known pH-sensitive imidazole derivative (Chen et al., 2018; Gao et al., 2019b) composed of Zn2+ coordinated by four imidazolate rings (Mateo et al., 2019). ZnO nanoparticles are also degraded readily to Zn2+ (Hahn and Ahmad, 2012). ZnO is stable at pH ~7, but dissolves rapidly at pH < 6 (Dhivya et al., 2018). The second type of pH-responsive material is a natural product with pH instability. Chitosan is a commonly used drug-delivery agent with special amphiphilic characteristics that enables “packaging” of a poorly soluble drug in water phases (Younes, 2015). Its pH-responsive function is attributed mainly to protonation of amino groups in acidic conditions, which make chitosan hydrogels swell rapidly and release the loaded drug. The third type of pH-responsive materials is artificial polymers such as PAE, PAGA, and PLGA. The fourth (and most common) types of pH-responsive material used in the delivery of active compounds from TCM are amine bonds and hydrazone bonds. They are linked ingeniously with the active agent from the TCM and the matrix of NFs until they encounter an acidic microenvironment. This strategy protects the drug from leaking prematurely in the circulation and promotes the targeted release of the drug at the lesion site.
Redox-responsive NFs of active compounds from TCM are dependent mainly on modification of disulfide bonds. Metabolic imbalances in tumor cells produce high levels of GSH, which can cleave disulfide bonds to release the loaded agent in target cells. In addition, pathogen infection induces an inflammatory reaction to increase H2O2 levels in infected tissues, which are prone to redox reactions.
In light-responsive NFs, besides the many chemical photosensitizers, several naturally active ingredients of TCM are used as photosensitizers in PDT, including curcumin (Tao et al., 2019b), hypericin (Lin et al., 2016; Li et al., 2018b; Zhang et al., 2018a), and aloe emodin. These active compounds from TCM have been shown to be useful in PDT or PTT in multiple studies.
Some active substances from TCM have been used for nanotargeted delivery. In doxorubicin-loaded CTS/PEG-GA nanoparticles (Tian et al., 2010), GA can target GA receptors on liver cancer cells. Also, GA receptors have been shown to be useful as liver-cancer targets in GA NFs (Sun et al., 2017). Similarly, in quercetin/Fe (III) (Bertleff-Zieschang et al., 2017) delivery, quercetin (as a phenolic compound) can form a complex organic–inorganic network with iron and gallic acid, and STE contributes to regulation of iron ions to form an “ideal” size of magnetic nanoparticles. Some active compounds from TCM contain both water-soluble sugar groups and water-insoluble aglycones, which form biocompatible NFs readily by self-assembly. Also, most active compounds from TCM have multiple active binding sites in biological tissues.
In stimuli-responsive NFs, internal stimulus–responsive NFs have great advantages in specifically releasing active compounds from TCM to the target site, subsequently increasing their therapeutic efficacy and decreasing the side effects. However, it is very difficult to artificially control the amounts and time of drug release from internal stimulus–responsive NFs. External stimulus–responsive NFs have advantageous properties in artificial control of drug release because they could respond to external light and magnetic energy. Therefore, we could regulate light and magnetic energy to control the release amounts and time of drugs from these NFs.
The information provided above indicates that smart responsive NFs hold great promise in targeted delivery of active compounds from TCM. However, the confirmed evidences are mainly from in vitro and in vivo experimental studies. Few clinical trials are investigated on smart responsive NFs as the targeted carriers for delivering active compounds from TCM. The main challenges are the complexity of smart responsive NFs such as tedious preparation, complicated characterization, biosafety of nanoparticle materials, and uncertainty of the in vivo fate of NFs. Thus, further exploring and addressing the above problem should be urgent tasks for translating smart responsive NFs as targeted delivery carriers of active compounds from TCM to clinical application.
Author Contributions
All authors listed have made a substantial, direct and intellectual contribution to the work, and approved it for publication.
Conflict of Interest
The authors declare that the research was conducted in the absence of any commercial or financial relationships that could be construed as a potential conflict of interest.
References
Adiwidjaja, J., and McLachlan, A. J. (2017). Curcumin as a clinically-promising anti-cancer agent: pharmacokinetics and drug interactions. Exp. Opin. Drug Metab. Toxicol. 13, 953–972. doi: 10.1080/17425255.2017.1360279
Ai, H., Wu, X., Qi, M., Zhang, L., Hu, H., Zhao, Q., et al. (2018). Study on the mechanisms of active compounds in traditional chinese medicine for the treatment of influenza virus by virtual screening. Interdiscip. Sci. 10, 320–328. doi: 10.1007/s12539-018-0289-0
Asano, M., and Iwahashi, H. (2017). Determination of the structures of radicals formed in the reaction of antimalarial drug artemisinin with ferrous ions. Eur. J. Med. Chem. 127, 740–747. doi: 10.1016/j.ejmech.2016.10.053
Bertleff-Zieschang, N., Rahim, M. A., Ju, Y., Braunger, J. A., Suma, T., Dai, Y., et al. (2017). Biofunctional metal-phenolic films from dietary flavonoids. Chem. Commun. 53, 1068–1071. doi: 10.1039/C6CC08607A
Bhaw-Luximon, A., and Jhurry, D. (2017). Artemisinin and its derivatives in cancer therapy: status of progress, mechanism of action, and future perspectives. Cancer Chemother. Pharmacol. 79, 451–466. doi: 10.1007/s00280-017-3251-7
Bie, B., Sun, J., Guo, Y., Li, J., Jiang, W., Huang, C., et al. (2017). Baicalein: a review of its anti-cancer effects and mechanisms in hepatocellular carcinoma. Biomed. Pharmacother. 93, 1285–1291. doi: 10.1016/j.biopha.2017.07.068
Cai, X., Liu, M., Zhang, C., Sun, D., and Zhai, G. (2016). pH-responsive copolymers based on pluronic P123-poly(β-amino ester): synthesis, characterization and application of copolymer micelles. Colloids Surf. B Biointerfaces 142, 114–122. doi: 10.1016/j.colsurfb.2016.02.033
Chen, D., Dong, X., Qi, M., Song, X., and Sun, J. (2017). Dual pH/redox responsive and CD44 receptor targeting hybrid nano-chrysalis based on new oligosaccharides of hyaluronan conjugates. Carbohydr. Polym. 157, 1272–1280. doi: 10.1016/j.carbpol.2016.10.089
Chen, D., Lian, S., Sun, J., Liu, Z., Zhao, F., Jiang, Y., et al. (2016a). Design of novel multifunctional targeting nano-carrier drug delivery system based on CD44 receptor and tumor microenvironment pH condition. Drug Deliv. 23, 808–813. doi: 10.3109/10717544.2014.917130
Chen, H., and Zhao, Y. (2018). Applications of light-responsive systems for cancer theranostics. ACS Appl. Mater. Interfaces 10, 21021–21034. doi: 10.1021/acsami.8b01114
Chen, J., Guo, Z., Wang, H. B., Zhou, J. J., Zhang, W. J., and Chen, Q. W. (2014). Multifunctional mesoporous nanoparticles as pH-responsive Fe (2+) reservoirs and artemisinin vehicles for synergistic inhibition of tumor growth. Biomaterials 35, 6498–6507. doi: 10.1016/j.biomaterials.2014.04.028
Chen, J., Zhang, W., Zhang, M., Guo, Z., Wang, H., He, M., et al. (2015). Mn (II) mediated degradation of artemisinin based on Fe3O4@MnSiO3-FA nanospheres for cancer therapy in vivo. Nanoscale 7, 12542–12551. doi: 10.1039/C5NR02402A
Chen, M., Xiong, F., Ma, L., Yao, H., Wang, Q., Wen, L., et al. (2016b). Inhibitory effect of magnetic FeO nanoparticles coloaded with homoharringtonine on human leukemia cells in vivo and in vitro. Int. J. Nanomed. 11, 4413–4422. doi: 10.2147/IJN.S105543
Chen, X., Chen, Z., Hu, B., Cai, P., Wang, S., Xiao, S., et al. (2018). Synergistic lysosomal activatable polymeric nanoprobe encapsulating pH sensitive imidazole derivative for tumor diagnosis. Small 14:1703164. doi: 10.1002/smll.201703164
Chu, M., Ding, R., Chu, Z. Y., Zhang, M. B., Liu, X. Y., Xie, S. H., et al. (2014). Role of berberine in anti-bacterial as a high-affinity LPS antagonist binding to TLR4/MD-2 receptor. BMC Comp. Altern. Med. 14:89. doi: 10.1186/1472-6882-14-89
Cordeiro, R. A., Serra, A., and Coelho, J. F. J. (2019). Poly (β-amino ester)-based gene delivery systems: from discovery to therapeutic applications. J. Control. Release 310, 155–187. doi: 10.1016/j.jconrel.2019.08.024
Cruz Dos Santos, S., Osti Silva, N., Dos Santos Espinelli, J. B. Jr., Germani Marinho, M. A., Vieira Borges, Z., Bruzamarello Caon Branco, N., et al. (2019). Molecular interactions and physico-chemical characterization of quercetin-loaded magnetoliposomes. Chem. Phys. Lipids 218, 22–33. doi: 10.1016/j.chemphyslip.2018.11.010
Cui, T., Zhang, S., and Sun, H. (2017). Co-delivery of doxorubicin and pH-sensitive curcumin prodrug by transferrin-targeted nanoparticles for breast cancer treatment. Oncol. Report. 37, 1253-1260. doi: 10.3892/or.2017.5345
Daglioglu, C. (2017). Enhancing Tumor Cell Response to Multidrug Resistance with pH-Sensitive Quercetin and Doxorubicin Conjugated Multifunctional Nanoparticles. Colloids Surf. B Biointerfaces 156, 175–185. doi: 10.1016/j.colsurfb.2017.05.012
de Oliveira Pedro, R., Goycoolea, F. M., Pereira, S., Schmitt, C. C., and Neumann, M. G. (2018). Synergistic effect of quercetin and pH-responsive DEAE-chitosan carriers as drug delivery system for breast cancer treatment. Int. J. Biol. Macromol. 106, 579–586. doi: 10.1016/j.ijbiomac.2017.08.056
Dhivya, R., Ranjani, J., Rajendhran, J., Mayandi, J., and Annaraj, J. (2018). Enhancing the anti-gastric cancer activity of curcumin with biocompatible and pH sensitive PMMA-AA/ZnO nanoparticles. Mater. Sci. Eng. C Mater. Biol. Appl. 82, 182–189. doi: 10.1016/j.msec.2017.08.058
Ding, B., Wahid, M. A., Wang, Z., Xie, C., Thakkar, A., Prabhu, S., et al. (2017). Triptolide and celastrol loaded silk fibroin nanoparticles show synergistic effect against human pancreatic cancer cells. Nanoscale 9, 11739–11753. doi: 10.1039/C7NR03016A
Ding, Y., Wan, J., Zhang, Z., Wang, F., Guo, J., and Wang, C. (2018). Localized Fe(II)-induced cytotoxic reactive oxygen species generating nanosystem for enhanced anticancer therapy. ACS Appl. Mater. Interfaces 10, 4439–4449. doi: 10.1021/acsami.7b16999
Dong, X., Zou, S., Guo, C., Wang, K., Zhao, F., Fan, H., et al. (2018). Multifunctional redox-responsive and CD44 receptor targeting polymer-drug nanomedicine based curcumin and alendronate: synthesis, characterization and in vitro evaluation. Artif. Cells Nanomed. Biotechnol. 46, 168–177. doi: 10.1080/21691401.2017.1416390
Efferth, T. (2017). Cancer combination therapies with artemisinin-type drugs. Biochem. Pharmacol. 139, 56–70. doi: 10.1016/j.bcp.2017.03.019
Fang, F., Gong, C., Qian, Z., Zhang, X., Gou, M., You, C., et al. (2009). Honokiol nanoparticles in thermosensitive hydrogel: therapeutic effects on malignant pleural effusion. ACS Nano 3, 4080–4088. doi: 10.1021/nn900785b
Fang, L., Fan, H., Guo, C., Cui, L., Zhang, P., and Mu, H. (2019a). Novel mitochondrial targeting multifunctional surface charge-reversal polymeric nanoparticles for cancer treatment. J Biomed Nanotechnol. 15, 2151–2163. doi: 10.1166/jbn.2019.2854
Fang, L., Zhang, W., Wang, Z., Fan, X., Cheng, Z., Hou, X., et al. (2019b). Novel mitochondrial targeting charge-reversal polysaccharide hybrid shell/core nanoparticles for prolonged systemic circulation and antitumor drug delivery. Drug Deliv. 26, 1125–1139. doi: 10.1080/10717544.2019.1687614
Fang, X. B., Zhang, J. M., Xie, X., Liu, D., He, C. W., Wan, J. B., et al. (2016). pH-sensitive micelles based on acid-labile pluronic F68-curcumin conjugates for improved tumor intracellular drug delivery. Int. J. Pharm. 502, 28–37. doi: 10.1016/j.ijpharm.2016.01.029
Feng, Y., Li, N. X., Yin, H. L., Chen, T. Y., Yang, Q., and Wu, M. (2019). Thermo- and pH-responsive, lipid-coated, mesoporous silica nanoparticle-based dual drug delivery system to improve the antitumor effect of hydrophobic drugs. Mol. Pharm. 16, 422–436. doi: 10.1021/acs.molpharmaceut.8b01073
Ganapathy-Kanniappan, S., and Geschwind, J. F. (2013). Tumor glycolysis as a target for cancer therapy: progress and prospects. Mol. Cancer 12:152. doi: 10.1186/1476-4598-12-152
Gao, C., Jian, J., Lin, Z., Yu, Y. X., Jiang, B. P., Chen, H., et al. (2019a). Hypericin-loaded carbon nanohorn hybrid for combined photodynamic and photothermal therapy in vivo. Langmuir 35, 8228–8237. doi: 10.1021/acs.langmuir.9b00624
Gao, L., Chen, Q., Gong, T., Liu, J., and Li, C. (2019b). Recent advancement of imidazolate framework (ZIF-8) based nanoformulations for synergistic tumor therapy. Nanoscale 11, 21030–21045. doi: 10.1039/C9NR06558J
Gharib, A., Faezizadeh, Z., and Mesbah-Namin, S. A. (2015). Experimental treatment of breast cancer-bearing BALB/c mice by artemisinin and transferrin-loaded magnetic nanoliposomes. Pharmacogn. Mag. 11, S117–S122. doi: 10.4103/0973-1296.157710
Ghorbani, F., Imanparast, A., and Hataminia, F. (2018). A novel nano-superparamagnetic agent for photodynamic and photothermal therapies: an in-vitro study. Photodiagn. Photodyn. Ther. 23, 314–324. doi: 10.1016/j.pdpdt.2018.07.008
Golombek, S. K., May, J. N., Theek, B., Appold, L., Drude, N., Kiessling, F., et al. (2018). Tumor targeting via EPR: strategies to enhance patient responses. Adv. Drug Deliv. Rev. 130, 17–38. doi: 10.1016/j.addr.2018.07.007
Gupta, A., Kaur, C. D., Saraf, S., and Saraf, S. (2016). Formulation, characterization, and evaluation of ligand-conjugated biodegradable quercetin nanoparticles for active targeting. Artif Cells Nanomed Biotechnol. 44, 960–970. doi: 10.3109/21691401.2015.1008503
Gupta, R., and Sharma, D. (2019). Biofunctionalization of magnetite nanoparticles with stevioside: effect on the size and thermal behaviour for use in hyperthermia applications. Int. J. Hyperthermia 36, 302–312. doi: 10.1080/02656736.2019.1565787
Hahn, Y. B., and Ahmad, R. (2012). Chemical and biological sensors based on metal oxide nanostructures. Chem. Commun. 48, 10369–10385. doi: 10.1039/c2cc34706g
Han, X., Taratula, O., Taratula, O., Xu, K., St Lorenz, A., Moses, A., et al. (2020). Biodegradable hypericin-containing nanoparticles for necrosis targeting and fluorescence imaging. Mol. Pharm. 17, 1538–1545. doi: 10.1021/acs.molpharmaceut.9b01238
Huntosova, V., Buzova, D., Petrovajova, D., Kasak, P., Nadova, Z., Jancura, D., et al. (2012). Development of a new LDL-based transport system for hydrophobic/amphiphilic drug delivery to cancer cells. Int. J. Pharm. 436, 463–471. doi: 10.1016/j.ijpharm.2012.07.005
Jaman, M. S. (2018). Ellagic acid, sulforaphane, and ursolic acid in the prevention and therapy of breast cancer: current evidence and future perspectives. Breast Cancer 25, 517–528. doi: 10.1007/s12282-018-0866-4
Jelezova, I., Drakalska, E., Momekova, D., Shalimova, N., Momekov, G., Konstantinov, S., et al. (2015). Curcumin loaded pH-sensitive hybrid lipid/block copolymer nanosized drug delivery systems. Eur. J. Pharm. Sci. 78, 67–78. doi: 10.1016/j.ejps.2015.07.005
Jiang, K., Chi, T., Li, T., Zheng, G., Fan, L., Liu, Y., et al. (2017). A smart pH-responsive nano-carrier as a drug delivery system for the targeted delivery of ursolic acid: suppresses cancer growth and metastasis by modulating P53/MMP-9/PTEN/CD44 mediated multiple signaling pathways. Nanoscale 9, 9428–9439. doi: 10.1039/C7NR01677H
Jin, Q., Zhu, W., Jiang, D., Zhang, R., Kutyreff, C. J., Engle, J. W., et al. (2017). Ultra-small iron-gallic acid coordination polymer nanoparticles for chelator-free labeling of Cu and multimodal imaging-guided photothermal therapy. Nanoscale 9, 12609–12617. doi: 10.1039/C7NR03086J
Kang, T., Li, F., Baik, S., Shao, W., Ling, D., and Hyeon, T. (2017). Surface design of magnetic nanoparticles for stimuli-responsive cancer imaging and therapy. Biomaterials 136, 98–114. doi: 10.1016/j.biomaterials.2017.05.013
Kim, G. S., Kim, D. H., Lim, J. J., Lee, J. J., Han, D. Y., Lee, W. M., et al. (2008). Biological and antibacterial activities of the natural herb Houttuynia cordata water extract against the intracellular bacterial pathogen salmonella within the RAW 264.7 macrophage. Biol. Pharm. Bull. 31, 2012–2017. doi: 10.1248/bpb.31.2012
Kim, S. T., Kim, S. Y., Lee, J., Kim, K., Park, S. H., Park, Y. S., et al. (2018). Triptolide as a novel agent in pancreatic cancer: the validation using patient derived pancreatic tumor cell line. BMC Cancer 18:1103. doi: 10.1186/s12885-018-4995-0
Kong, C., Li, Y., Liu, Z., Ye, J., Wang, Z., Zhang, L., et al. (2019). Targeting the oncogene KRAS mutant pancreatic cancer by synergistic blocking of lysosomal acidification and rapid drug release. ACS Nano 13, 4049–4063. doi: 10.1021/acsnano.8b08246
Li, H., Li, X., Shi, X., Li, Z., and Sun, Y. (2019a). Effects of magnetic dihydroartemisinin nano-liposome in inhibiting the proliferation of head and neck squamous cell carcinomas. Phytomedicine 56, 215–228. doi: 10.1016/j.phymed.2018.11.007
Li, K. T., Duan, Q. Q., Chen, Q., He, J. W., Tian, S., Lin, H. D., et al. (2016). The effect of aloe emodin-encapsulated nanoliposome-mediated r-caspase-3 gene transfection and photodynamic therapy on human gastric cancer cells. Cancer Med. 5, 361–369. doi: 10.1002/cam4.584
Li, M., Wang, Y., Jiang, S., Gao, Y., Zhang, W., Hu, S., et al. (2020). Biodistribution and biocompatibility of glycyrrhetinic acid and galactose-modified chitosan nanoparticles as a novel targeting vehicle for hepatocellular carcinoma. Nanomedicine 15, 145–161. doi: 10.2217/nnm-2018-0455
Li, S., Wang, L., Li, N., Liu, Y., and Su, H. (2017). Combination lung cancer chemotherapy: design of a pH-sensitive transferrin-PEG-Hz-lipid conjugate for the co-delivery of docetaxel and baicalin. Biomed. Pharmacother. 95, 548–555. doi: 10.1016/j.biopha.2017.08.090
Li, Y., Chen, M., Yao, B., Lu, X., Zhang, X., He, P., et al. (2019b). Transferrin receptor-targeted redox/pH-sensitive podophyllotoxin prodrug micelles for multidrug-resistant breast cancer therapy. J. Mater. Chem. B 7, 5814–5824. doi: 10.1039/C9TB00651F
Li, Z. H., Li, Y. Y., Hou, M., Yang, T., Lu, L. C., and Xu, X. Y. (2018b). Topically applied hypericin exhibits skin penetrability on nude mice. Lasers Med. Sci. 33, 1279–1286. doi: 10.1007/s10103-018-2479-8
Li, W., Wang, X. H., Luo, Z., Liu, L. F., Yan, C., Yan, C. Y., et al. (2018a). Traditional Chinese medicine as a potential source for HSV-1 therapy by acting on virus or the susceptibility of host. Int. J. Mol. Sci. 19:3266. doi: 10.3390/ijms19103266
Lin, S., Lei, K., Du, W., Yang, L., Shi, H., Gao, Y., et al. (2016). Enhancement of oxaliplatin sensitivity in human colorectal cancer by hypericin mediated photodynamic therapy via ROS-related mechanism. Int. J. Biochem. Cell Biol. 71, 24–34. doi: 10.1016/j.biocel.2015.12.003
Lin, Y. H., Lin, J. H., Chou, S. C., Chang, S. J., Chung, C. C., Chen, Y. S., et al. (2015). Berberine-loaded targeted nanoparticles as specific Helicobacter pylori eradication therapy: in vitro and in vivo study. Nanomedicine 10, 57–71. doi: 10.2217/nnm.14.76
Ling, D., Xia, H., Park, W., Hackett, M. J., Song, C., Na, K., et al. (2014). pH-sensitive nanoformulated triptolide as a targeted therapeutic strategy for hepatocellular carcinoma. ACS Nano 8, 8027–8039. doi: 10.1021/nn502074x
Liu, C., and Chang, F. (2011). Development and characterization of eucalyptol microemulsions for topic delivery of curcumin. Chem. Pharm. Bull. 59, 172–178. doi: 10.1248/cpb.59.172
Liu, H., Dong, Y., Gao, Y., Du, Z., Wang, Y., Cheng, P., et al. (2016). The fascinating effects of baicalein on cancer: a review. Int J. Mol. Sci. 17:1681. doi: 10.3390/ijms17101681
Liu, J., Huang, Y., Kumar, A., Tan, A., Jin, S., Mozhi, A., et al. (2014). pH-sensitive nano-systems for drug delivery in cancer therapy. Biotechnol. Adv. 32, 693–710. doi: 10.1016/j.biotechadv.2013.11.009
Liu, L., Wei, Y., Zhai, S., Chen, Q., and Xing, D. (2015). Dihydroartemisinin and transferrin dual-dressed nano-graphene oxide for a pH-triggered chemotherapy. Biomaterials 62, 35–46. doi: 10.1016/j.biomaterials.2015.05.036
Liu, M., Wang, B., Guo, C., Hou, X., Cheng, Z., and Chen, D. (2019). Novel multifunctional triple folic acid, biotin and CD44 targeting pH-sensitive nano-actiniaes for breast cancer combinational therapy. Drug Deliv. 26, 1002–1016. doi: 10.1080/10717544.2019.1669734
Liu, Q., Chen, F., Hou, L., Shen, L., Zhang, X., Wang, D., et al. (2018). Nanocarrier-mediated chemo-immunotherapy arrested cancer progression and induced tumor dormancy in desmoplastic melanoma. ACS Nano 12, 7812–7825. doi: 10.1021/acsnano.8b01890
Lu, Y., Han, S., Zheng, H., Ma, R., Ping, Y., Zou, J., et al. (2018). A novel RGDyC/PEG co-modified PAMAM dendrimer-loaded arsenic trioxide of glioma targeting delivery system. Int. J. Nanomedicine 13, 5937–5952. doi: 10.2147/IJN.S175418
Luan, J., Wu, K., Li, C., Liu, J., Ni, X., Xiao, M., et al. (2017). pH-Sensitive drug delivery system based on hydrophobic modified konjac glucomannan. Carbohydr. Polym. 171, 9–17. doi: 10.1016/j.carbpol.2017.04.094
Luo, H., Vong, C. T., Chen, H., Gao, Y., Lyu, P., Qiu, L., et al. (2019). Naturally occurring anti-cancer compounds: shining from Chinese herbal medicine. Chin. Med. 14:48. doi: 10.1186/s13020-019-0270-9
Lv, H., Zhen, C., Liu, J., Yang, P., Hu, L., and Shang, P. (2019). Unraveling the potential role of glutathione in multiple forms of cell death in cancer therapy. Oxid. Med. Cell Longev. 2019:3150145. doi: 10.1155/2019/3150145
Ma, S., Song, W., Xu, Y., Si, X., Lv, S., Zhang, Y., et al. (2020). Rationally designed polymer conjugate for tumor-specific amplification of oxidative stress and boosting antitumor immunity. Nano Lett. 20, 2514–2521. doi: 10.1021/acs.nanolett.9b05265
Ma, Z., Fan, Y., Wu, Y., Kebebe, D., Zhang, B., Lu, P., et al. (2019). Traditional Chinese medicine-combination therapies utilizing nanotechnology-based targeted delivery systems: a new strategy for antitumor treatment. Int. J. Nanomed. 14, 2029–2053. doi: 10.2147/IJN.S197889
Madhusudana Rao, K., Krishna Rao, K. S., Ramanjaneyulu, G., and Ha, C. S. (2015). Curcumin encapsulated pH sensitive gelatin based interpenetrating polymeric network nanogels for anti cancer drug delivery. Int. J. Pharm. 478, 788–795. doi: 10.1016/j.ijpharm.2014.12.001
Maeda, H. (2017). Polymer therapeutics and the EPR effect. J. Drug Target 25, 781–785. doi: 10.1080/1061186X.2017.1365878
Manayi, A., Nikan, M., Nobakht-Haghighi, N., and Abdollahi, M. (2018). Advances in the anticancer value of the ursolic acid through nanodelivery. Curr. Med. Chem. 25, 4866–4875. doi: 10.2174/0929867324666170713102918
Massaro, M., Amorati, R., Cavallaro, G., Guernelli, S., Lazzara, G., Milioto, S., et al. (2016). Direct chemical grafted curcumin on halloysite nanotubes as dual-responsive prodrug for pharmacological applications. Colloids Surf. B Biointerfaces 140, 505–513. doi: 10.1016/j.colsurfb.2016.01.025
Mateo, D., Santiago-Portillo, A., Albero, J., Navalón, S., Alvaro, M., and García, H. (2019). Long-term photostability in terephthalate metal-organic frameworks. Angew. Chem. Int. Ed. Engl. 58, 17843–17848. doi: 10.1002/anie.201911600
Mlala, S., Oyedeji, A. O., Gondwe, M., and Oyedeji, O. O. (2019). Ursolic acid and its derivatives as bioactive agents. Molecules 24:E2751. doi: 10.3390/molecules24152751
Moloney, M. G. (2016). Natural products as a source for novel antibiotics. Trends Pharmacol. Sci. 37, 689–701. doi: 10.1016/j.tips.2016.05.001
Montanha, M. C., Silva, L. L., Pangoni, F., Cesar, G. B., Gon?alves, R. S., Caetano, W., et al. (2017). Response surface method optimization of a novel Hypericin formulation in P123 micelles for colorectal cancer and antimicrobial photodynamic therapy. J. Photochem. Photobiol. B Biol. 170, 247–255. doi: 10.1016/j.jphotobiol.2017.04.008
Noh, J., Kwon, B., Han, E., Park, M., Yang, W., Cho, W., et al. (2015). Amplification of oxidative stress by a dual stimuli-responsive hybrid drug enhances cancer cell death. Nat. Commun. 6:6907. doi: 10.1038/ncomms7907
Ou, K., Kang, Y., Chen, L., Zhang, X., Chen, X., Zheng, Y., et al. (2019). H2O2-responsive nano-prodrug for podophyllotoxin delivery. Biomater. Sci. 7, 2491–2498. doi: 10.1039/C9BM00344D
Pandey, S., Mewada, A., Thakur, M., Shah, R., Oza, G., and Sharon, M. (2013). Biogenic gold nanoparticles as fotillas to fire berberine hydrochloride using folic acid as molecular road map. Mater. Sci. Eng. C Mater. Biol. Appl. 33, 3716–3722. doi: 10.1016/j.msec.2013.05.007
Pang, Y., Mai, Z., Wang, B., Wang, L., Wu, L., Wang, X., et al. (2017). Artesunate-modified nano-graphene oxide for chemo-photothermal cancer therapy. Oncotarget 8, 93800–93812. doi: 10.18632/oncotarget.21191
Park, S. C., Kim, N. H., Yang, W., Nah, J. W., Jang, M. K., and Lee, D. (2016). Polymeric micellar nanoplatforms for Fenton reaction as a new class of antibacterial agents. J. Control. Release 221, 37–47. doi: 10.1016/j.jconrel.2015.11.027
Patel, R. V., Mistry, B. M., Shinde, S. K., Syed, R., Singh, V., and Shin, H. S. (2018). Therapeutic potential of quercetin as a cardiovascular agent. Eur. J. Med. Chem. 155, 889–904. doi: 10.1016/j.ejmech.2018.06.053
Reeßing, F., and Szymanski, W. (2017). Beyond photodynamic therapy: light-activated cancer chemotherapy. Curr. Med. Chem. 24, 4905–4950. doi: 10.2174/0929867323666160906103223
Rejinold, N. S., Sreerekha, P. R., Chennazhi, K. P., Nair, S. V., and Jayakumar, R. (2011). Biocompatible, biodegradable and thermo-sensitive chitosan-g-poly (N-isopropylacrylamide) nanocarrier for curcumin drug delivery. Int. J. Biol. Macromol. 49, 161–172. doi: 10.1016/j.ijbiomac.2011.04.008
Rkein, A. M. (2014). Photodynamic therapy. Dermatol. Clin. 32, 415–425. doi: 10.1016/j.det.2014.03.009
Sadeghi, S., Davoodvandi, A., Pourhanifeh, M. H., Sharifi, N., ArefNezhad, R., Sahebnasagh, R., et al. (2019). Anti-cancer effects of cinnamon: insights into its apoptosis effects. Eur. J. Med. Chem. 178, 131–140. doi: 10.1016/j.ejmech.2019.05.067
Sahu, P., Kashaw, S. K., Jain, S., Sau, S., and Iyer, A. K. (2017). Assessment of penetration potential of pH responsive double walled biodegradable nanogels coated with eucalyptus oil for the controlled delivery of 5-fluorouracil: in vitro and ex vivo studies. J. Control. Release 253, 122–136. doi: 10.1016/j.jconrel.2017.03.023
Sedghi, R., Oskooie, H. A., Heravi, M. M., Nabid, M. R., and Zarnani, A. H. (2013). Divergent synthesis of dendrimer-like pH-responsive macromolecules through a combination of ATRP and ROP for controlled release of anti-cancer drug. J. Mater. Chem. B 1, 773–786. doi: 10.1039/C2TB00359G
Shanmugam, M. K., Warrier, S., Kumar, A. P., Sethi, G., and Arfuso, F. (2017). Potential role of natural compounds as anti-angiogenic agents in cancer. Curr. Vasc. Pharmacol. 15, 503–519. doi: 10.2174/1570161115666170713094319
Shao, M., Chang, C., Liu, Z., Chen, K., Zhou, Y., Zheng, G., et al. (2019). Polydopamine coated hollow mesoporous silica nanoparticles as pH-sensitive nanocarriers for overcoming multidrug resistance. Colloids Surf. B Biointerfaces 183, 110427. doi: 10.1016/j.colsurfb.2019.110427
Shi, Y., Liu, S., Ahmad, S., and Gao, Q. (2018). Targeting key transporters in tumor glycolysis as a novel anticancer strategy. Curr. Top. Med. Chem. 18, 454–466. doi: 10.2174/1568026618666180523105234
Som, A., Bloch, S., Ippolito, J. E., and Achilefu, S. (2016). Acidic extracellular pH of tumors induces octamer-binding transcription factor 4 expression in murine fibroblasts in vitro and in vivo. Sci. Rep. 6:27803. doi: 10.1038/srep27803
Soomro, N. A., Wu, Q., Amur, S. A., Liang, H., Ur Rahman, A., Yuan, Q., et al. (2019). Natural drug physcion encapsulated zeolitic imidazolate framework, and their application as antimicrobial agent. Colloids Surf. B Biointerfaces 182:110364. doi: 10.1016/j.colsurfb.2019.110364
Sun, Y. Q., Dai, C. M., Zheng, Y., Shi, S. D., Hu, H. Y., and Chen, D. W. (2017). Binding effect of fluorescence labeled glycyrrhetinic acid with GA receptors in hepatocellular carcinoma cells. Life Sci. 188, 186–191. doi: 10.1016/j.lfs.2017.07.032
Tang, H., Zhao, W., Yu, J., Li, Y., and Zhao, C. (2018). Recent development of pH-responsive polymers for cancer nanomedicine. Molecules 24:E4. doi: 10.3390/molecules24010004
Tao, B., Deng, Y., Song, L., Ma, W., Qian, Y., Lin, C., et al. (2019a). BMP2-loaded titania nanotubes coating with pH-responsive multilayers for bacterial infections inhibition and osteogenic activity improvement. Colloids Surf B Biointerfaces 177, 242–252. doi: 10.1016/j.colsurfb.2019.02.014
Tao, R., Zhang, F., Tang, Q. J., Xu, C. S., Ni, Z. J., and Meng, X. H. (2019b). Effects of curcumin-based photodynamic treatment on the storage quality of fresh-cut apples. Food Chem. 274, 415–421. doi: 10.1016/j.foodchem.2018.08.042
Tian, C., Asghar, S., Xu, Y., Chen, Z., Zhang, M., Huang, L., et al. (2018). The effect of the molecular weight of hyaluronic acid on the physicochemical characterization of hyaluronic acid-curcumin conjugates and in vitro evaluation in glioma cells. Colloids Surf. B Biointerfaces 165, 45–55. doi: 10.1016/j.colsurfb.2018.02.016
Tian, Q., Zhang, C. N., Wang, X. H., Wang, W., Huang, W., Cha, R. T., et al. (2010). Glycyrrhetinic acid-modified chitosan/poly (ethylene glycol) nanoparticles for liver-targeted delivery. Biomaterials 31, 4748–4756. doi: 10.1016/j.biomaterials.2010.02.042
Tohmé, M. J., Giménez, M. C., Peralta, A., Colombo, M. I., and Delgui, L. R. (2019). Ursolic acid: a novel antiviral compound inhibiting rotavirus infection in vitro. Int. J. Antimicrob. Agents 54, 601–609. doi: 10.1016/j.ijantimicag.2019.07.015
Traverso, N., Ricciarelli, R., Nitti, M., Marengo, B., Furfaro, A. L., Pronzato, M. A., et al. (2013). Role of glutathione in cancer progression and chemoresistance. Oxid. Med. Cell Longev. 2013:972913. doi: 10.1155/2013/972913
Tu, Y. (2016). Artemisinin – a gift from traditional Chinese medicine to the world (Nobel Lecture). Angew. Chem. Int. Ed. Engl. 55, 10210–10226. doi: 10.1002/anie.201601967
Vangijzegem, T., Stanicki, D., and Laurent, S. (2019). Magnetic iron oxide nanoparticles for drug delivery: applications and characteristics. Exp. Opin. Drug Deliv. 16:69–78. doi: 10.1080/17425247.2019.1554647
Vinayak, M. (2019). Quercetin loaded nanoparticles in targeting cancer: recent development. Anticancer Agents Med. Chem. 19, 1560–1576. doi: 10.2174/1871520619666190705150214
Wang, B., Zhang, W., Zhou, X., Liu, M., Hou, X., Cheng, Z., et al. (2019a). Development of dual-targeted nano-dandelion based on an oligomeric hyaluronic acid polymer targeting tumor-associated macrophages for combination therapy of non-small cell lung cancer. Drug Deliv. 26, 1265–1279. doi: 10.1080/10717544.2019.1693707
Wang, C. Y., Bai, X. Y., and Wang, C. H. (2014). Traditional Chinese medicine: a treasured natural resource of anticancer drug research and development. Am. J. Chin. Med. 42, 543–559. doi: 10.1142/S0192415X14500359
Wang, D., Zhou, J., Chen, R., Shi, R., Xia, G., Zhou, S., et al. (2016a). Magnetically guided delivery of DHA and Fe ions for enhanced cancer therapy based on pH-responsive degradation of DHA-loaded Fe3O4@C@MIL-100(Fe) nanoparticles. Biomaterials 107, 88–101. doi: 10.1016/j.biomaterials.2016.08.039
Wang, D., Zhou, J., Chen, R., Shi, R., Zhao, G., Xia, G., et al. (2016b). Controllable synthesis of dual-MOFs nanostructures for pH-responsive artemisinin delivery, magnetic resonance and optical dual-model imaging-guided chemo/photothermal combinational cancer therapy. Biomaterials 100, 27–40. doi: 10.1016/j.biomaterials.2016.05.027
Wang, K., Guo, C., Zou, S., Yu, Y., Fan, X., Wang, B., et al. (2018). Synthesis, characterization and in vitro/in vivo evaluation of novel reduction-sensitive hybrid nano-echinus-like nanomedicine. Artif. Cells Nanomed. Biotechnol. 46, 659–667. doi: 10.1080/21691401.2018.1466147
Wang, K., Hu, H., Zhang, Q., Zhang, Y., and Shi, C. (2019b). Synthesis, purification, and anticancer effect of magnetic Fe3O4-loaded poly (lactic-co-glycolic) nanoparticles of the natural drug tetrandrine. J. Microencapsul. 36, 356–370. doi: 10.1080/02652048.2019.1631403
Wang, M., Zhao, T., Liu, Y., Wang, Q., Xing, S., Li, L., et al. (2017). Ursolic acid liposomes with chitosan modification: promising antitumor drug delivery and efficacy. Mater. Sci. Eng. C Mater. Biol. Appl. 71, 1231–1240. doi: 10.1016/j.msec.2016.11.014
Wang, S., Meng, X., and Dong, Y. (2017). Ursolic acid nanoparticles inhibit cervical cancer growth in vitro and in vivo via apoptosis induction. Int. J. Oncol. 50, 1330–1340. doi: 10.3892/ijo.2017.3890
Wang, Y., Wang, F., Liu, Y., Xu, S., Shen, Y., Feng, N., et al. (2018). Glutathione detonated and pH responsive nano-clusters of Au nanorods with a high dose of DOX for treatment of multidrug resistant cancer. Acta Biomater. 75, 334–345. doi: 10.1016/j.actbio.2018.06.012
Wang, Z., Chang, Z., Lu, M., Shao, D., Yue, J., Yang, D., et al. (2018a). Shape-controlled magnetic mesoporous silica nanoparticles for magnetically-mediated suicide gene therapy of hepatocellular carcinoma. Biomaterials 154, 147–157. doi: 10.1016/j.biomaterials.2017.10.047
Wang, Z., Wang, Y. S., Chang, Z. M., Li, L., Zhang, Y., Lu, M. M., et al. (2017). Berberine-loaded Janus nanocarriers for magnetic field-enhanced therapy against hepatocellular carcinoma. Chem. Biol. Drug Res. 89, 464–469. doi: 10.1111/cbdd.12866
Wang, Z., Xia, Q., Liu, X., Liu, W., Huang, W., Mei, X., et al. (2018b). Phytochemistry, pharmacology, quality control and future research of Forsythia suspensa (Thunb.) Vahl: a review. J. Ethnopharmacol. 210, 318–339. doi: 10.1016/j.jep.2017.08.040
Wani, K. D., Kadu, B. S., Mansara, P., Gupta, P., Deore, A. V., Chikate, R. C., et al. (2014). Synthesis, characterization and in vitro study of biocompatible cinnamaldehyde functionalized magnetite nanoparticles (CPGF Nps) for hyperthermia and drug delivery applications in breast cancer. PLoS ONE 9:e107315. doi: 10.1371/journal.pone.0107315
Wu, M., Cao, Z., Zhao, Y., Zeng, R., Tu, M., and Zhao, J. (2016). Novel self-assembled pH-responsive biomimetic nanocarriers for drug delivery. Mater. Sci. Eng. C Mater. Biol. Appl. 64, 346–353. doi: 10.1016/j.msec.2016.03.099
Wu, Q., Gao, H., Vriesekoop, F., Liu, Z., He, J., and Liang, H. (2020). Calcium phosphate coated core-shell protein nanocarriers: Robust stability, controlled release and enhanced anticancer activity for curcumin delivery. Mater. Sci. Eng. C Mater. Biol. Appl. 115:111094. doi: 10.1016/j.msec.2020.111094
Xing, L., Lyu, J. Y., Yang, Y., Cui, P. F., Gu, L. Q., Qiao, J. B., et al. (2017). pH-Responsive de-PEGylated nanoparticles based on triphenylphosphine-quercetin self-assemblies for mitochondria-targeted cancer therapy. Chem. Comm. 53, 8790–8793. doi: 10.1039/c7cc04058j
Yan, T., Cheng, J., Liu, Z., Cheng, F., Wei, X., Huang, Y., et al. (2018). Acid-sensitive polymeric vector targeting to hepatocarcinoma cells via glycyrrhetinic acid receptor-mediated endocytosis. Mater. Sci. Eng. C Mater. Biol. Appl. 87, 32–40. doi: 10.1016/j.msec.2018.02.013
Yang, Y., Islam, M. S., Wang, J., Li, Y., and Chen, X. (2020). Traditional Chinese medicine in the treatment of patients infected with 2019-new coronavirus (SARS-CoV-2): a review and perspective. Int. J. Biol. Sci. 16, 1708–1717. doi: 10.7150/ijbs.45538
Yao, J., Zhang, H., Ma, L., Mu, X., Wang, Y., Lu, Y., et al. (2018). Effect of traditional Chinese medicine Bupleurum in the treatment of influenza A (H1N1). Pak. J. Pharm. Sci. 31, 1713–1717.
Yin, R., Li, T., Tian, J. X., Xi, P., and Liu, R. H. (2016). Ursolic acid, a potential anticancer compound for breast cancer therapy. Crit. Rev. Food Sci. Nutr. 2016, 1–7. doi: 10.1080/10408398.2016.1203755
Yoo, W., Yoo, D., Hong, E., Jung, E., Go, Y., Singh, S., et al. (2018). Acid-activatable oxidative stress-inducing polysaccharide nanoparticles for anticancer therapy. J. Control. Release 269, 235–244. doi: 10.1016/j.jconrel.2017.11.023
Younes, I. (2015). Chitin and chitosan preparation from marine sources. Structure, properties and applications. Mar. Drugs 13, 1133–1174. doi: 10.3390/md13031133
Yu, D., Ruan, P., Meng, Z., and Zhou, J. (2015). The structure-dependent electric release and enhanced oxidation of drug in graphene oxide-based nanocarrier loaded with anticancer herbal drug berberine. J. Pharm. Sci. 104, 2489–2500. doi: 10.1002/jps.24491
Yu, Y., and Zhang, X. (2014). The anti-tumor efficacy of curcumin when delivered by size/charge-changing multistage polymeric micelles based on amphiphilic poly (β-amino ester) derivates. Biomaterials 35, 3467–3479. doi: 10.1016/j.biomaterials.2013.12.096
Zhang, H. Y., Sun, C. Y., Adu-Frimpong, M., Yu, J. N., and Xu, X. M. (2019). Glutathione-sensitive PEGylated curcumin prodrug nanomicelles: preparation, characterization, cellular uptake and bioavailability evaluation. Int. J. Pharm. 555, 270–279. doi: 10.1016/j.ijpharm.2018.11.049
Zhang, J., Li, J., Shi, Z., Yang, Y., Xie, X., Lee, S. M., et al. (2017). pH-sensitive polymeric nanoparticles for co-delivery of doxorubicin and curcumin to treat cancer via enhanced pro-apoptotic and anti-angiogenic activities. Acta Biomater. 58, 349–364. doi: 10.1016/j.actbio.2017.04.029
Zhang, K., Gao, S., Guo, J., Ni, G., Chen, Z., Li, F., et al. (2018a). Hypericin-photodynamic therapy inhibits proliferation and induces apoptosis in human rheumatoid arthritis fibroblast-like synoviocytes cell line MH7A. Iran J. Basic Med. Sci. 21, 130–137. doi: 10.22038/IJBMS.2018.23871.5991
Zhang, P., Wu, J., Xiao, F., Zhao, D., and Luan, Y. (2018b). Disulfide bond based polymeric drug carriers for cancer chemotherapy and relevant redox environments in mammals. Med. Res. Rev. 38, 1485–1510. doi: 10.1002/med.21485
Zhang, Z., Cheng, W., Pan, Y., and Jia, L. (2020a). An anticancer agent-loaded PLGA nanomedicine with glutathione-response and targeted delivery for the treatment of lung cancer. J. Mater. Chem. B 8, 655–665. doi: 10.1039/C9TB02284H
Zhang, Z., Wang, R., Huang, X., Luo, R., Xue, J., Gao, J., et al. (2020b). Self-delivered and self-monitored chemo-photodynamic nanoparticles with light-triggered synergistic antitumor therapies by downregulation of HIF-1α and depletion of GSH. ACS App. Mater. Interfaces. 12, 5680–5694. doi: 10.1021/acsami.9b23325
Zhang, Z., Xu, S., Wang, Y., Yu, Y., Li, F., Zhu, H., et al. (2018c). Near-infrared triggered co-delivery of doxorubicin and quercetin by using gold nanocages with tetradecanol to maximize anti-tumor effects on MCF-7/ADR cells. J Colloid Interface Sci. 509, 47–57. doi: 10.1016/j.jcis.2017.08.097
Zhao, C., Cao, W., Zheng, H., Xiao, Z., Hu, J., Yang, L., et al. (2019a). Acid-responsive nanoparticles as a novel oxidative stress-inducing anticancer therapeutic agent for colon cancer. Int. J. Nanomed. 14, 1597–1618. doi: 10.2147/IJN.S189923
Zhao, Y., Li, H., Wei, S., Zhou, X., and Xiao, X. (2019b). Antimicrobial effects of chemical compounds isolated from traditional Chinese herbal medicine (TCHM) against drug-resistant bacteria: a review paper. Mini Rev. Med. Chem. 19, 125–137. doi: 10.2174/1389557518666181017143141
Zhong, Y., Zou, Y., Liu, L., Li, R., Xue, F., and Yi, T. (2020). pH-responsive Ag2S nanodots loaded with heat shock protein 70 inhibitor for photoacoustic imaging-guided photothermal cancer therapy. Acta Biomater. 115, 358–70. doi: 10.1016/j.actbio.2020.08.007
Zhu, K., Zhou, L., Zou, M., Ning, S., Liu, S., Zhou, Y., et al. (2020). 18-GA-Suc modified liposome loading cantharidin for augmenting hepatic specificity: preparation, characterization, antitumor effects, and liver-targeting efficiency. J. Pharm. Sci. 109, 2038–2047. doi: 10.1016/j.xphs.2020.03.001
Zhu, L., Zhou, Z., and Mao, H. (2017). Magnetic nanoparticles for precision oncology: theranostic magnetic iron oxide nanoparticles for image-guided and targeted cancer therapy. Nanomedicine 12, 73–87. doi: 10.2217/nnm-2016-0316
Keywords: smart responsive nanoformulation, traditional Chinese medicine, targeted drug delivery, tumor, infectious disease
Citation: Jiang X, Lin M, Huang J, Mo M, Liu H, Jiang Y, Cai X, Leung W and Xu C (2020) Smart Responsive Nanoformulation for Targeted Delivery of Active Compounds From Traditional Chinese Medicine. Front. Chem. 8:559159. doi: 10.3389/fchem.2020.559159
Received: 05 May 2020; Accepted: 30 October 2020;
Published: 10 December 2020.
Edited by:
Fang Liu, Guangzhou University of Chinese Medicine, ChinaReviewed by:
Zhiling Yu, Hong Kong Baptist University, Hong KongYingwei Hou, University of Illinois at Urbana-Champaign, United States
Copyright © 2020 Jiang, Lin, Huang, Mo, Liu, Jiang, Cai, Leung and Xu. This is an open-access article distributed under the terms of the Creative Commons Attribution License (CC BY). The use, distribution or reproduction in other forums is permitted, provided the original author(s) and the copyright owner(s) are credited and that the original publication in this journal is cited, in accordance with accepted academic practice. No use, distribution or reproduction is permitted which does not comply with these terms.
*Correspondence: Chuanshan Xu, eGNzaGFuQDE2My5jb20=