- 1Department of Pharmacy, Faculty of Biological Sciences, University of Malakand, Chakdara, Pakistan
- 2Department of Chemistry, COMSATS University Islamabad, Abbottabad Campus, Abbottabad, Pakistan
- 3Department of Biochemistry, University of Malakand, Chakdara, Pakistan
- 4Department of Pharmacognosy, College of Pharmacy, King Saud University, Riyadh, Saudi Arabia
- 5Department of Pharmacy, COMSATS University Islamabad, Abbottabad Campus, Abbottabad, Pakistan
- 6Department of Biotechnology, Abdul Wali Khan University Mardan, Mardan, Pakistan
- 7Department of Biotechnology, Faculty of Biological Sciences, University of Malakand, Chakdara, Pakistan
Natural-based drugs are believed to be safe, effective and economical. Based on the medicinal importance of the genus Eryngium and unexplored nature of Eryngium caeruleum, we have evaluated its antidiabetic and antioxidant potentials. Both in-vitro and in-vivo assays have been carried out for antidiabetic assays. The antioxidant activity was determined by using different free radicals [i.e., 1,1-diphenyl,2-picrylhydrazyl (DPPH), 2,2-azinobis[3-ethylbenzthiazoline]-6-sulfonic acid (ABTS), and hydrogen peroxide (H2O2)]. Moreover, different phytoconstituents were identified in the most active solvent fraction by GC-MS analysis. Furthermore, comparative fingerprints of methanolic extract and chloroform fraction were also analyzed via High Performance Liquid Chromatography coupled with Diode Array Detector (HPLC-DAD). The crude methanolic extract of E. caeruleum (Ec.Cr) and its sub-fractions [i.e., n-hexane (Ec.Hex), chloroform (Ec.Chf), ethyl acetate (Ec.EtAc), and aqueous (Ec.Aq) were employed in this study]. In the α-glucosidase inhibition assay, a concentration-dependent inhibitory response was observed against the enzyme. The most active sample was Ec.Chf which revealed an IC50 of 437 μg/ml in comparison to the standard acarbose (IC50 25 μg/ml). The rest of the samples showed moderate inhibition of α-glucosidase. In antioxidant assays, Ec.Chf and Ec.Cr exhibited a considerable scavenging effect against all the free radicals. The IC50 values recorded for Ec.Chf were 112, 109, and 150 μg/ml against DPPH, ABTS, and H2O2 respectively. Based on the in-vitro potential of Ec.Chf, this was subjected to the in-vivo model experiment. The Ec.Chf lowered the blood glucose level up to 10.3 mmol/L at 500 μg/Kg. The Ec.Chf was also subjected to GC-MS analysis. The GC-MS analysis confirmed the presence of 60 compounds. The identified phytoconstituents consist of some essential compounds previously reported with antidiabetic and antioxidant studies, which include thymol, tocopherol, phytol, nerolidol, (I)-neophytadiene, linolenic acid, and falcarinol. Similarly, the HPLC-DAD chromatograms of Ec.Cr and Ec.Chf exhibited a variety of peaks, which further demonstrates the possibility of important phytochemicals. In a nutshell, we can conclude that Eryngium caeruleum is a potential source of bioactive compounds which may be beneficial for the management of ailments like diabetes and free radicals mediated disorders. Molecular docking was performed to explore the possible role of all the identified bioactive compounds in the chloroform fraction of Eryngium caeruleum into active sites of the homology model of α-glucosidase.
Introduction
Diabetes mellitus (DM) is a disorder of carbohydrate, fat and protein metabolism attributed to low production of insulin or mounting resistance to its action leading to persistent hyperglycemia. DM affects about 5% of the population globally and its management exclusive of any side effects is still an issue that confronts the scientific community (Kim et al., 2005) Elevated postprandial blood glucose levels are extensively known as one of the initial disease markers in the prediction of consequent micro-vascular and macro-vascular complications, which ultimately progresses to type 2 DM (Fonseca, 2003). Type 2 DM usually results from defects in insulin secretion and its action. Such insufficiency leads to high concentrations of blood glucose, which sequentially damages many of the body's systems, particularly the blood vessels. Other complications include retinopathy, neuropathy, nephropathy, and angiopathy. One of the strategies for the management of non-insulin dependent diabetes mellitus (NIDDM) is to reduce postprandial hyperglycemia by reducing glucose absorption from the intestinal tract. This strategy is based on the inhibition of key enzymes involved in the metabolism of carbohydrates to simple monosaccharides which are subsequently absorbed (Hussain et al., 2019; Rahim et al., 2019). Among these enzymes α-glucosidase is a crucial enzyme which is widely distributed in animals, plants, and microorganisms (Kimura et al., 2004). This membrane-bound intestinal enzyme is implicated in the catabolism and liberation of α-glucose from the non-reducing side of the complex sugars, making them available for absorption (Nichols et al., 2002). Thus, the administration of enzyme inhibitors will reduce the cleavage of dietary complex carbohydrates to simple monosaccharides, thus leading to a reduction in postprandial glucose levels and suppression of postprandial hyperglycemia (van de Laar, 2008).
Among the currently available synthetic drugs that target the dual metabolic defects of type 2 DM that are impaired insulin secretion and insulin resistance, some of these drugs produce some serious side effects at high doses (Carroll et al., 2003). Therefore, the main goal of anti-diabetic research is to discover anti-hyperglycemic agents that are comparatively safe with fewer side effects. In this regard, research has begun to study traditional medicines and foods for the discovery of new therapeutic drugs against type 2 DM. Traditional medicines including plants and herbal extracts have a long history of use as anti-diabetic agents (Grover et al., 2002). Indeed, a lot of medicinal plants have been reported inhibiting the activity of α-glucosidase (Bnouham et al., 2006). Dietary α-glucosidase inhibitors that act by inhibiting the enzymatic breakdown of starch, soluble carbohydrates, and their derived products have been recognized as a potential natural and safe approach for reduction of hyperglycemia via modulation of meal derived glucose absorption (Bischoff, 1994).
In DM, hyperglycemia set free reactive oxygen species (ROS) called free radicals, which subsequently cause lipid peroxidation, membrane damage and play a vital role in the initiation of secondary complications of kidneys, eyes, blood vessels, nervous system, and DM (Hunt et al., 1988). Numerous studies established that oxidative stress is implicated in the development of insulin resistance via inhibition of insulin signals and adipokines dysregulation (Houstis et al., 2006). Antioxidants have been reported to thwart the destruction of β-cells via inhibition of peroxidation chain reaction and consequently offer protection against the development of DM (Montonen, 2005). The antioxidant can be from both organic and inorganic sources (Bibi et al., 2019; Zafar et al., 2020). However, medicinal plants are enriched with natural antioxidants that can protect β-cell function and stop diabetes mediated ROS formation (Sadiq et al., 2015a; Begum et al., 2019).
Eryngium caeruleum belongs to the family Apiaceae. The genus Eryngium comprises of 250 species out of which less than 30 species have been investigated phytochemically to date (Capetanos et al., 2007). E. caeruleum is one of the unexplored flora of Eryngium. Several species of this genus have been used traditionally for the treatment of various ailments for instance, edema, inflammatory disorders, sinusitis, snake and scorpion bites, wound healing, urinary tract infections, as antitussive, fertility disorders etc., (Singh et al., 2002).
Rehman et al. investigated the aerial parts of Eryngium caeruleum and isolated two new flavone glycosides (1 and 2, Figure 1) (Rehman et al., 2017). These two isolated compounds were evaluated for their potential against α-glucosidase, aldose reductase (ALR1 and ALR2). Compounds were only found active against ALR1 and ALR2.
We previously reported the antimicrobial properties of E. caeruleum (Sadiq et al., 2016). As is obvious from the current literature, only the essential oil of this plant has been explored up to some extent with excellent pharmacological activities (Saeedi and Morteza-Semnani, 2008). The plant powder and extract of E. caeruleum are still to be explored. Moreover, the isolated chemicals by Rehman et al. were not able to show α- β-glucosidase inhibition and antiglycation potential. Being unexplored and one of the specie of important genus, this investigational study was inevitable. Therefore, the current investigational study is arranged to assay various solvent fractions of E. caeruleum for inhibitions of α-glucosidase and free radicals followed by GC-MS analysis of the most potent solvent fraction.
Methods
Chemicals and Drugs
The solvents used in the study were of analytical grades which were purchased from Sigma Aldrich via local venders. The solvents used in HPLC analysis were of HPLC grades. The chemicals and drugs used were purchased from the local suppliers of Sigma Aldrich. The chemicals and drugs used were α-glucosidase (9001-42-7), alloxan monohydrate (ALX2244-11-3), Acarbose (56180-94-0), p-Nitrophenyl-α-D-glucopyranoside (3767-28-0) 2,2-diphenyl-1-picrylhydrazyl (1898-66-4), 2,2′-Azino-bis(3-ethylbenzothiazoline-6-sulfonic acid) (30931-67-0), Hydrogen peroxide, glibenclamide (10238-21-8), Ascorbic acid (50-81-7).
Plant Collection and Extraction
The aerial parts of Eryngium caeruleum were collected from various areas of the Malakand division and authenticated by Dr. Nasrullah at Department of Botany, University of Malakand. The plant was pressed and kept in the herbarium of University of Malakand, Pakistan under voucher specimen number H.UOM.BG.109. After collection, the plant was cut into small pieces and allowed to dry in shade. After drying, the plant was powdered using a cutter mill and macerated in 80% methanol for two weeks. After soaking, the plant sample was filtered using Whatman filter paper. The filtrates were evaporated under reduced pressure using rotary evaporator at 40°C (Farooq et al., 2019; Sadiq et al., 2019). Semi solid masse of methanolic extract of E. caerulum was obtained weighing ~400 g.
Fractionation
The successive solvent-solvent extraction method was followed for the fractionation of crude methanolic extract (Shah et al., 2014a). In brief, the crude extract of E. caerulum weighing 300 g was suspended in 500 ml of distilled water in separating funnel and 500 ml of n-hexane was poured into it. It was vigorously shaken and allowed to stand in a separating funnel to be separated into two distinct layers. The upper n-hexane layer was collected, and the same procedure was followed until a colorless n-hexane layer was obtained. After the collection of n-hexane fraction, it was fractionated with other solvents with increasing order of polarity (i.e., chloroform, ethyl acetate and the aqueous fraction). The fractions obtained for n-hexane, chloroform, ethyl acetate and aqueous fractions of E. caerulum were allowed to get converted into semisolid masses weighing 62 g (20. 66%), 37 g (12.33%), 59 g (19.66%), and 110 g (36.66%) respectively.
In-vitro α-Glucosidase Inhibition Assay
The α-glucosidase inhibitory potentials of solvent extracts from E. caeruleum were investigated following the reported protocol (Aslam et al., 2018). The enzyme solution was made by dissolving 0.5 unit/ml in a 0.1 M phosphate buffer (pH 6.9). The final concentration of enzyme solution was 20 μl α-glucosidase (0.5 unit/ml). Whereas, p-Nitrophenyl-α-D-glucopyranoside (5 mM) was made in the same buffer (pH 6.9) which acted as substrate for the reaction. Test samples prepared were mixed with the enzyme solution and incubated for 15 min at 37°C. Finally, 20 μl substrate solution was added to the enzyme mixture and was incubated for 15 min at 37°C. The reaction was completed by the addition of 80 μl of 0.2 M sodium carbonate solution. Absorbances were measured at 405 nm using UV spectrophotometer (Thermo electron corporation, USA). The system without α-glucosidase was used as the blank and acarbose was the positive control. Each experiment was conducted in triplicate and percent inhibitions were calculated using the following formula;
Antioxidant Assays
DPPH Radical Scavenging Assay
DPPH free radical scavenging ability of test samples were tested according to previously reported procedures (Shah et al., 2015; Zahoor et al., 2018). Samples solutions were prepared ranging from 125 to 1,000 μg/ml and were added to 0.004% methanolic solution of DPPH. Following incubation for 30 min, absorbance values were recorded at 517 nm using UV spectrophotometer (Thermo electron corporation, USA). DPPH scavenging activities were calculated as;
Where A0 characterizes absorbance of the control and A1 is the absorbance of test samples. Ascorbic acid was used as a positive control. Where A0 characterizes absorbance of control and A1 is the absorbance of test samples. All experiments were performed in triplicate and inhibition graphs were made with the help of GraphPad prism program (GraphPAD, San Diego, California, USA).
ABTS Free Radical Scavenging Assay
The ABTS free radical scavenging potential of Plant samples were tested following previously published procedure (Zafar et al., 2019). Briefly, solutions of ABTS 7 mM and potassium persulphate (K2S2O4) 2.45 mM were mixed and stored in a dark place at room temperature for about 12–16 h to obtain a dark colored solution. This solution was diluted using Phosphate buffer (0.01 M) pH 7.4 and absorbance value was adjusted to 0.70 at 734 nm. Finally, 300 μl solution of the test sample was added to 3.0 ml of ABTS solution in cuvette and was analyzed using a UV spectrophotometer (Thermo electron corporation, USA) at 734 nm. Reduction in absorbances was determined following 1 min of mixing the solutions and analysis was continued for 6 min. Ascorbic acid was used as a positive control. The assay was repeated in triplicate and percentage inhibition was calculated using the formula;
Hydrogen Peroxide Scavenging Assay
Hydrogen peroxide (H2O2) scavenging capacity of plant samples were evaluated using the previously reported method (Ahmad et al., 2019). In brief, 2 mM hydrogen peroxide solution was prepared in 50 mM phosphate buffer with 7.4 pH. Plants samples (0.1 ml) were taken in test tubes and their volumes were raised to 0.4 ml using 50 mM phosphate buffer solution. H2O2 (0.6 ml) was added to the tubes and were properly mixed using vertex mixer. Absorbance of each sample was measured at 230 nm against the blank after 10 min. H2O2 scavenging activity was calculated using the equation;
Experimental Animals
In the in-vivo antidiabetic study, we used Albino mice of either sex with an average weight of up to 30 gm. The animals were kept in an animal house as per the standard procedure of the animals' Bye-Laws and under the approval of Ethical Committee, Department of Pharmacy, Faculty of Biological Sciences, University of Malakand, Pakistan as per the animals Bye-Laws 2008 (Scientific Procedure Issue-I).
Acute Toxicity
The mice were divided into two groups (i.e., test group and control group, having five animals in each of the group). The samples were administered I.P in a concentration ranges of 250–2,000 mg/kg body weight. After the samples' administrations, the animals were critically observed for 3 days for any abnormal response or mortality.
Induction of Diabetes
Initially, we administered alloxan monohydrate (10%, 150 mg/kg) intraperitoneally to the experimental animals for the induction of hyperglycemia. Meanwhile, a control group was also maintained by experimental animals which received an intraperitoneal dose of normal saline. After the alloxan monohydrate injection, the animals were sorted out into diabetic and non-diabetic animals by using a glucometer. The animals with high blood glucose level (diabetes) were subjected to further studies as per the previous reported procedure (Hussain et al., 2019).
In-vivo Experiment
In the in-vivo experiment, the diabetic mice were divided into groups I-IV (having 5 mice in each). The group I was the normal one given normal saline and having no diabetes. Group II was given Tween 80 solution and was the diabetic group. Group III was given a standard drug for the treatment of diabetes (i.e., on 500 μg/kg body weight of glibenclamide). Group IV was given different concentrations of the potent chloroform fraction (Hussain et al., 2019).
Gas Chromatography (GC) Analysis
Chloroform fraction was analyzed by means of an Agilent USB-393752 gas chromatograph (Agilent Technologies, Palo Alto, CA, USA) with HHP-5MS 5% phenylmethylsiloxane capillary column (30 m × 0.25 mm × 0.25 μm film thickness; Restek, Bellefonte, PA) equipped with an FID detector. The temperature of the oven was retained at 70°C for 1 min at first, and then raised at the rate of 6°C/min to 180°C for 5 min and to finish at the rate of 5°C/ min to 280°C for 20 min. Injector and detector temperatures were set at 220 and 290°C, correspondingly. Helium was utilized as carrier gas at a flow rate of 1 ml/min (Mahmood et al., 2019).
Gas Chromatography–Mass Spectrometry (GC/MS) Analysis
GC/MS analysis of the chloroform fraction was processed by means of an Agilent USB-393752 gas chromatograph (Agilent Technologies, Palo Alto, CA, USA) with a HHP-5MS 5% phenylmethylsiloxane capillary column (30 m × 0.25 mm × 0.25 μm film thickness; Restek, Bellefonte, PA) prepared with an Agilent HP-5973 mass selective detector in the electron impact mode (Ionization energy: 70 eV) working under a similar experimental environment as illustrated for GC (Shah et al., 2012).
Identification of Components
Compounds present in chloroform fraction of E. caeruleum were identified by comparison of the retention times of each of them with those of authentic compounds in the literature. Additional identification was performed from the spectral data obtained from the Wiley and NIST libraries and further identifications were completed by comparisons of the fragmentation pattern of the mass spectra with the published data in the literature (Zeb et al., 2017).
HPLC-DAD Analysis
Preparation of Samples for HPLC
For the preparation of samples, 20 ml of methanol and water (1:1 v/v) were mixed with 1 gram of E. caeruleum samples. This mixture was then heated on a water bath at 70°C for 1 h. After cooling, the sample was centrifuged at 4,000 rpm for 10 min. Then 2 ml of sample was filtered with Whatman filter paper into HPLC vials.
HPLC –DAD Procedure
The determination of various compounds' peaks was carried out by means of an Agilent 1260 infinity High-performance liquid chromatography (HPLC) system, having basic parts like quaternary pump, auto-sampler, degasser and ultraviolet array detector (UVAD). The separation was achieved via Agilent Zorbax Eclipse XDB-C18 column. The gradients system comprises of solvent B (methanol: acetic acid; deionized water, 100: 20: 180, v/v) and solvent C (methanol : acetic acid : deionized water, 900: 20: 80, v/v). The efficient gradient program was started with 100% B at 0 min, 85% B at 5 min, 50% B at 20 min, 30% B at 25 min, and 100% C from 30 to 40 min (Zeb et al., 2017). After 25 min, elution occurred. For the analysis of antioxidants compounds, the ultraviolet array detector (UVAD) was set to 280 nm and the spectra were recorded from 190 to 500 nm.
Estimation of IC50 Values
For α-glucosidase results, IC50 values were calculated from a dose response curve using Microsoft Excel program. Furthermore, in anti-radicals assays including DPPH, ABTS and H2O2 the IC50 were calculated following the same protocol.
Statistical Data Analysis
All experiments were performed in triplicate and results were expressed as mean ± S.E.M of percent inhibition values. One-way ANOVA followed by Dunnett's multiple comparison test was applied for the comparison of positive control with the test group using GraphPad prism Software USA. The P < 0.05 were considered as statistically significant (Shah et al., 2014b).
Docking Studies
Molecular docking studies were made by Molecular-Operating Environment (MOE-2016.08) (Munir et al., 2020). Docking study for α-glucosidase enzyme was performed on our previously reported homology modeled α-glucosidase (Ali et al., 2018). Preparation of ligand's downloaded enzymes such as determination of binding sites, energy minimization and 3-D protonation was performed by previously reported methods (Iftikhar et al., 2018; Ullah et al., 2018; Tanoli et al., 2019). Docking results were interpreted, and their surfaces were analyzed with graphical representation utilizing discovery-studio visualizer (Biovia, 2017).
Results
α-Glucosidase Inhibition Assay
In α-glucosidase inhibition assay, Ec. Chf was observed to be the most active fraction. Ec. Chf exhibited 59.57 ± 1.18, 52.67 ± 0.11, 43.86 ± 0.02, and 36.72 ± 0.45% enzyme at concentrations of 1,000, 500, 250, and 125 μg/ml respectively attaining IC50 of 734 μg/ml. Likewise, Ec. Cr displayed 55.46 ± 0.44, 39.51 ± 1.11, 33.77 ± 1.14, and 27.72 ± 1.45% inhibitions at the same tested concentrations with an IC50 value of 855 μg/ml, respectively. In comparison, the standard drug acarbose showed 76.87 ± 0.06, 73.94 ± 1.92, 67.49 ± 0.22, and 61.53 ± 0.89% inhibitions at concentrations of 1,000, 500, 250, and 125 μg/ml respectively with IC50 of 25 μg/ml. Other fractions, including Ec. EtAc, Ec. Hex, and Ec. Aq exhibited dose-dependent enzyme inhibition with IC50 of 754, 1,388, and 1,208 μg/ml respectively as shown in Table 1.
Antioxidant Assays
The results obtained from the antioxidant activity of E. caeruleum using DPPH, ABTS and H2O2 free radicals assays are summarized in Table 2.
DPPH Free Radicals Scavenging Assay
In DPPH free radicals scavenging assay, Ec.Chf, Ec.Cr, and Ec.EtAc were the potent fractions. Ec.Chf showed 76.50 ± 0.57, 67.16 ± 0.60, 58.83 ± 0.88, and 52.50 ± 0.28% inhibitions of free radicals at concentrations of 1,000, 500, 250, and 125 μg/ml respectively. Similarly, Ec.Cr displayed 73.33 ± 0.60, 64.16 ± 0.44, 52.83 ± 0.72, and 44.50 ± 0.86% inhibitions at concentrations 1,000, 500, 250, and 125 μg/ml, respectively. Furthermore, Ec.EtAc enzyme inhibitions were 70.16 ± 0.72, 61.60 ± 0.49, 51.70 ± 0.47, and 42.20 ± 0.41% at the same tested concentrations. The IC50 values for Ec.Chf, Ec.Cr, and Ec.EtAc were 112, 163, and 204 μg/ml, respectively. Standard drug ascorbic acid showed 90.66 ± 0.70, 85.46 ± 0.39, 76.70 ± 0.40, and 69.06 ±0.58% scavenging of free radicals at concentrations 1,000, 500, 250, and 125 μg/ml respectively. The IC50 value calculated for ascorbic acid was 10 μg/ml. Ec.Hex and Ec.Aq also displayed a dose dependent response with IC50 of 405 and 580 μg/ml, respectively as shown in Table 2.
ABTS Free Radicals Scavenging Assay
In ABTS anti-radicals assay, Ec.Chf displayed the highest activity causing 75.13 ± 0.69, 67.23 ± 0.61, 60.56 ± 0.42, and 54.06 ± 0.58% scavenging of free radicals at concentrations of 1,000, 500, 250, and 125 μg/ml, respectively. Ec.Cr was equally effective causing 72.30 ± 0.62, 64.13 ± 0.46, 53.26 ± 0.59, and 46.16 ± 0.60 μg/ml respectively at concentrations of 1,000, 500, 250, and 125 μg/ml, respectively. For Ec.Chf and Ec.Cr the IC50 values were 109 and 175 μg/ml respectively. Ec.EtAc, Ec.Hex, and Ec.Aq showed 69.10 ± 0.66, 66.16 ± 0.44, and 57.20 ± 0.41 at highest tested concentration attaining IC50 values of 200, 332, and 590 μg/ml, respectively. In scavenging of ABTS free radicals, the standard drug ascorbic acid exhibited 89.10 ± 0.49, 81.20 ± 0.41, 70.36 ± 0.57, and 63.26 ± 0.67% inhibitions respectively at the same concentrations with IC50 of 60 μg/ml (Table 2).
H2O2 Free Radicals Scavenging Assay
In hydrogen peroxide radicals scavenging assay, Ec.Chf and Ec.Cr exhibited the highest radicals scavenging activity. Ec.Chf showed 75.46 ± 0.97, 68.16 ± 0.44, 54.06 ± 0.52, and 46.10 ± 0.58% anti-radicals activity at concentrations of 1,000, 500, 250, and 125 μg/ml, respectively. For Ec.Cr, the observed scavenging efficiency was 75.16 ± 0.72, 66.06 ± 0.52, 55.10 ± 0.66, and 46.33 ± 0.52% at the same concentrations. The IC50 values for Ec.Chf, Ec.Cr were 150 and 145 μg/ml respectively. Among other fractions, Ec.Hex caused 66.13 ± 0.46, 56.13 ± 0.69, 47.23 ± 0.61, and 36.03 ± 0.60% inhibitions at concentrations of 1,000, 500, 250, and 125 μg/ml respectively. The ABTS free radicals scavenging efficacy of Ec. EtAc was mediocre with IC50 of 330 μg/ml. The aqueous fraction showed the least activity with IC50 value of 790 μg/ml. Standard drug ascorbic acid inhibitory activity was 85.13 ± 0.46, 74.13 ± 0.55, 65.46 ± 0.73, and 53.36 ± 0.63% at concentrations of 1,000, 500, 250, and 125 μg/ml respectively with IC50 of 85 μg/ml.
In-vivo Antidiabetic Studies
Based on the in-vitro assays of all fractions of Eryngium caeruleum, the chloroform fraction was selected for the in-vivo assay as can be seen in Table 3. In acute toxicity studies, the Ec.Chf was found to be safe, causing no mortality or unwanted reactions. The chloroform fraction of Eryngium caeruleum was observed to be a potent antidiabetic agent following the in-vivo experiments. The Ec.Chf at concentrations of 500, 250, 125, 62.5, and 31.25 μg/kg body weight exhibited a blood glucose level of 10.3, 15.4, 16.8, 17.8, and 19.4 mmol/liter in mice. Obviously, the blood glucose level at 24 h and 500 μg/kg was 10.3 mmol/liter which was almost in comparison to the standard drug value which was 7.9 mmol/liter.
Identification of phytoconstituents (GC-MS Analysis)
The GC-MS analysis of the most active fraction (i.e., the chloroform fraction was performed as described). A total number of 60 compounds were identified in the GC-MS analysis of chloroform fraction (Table 4). Various parameters provided for the major identified compounds are summarized in Table 5. The chromatogram of this fraction representing various peaks is shown in Figure 2. Out of these compounds, 15 were found to be in dominant concentrations (compounds A-O), which are summarized in Figure 3. Furthermore, we assessed overall GC-MS analysis of the chloroform fraction and found some interesting bioactive compounds which might be responsible for the proposed activities. These compounds include triacontane, tocopherol, 2,5-phyrrolidione, N-[2-(thienyl)acetyloxyl], methyl palmitate, cyclohexene, 3-(1,5-dimethyl-4-hexenyl)-6-methylene, phytol, neophytadiene, thymol, falcarinol, and linolenic acid as shown in Figure 4.
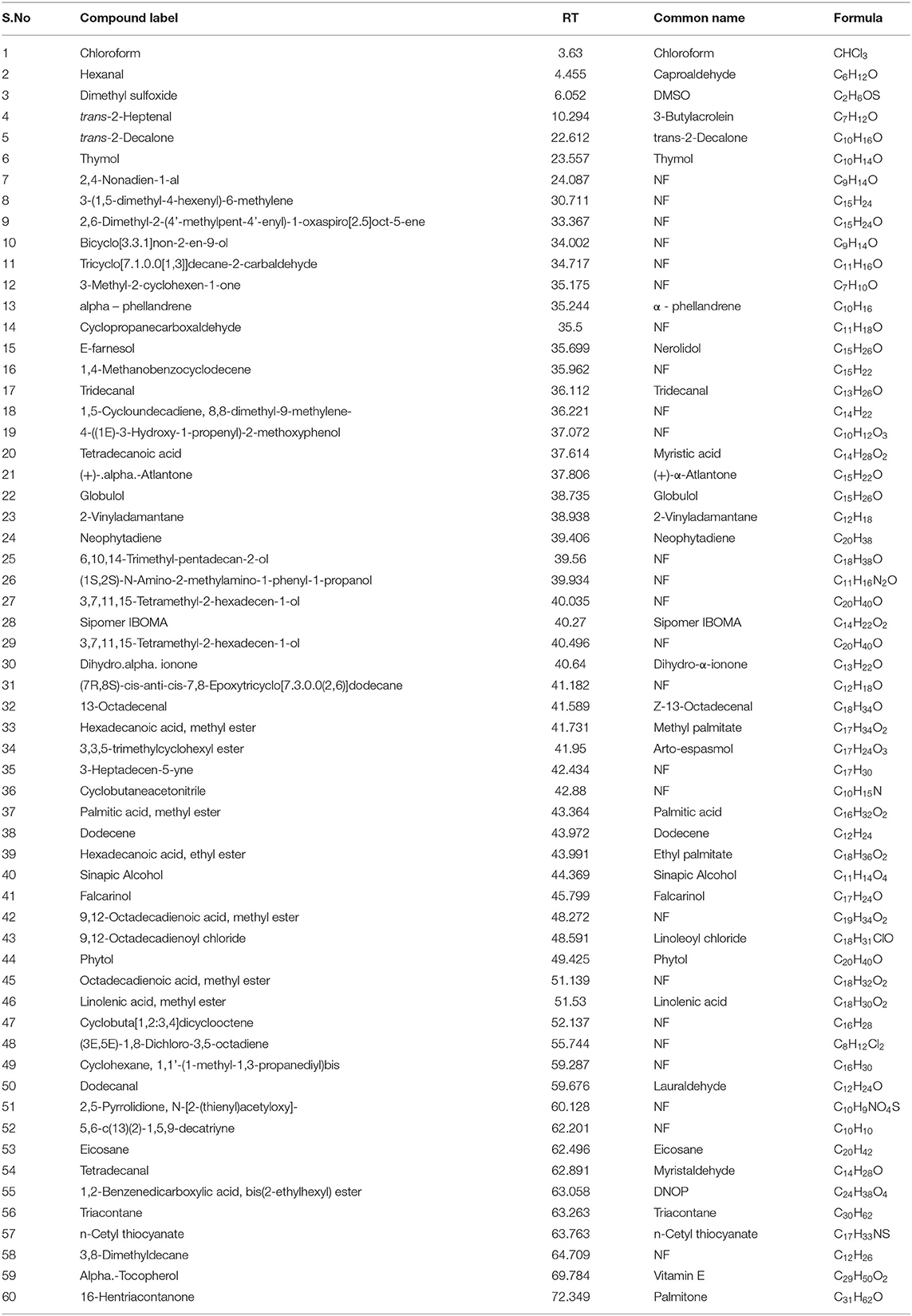
Table 4. Phytoconstituents identified by GC-MS analysis in the chloroform fraction of Eryngium caeruleum.
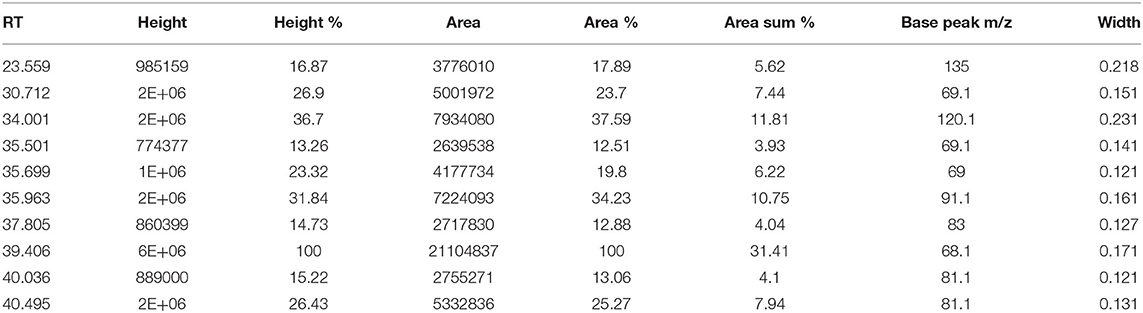
Table 5. Chromatogram peak list of the major identified compounds in chloroform fraction of Eryngium caeruleum.
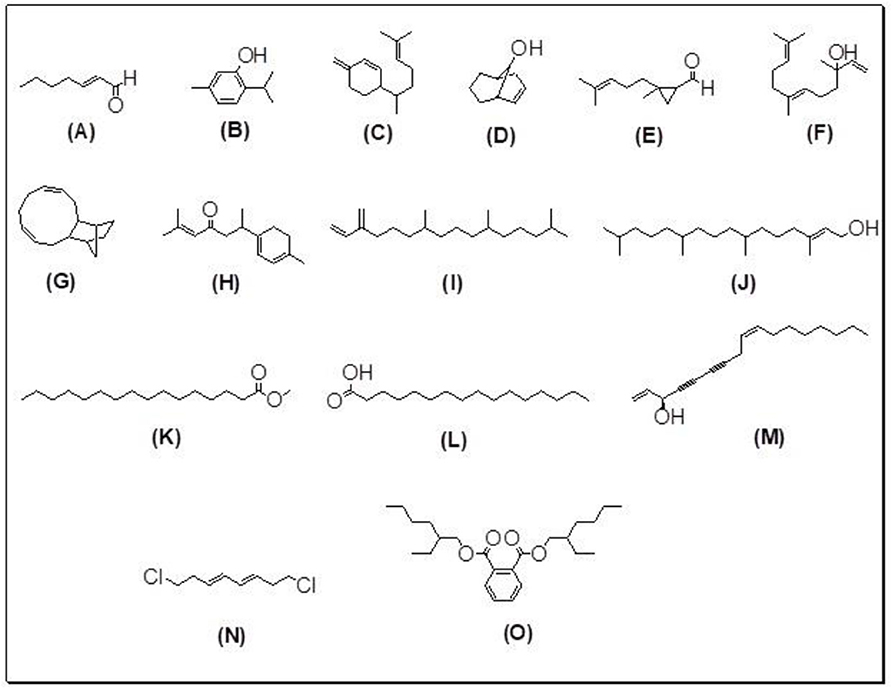
Figure 3. (A–O) Major phytoconstituents identified by GC-MS analysis in the chloroform fraction of Eryngium caeruleum.
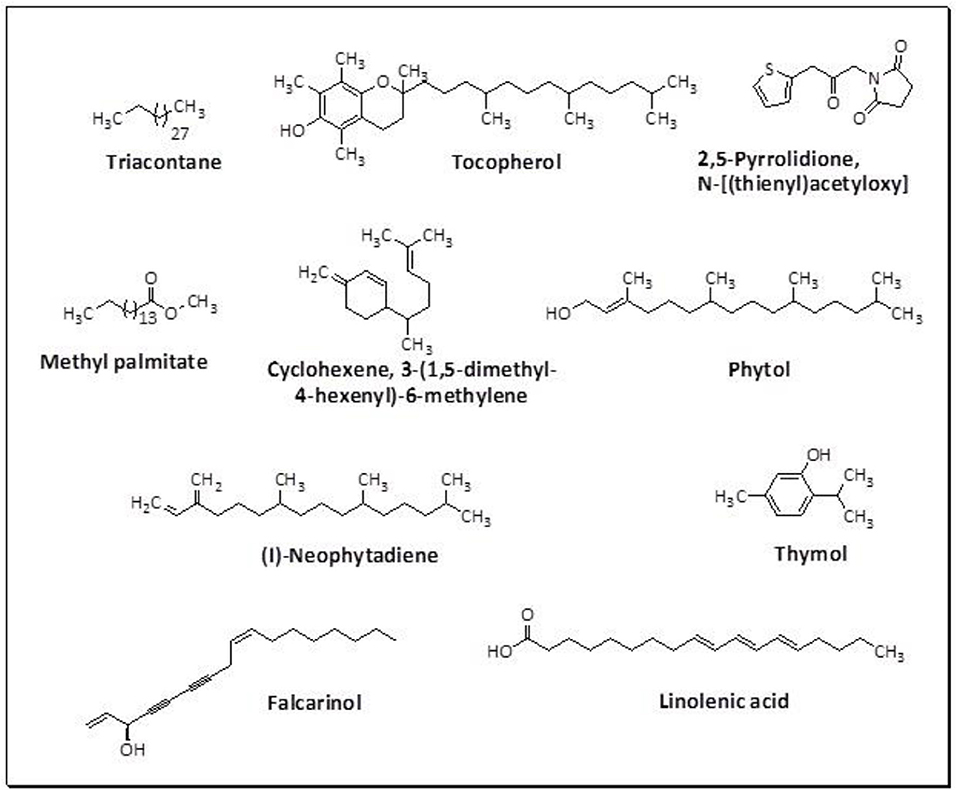
Figure 4. Structures of putative bioactive compounds identified in the chloroform fraction of Eryngium caeruleum.
Results of HPLC-DAD Analysis
The methanolic extract and chloroform fraction of E. caeruleum were analyzed via HPLC-DAD. The chromatograms obtained for methanolic extract and chloroform fraction has been represented in Figures 5, 6, respectively.
In the chromatogram of methanolic extract, various components are separated in different ratios. The ratios of some distinct peaks are summarized in Table 6 with their retention times. The highest concentrated signal was observed at a retention time of 22.96 min which was 22.78%. All the other signals especially after 10 min, were smaller. On the other hand, the HPLC spectrum of chloroform fraction was observed to be different with respect to the nature and ratios of components. After retention time 10, various peaks were observed in dominant ratios, which shows the possible solubility of these components in chloroform. The dominant peaks after a retention time of 10 min were observed at 14.06, 14.97, 15.89, 16.08, 18.83, 20.20, 21.91, 28.05, 34.18, 37.91, and 40.24 min. All the peaks with their retention times and ratios are shown in Table 7.
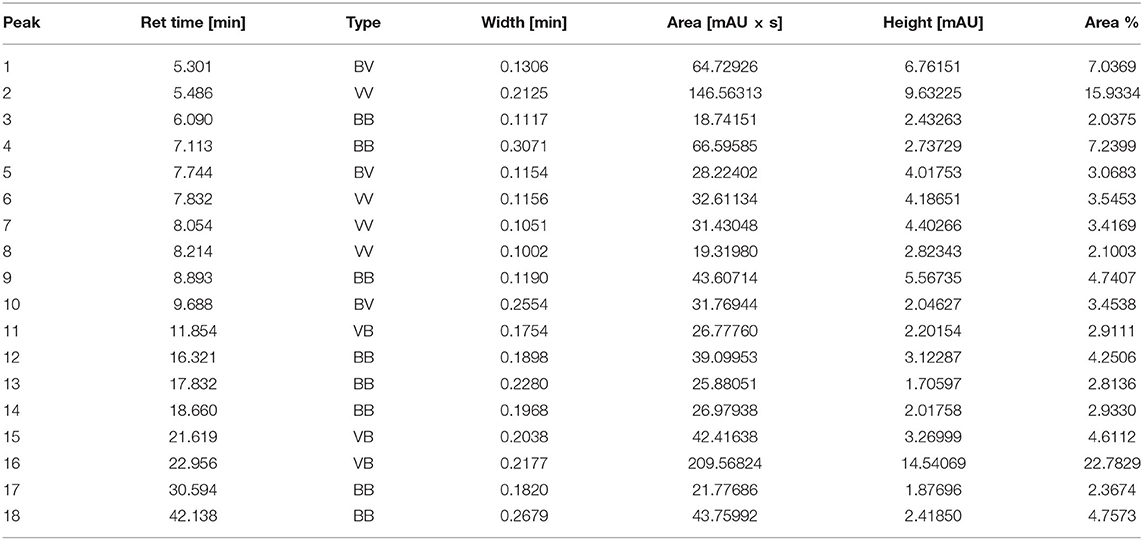
Table 6. Various parameters of peaks obtained in HPLC-DAD chromatogram in the methanolic extract of Eryngium caeruleum.
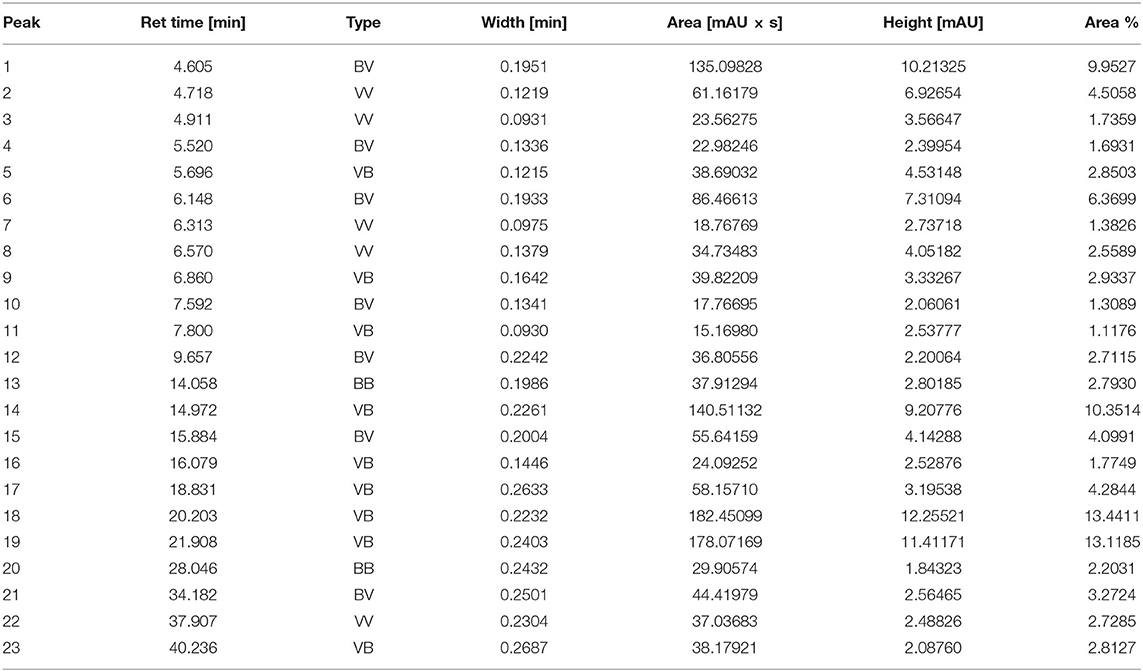
Table 7. Various parameters of peaks obtained in HPLC-DAD chromatogram in the chloroform fraction of Eryngium caeruleum.
Results of Docking Studies
Our research group has already explored the synergistic effects of constituents of different plant extracts against α-glucosidase via docking simulations (Nadeem et al., 2019, 2020a,b; Farooq et al., 2020). Hence, we also performed docking simulations in order to correlate the in-vitro results and to explore the possible role of putative bioactive compounds identified in the chloroform fraction of Eryngium caeruleum. A Molecular Operating Environment (MOE) software package was used for this purpose. These putative bioactive compounds identified in the chloroform fraction of Eryngium caeruleum were tested in-vitro against α-glucosidase from Baker's yeast. The 3-D structure of Saccharomyces cerevisiae (Baker's yeast) has not yet been reported. Hence, we performed docking simulations by using our previously reported Homology modeled α-glucosidase (Ali et al., 2018).
The 3-D binding orientations and interaction plots are shown in Figures 7, 8. While the binding energy data and list of interacting residues are shown in Table 8. Tocopherol, phytol, thymol, Falcarinol and linolenic acid form hydrogen bond interactions with the residues present in the catalytic triad (Asp214, Glu276, and Asp349). A number of active constituents' form π-π stacking with key amino acid residues; Phe157, Phe177, Phe300 (Table 8) (Nadeem et al., 2019, 2020a,b; Farooq et al., 2020). Tocopherol has shown the most stable ligand-enzyme complex with the binding energy −7.7008 kcal/mol. While, phytol and linolenic acid also showed the lowest binding energy (−7.0629 and −7.01746 kcal/mol, Table 8).
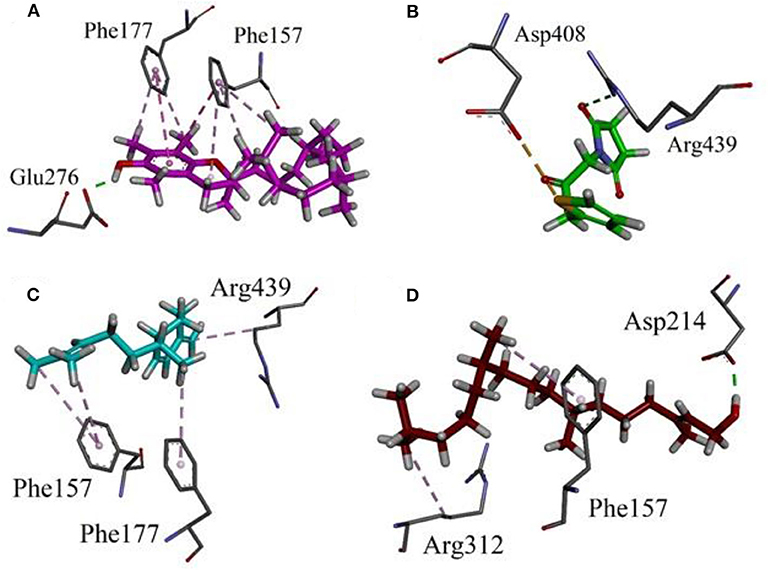
Figure 7. Binding orientation and 3-D interaction plot of putative bioactive compounds identified in the chloroform fraction of Eryngium caeruleum into active sites of homology model of α-glucosidase. (A) tocopherol (B) 2,5-Pyrrolidione, N-[(thienyl) acetyloxy] (C) Cyclohexene, 3-(1,5-dimethyl-4-hexenyl)-6-methylene, and (D) phytol.
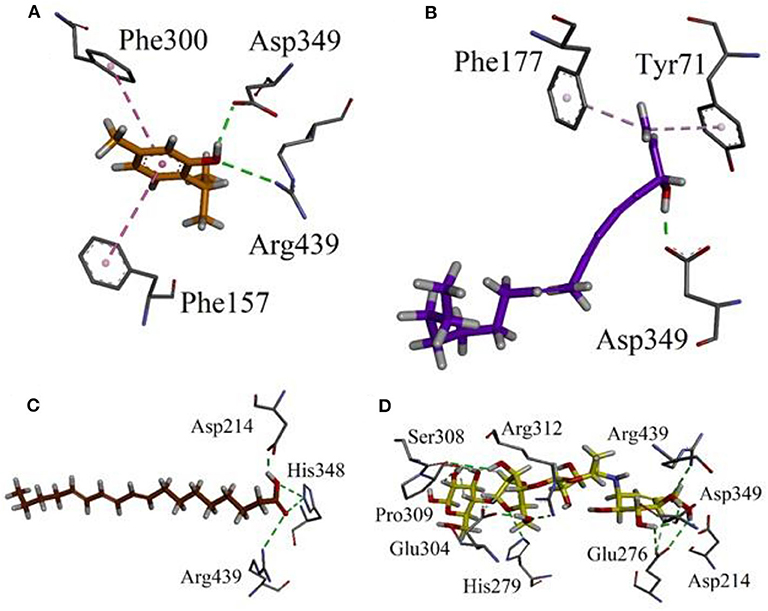
Figure 8. Binding orientation and 3-D interaction plot of putative bioactive compounds identified in the chloroform fraction of Eryngium caeruleum into active sites of homology model of α-glucosidase. (A) thymol (B) Falcarinol (C) linolenic acid and (D) standard drug acarbose.
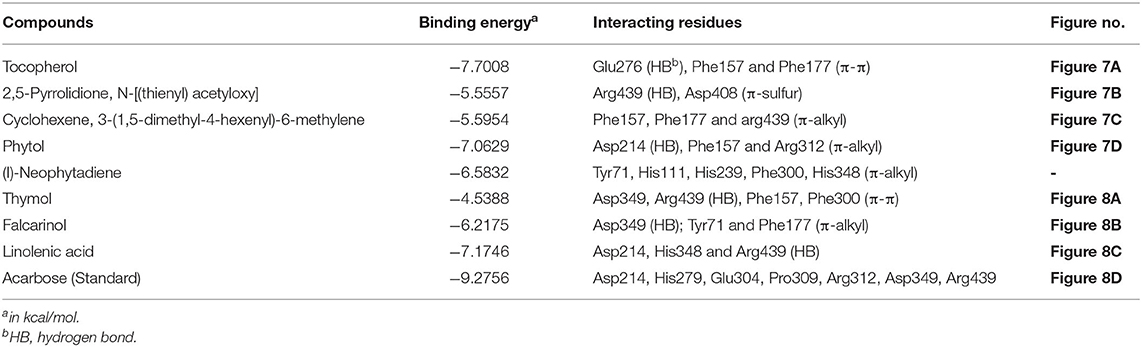
Table 8. Binding energy values and ligand interaction pattern revealed by possible isolated phytoconstituents and acarbose toward yeast α-glucosidase.
Subsequently, we docked major phytoconstituents identified by GC-MS analysis (Figure 3) into the homology model of α-glucosidase. The binding energy data of the docked compounds is shown in Table 9. The binding energy of these compounds were found in the range of −6.6118 to −4.0372 kcal/mol. Highest activity was shown by bis(2-ethylhexyl) phthalate (O in Figure 3).
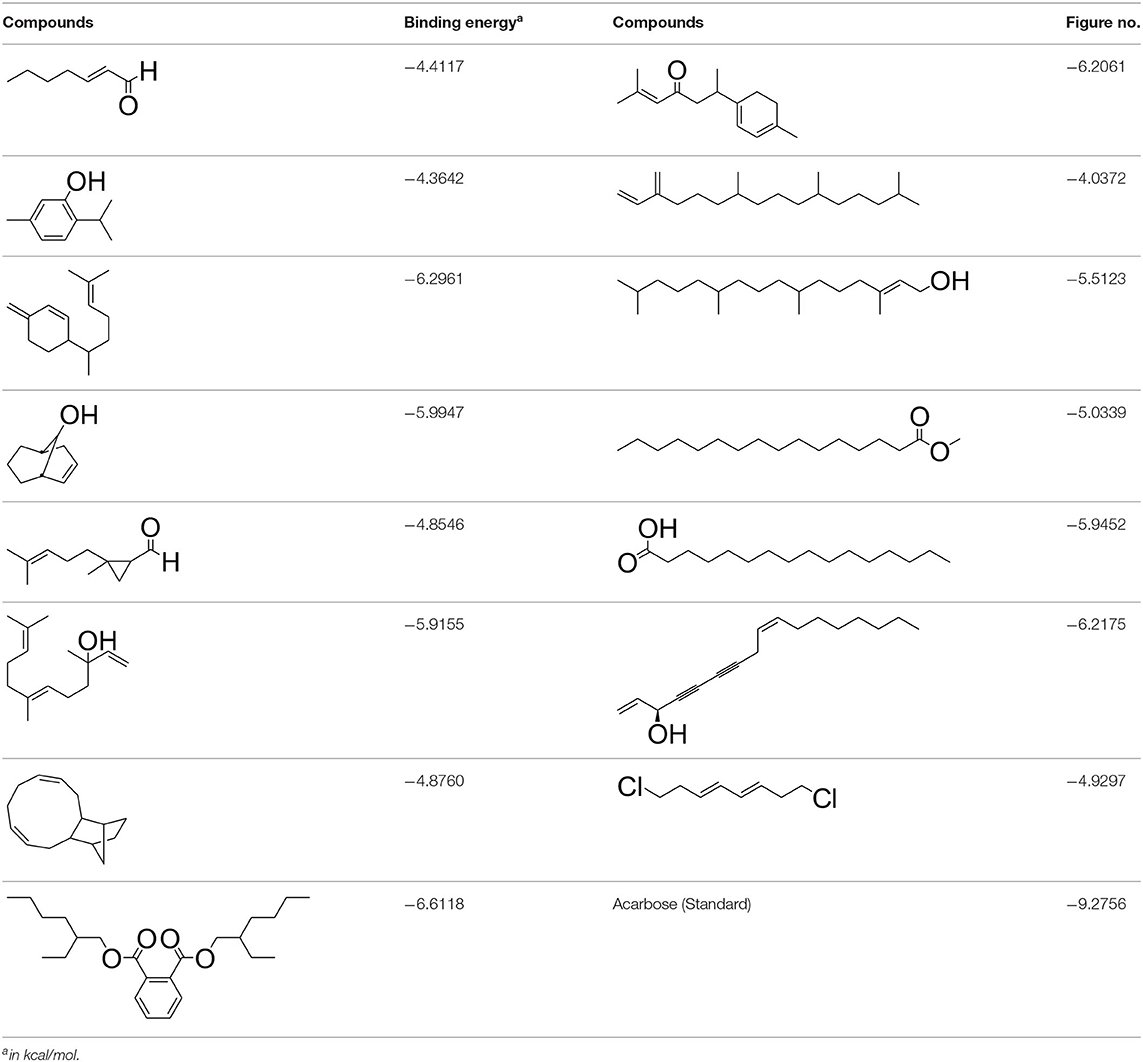
Table 9. Binding energy values and ligand interaction pattern revealed by possible isolated phytoconstituents and acarbose toward yeast α-glucosidase.
Discussion
Alpha-glucosidase inhibitors represent a group of antidiabetic drugs that regulate postprandial hyperglycemia through inhibition of carbohydrates digestion in the small intestine and thus hamper the diet associated with acute glucose excursion (Dwek et al., 2002). Among these drugs, acarbose and voglibose have got substantial attention in the past decades due to their effectiveness in controlling type 2 DM (Scheen, 2009). Acarbose is a natural product obtained from the fermentation of Actinoplanes species (Truscheit et al., 1981). Apart from presently available drugs, several herbal medicines have been recommended for the management DM. Traditional medicinal plants and plants-based remedies are used all over the world for a range of diseases, including DM (Aslam et al., 2018). The hypoglycaemic activity of medicinal plants including Euclea undulata var. myrtina, Schkuhria pinnata, Pteronia divaricata, and Elaeodendron transvaalense which are traditionally used against DM were scientifically proved (Deutschländer et al., 2009). Different solvent extracts from Calamintha origanifolia, Satureja thymbra, Prangosasperula, Sideritis perfoliata, Asperula glomerata, Hyssopus officinalis, Erythraea centaurium, Marrubium radiatum, and Salvia acetabulosa were recently reported to possess strong anti- glucosidase potentials (Loizzo et al., 2008). Likewise, the solvent extracts prepared from Azadirachta indica, Murraya koenigii, Ocimum tenuflorum, Syzygium cumini, Linum usitatissimum, and Bougainvillea spectabilis which are ethno-botanically known medicinal plants against DM was reported to possess anti-glucosidase potentials (Bhat et al., 2011).
In the current study, we screened various solvent extracts from Eryngium caeruleum against α-glucosidase enzyme and a number of free radicals both of which are important targets in the management of type 2 DM. Although the percent of inhibitions of crude extracts were low in comparison to the standard drug, the presence of active anti-glucosidase constituents were still observed. The Ec.Chf was observed to be more potent as compared to other fractions in all in-vitro assays. Similarly, the Ec.Chf was also found to be effective in in-vivo antidiabetic assay at various concentrations. The first extracted fraction was with n-hexane which probably showed less potency due to the presence of non-polar and inactive compounds. In comparison, the next two fractions (i.e., with chloroform and ethyl acetate were relatively effective). One of the reasons for their effectiveness might be the solubility of active polar organic compounds in it. The remaining aqueous fraction was the least active. A major reason was that the majority of the active polar compounds tend to be extracted with chloroform and ethyl acetate, leaving a very small ratio of remaining compounds in the last fraction. For the preliminary identification of active compounds GC followed by GC-MS analysis of the active fraction was performed. We observed that 15 compounds were in greater concentration. Apart from the GC-MS analysis, further fingerprinting of E. caeruleum has been performed with the help of HPLC-DAD. The GC-MS and HPLC-DAD reveal the importance of E. caeruleum in terms of natural compounds.
Experimental evidence implies the contribution of free radicals in the inception of DM and more significantly in the progress of diabetic complications (Zeb et al., 2014). Free radical scavengers are useful in the prevention of DM in experimental diabetic animal models (Sadiq et al., 2018). Unrelenting hyperglycemia in DM patients can lead to oxidative stress via auto-oxidation of glucose, non-enzymatic glycosylation and polyol pathways. Auto-oxidation of glucose results from the natural reduction of molecular oxygen species to superoxide and hydroxyl radicals which, being extremely reactive, reacts with all biomolecules (Sadiq et al., 2015b; Jabeen et al., 2018). These free radicals also speed up formation of advanced glycation end products like pyrroles and imidazoles. Cross-linking of these glycation end products with proteins in tissues fallout as irregularities in the function of cells and tissues. The third mechanism of free radical generation is Polyol pathway during which a greater amount NADPH is diminished which impair the formation of key antioxidants like glutathione (Giugliano et al., 1995). As a result, the protein glycation capability of antioxidant enzymes is also decreased. Long-lived structural proteins, collagen and elastin, experience persistent non-enzymatic cross-linking during aging, and in diabetic individuals (Vasan et al., 2003). Chronic oxidative stress also leads to insulin resistance, dyslipidemia, β-cell dysfunction, impaired glucose tolerance and type-2 DM. Prolonged oxidative stress, hyperglycemia and dyslipidemia are principally harmful for β-cells. The impairment of β-cell function leads to under production of insulin, weakened glucose-stimulated insulin secretion, fasting hyperglycemia, and ultimately the development of type-2 DM (Tangvarasittichai, 2015).
As Ec.Chf was the most active fraction in α-glucosidase inhibition and antioxidant assays, so it was subjected to in-vivo and GC-MS analysis for preliminary identification of bioactive compounds. The GC-MS resulted in the identification of 60 compounds. Out of these, 15 compounds were found to represent the major peaks of the spectrum. Several compounds identified in the GC-MS data were found active in terms of anti-diabetic and antioxidant potentials as evident from their reported literature. In anti-diabetic context, these include thymol, nerolidol, neophytadiene, falcarinol, linolenic acid, palmitic acid, pyrrolidione derivative etc. Briefly, the thymol a monoterpene isolated from Thymus vulgaris and reported to possess antioxidant and anti-diabetic potentials (Hyun et al., 2014). Nerolidol and neophytadiene are terpenes found in many plants, for example, it has been found recently in the extract of Achillea ligustica and in the extract was also assayed for its anti-radical and anti-diabetic potentials with excellent results (Conforti et al., 2005). Similarly, falcarinol, a known pesticide which is also found in some plant extracts along with anti-diabetic activity (An et al., 2014). In the same way, linolenic acid has also been reported to possess anti-diabetic potential (Kato et al., 2000). Palmitic acid has also been proven to be an excellent candidate in type-2 diabetes mellitus (Chae et al., 2010). Correspondingly, the pyrolidone derivative has been proven to possess strong α-glucosidase inhibitory and anti-inflammatory properties (Trapero and Llebaria, 2012; Jan et al., 2020). Moreover, several antioxidant compounds identified in the GC-MS spectrum have been reported to scavenge the free radicals effectively. Some of which include thymol, tocopherol, palmitate, phytol, triacontane, cyclohexene, 3-(1,5-dimethyl-4-hexenyl)-6-methylene etc. The thymol has been verified previously to possess strong antioxidant potential (Braga et al., 2006). The vitamin E is also an excellent antioxidant compound used in various diseased conditions (Müller et al., 2010). In the same way, ascorbyl palmitate has been reported to possess antioxidant properties (Hra et al., 2000). The antioxidant activity of phytol has been demonstrated by several investigators (Santos et al., 2013). Likewise, the 16-hydroxy-18-Triacontane and 4-hydroxy-triacontane-16,18-dione has been isolated as a natural antioxidant from Eucalyptus leaf (Osawa and Namiki, 1985). The cyclohexene, 3-(1,5-dimethyl-4-hexenyl)-6-methylene was previously identified in the Karungkuravai (a type of medicinal rice) and assayed for antioxidant activity with positive results (Krishnanunni et al., 2015). All the putative bioactive compounds identified in the Ec.Chf are shown in Figure 3. The identification of numerous bioactive compounds in the Ec.Chf and the current investigational studies shows the role of E. caeruleum in the management of oxidative stress and diabetes mellitus.
As discussed in the Introduction section, the isolated two new flavone glycosides reported by Rehman et al. were evaluated for their potential against α-glucosidase, aldose reductase (ALR1 and ALR2). Compounds were only found active against ALR1 and ALR2 and were not able to show α- β-glucosidase inhibition and antiglycation potential. Docking studies were performed only on aldose reductase targets. In the current study, we performed a docking experiment to justify the synergistic effect of all these isolated bioactive compounds. The data obtained from docking studies agrees with the α-glucosidase inhibition activity. The computed binding energy of standard drug acarbose is −9.2756 kcal/mol. Putative bioactive compounds showed binding energy values in the range of −7.7008 to −4.5388 kcal/mol. Among simulated constituents, tocopherol showed best binding energy (−7.7008 kcal/mol). While the binding energy from major phytoconstituents identified by GC-MS analysis were found in the range of −6.6118 to −4.0372 kcal/mol. The highest affinity was shown by bis(2-ethylhexyl) phthalate (O in Figure 3). The binding energy data showed in Tables 8, 9 revealed that all the compounds identified in the chloroform fraction of Eryngium caeruleum are responsible for the synergistic effect of hypoglycemia.
Conclusions
The sorting out of the most active solvent fraction of Eryngium caeruleum in the terms of antioxidant and anti-diabetic potentials followed by its GC-MS and HPLC-DAD analyses revealed that this plant is an excellent source of bioactive compounds. Based on the literature survey of identified compounds and ethnopharmacology of Eryngium caeruleum, it may be concluded that this plant is rich in antioxidant and anti-diabetic compounds and may be an effective remedy against various diseases, especially free radicals mediated and diabetic mediated disorders. Binding energy data computed via docking studies revealed that all putative bioactive compounds identified in the chloroform fraction of Eryngium caeruleum can inhibit α-glucosidase synergistically to treat hyperglycemia.
Data Availability Statement
The raw data supporting the conclusions of this article will be made available by the authors, without undue reservation.
Ethics Statement
The animal study was reviewed and approved by the Ethical Committee, Department of Pharmacy, Faculty of Biological Sciences, University of Malakand, Pakistan as per the animals Bye-Laws 2008 (Scientific Procedure Issue-I).
Author Contributions
SA, FU, and MA helped in in-vitro assays. IK helped in in-vivo experiment. MZ performed the HPLC analysis and helped in antioxidant assay. UR performed the molecular docking studies. MFA, RU, ON, Z-UI, and WA helped in GC-MS analysis and enzyme assay. AS supervised the whole project and drafted the manuscript for publication. All the authors have read the manuscript and approved for submission.
Conflict of Interest
The authors declare that the research was conducted in the absence of any commercial or financial relationships that could be construed as a potential conflict of interest.
Acknowledgments
The authors extend their appreciation to The Researchers Supporting Project number (RSP-2020/122) King Saud University, Riyadh, Saudi Arabia. We are also grateful to Dr. Nasrullah, Department of Botany, University of Malakand, Pakistan for the identification of plants. UR has purchased an MOE license under HEC NRPU-project (5291/Federal/NRPU/R&D/HEC/2016). MZ is thankful to the Higher Education Commission of Pakistan for providing funding for the HPLC under project number HEC-NRPU-20-2515. AS is thankful to the Higher Education Commission of Pakistan for providing funding for consumables under the project number 10562/KPK/NRPU/R&D/2017.
Supplementary Material
The Supplementary Material for this article can be found online at: https://www.frontiersin.org/articles/10.3389/fchem.2020.558641/full#supplementary-material
Supporting Information 1 and 2. The GC-MS analysis and fragmentation pattern of major and bioactive identified compounds.
References
Ahmad, A., Ullah, F., Sadiq, A., Ayaz, M., Rahim, H., Rashid, U., et al. (2019). Pharmacological evaluation of aldehydic-pyrrolidinedione against HCT-116, MDA-MB231, NIH/3T3, MCF-7 cancer cell lines, antioxidant and enzyme inhibition studies. Drug Des. Devel. Ther. 13:4185. doi: 10.2147/DDDT.S226080
Ali, M., Ali, S., Khan, M., Rashid, U., Ahmad, M., Khan, A., et al. (2018). Synthesis, biological activities, and molecular docking studies of 2-mercaptobenzimidazole based derivatives. Bioorg. Chem. 80, 472–479. doi: 10.1016/j.bioorg.2018.06.032
An, N. Y., Kim, J. E., Hwang, D., and Ryu, H. K. (2014). Anti-diabetic effects of aqueous and ethanol extract of Dendropanax morbifera Leveille in streptozotocin-induced diabetes model. J. Nutr. Health, 47, 394–402. doi: 10.4163/jnh.2014.47.6.394
Aslam, H., Khan, A. U., Naureen, H., Ali, F., Ullah, F., and Sadiq, A. (2018). Potential application of Conyza canadensis (L) Cronquist in the management of diabetes: in vitro and in vivo evaluation. Trop. J. Pharm. Res. 17, 1287–1293. doi: 10.4314/tjpr.v17i7.9
Begum, H. A., Asad, F., Sadiq, A., Mulk, S., and Ali, K. (2019). Antioxidant, antimicrobial activity and phytochemical analysis of the seeds extract of Cucumis sativus Linn. Pure Appl. Biol. 8, 433–441. doi: 10.19045/bspab.2018.700202
Bhat, M., Zinjarde, S. S., Bhargava, S. Y., Kumar, A. R., and Joshi, B. N. (2011). Antidiabetic Indian plants: a good source of potent amylase inhibitors. Evid. Based Complement. Altern. Med. 2011:40. doi: 10.1093/ecam/nen040
Bibi, A., Shah, T., Sadiq, A., Khalid, N., Ullah, F., and Iqbal, A. (2019). L-isoleucine-catalyzed michael synthesis of N-alkylsuccinimide derivatives and their antioxidant activity assessment. Russ. J. Org. Chem. 55, 1749–1754. doi: 10.1134/S1070428019110174
Bischoff, H. B. A. G. (1994). Pharmacology of alpha-glucosidase inhibition. Eur. J. Clin. Invest. 24, 3–10.
Bnouham, M., Ziyyat, A., Mekhfi, H., Tahri, A., and Legssyer, A. (2006). Medicinal plants with potential antidiabetic activity-a review of ten years of herbal medicine research (1990-2000). Int. J. Diabetes Metabol. 14:1. doi: 10.1159/000497588
Braga, P. C., Dal Sasso, M., Culici, M., Galastri, L., Marceca, M. T., and Guffanti, E. E. (2006). Antioxidant potential of thymol determined by chemiluminescence inhibition in human neutrophils and cell-free systems. Pharmacol. 76, 61–68. doi: 10.1159/000089719
Capetanos, C., Saroglou, V., Marin, P. D., Simić, A., and Skaltsa, H. D. (2007). Essential oil analysis of two endemic Eryngium species from Serbia. J. Serb. Chem. Soc. 72, 961–965. doi: 10.2298/JSC0710961C
Carroll, M. F., Gutierrez, A., Castro, M., Tsewang, D., and Schade, D. S. (2003). Targeting postprandial hyperglycemia: a comparative study of insulinotropic agents in type 2 diabetes. J. Clin. Endocrinol. Metab. 88, 5248–5254. doi: 10.1210/jc.2003-030649
Chae, S. Y., Choi, Y. G., Son, S., Jung, S. Y., Lee, D. S., and Lee, K. C. (2010). The fatty acid conjugated exendin-4 analogs for type 2 antidiabetic therapeutics. J. Cont. Release 144, 10–16. doi: 10.1016/j.jconrel.2010.01.024
Conforti, F., Loizzo, M. R., Statti, G. A., and Menichini, F. (2005). Comparative radical scavenging and antidiabetic activities of methanolic extract and fractions from Achillea ligustica A LL. Biol. Pharm. Bull. 28, 1791–1794. doi: 10.1248/bpb.28.1791
Deutschländer, M. S., Van de Venter, M., Roux, S., Louw, J., and Lall, N. (2009). Hypoglycaemic activity of four plant extracts traditionally used in South Africa for diabetes. J. Ethnopharmacol. 124, 619–624. doi: 10.1016/j.jep.2009.04.052
Dwek, R. A., Butters, T. D., Platt, F. M., and Zitzmann, N. (2002). Targeting glycosylation as a therapeutic approach. Nat. Rev. Drug discov. 1, 65–75. doi: 10.1038/nrd708
Farooq, M. U., Mumtaz, M. W., Mukhtar, H., Rashid, U., Akhtar, M. T., Raza, S. A., et al. (2020). UHPLC-QTOF-MS/MS based phytochemical characterization and anti-hyperglycemic prospective of hydro-ethanolic leaf extract of Butea monosperma. Sci. Rep. 10, 1–14. doi: 10.1038/s41598-020-60076-5
Farooq, U., Naz, S., Shams, A., Raza, Y., Ahmed, A., Rashid, U., et al. (2019). Isolation of dihydrobenzofuran derivatives from ethnomedicinal species Polygonum barbatum as anticancer compounds. Biol. Res, 52:1. doi: 10.1186/s40659-018-0209-0
Fonseca, V. (2003). Clinical significance of targeting postprandial and fasting hyperglycemia in managing type 2 diabetes mellitus. Curr. Med. Res. Opin. 19, 635–631. doi: 10.1185/030079903125002351
Giugliano, D., Ceriello, A., and Paolisso, G. (1995). Diabetes mellitus, hypertension, and cardiovascular disease: which role for oxidative stress?. Metabolism 44, 363–368. doi: 10.1016/0026-0495(95)90167-1
Grover, J. K., Yadav, S., and Vats, V. (2002). Medicinal plants of India with anti-diabetic potential. J. Ethnopharmacol, 81, 81–100. doi: 10.1016/S0378-8741(02)00059-4
Houstis, N., Rosen, E. D., and Lander, E. S. (2006). Reactive oxygen species have a causal role in multiple forms of insulin resistance. Nature 440, 944–948. doi: 10.1038/nature04634
Hraš, A. R., Hadolin, M., Knez, Ž., and Bauman, D. (2000). Comparison of antioxidative and synergistic effects of rosemary extract with α-tocopherol, ascorbyl palmitate and citric acid in sunflower oil. Food Chem. 71, 229–233. doi: 10.1016/S0308-8146(00)00161-8
Hunt, J. V., Dean, R. T., and Wolff, S. P. (1988). Hydroxyl radical production and autoxidative glycosylation. Glucose autoxidation as the cause of protein damage in the experimental glycation model of diabetes mellitus and ageing. Biochem. J. 256, 205–212. doi: 10.1042/bj2560205
Hussain, F., Khan, Z., Jan, M. S., Ahmad, S., Ahmad, A., Rashid, U., et al. (2019). Synthesis, in-vitro α-glucosidase inhibition, antioxidant, in-vivo antidiabetic and molecular docking studies of pyrrolidine-2, 5-dione and thiazolidine-2, 4-dione derivatives. Bioorg. Chem. 91:103128. doi: 10.1016/j.bioorg.2019.103128
Hyun, T. K., Kim, H. C., and Kim, J. S. (2014). Antioxidant and antidiabetic activity of Thymus quinquecostatus Celak. Ind. Crops Prod. 52, 611–616. doi: 10.1016/j.indcrop.2013.11.039
Iftikhar, F., Yaqoob, F., Tabassum, N., Jan, M. S., Sadiq, A., Tahir, S., et al. (2018). Design, synthesis, in-vitro thymidine phosphorylase inhibition, in-vivo antiangiogenic and in-silico studies of C-6 substituted dihydropyrimidines. Bioorg. Chem. 80, 99–111. doi: 10.1016/j.bioorg.2018.05.026
Jabeen, M., Ahmad, S., Shahid, K., Sadiq, A., and Rashid, U. (2018). Ursolic acid hydrazide based organometallic complexes: synthesis, characterization, antibacterial, antioxidant, and docking studies. Front. Chem. 6:55. doi: 10.3389/fchem.2018.00055
Jan, M. S., Ahmad, S., Hussain, F., Ahmad, A., Mahmood, F., Rashid, U., et al. (2020). Design, synthesis, in-vitro, in-vivo, and in-silico studies of pyrrolidine-2, 5-dione derivatives as multitarget anti-inflammatory agents. Eur. J. Med. Chem. 186:111863. doi: 10.1016/j.ejmech.2019.111863
Kato, M., Miura, T., Nakao, M., Iwamoto, N., Ishida, T., and Tanigawa, K. (2000). Effect of alpha-linolenic acid on blood glucose, insulin and GLUT4 protein content of type 2 diabetic mice. J. Health Sci. 46, 489–492. doi: 10.1248/jhs.46.489
Kim, Y. M., Jeong, Y. K., Wang, M. H., Lee, W. Y., and Rhee, H. I. (2005). Inhibitory effect of pine extract on α-glucosidase activity and postprandial hyperglycemia. Nutrition 21, 756–761. doi: 10.1016/j.nut.2004.10.014
Kimura, A., Lee, J. H., Lee, I. S., Lee, H. S., Park, K. H., Chiba, S., et al. (2004). Two potent competitive inhibitors discriminating α-glucosidase family I from family II. Carbohydr. Res. 339, 1035–1040. doi: 10.1016/j.carres.2003.10.035
Krishnanunni, K., Senthilvel, P., Ramaiah, S., and Anbarasu, A. (2015). Study of chemical composition and volatile compounds along with in-vitro assay of antioxidant activity of two medicinal rice varieties: Karungkuravai and Mappilai samba. J. Food Sci. Technol. 52, 2572–2584. doi: 10.1007/s13197-014-1292-z
Loizzo, M. R., Saab, A. M., Tundis, R., Menichini, F., Bonesi, M., Piccolo, V., et al. (2008). In vitro inhibitory activities of plants used in Lebanon traditional medicine against angiotensin converting enzyme (ACE) and digestive enzymes related to diabetes. J. Ethnopharmacol. 119, 109–116. doi: 10.1016/j.jep.2008.06.003
Mahmood, F., Ali, R., Jan, M. S., Chishti, K. A., Ahmad, S., Zeb, A., et al. (2019). Chemical characterization and analgesic potential of Notholirion thomsonianum extract. Lat. Am. J. Pharm. 38, 807–812.
Montonen, J. (2005). Plant Foods in the Prevention of Type 2 Diabetes mellitus With Emphasis on Dietary fiber and Antioxidant Vitamins.
Müller, L., Theile, K., and Böhm, V. (2010). In vitro antioxidant activity of tocopherols and tocotrienols and comparison of vitamin E concentration and lipophilic antioxidant capacity in human plasma. Mol. Nutr. Food Res. 54, 731–742. doi: 10.1002/mnfr.200900399
Munir, A., Khushal, A., Saeed, K., Sadiq, A., Ullah, R., Ali, G., et al. (2020). Synthesis, in-vitro, in-vivo anti-inflammatory activities and molecular docking studies of acyl and salicylic acid hydrazide derivatives. Bioorg. Chem. 104:104168. doi: 10.1016/j.bioorg.2020.104168
Nadeem, M., Mumtaz, M. W., Danish, M., Rashid, U., Mukhtar, H., Anwar, F., et al. (2019). Calotropis procera: UHPLC-QTOF-MS/MS based profiling of bioactives, antioxidant and anti-diabetic potential of leaf extracts and an insight into molecular docking. J. Food Meas. Charact. 13, 3206–3220. doi: 10.1007/s11694-019-00243-z
Nadeem, M., Mumtaz, M. W., Danish, M., Rashid, U., Mukhtar, H., and Irfan, A. (2020a). Antidiabetic functionality of Vitex negundo L. leaves based on UHPLC-QTOF-MS/MS based bioactives profiling and molecular docking insights. Ind. Crops Prod. 152:112445. doi: 10.1016/j.indcrop.2020.112445
Nadeem, M., Mumtaz, M. W., Danish, M., Rashid, U., Mukhtar, H., Irfan, A., et al. (2020b). UHPLC-QTOF-MS/MS metabolites profiling and antioxidant/antidiabetic attributes of Cuscuta reflexa grown on Casearia tomentosa: exploring phytochemicals role via molecular docking. Int. J. Food Prop. 23, 918–940. doi: 10.1080/10942912.2020.1764578
Nichols, B. L., Avery, S. E., Karnsakul, W., Jahoor, F., Sen, P., Swallow, D. M., et al. (2002). Congenital maltase-glucoamylase deficiency associated with lactase and sucrase deficiencies. J. Ped. Gastroenterol. Nutr. 35, 573–579. doi: 10.1097/00005176-200210000-00022
Osawa, T., and Namiki, M. (1985). Natural antioxidants isolated from Eucalyptus leaf waxes. J. Agr. Food Chem. 33, 777–780. doi: 10.1021/jf00065a001
Rahim, H., Sadiq, A., Khan, S., Amin, F., Ullah, R., Shahat, A. A., et al. (2019). Fabrication and characterization of glimepiride nanosuspension by ultrasonication-assisted precipitation for improvement of oral bioavailability and in vitro α-glucosidase inhibition. Int. J. Nanomed. 14:6287. doi: 10.2147/IJN.S210548
Rehman, A. U., Hashmi, M. A., Tehseen, Y., Khan, A., Khan, S. S., Iqbal, J., et al. (2017). Antidiabetic flavonol glycosides from Eryngium caeruleum. Rec. Nat. Prod. 11:15.
Sadiq, A., Ahmad, S., Ali, R., Ahmad, F., Ahmad, S., Zeb, A., et al. (2016). Antibacterial and antifungal potentials of the solvents extracts from Eryngium caeruleum, Notholirion thomsonianum, and Allium consanguineum. BMC Complement. Altern. Med. 16:478. doi: 10.1186/s12906-016-1465-6
Sadiq, A., Khan, S., and Shah, S. M. H. (2015a). Larvicidal, insecticidal, brine shrimp cytotoxicity and anti-oxidant activities of Diospyros kaki (L.) reported from Pakistan. Pak. J. Pharm. Sci. 28, 1239–1243.
Sadiq, A., Mahmood, F., Ullah, F., Ayaz, M., Ahmad, S., Haq, F. U., et al. (2015b). Synthesis, anticholinesterase and antioxidant potentials of ketoesters derivatives of succinimides: a possible role in the management of Alzheimer's. Chem. Cent. J. 9:31. doi: 10.1186/s13065-015-0107-2
Sadiq, A., Zeb, A., Ahmad, S., Ullah, F., Ayaz, M., Ullah, F., et al. (2019). Evaluation of crude saponins, methanolic extract and subsequent fractions from Isodon rugosus Wall. ex Benth: Potentials of anti-angiogenesis in egg and anti-tumorigenesis in potato. Pak. J. Pharm. Sci. 32:1971–7.
Sadiq, A., Zeb, A., Ullah, F., Ahmad, S., Ayaz, M., Rashid, U., et al. (2018). Chemical characterization, analgesic, antioxidant, and anticholinesterase potentials of essential oils from Isodon rugosus Wall. ex. Benth. Front. Pharmacol. 9:623. doi: 10.3389/fphar.2018.00623
Saeedi, M., and Morteza-Semnani, K. (2008). Effect of the essential oil of Eryngium caeruleum on percutaneous absorption of piroxicam through rat skin. J. Essent. Oil Bearing Plants, 11, 485–495. doi: 10.1080/0972060X.2008.10643657
Santos, C. C. D. M. P., Salvadori, M. S., Mota, V. G., Costa, L. M., de Almeida, A. A. C., de Oliveira, G. A. L., et al. (2013). Antinociceptive and antioxidant activities of phytol in vivo and in vitro models. Neurosci. J. 2013:949452. doi: 10.1155/2013/949452
Scheen, A. (2009). Voglibose for prevention of type 2 diabetes mellitus. Lancet 373, 1579–1580. doi: 10.1016/S0140-6736(09)60575-4
Shah, S., Shah, S. M. M., Ahmad, Z., Yaseen, M., Shah, R., Sadiq, A., et al. (2015). Phytochemicals, in vitro antioxidant, total phenolic contents and phytotoxic activity of Cornus macrophylla Wall bark collected from the North-West of Pakistan. Pak. J. Pharm. Sci. 28, 23–28.
Shah, S. M. M., Sadiq, A., Shah, S. M. H., and Khan, S. (2014a). Extraction of saponins and toxicological profile of Teucrium stocksianum boiss extracts collected from District Swat, Pakistan. Biol. Res. 47, 1–5. doi: 10.1186/0717-6287-47-65
Shah, S. M. M., Sadiq, A., Shah, S. M. H., and Ullah, F. (2014b). Antioxidant, total phenolic contents and antinociceptive potential of Teucrium stocksianum methanolic extract in different animal models. BMC Complement. Altern. Med. 14:181. doi: 10.1186/1472-6882-14-181
Shah, S. M. M., Ullah, F., Shah, S. M. H., Zahoor, M., and Sadiq, A. (2012). Analysis of chemical constituents and antinociceptive potential of essential oil of Teucrium stocksianum bioss collected from the North West of Pakistan. BMC Complement. Altern. Med. 12:244. doi: 10.1186/1472-6882-12-244
Singh, V. K., Govil, J. N., and Singh, G. (2002). Recent progress in medicinal plants. Vol 1: Ethnomedicine and pharmacognosy. Recent Prog. Med. Plants.
Tangvarasittichai, S. (2015). Oxidative stress, insulin resistance, dyslipidemia and type 2 diabetes mellitus. World J. Diabetes 6:456. doi: 10.4239/wjd.v6.i3.456
Tanoli, S. T., Ramzan, M., Hassan, A., Sadiq, A., Jan, M. S., Khan, F. A., et al. (2019). Design, synthesis and bioevaluation of tricyclic fused ring system as dual binding site acetylcholinesterase inhibitors. Bioorg, Chem. 83, 336–347. doi: 10.1016/j.bioorg.2018.10.035
Trapero, A., and Llebaria, A. (2012). A prospect for pyrrolidine iminosugars as antidiabetic α-glucosidase inhibitors. J. Med. Chem. 55, 10345–6. doi: 10.1021/jm301682r
Truscheit, E., Frommer, W., Junge, B., Müller, L., Schmidt, D. D., and Wingender, W. (1981). Chemistry and biochemistry of microbial α-glucosidase inhibitors. Angew. Chem. Int. Ed. 20, 744–761. doi: 10.1002/anie.198107441
Ullah, A., Iftikhar, F., Arfan, M., Kazmi, S. T. B., Anjum, M. N., Haq, I. U., et al. (2018). Amino acid conjugated antimicrobial drugs: synthesis, lipophilicity-activity relationship, antibacterial and urease inhibition activity. Eur. J. Med. Chem. 145, 140–153. doi: 10.1016/j.ejmech.2017.12.089
van de Laar, F. A. (2008). Alpha-glucosidase inhibitors in the early treatment of type 2 diabetes. Vasc. Health. Risk. Manag. 4:1189. doi: 10.2147/VHRM.S3119
Vasan, S., Foiles, P., and Founds, H. (2003). Therapeutic potential of breakers of advanced glycation end product–protein crosslinks. Arch. Biochem. Biophys. 419, 89–96. doi: 10.1016/j.abb.2003.08.016
Zafar, R., Ullah, H., Zahoor, M., and Sadiq, A. (2019). Isolation of bioactive compounds from Bergenia ciliata (haw.) Sternb rhizome and their antioxidant and anticholinesterase activities. BMC Complement. Altern. Med. 19:296. doi: 10.1186/s12906-019-2679-1
Zafar, R., Zubair, M., Ali, S., Shahid, K., Waseem, W., Naureen, H., et al. (2020). Zinc metal carboxylates as potential anti-Alzheimer's candidate: in vitro anticholinesterase, antioxidant and molecular docking studies. J. Biomol. Struct. Dyn. 1–11. doi: 10.1080/07391102.2020.1724569
Zahoor, M., Shafiq, S., Ullah, H., Sadiq, A., and Ullah, F. (2018). Isolation of quercetin and mandelic acid from Aesculus indica fruit and their biological activities. BMC Biochem. 19:5. doi: 10.1186/s12858-018-0095-7
Zeb, A., Sadiq, A., Ullah, F., Ahmad, S., and Ayaz, M. (2014). Investigations of anticholinestrase and antioxidant potentials of methanolic extract, subsequent fractions, crude saponins and flavonoids isolated from Isodon rugosus. Biol. Res. 47, 1–10. doi: 10.1186/0717-6287-47-76
Zeb, A., Ullah, F., Ayaz, M., Ahmad, S., and Sadiq, A. (2017). Demonstration of biological activities of extracts from Isodon rugosus Wall. Ex Benth: Separation and identification of bioactive phytoconstituents by GC-MS analysis in the ethyl acetate extract. BMC Complement. Altern. Med. 17:284. doi: 10.1186/s12906-017-1798-9
Keywords: Eryngium caeruleum, h-glucosidase inhibitors, antioxidant, diabetes mellitus, GC- MS, HPLC, molecular docking
Citation: Sadiq A, Rashid U, Ahmad S, Zahoor M, AlAjmi MF, Ullah R, Noman OM, Ullah F, Ayaz M, Khan I, Islam Z-U and Ali W (2020) Treating Hyperglycemia From Eryngium caeruleum M. Bieb: In-vitro α-Glucosidase, Antioxidant, in-vivo Antidiabetic and Molecular Docking-Based Approaches. Front. Chem. 8:558641. doi: 10.3389/fchem.2020.558641
Received: 03 May 2020; Accepted: 14 October 2020;
Published: 26 November 2020.
Edited by:
Simone Brogi, University of Pisa, ItalyReviewed by:
Tsun-Thai Chai, Tunku Abdul Rahman University, MalaysiaMartin González-Andrade, National Autonomous University of Mexico, Mexico
Copyright © 2020 Sadiq, Rashid, Ahmad, Zahoor, AlAjmi, Ullah, Noman, Ullah, Ayaz, Khan, Islam and Ali. This is an open-access article distributed under the terms of the Creative Commons Attribution License (CC BY). The use, distribution or reproduction in other forums is permitted, provided the original author(s) and the copyright owner(s) are credited and that the original publication in this journal is cited, in accordance with accepted academic practice. No use, distribution or reproduction is permitted which does not comply with these terms.
*Correspondence: Abdul Sadiq, sadiquom@yahoo.com