- 1Department of Chemical Sciences, University of Johannesburg, Doornfontein Campus, Doornfontein, South Africa
- 2Department of Science and Innovation/National Research Foundation South African Research Chairs Initiative Chair: Nanotechnology for Water, University of Johannesburg, Doornfontein, South Africa
- 3Department of Science and Innovation/Mintek Nanotechnology Innovation Centre, University of Johannesburg, Doornfontein, South Africa
In this study, a magnetic porphyrin-based porous organic polymer (MP-POP) nanocomposite was successfully synthesized according previous studies and applied as an adsorbent for simultaneous extraction and preconcentration of four neonicotinoid insecticides from surface river water. The MP-POP was characterized using X-ray diffraction (XRD), transmission electron microscopy (TEM), scanning electron microscopy/energy dispersive x-ray spectroscopy (SEM/EDS), N2-adsorption/desorption analysis, Fourier Transform infrared spectroscopy (FTIR). The neonicotinoid insecticides were quantified using high performance chromatography coupled with diode array detector (HPLC-DAD). The MP-POP shown to have a high surface area, highly porous structure and strong affinity toward the investigated analytes. The adsorption capacities were 99.0, 85.5, 90.0, and 79.4 mg g−1 for acetamiprid, clothiandin, thiacloprid and imidacloprid, respectively. The influential parameters affecting the magmatic μ-solid phase extraction (M-μ-SPE) procedure were investigated using fractional factorial design and surface response methodology (RSM). Under optimum conditions, the method exhibited relatively low limit of detection in the range of 1.3–3.2 ng L−1, limit of quantification in the range of 4.3–11 ng L−1 and wide linearity (up to 600 μg L−1). The intraday and interday precision, expressed as the relative standard deviation (RSD) were <5%. The percentage recoveries for the four target analytes ranged from 91 to 99.3% for the spiked river water samples. The method was applied for determination of neonicotinoids in river water samples and concentrations ranged from 0 to 190 ng L−1.
Introduction
Neonicotinoid insecticides are applied worldwide to combat unwanted insects from attacking crops which led to their entry into the environment (Goulson, 2013; Klarich et al., 2017; Hladik et al., 2018). However, over- application of neonicotinoid insecticides in the environment can cause negative effects on human health and living organisms (Klarich et al., 2017; Struger et al., 2017). Several severe human diseases such as cancer, chronic pulmonary disease, birth defects and infertility are associated with the exposure to neonicotinoid insecticides (Giroud et al., 2013; Sánchez-Bayo and Hyne, 2014; Vichapong et al., 2015). The permissible maximum residue limits for neonicotinoid insecticides have been controlled by the Codex Alimentarius Commision and European Union and World Health Organization to protect human health (Vichapong et al., 2016). However, these regulated limits are only applicable to crops and soil, not to portable water, river water, water reservoirs and surface or ground water (Vichapong et al., 2015, 2016). Therefore, to ensure safe water to humans and living organisms, an effective technique for the detection of the neonicotinoid insecticides in surface water, portable water, river water and water reservoirs is of remarkable significance.
Different types of analytical techniques including gas chromatography (Ai et al., 2010; Kiljanek et al., 2016; Balsebre et al., 2018), high performance liquid chromatography (HPLC) (Wu et al., 2011; Giroud et al., 2013; Vichapong et al., 2015; Cao et al., 2018; Kachangoon et al., 2020) and liquid chromatography tandem mass spectrometry (LC-MS/MS) (Bolzan et al., 2015; Kiljanek et al., 2016; Zhou et al., 2018; Hou et al., 2019) have been used for the detection and analysis of neonicotinoid insecticides in various samples. However, due to the intricacy of different sample matrices and the trace amounts of the neonicotinoid insecticides, sample clean-up techniques are required prior to instrumental analysis. The benefits of sample preparation do not only involve sample clean-up, but also preconcentration of the target analytes (Farajzadeh et al., 2016; Vichapong et al., 2016; Moyakao et al., 2018; Kachangoon et al., 2020). Currently, several sample extraction techniques, such as cloud point extraction (CPE) (Kachangoon et al., 2020), liquid-phase microextraction (LLME) (Zhang et al., 2012; Jovanov et al., 2013; Bolzan et al., 2015; Vichapong et al., 2015; Hou et al., 2019; Kachangoon et al., 2020) solid-phase extraction (SPE) (Xie et al., 2011; Shi et al., 2017; Zhang et al., 2017; Cao et al., 2018; Moyakao et al., 2018; Xiong et al., 2018; Hou et al., 2019) and solid-phase microextraction (SPME) (Ding et al., 2019; Queiroz et al., 2019; Xue et al., 2019), have been reported for the extraction of the neonicotinoid insecticides in various samples. Among the SPE based methods magnetic solid-phase extraction (MSPE) has gained a lot of attention due to its attractive properties such as simplicity, rapidity, robustness, high enrichment factors, and environmentally friendliness (Deng et al., 2009, 2019; Wang et al., 2018b; Queiroz et al., 2019). In MSPE, the magnetic sorbent play a major role on the analytical performance of the method (He et al., 2014; Fumes et al., 2015; Jiang et al., 2019; Li and Shi, 2019). Therefore, it is important to design, synthesize, and explore efficient magnetic adsorbents with high affinity toward target analytes.
Recently, literature has suggested that porous adsorbents such as porous organic polymers (POPs) have received significant attention in different scientific field. They have been applied as novel materials in luminescent sensing (Li et al., 2019; Pan et al., 2019) catalysis (Kaur et al., 2011; Zhang and Riduan, 2012; Das et al., 2019; Li et al., 2020), gas storage (Wood et al., 2008; Das et al., 2019), electrochemical sensing (Vilian et al., 2018), drug delivery, and adsorption and SPE (Huang et al., 2017; Wang et al., 2017, 2018a,b; Xiong et al., 2019; Li et al., 2020). These materials are prepared by combining various monomer units using different types of chemical reactions (Li et al., 2020). Depending on the type of chemical reaction, a wide range of structural frameworks are achieved (Wood et al., 2008; Wang et al., 2017, 2018a,b; Li et al., 2020). Structurally, POPs poses strong covalent bonds that leads to attractive features such as high mechanical, chemical and thermal stabilities (Wang et al., 2017, 2018a,b). Among different types of porous materials, the application of porphyrin based POPs (PPOPs) as adsorbents is increasing due to their large macrocyclic cavity, surface areas, remarkable stability and excellent affinity toward organic pollutants (Wang et al., 2017, 2018a,b). However, little has been reported about their application as adsorbent in sample preparation methodologies. Owing to the attractive features of P-POPs materials, they serves as suitable sorbents for extraction and preconcentration of low concentration neonicotinoid insecticides in various matrices.
Previous studied on sample preparation methods have applied once factors at time approach for the optimization of factors affecting preconcentration of target analytes (Asadollahzadeh et al., 2014). However, this approach is time-consuming, laborious, and sometimes unable to reach the accurate optimum because the interactions among the investigated variables are ignored (Asadollahzadeh et al., 2014). To overcome these challenges, optimization of factors affecting different variables has been achieved using design of experiments (DOE). This approach allows minimal number of with of experimental runs, thus leading to cost-effective method and acceptable results (Asfaram et al., 2017). In addition, DOE permits the investigation of interaction among the selected variables (Asfaram et al., 2017; Bagewadi et al., 2018). The use of DOE models such as Plackett–Burman design (PBD), full factorial design, fractional factorial design and response surface methodology (RSM) [such as Box–Behnken design, Doehlert design, central composite design (CCD)] has been reported in the literature (Zolgharnein et al., 2013; Asadollahzadeh et al., 2014; Benredouane et al., 2016; Asfaram et al., 2017; Bagewadi et al., 2018). Among the above-mentioned RSM models, CCD, is one of the most effective experimental designs. This is because CCD allows each independent variable to be investigated at five levels (that is, two-level factorial (±1), axis points (±α) and central points (0) (Mousavi et al., 2018). In addition, in cases where many variables need to be optimized, screening designs such as full or fractional factorial designs are mostly applied (Asadollahzadeh et al., 2014; Benredouane et al., 2016).
The aim of this study was to prepare magnetic porphyrin-based porous organic polymer (MP-POP) nanocomposite as a magnetic adsorbent in ultrasound assisted dispersive magnetic solid phase extraction (UA-DMSPE) and preconcentration of neonicotinoid insecticides from river water samples. The neonicotinoid insecticides in samples were quantified using HPLC-DAD. Previous studies reported the application MP-POP as an adsorbent for preconcentration of nitrogen bearing analytes such as benzoylurea insecticides and phenylurea herbicides and the result revealed that MP-POP has high affinity toward the target analytes (Wang et al., 2017, 2018a,b). Therefore, the adsorbent was chosen because of its high adsorption capacity, high surface area and strong affinity to aromatic compounds containing nitrogen atoms. The MP-POP nanocomposite good magnetic properties and could be easily separated from aqueous solution by an external magnet. The factors affecting the extraction and preconcentration procedure were optimized using fractional factorial design (FFD) and central composite design (CCD). These multivariate approach chosen because they can reduce the number of experiments required and gives more quantitative information about the significance of independent variable and their interactions (Bezerra et al., 2019; Tan and Lee, 2019). FFD was used screening was carried out to obtain the critical parameters for the extraction and preconcentration of neonicotinoid insecticides The UA-DMSPE/HPLC-DAD procedure was successfully used for simultaneous extraction, preconcentration, separation, and quantification of neonicotinoid insecticides in river water samples.
Experimental
Reagents and Standards
Terephthalaldehyde (98%), Pyrrole (99%), Ferrous chloride tetrahydrate (FeCl2·4H2O), Ferric chloride hexahydrate (FeCl3·6H2O), and aqueous ammonia (w, 30%), tetrahydrofuran (THF), dichloromethane, glacial acetic acid and HPLC grade methanol (99, 9%) and acetonitrile (99, 9 %) were all purchased from Sigma-Aldrich (St Louis, MO, USA). The analytes were reagent grade insecticides (clothiandin, imidacloprid, acetamiprid, and thiacloprid) were procured from Sigma-Aldrich (South Africa) Ltd. A 10 mg L−1 stock solution containing the analytes was prepared by weighing and dissolving the insecticides in acetonitrile. The stock solution was transferred in amber storage bottles and stored at 4°C before use. Working synthetic samples were prepared daily by diluting appropriate volumes of the stock solution with ultra-pure water (Direct-Q 3 UV-R purifier).
Sampling and Sample Collection
River water samples were collected in different points at Apies River (Pretoria, Gauteng, South Africa). Schott bottles with caps (500 mL) were cleaned and used to collect water samples. When not in use, the samples were stored at 4°C.
Instrumentations
The functional groups and structural changes of the nanocomposite were investigated using on a Perkin-Elmer Spectrum 100 spectrometer (Perkin-Elmer, USA). The samples were mixed with the potassium bromide (KBr) for form pellet and the spectra were recorded in the 400–4,000 cm−1 region. The morphological structure and elemental composition of the nanocomposite was were assessed using scanning electron microscopy equipped with energy dispersive X-ray spectroscopy (EDS) at a voltage of 20 kV (SEM, TESCAN VEGA 3 XMU, LMH instrument (Czech Republic). The internal structure of the composite was studied by dispersing the adsorbent in methanol, transferring a drop of the mixture onto a copper grid and analysis using transmission electron microscopy at a voltage of 120 kV (TEM JOEL JEM-2,100, Japan). The crystal structure and pore size distribution were studied using X-Ray diffraction (XRD) and BET N2 adsorption, respectively, Analyzer (ASAP2020 V3. 00H, Micromeritics Instrument Corporation, Norcross, USA). The Barrerr-Joyner-Halenda method was used to calculate the adsorbent pore volumes. An OHAUS starter 2,100 pH meter (Pine Brook, NJ, USA) for pH adjustments of reagents and pH of samples. Chromatographic analysis was carried out at wavelengths 250 nm and 260 nm using an Agilent HPLC 1,200 infinity series, with a diode array detector (Agilent technologies, Waldbronn Germany) and a Agilent Zorbax Eclipse Plus C18 column (3.5 μm × 150 mm × 4.6 mm) (Agilent Newport, CA, USA) baked at oven temperature of 25°C. All quantifications were done using an isocratic elution programme with mobile phase system containing water (mobile phase A, 70%) and acetonitrile (mobile phase B, 30 %) at a flow rate of 0.1.00 mL min−1.
The Preparation of MP-POP Nanocomposite
Magnetic porphyrin based porous organic polymer was synthesized following methods described by Wang et al. (2017, 2018a,b). The preparation of MP-POP was a two-step process where the porphyrin based porous organic polymer was synthesized in step 1, followed by co-precipitation in step two to make the MP-POP.
Preparation of the P-POP
The synthesis of P-POP was carried out as follows: ~0.10 g of fresh pyrrole and 0.20 g terephthaldehyde were placed into a dry round bottom flask containing glacial CH3COOH (50 mL) and iron(III) chloride (0.47 g). The mixture was then agitated using a magnetic stirrer bar under a gentle stream of nitrogen for 3 h. The resultant solution was gentle transferred into a Teflon lined autoclave. The mixture in the autoclave was hydrothermal treated by placing the autoclave in an oven at 180°C for 24 h. The reaction vessel (autoclave) was cooled at room temperature and the resultant dark brown precipitate was separated from aqueous solution by centrifugation. The solid product was with water, methanol and tetrahydrofuran (THF), respectively, and it was dried under vacuum at 70°C.
The Preparation of the MP-POP
1.17 g FeCl3·6H2O and 0.43 g FeCl2·4H2O were placed in a round bottom flask having 500 mg of P-POP and 250 mL of water and mechanically stirred at room temperature under N2 atmosphere. The solution was then heated to 50°C followed by the dropwise addition of 14% ammonia solution under a pH of 11-12 was achieved. The reaction was continued for an hour to allow for the complete growth of nanoparticles. Magnetic separation was then used to collect the final product which was washed with deionised water several times until pH 7 and then with ethanol before finally being dried at 60°C.
Ultrasound Assisted Dispersive Magnetic Solid Phase Extraction Procedure
A suitable amount of MP-POP nanocomposite was placed into 10 mL glass sample bottles having 5 mL of synthetic sample (containing a mixture of clothiandin, thiacloprid, acetamiprid and imidacloprid all at 100 μg L−1). The mixtures were then sonicated in an ultrasound water bath for 10–15 min. Magnetic separation done to separate the supernatant from the adsorbent. The supernatant was then decanted, and the analytes were eluted by sonicating the adsorbent with 500–100 μL of 100% acetonitrile for 3 min. The analysis of the analytes concentrations was done by the employment of the HPLC-DAD.
Optimisation of UA- DMSPE Procedure
The most significant independent variables affecting the UA- DMSPE method were optimized using design of experiments (DOE). The firstly, screening of the parameters was done using the multivariate optimisation approach, specifically fractional factorial design (24−1) and central composite design was carried out to determine the optimum conditions, and the parameters involved in the design are shown in Supplementary Table 1. Under optimum conditions, the method was applied investigate the effect of sample volume (5–50 mL) and initial concentration (50–2,000 μg L−1).
Validation of the Method and Quality Assurance/Quality Control
The established method performance was validated by evaluating the accuracy (recovery), precision (intraday (repeatability) and interday (reproducibility), the linear dynamic range, preconcentration factor, enrichment factor and limit of detection (LOD) and limit of quantification (LOQ). The accuracy was evaluated for the target neonicotinoids in river water samples spiked at three concentration levels, 5, 100, and 500 μg L−1. The linearity was investigated by construction a seven-points calibration curve (the standards were prepared by spiking ultrapure water with a mixture of target analytes at 0 to 1,200 μg L−1). The LOD and LOQ were calculated as: LOD = and LOQ = , where Sd is the standard deviation of 10 replicate determinations of the lowest concentration of calibration curves (0.5 μg L−1) and b is the slope of each calibration curve. In addition, the LOD and LOQs were determined using spiked blank river water samples with decreasing concentrations (0.01, 0.05, and 0.10 μg L−1) of each target analyte. For this purpose river water sample free from the target analytes was used. Linearity was performed using matrix-matched calibration curves. The blank river water sample was spiked with 0–700 μg L−1 solutions containing all target analytes. The sample were then processed with the developed method and seven point calibration was constructed. The repeatability and reproducibility of the developed method were calculated from several measurements of 5.0, 100, and 500 μg L−1 in river water samples. This was carried out to assess the matrix effect on the extraction efficiency of the developed method. The preconcentration factors were explained as the ratios between sample volume (Vs) and eluent volume (Ve) (Equation 1):
The enrichment factors (EF) was estimated as the fraction between the slopes before and after preconcentration (Equation 2):
Where Sap = slope after preconcentration; bp = slope before preconcentration.
In order to comply with the quality assurance/quality control (QA/QC) guidelines, blanks were injected to the HPLC-DAD system before the injection of any sample containing analytes. The chromatograms of the blank samples revealed that the samples (blanks) were free of target analytes. This indicated that the blank correction of the results obtained from samples containing the analytes was not required. Standard solutions of neonicotinoid insecticides at concentration of 50 and 500 μg L−1 were used as used as QA/QC samples. In the course of sample analysis, procedure blank samples (treated the same way as samples) and QA/QC standard solutions were analyzed after every ten samples.
Adsorption Capacity and Regeneration Studies
The adsorption equilibrium experiments were conducted as follows: briefly 15 mg of MP-POP was weighed and transferred into 10 sealable glass containers. Then 30 mL of stock solution with varying concentrations (2–50 mg L−1) were then added into the glass bottle containing the adsorbent. Agitation of the solution by a sonicator was performed for 5 min. The absorbent and the supernatant were separated with the aid of an external magnet. Analysis of the supernatant was then carried out using HPLC-DAD. The adsorbent reusability was investigated by a series of extraction, elution, washing and drying. 15 mg of the adsorbent was added into a glass bottle container, then 30 mL of the sample was added into the glass container with adsorbent. The solution was sonicated for 15 min, then the supernatant and the absorbent were separated with the magnet. Elution was then carried out using acetonitrile, lastly the elute was then analyzed using HPLC-DAD. After each analysis the adsorbent was dried and reused.
Results and Discussion
Characterization of the Nanocomposite
The functional groups of the synthesized MP-POP were confirmed using FTIR spectroscopy (Figure 1). The broad peak that appeared in both spectra at around 3,500 cm−1 indicates the N-H peak, and then followed by a smaller and sharp peak at around 2,986 cm−1 which is for phenyl (Wang et al., 2017, 2018a,b)A peak for alkene carbons (C=C) in the pyrrole ring was observed at 1,604 cm−1 and Fe-N peak at 1,047 The Fe-N peak shows that the magnetic iron was successfully incorporated on the POP without any alterations (Wang et al., 2017, 2018a,b). Lastly, a strong signal seen at around 570 cm−1 for Fe-O indicates the adherence of Fe3O4 on the surface of the POP (Ai et al., 2010; Wang et al., 2017). These verdicts were in line with those shown in the literature (Wang et al., 2017, 2018a,b).
Scanning electron microscopy and TEM were employed to investigate the porosity and morphology of POP and MP-POP nanomaterials. The SEM images (Figures 2A,B) displays that highly porous materials were successfully synthesized. The TEM image (Figure 2C) revealed that P-POP composed of was spherical shapes. Furthermore, Figure 2D revealed that that the Fe3O4 nanoparticles were successfully incorporated on the surface of P-POP (Wang et al., 2017, 2018a,b).
The energy dispersive x-ray spectroscopy (EDS) spectra for POP and MP-POP are displayed in Figure 3. As seen in Figure 3A, as expected the spectrum for POP revealed presence of the N and C in the structure of POP. The presence of Cl and Fe was from the ferric chloride which was used in the synthesis. Figure 3B revealed all major elements present in MP-POP nanocomposite. The intense peak of Fe indicates that the Fe3O4 was successfully incorporated.
The XRD spectroscopy was used to study the crystalline structure of P-POP and MP-POP. Figure 4A demonstrated that P-POP had weak diffraction peak around 2θ = 24° suggesting that the material was naturally amorphous (Wang et al., 2017, 2018a,b). The XRD patterns for MP-POP (Figure 4B) showed crystalline structure confirming the incorporation of magnetic particles on the surface of P-POP. The prominent diffraction peaks around 30.5° (311), 36.3° (400, 43.4° (422), 56.9° (511), and 62.8° (440) corresponded to the crystalline structure of Fe3O4 (JCPDS file 19-0629) (Deng et al., 2009, 2019; Wang et al., 2017, 2018a,b; Munonde et al., 2018) These observations were in agreement with EDS and TEM results and other reports in the literature (Wang et al., 2017, 2018a,b).
The surface properties of P-POP and MP-POP were investigated by N2 adsorption/desorption. The Brunauer-Emmett-Teller (BET) surface areas of the P-POP and MP-POP were found to be 478 and 295 m2 g−1, respectively. Moreover, the total pore volumes were 0.55 and 0.39 m3 g−1 for P-POP and MP-POP. It can be seen that the incorporation of Fe3O4 reduced the BET surface area of nanocomposite. According to Figure 5A, P-POP gives a typical type I isotherm signifying the classical characteristic of microporous materials (Cao et al., 2018; Wang et al., 2018b; Hao et al., 2019; Li et al., 2020). As shown in Figure 5B, the N2 adsorption-desorption isotherm for MP-POP present a distinctive type IV isotherm. These results reveal the presence of various pore sizes varying from micropores to mesopores (Wood et al., 2008; Nqombolo et al., 2019). In addition, large hysteresis loop at high relative pressure approaching 1.0, suggested the presence of microporous structures (Cao et al., 2018; Wang et al., 2018b; Hao et al., 2019; Nqombolo et al., 2019; Li et al., 2020).
Selection of Eluent Type
The choice of a suitable elution solvent was investigated in order to achieved quantitative desorption of the analytes that are adsorbed on the surface adsorbent as well as attaining relatively high enrichment factor. In this study, the desorption capabilities of various HPLC grade organic solvents (ethanol, acetonitrile, acetone and methanol) were investigated and the adsorption-desorption experiments were carried in triplicate. The results in Figure 6 showed that the aprotic solvents (acetonitrile and acetone) had better elution capabilities as compared to methanol and ethanol. Hence, acetonitrile was chosen as the desorption solvent because it was the component of the mobile phase.
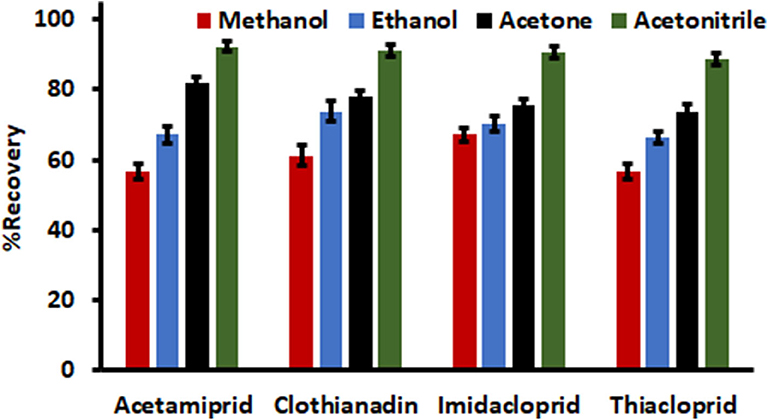
Figure 6. Selection of eluent type. Experimental conditions: sample volume, 5 mL; mass of adsorbent, 15 mg; acetonitrile volume, 750 μL; desorption time, 5 min; extraction time, 15 min and sample pH, 7.0.
Optimization Strategy
Fractional Factorial Design
A fractional factorial design (FFD) with four independent variables including pH of the sample, eluent volume (EV), mass of the adsorbent (MA) and extraction time (ET) at three levels which includes central points was used for the screening process. The design matrix and respective analytical response (percentage recovery) for each analyte are displayed (Supplementary Table 2). The data was assessed using analysis of variance (ANOVA). Pareto charts reproduced from the ANOVA results is used to indicate parameters that are significant for the preconcentration and extraction method. The blue bar on the left-hand side they represent the individual parameters and if the bar crosses the 95% confidence interval line (red line), it means that the parameter is significant (Zhang et al., 2017; Bezerra et al., 2019; Tan and Lee, 2019). As seen in Supplementary Figures 1A–D, the Pareto charts indicate that none of the parameters were insignificant at 95% confidence interval. Though, the bar length of EV, MA, and ET suggested that these three parameters has a significant role in the extraction and preconcentration of neonicotinoid insecticides. Therefore, EV, MA, and ET were further optimized using response surface methodology based on central composite design. The sample pH of the sample was fixed at 7.0.
Response Surface Methodology Based on Central Composite Design
Response surface methodology (RSM) based on CCD with three independent experimental parameters investigated at five levels, was used to investigate the interactions and quadratic effects of the main effects (Supplementary Table 3). The 3D surface plots demonstrating the effect of independent factors and their interactions on the analytical response are shown in Supplementary Figure 2. The influence of eluent volume (Supplementary Figures 2A,C) reveals that the quantitative recoveries were obtained between 600 and 1,200 μL of acetonitrile. Mass of adsorbent in solid phase extraction method plays a major role in achieving quantitative recoveries. As seen in Supplementary Figures 2A,B, mass of MP-POP as low as 15 mg could lead to extraction efficiency. Supplementary Figures 2B,C revealed that the effect of extraction lead to high extraction efficiency after 15 min.
Profile for Predicted Values and Desirability
The desirability function shown in Supplementary Figure 3, allows the simultaneous estimation of optimal values for all the investigated factors. Desirability always take values within 0-1 range where 0 indicates the least desired results (0.0%), 0.5 being the central point (50.8%), and 1 being the most desirable value assigned a percentage of (101.5%) (Candioti et al., 2014; Bezerra et al., 2019; Mashile et al., 2020). According to the desirability profiles, the optimal conditions for preconcentration and extraction process were MA of 15 mg, EV of 1,130 μL and extraction time of 15 min. The overall optimum parameters for extraction and preconcentration were 15 mg, 1,130 μL, 15 min and 7.0 for mass of the adsorbent, acetonitrile volume, extraction time and sample pH, respectively. The predicated optimum experimental parameters were verified experimentally. The results obtained for simultaneous extraction and preconcentration of four neonicotinoid insecticides ranged from 97.8 to 99.0%. The experimental findings were in full agreement with predicted values, suggesting that the model was valid. The obtained optimum conditions were used for further investigation.
Effect of Initial Concentration and Sample Volume on the Recovery of Neonicotinoid Insecticides
Under optimized conditions, the influence of initial concentration on the percentage recovery of the selected neonicotinoid insecticides was assessed by processing various neonicotinoid insecticides solution at concentrations between 50 and 2000 μg L−1. The results in Supplementary Figure 4A, revealed that optimum recoveries (≥99%) at initial concentrations ranging from 50 to 1,200 μg L−1. Furthermore, the effect of sample volume was investigated in order to assess the possibility of achieving high preconcentration factors and examine its effect on percentage recoveries of the analytes. This was achieved by applying the optimized method to a series of sample solution ranging from 5 to 50 mL containing a fixed concentration of the analytes (100 μg L−1) of target analytes. The results obtained are presented in Supplementary Figure 4B and it can be seen that quantitative recoveries (>95%) of all four analytes was obtained for sample volumes of ≥ 30 mL. Therefore, the extracted analytes were could be eluted with 1,130 μL acetonitrile. The preconcentration factor of 27 was realized by the current method.
Adsorption Capacity
Adsorption isotherms models are vital in describing and explaining interactive behavior of adsorbate and the adsorbent (MP-POP) (Bordoloi et al., 2017; Rafati et al., 2018). Therefore, equilibrium studies were carried in order to establish the adsorption mechanism. Equilibrium is established when the sample containing adsorbate is in contact with the absorbent for a specific time (Pezoti et al., 2016). The equilibrium data for the adsorption of neonicotinoid insecticides was done using various isotherms such as Langmuir, Freundlich, Redlich-Peterson and Dubin-Radushkevich models.
From the Table 1 below, isotherm models and parameters, the adsorption data followed Langmuir isotherm model with correlation efficiency of 0.9499, which was higher than that of Freundlich model for all the insecticides, this describes monolayer adsorption (Pezoti et al., 2016). Redlich-Peterson is a mixture of both Freundlich and Langmuir isotherms models, the focus here is at beta value, which is the exponent from the linear plot and is between 0 and 1 (Esfandiyari et al., 2017). When beta is equivalent to 1, the model reduces to Langmuir equation and when beta is 0 it reduces to Freundlich equation (Khare et al., 2018), from this study, the θ values were found to be 0.88 for acetamiprid, 0.65 for clothiandin, 0.75 for thiacloprid and 0.52 for imidacloprid which was ~1 suggesting the model reduces to Langmuir. The favoring of Langmuir by the isotherm models indicates that the adsorption was a monolayer adsorption. Dubin-Radushkevish model is normally used to differentiate between chemical and physical adsorption by looking at the mean energy (E) (Bordoloi et al., 2017; Rafati et al., 2018). The focus is on the energy value (E), if the value of energy is <8 kJ mol−1, then the adsorption was a physical one. If the energy value is among 8 and 16 kJ mol−1 then the adsorption was a chemical one (Bordoloi et al., 2017). In this study the calculated E values were 0.34, 0.22, 0.44, and 0.31 kJ mol−1 and for acetamiprid, cloathiandin, thiacloprid, and imidacloprid, respectively. The obtained energy values indicated physisorption processes.
Analytical Figures of Merit
The analytical performances of the established method for preconcentration and simultaneous extraction of neonicotinoid insecticides were investigated (Table 2), under optimum experimental conditions. The findings exhibited relatively wide linear dynamic ranges with coefficient of coefficients (R2) ranging from 0.9981 to 0.9994. The instrumental LODs and LOQs ranged from 1.3 to 3.2 ng L−1 and 4.3 to 11 ng L−1, respectively. The repeatability (intraday) and reproducibility (interday) expressed in terms of %RSD were <5% (Table 2), suggesting that the established method had relatively good precision. The preconcentration factor was estimated to be 27.
The development was also validated methods using spiked water samples. The linearity, LODs and LOQs and RSD for the studied analytes are presented in Table 3. The linearity ranging from LOQ-650 was obtained for the neonicotinoid insecticides. As seen the LODs and LOQs were ranged from 1.8 to 3.7 ng·L−1 and 6.0 to 12 ng·L−1, respectively. Whereas, the interday precision with spiked blank river water samples ranged from 2.1 to 6.1%. To evaluate and understand the effect of sample matrix on the extraction performance of the developed method, relative recoveries were tested by spiking ultra-pure water and river water samples. As seen, the relative recoveries of the target analytes were between 95 and 99%, suggesting that the current method did not suffer from matrix.
The accuracy of the established method was investigated by analyzing spiked river water sample at two concentration points (Table 4) The river water samples were spiked with target analytes at three points (50, 100, and 500 ng L−1) and the samples were analyzed using the established method. The samples were also used to investigate the intraday and interday precision. As seen in Table 4, the percentage recoveries for the four target analytes ranged from 91 to 99.3% and the %RSD values ranged between 1.4 and 4.7%. The typical chromatogram of river spiked with 100 ng L−1 target is presented in Supplementary Figure 5. As seen the chromatogram shows good separation and there are no interfering peaks. This proves that the developed method was able to clean-up sample matrix, extract and preconcentrate the target analytes.
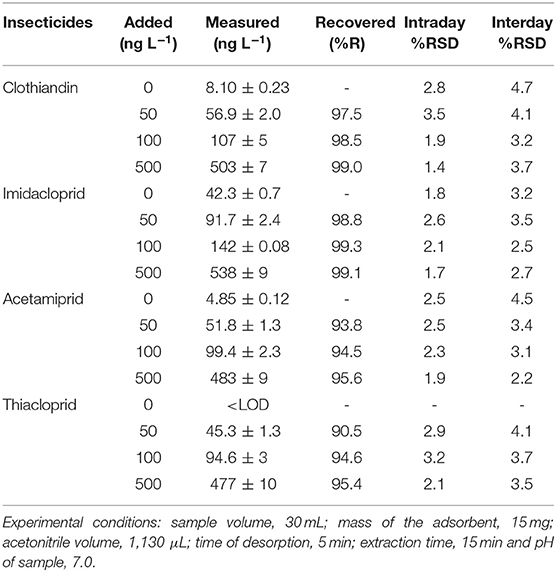
Table 4. Analysis of neonicotinoid insecticides in spiked river sample using UA-DMSPE/HPLC-DAD method.
Comparison of the Developed Method With Others Reported in the Literature
The analytical figures of merit for the developed method were compared with those that were previously reported for preconcentration and extraction of clothianidin, imidacloprid, acetamiprid, and thiacloprid their determination using chromatographic techniques are displayed in Table 5. As seen, the established method had lower LODs and LOQs compared those reported by elsewhere (Sánchez-Hernández et al., 2014; Bolzan et al., 2015; Cao et al., 2018; Kachangoon et al., 2020). The relative standard deviation was found to be better than those reported in literature (Table 5). In addition, the LODs and LODs were comparable (in the same magnitude) with those reported in the literature (Zhang et al., 2017; Moyakao et al., 2018; Xiong et al., 2018). However, the LODs and LOQs were greater than those reported elsewhere (Li and Shi, 2019). Lastly, the method proved to have attractive advantages such as good sensitivity and simplicity because of the low LOD and LOQ, high precision and wide linearity. Even though, the performance of the MPOP was comparable to traditional SPE adsorbent such as HLB/GCB (Zhang et al., 2017) as well as HLB combined with C18 (Dujaković et al., 2010), these method combined two types of traditional adsorbent in order to achieve low LODs and LOQs. As in Table 5, studies have proven that when C18 is used alone, the high LODs were between 1.0 and 2.3 μg L−1 were obtained (Sánchez-Bayo and Hyne, 2014). Therefore, the advantages of the developed method over the traditional SPE procedures include short preconcentration time (15 min extraction and 5 min desorption time), use of a single easily recoverable adsorbent with high surface area, easy operation and low cost. Moreover, the proposed method is environmentally friendly compared to traditional SPE because it uses small amount of a reusable adsorbent (15 mg) and the use of excessive organic solvents in minimized (in this work only 1,130 μL was used).
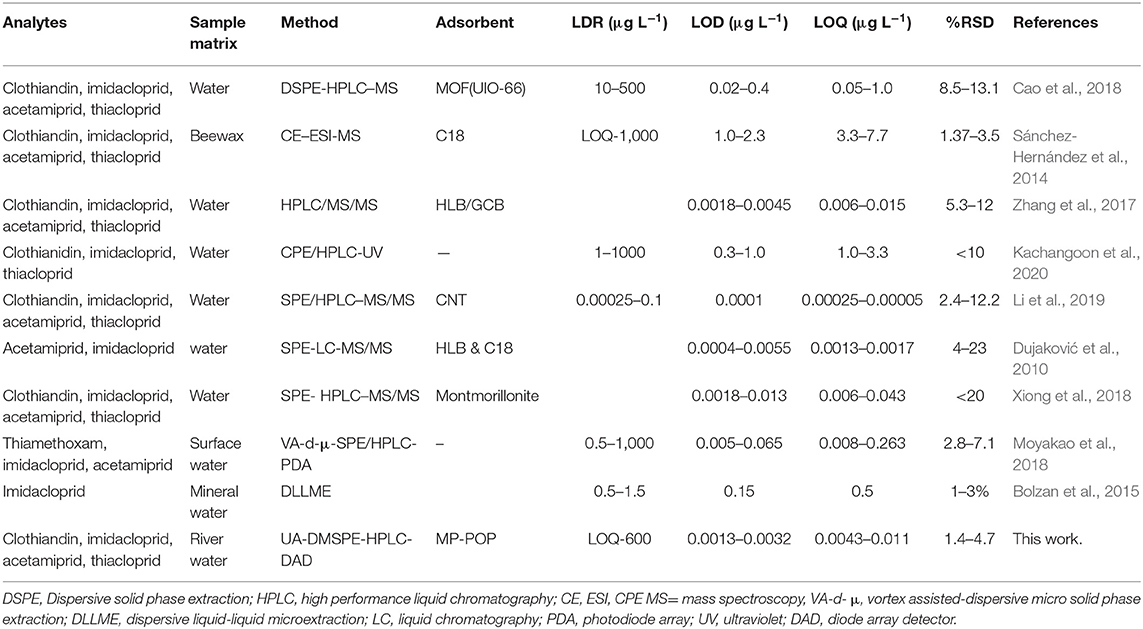
Table 5. Comparison of analytical performance of the developed method with others that are reported in literature.
Application to Real Water Samples
The applicability of MP-POP as a sorbent for extraction and preconcentration of neonicotinoid insecticides was carried out by the analysis of two river samples. As seen, trace amounts of target analytes were detected in river water samples (Table 6, Supplementary Figure 6) except thiacloprid in river sample 1. Clothiandin, acetamiprid and imidacloprid were frequently detected in all river samples at relatively higher concentrations (7.5–8.10 ng L−1 clothiandin, 4.6–109 ng L−1 imidacloprid and 4.85–20.7 ng L−1 acetamiprid) compared to thiacloprid. This is because these three neonicotinoid insecticides are the mostly used in tomato and maize plantation which are common in South Africa. The results showed that MP-POP can be applicable for extraction and preconcentration of neonicotinoid insecticides in real samples regardless of the complex matrix. This is shown by the smoothness of chromatograms that there was no interference from the complex matrix of the samples (Supplementary Figure 6).
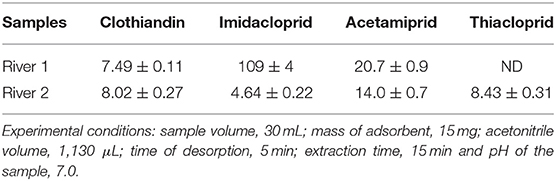
Table 6. Concentration of neonicotinoid insecticides in river water samples obtained using UA-DMSPE/HPLC-DAD method.
The maximum concentration of neonicotinoid insecticides obtained in this study were compared with those reported in the literature globally (Table 7). As see the concentration levels of neonicotinoid insecticides in this study were lower than those reported in United States (Starner and Goh, 2012; Ensminger et al., 2013), Canada (Main et al., 2014; Schaafsma et al., 2015), Benin (Berny's et al., 2019), and China (Zhang et al., 2017, 2019). They were higher than those reported in Japan (Yamamoto et al., 2012) and China (Xiong et al., 2018).
Reusability and Regeneration
The adsorbent regeneration and reusability were investigated by a series of extraction, elution, washing and drying. After each extraction the percentage recovery was calculated, and the findings are illustrated in Supplementary Figure 7. The percentage recoveries showed a significant decrease after cycle number 6. The results found demonstrated that recovery and adsorption for neonicotinoid insecticides were not affected for up to five cycles. Therefore, this showed a great reusability of the synthesized adsorbent and also showed its excellent regeneration properties. The significant decrease could be due to the collapsing of the pores of MP-POP and as the adsorbent was continuously used, it lost its affinity toward the neonicotinoid insecticides. The loss of affinity was also caused by the multiple washing of the adsorbent. Multiple washing of the adsorbent leads to the deterioration of the functional groups which are responsible for analyte binding on the adsorbent.
Adsorption Mechanism of the M-PPOP
The conceivable mechanism for the adsorption of neonicotinoid insecticides on the surface of the adsorbent was investigated following the method reported in the literature (Wang et al., 2017, 2018a). According to the literature, EF of each analyte can be used to investigate the adsorption affinity of the MP-POP toward the analytes on interest (Wang et al., 2017, 2018a,b). As seen, in the Supplementary Table 6, relatively high EFs (94–110) were obtained for the four investigated neonicotinoid insecticides. In addition, hydrophobicity indicator (Log Kow), hydrogen bonding preference indicators (that is, H bond acceptors and donors) were used to evaluated the adsorption mechanism (Wang et al., 2017, 2018a). As seen in Supplementary Table 4, the Log Kow (Chevillot et al., 2017; Sultana et al., 2018) was as follows: imidacloprid > clothianidin> acetamiprid > thiacloprid. This order suggested that for analytes with relatively high Log Kow like thiacloprid and acetamiprid the hydrophobic and π-staking interactions between the adsorbate and the adsorbent played a vital part during the extraction and preconcentration process (Wang et al., 2017, 2018a). Hydrogen bonding interaction had a major influence in the adsorption of imidacloprid and clothianidin. This because these two analytes have higher number hydrogen-bonding donor and acceptor sites as compared to imidacloprid and clothianidin (Supplementary Table 6). In view of the above, it was concluded that the extraction and preconcentration of neonicotinoid insecticides using MP-POP nanocomposite was driven by hydrogen bonding, hydrophobic and π-stacking interactions. These findings are alike to those reported in the literature (Wang et al., 2017, 2018a,b).
Conclusions
A rapid, simple, reliable and efficient UA-DMSPE/HPL-DAD method was developed for the simultaneous extraction, preconcentration determination of acetamiprid, clothiandin, thiacloprid, and imidacloprid in river water samples. The MP-POP adsorbent displayed relatively high chemical and thermal stabilities, remarkable regeneration and reusability properties as well as hig affinity torward target analytes. Coupling of HPLC-DAD with the preconcentration method resulted in improved LODs, LOQs, linear dynamic range, sensitivity, precision, accuracy and acceptable recoveries. As the result, the analytical performance of UA-DMSPE/HPL-DAD method was comparable to those found using LC-MS/MS. The applicability of the UA-DMSPE/HPL-DAD method was evaluated by analyzing the target analytes in spiked river water samples and percentage recovery values ranged from 91 to 99%. Furthermore, the method was later applied for determination of acetamiprid, clothiandin, thiacloprid, and imidacloprid in river water samples and the concentrations were lower or comparable with those detected in other countries. These results proved that the established method could be used for determination of neonicotinoid insecticides in complex matrices.
Data Availability Statement
The raw data supporting the conclusions of this article will be made available by the authors, without undue reservation.
Author Contributions
SS and PN: conceptualization. SS: methodology, investigation, methodology, validation, and writing- original draft preparation. AM, SS, and NW: data curation, sampling, reviewing, and editing. PN: software, funding acquisition, supervision, validation, writing- reviewing, and editing.
Conflict of Interest
The authors declare that the research was conducted in the absence of any commercial or financial relationships that could be construed as a potential conflict of interest.
Acknowledgments
The authors would like to thank the University of Johannesburg (Chemical sciences department) for providing lab facilities and the National Research Foundation (NRF, South Africa, Grant no. 91230) for financial support.
Supplementary Material
The Supplementary Material for this article can be found online at: https://www.frontiersin.org/articles/10.3389/fchem.2020.555847/full#supplementary-material
References
Ai, Z., Deng, K., Wan, Q., Zhang, L., and Lee, S. (2010). Facile microwave-assisted synthesis and magnetic and gas sensing properties of Fe3O4 nanoroses. J. Phys. Chem. C 114, 6237–6242. doi: 10.1021/jp910514f
Asadollahzadeh, M., Tavakoli, H., Torab-Mostaedi, M., Hosseini, G., and Hemmati, A. (2014). Response surface methodology based on central composite design as a chemometric tool for optimization of dispersive-solidification liquid–liquid microextraction for speciation of inorganic arsenic in environmental water samples. Talanta 123, 25–31. doi: 10.1016/j.talanta.2013.11.071
Asfaram, A., Ghaedi, M., Hajati, S., Goudarzi, A., and Dil, E. A. (2017). Screening and optimization of highly effective ultrasound-assisted simultaneous adsorption of cationic dyes onto Mn-doped Fe3O4-nanoparticle-loaded activated carbon. Ultrason. Sonochem. 34, 1–12. doi: 10.1016/j.ultsonch.2016.05.011
Bagewadi, Z. K., Mulla, S. I., and Ninnekar, H. Z. (2018). Optimization of endoglucanase production from Trichoderma harzianum strain HZN11 by central composite design under response surface methodology. Biomass Convers. Biorefin. 8, 305–316. doi: 10.1007/s13399-017-0285-3
Balsebre, A., Báez, M. E., Martínez, J., and Fuentes, E. (2018). Matrix solid-phase dispersion associated to gas chromatography for the assessment in honey bee of a group of pesticides of concern in the apicultural field. J. Chromatogr. A 1567, 47–54. doi: 10.1016/j.chroma.2018.06.062
Benredouane, S., Berrama, T., and Doufene, N. (2016). Strategy of screening and optimization of process parameters using experimental design: application to amoxicillin elimination by adsorption on activated carbon. Chemometr. Intell. Lab. Syst. 155, 128–137. doi: 10.1016/j.chemolab.2016.04.010
Berny's, G., Aïna, M. P., Toko, I. I., Igout, A., Douny, C., Brose, F., et al. (2019). Occurrence of acetamiprid residues in water reservoirs in the cotton basin of Northern Benin. Bull. Environ. Contam. Toxicol. 102, 7–12. doi: 10.1007/s00128-018-2476-4
Bezerra, M. A., Ferreira, S. L. C., Novaes, C. G., dos Santos, A. M. P., Valasques, G. S., da Mata Cerqueira, U. M. F., et al. (2019). Simultaneous optimization of multiple responses and its application in analytical chemistry–a review. Talanta 194, 941–959. doi: 10.1016/j.talanta.2018.10.088
Bolzan, C. M., Caldas, S. S., Guimarães, B. S., and Primel, E. G. (2015). Dispersive liquid-liquid microextraction with liquid chromatography-tandem mass spectrometry for the determination of triazine, neonicotinoid, triazole and imidazolinone pesticides in mineral water samples. J. Braz. Chem. Soc. 26, 1902–1913. doi: 10.5935/0103-5053.20150168
Bordoloi, N., Goswami, R., Kumar, M., and Kataki, R. (2017). Biosorption of Co (II) from aqueous solution using algal biochar: kinetics and isotherm studies. Bioresour. Technol. 244, 1465–1469. doi: 10.1016/j.biortech.2017.05.139
Candioti, L. V., De Zan, M. M., Cámara, M. S., and Goicoechea, H. C. (2014). Experimental design and multiple response optimization. Using the desirability function in analytical methods development. Talanta 124, 123–138. doi: 10.1016/j.talanta.2014.01.034
Cao, X., Jiang, Z., Wang, S., Hong, S., Li, H., Zhang, C., et al. (2018). Metal-organic framework UiO-66 for rapid dispersive solid phase extraction of neonicotinoid insecticides in water samples. J. Chromatogr. B 1077, 92–97. doi: 10.1016/j.jchromb.2017.11.034
Chevillot, F., Convert, Y., Desrosiers, M., Cadoret, N., Veilleux, É., Cabana, H., et al. (2017). Selective bioaccumulation of neonicotinoids and sub-lethal effects in the earthworm Eisenia andrei exposed to environmental concentrations in an artificial soil. Chemosphere 186, 839–847. doi: 10.1016/j.chemosphere.2017.08.046
Das, S. K., Chatterjee, S., Mondal, S., and Bhaumik, A. (2019). A new triazine-thiophene based porous organic polymer as efficient catalyst for the synthesis of chromenes via multicomponent coupling and catalyst support for facile synthesis of HMF from carbohydrates. Mol. Cataly. 475:110483. doi: 10.1016/j.mcat.2019.110483
Deng, Y., Deng, C., Qi, D., Liu, C., Liu, J., Zhang, X., et al. (2009). Synthesis of core/shell colloidal magnetic zeolite microspheres for the immobilization of trypsin. Adv. Mater. 21, 1377–1382. doi: 10.1002/adma.200801766
Deng, Z.-H., Li, N., Jiang, H.-L., Lin, J.-M., and Zhao, R.-S. (2019). Pretreatment techniques and analytical methods for phenolic endocrine disrupting chemicals in food and environmental samples. Trends Analyt. Chem. 119:115592. doi: 10.1016/j.trac.2019.07.003
Ding, H., Wang, R., Liu, W., Cheng, S., Ji, W., Zheng, Z., et al. (2019). A spherical metal-organic coordination polymer for the microextraction of neonicotinoid insecticides prior to their determination by HPLC. Microchim. Acta 186:103. doi: 10.1007/s00604-018-3210-y
Dujaković, N., Grujić, S., Radišić, M., Vasiljević, T., and Laušević, M. (2010). Determination of pesticides in surface and ground waters by liquid chromatography–electrospray–tandem mass spectrometry. Anal. Chim. Acta 678, 63–72. doi: 10.1016/j.aca.2010.08.016
Ensminger, M. P., Budd, R., Kelley, K. C., and Goh, K. S. (2013). Pesticide occurrence and aquatic benchmark exceedances in urban surface waters and sediments in three urban areas of California, USA, 2008–2011. Environ. Monit. Assess 185, 3697–3710. doi: 10.1007/s10661-012-2821-8
Esfandiyari, T., Nasirizadeh, N., Dehghani, M., and Ehrampoosh, M. H. (2017). Graphene oxide based carbon composite as adsorbent for Hg removal: preparation, characterization, kinetics and isotherm studies. Chin. J. Chem. Eng. 25, 1170–1175. doi: 10.1016/j.cjche.2017.02.006
Farajzadeh, M. A., Bamorowat, M., and Mogaddam, M. R. A. (2016). Ringer tablet-based ionic liquid phase microextraction: application in extraction and preconcentration of neonicotinoid insecticides from fruit juice and vegetable samples. Talanta 160, 211–216. doi: 10.1016/j.talanta.2016.03.097
Fumes, B. H., Silva, M. R., Andrade, F. N., Nazario, C. E. D., and Lanças, F. M. (2015). Recent advances and future trends in new materials for sample preparation. Trends Analyt. Chem. 71, 9–25. doi: 10.1016/j.trac.2015.04.011
Giroud, B., Vauchez, A., Vulliet, E., Wiest, L., and Buleté, A. (2013). Trace level determination of pyrethroid and neonicotinoid insecticides in beebread using acetonitrile-based extraction followed by analysis with ultra-high-performance liquid chromatography–tandem mass spectrometry. J. Chromatogr. A 1316, 53–61. doi: 10.1016/j.chroma.2013.09.088
Goulson, D. (2013). An overview of the environmental risks posed by neonicotinoid insecticides. J. Appl. Ecol. 50, 977–987. doi: 10.1111/1365-2664.12111
Hao, L., Wang, Y., Wang, C., Wu, Q., and Wang, Z. (2019). A magnetic covalent aromatic polymer as an efficient and recyclable adsorbent for phenylurea herbicides. Microchim. Acta 186:431. doi: 10.1007/s00604-019-3583-6
He, J., Huang, M., Wang, D., Zhang, Z., and Li, G. (2014). Magnetic separation techniques in sample preparation for biological analysis: a review. J. Pharm. Biomed. Anal. 101, 84–101. doi: 10.1016/j.jpba.2014.04.017
Hladik, M. L., Main, A. R., and Goulson, D. (2018). Environmental risks and challenges associated with neonicotinoid insecticides. Environ. Sci. Technol. 52, 3329–3335. doi: 10.1021/acs.est.7b06388
Hou, J., Xie, W., Hong, D., Zhang, W., Li, F., Qian, Y., et al. (2019). Simultaneous determination of ten neonicotinoid insecticides and two metabolites in honey and Royal-jelly by solid– phase extraction and liquid chromatography– tandem mass spectrometry. Food Chem. 270, 204–213. doi: 10.1016/j.foodchem.2018.07.068
Huang, Z., Liu, S., Xu, J., Yin, L., Zheng, J., Zhou, N., et al. (2017). Porous organic polymers with different pore structures for sensitive solid-phase microextraction of environmental organic pollutants. Anal. Chim. Acta 989, 21–28. doi: 10.1016/j.aca.2017.08.006
Jiang, H.-L., Li, N., Cui, L., Wang, X., and Zhao, R.-S. (2019). Recent application of magnetic solid phase extraction for food safety analysis. Trends Analy. Chem. 120:115632. doi: 10.1016/j.trac.2019.115632
Jovanov, P., Guzsvány, V., Franko, M., Lazić, S., Sakač, M., Šarić, B., et al. (2013). Multi-residue method for determination of selected neonicotinoid insecticides in honey using optimized dispersive liquid–liquid microextraction combined with liquid chromatography-tandem mass spectrometry. Talanta 111, 125–133. doi: 10.1016/j.talanta.2013.02.059
Kachangoon, R., Vichapong, J., Santaladchaiyakit, Y., and Srijaranai, S. (2020). Cloud-point extraction coupled to in-situ metathesis reaction of deep eutectic solvents for preconcentration and liquid chromatographic analysis of neonicotinoid insecticide residues in water, soil and urine samples. Microchem. J. 152:104377. doi: 10.1016/j.microc.2019.104377
Kaur, P., Hupp, J. T., and Nguyen, S. T. (2011). Porous organic polymers in catalysis: opportunities and challenges. ACS Catal. 1, 819–835. doi: 10.1021/cs200131g
Khare, N., Bajpai, J., and Bajpai, A. (2018). Graphene coated iron oxide (GCIO) nanoparticles as efficient adsorbent for removal of chromium ions: Preparation, characterization and batch adsorption studies. Environ. Nanotechnol. Monitor. Manag. 10, 148–162. doi: 10.1016/j.enmm.2018.06.002
Kiljanek, T., Niewiadowska, A., Semeniuk, S., Gawe,ł, M., BorzÄcka, M., and Posyniak, A. (2016). Multi-residue method for the determination of pesticides and pesticide metabolites in honeybees by liquid and gas chromatography coupled with tandem mass spectrometry—Honeybee poisoning incidents. J. Chromatogr. A 1435, 100–114. doi: 10.1016/j.chroma.2016.01.045
Klarich, K. L., Pflug, N. C., DeWald, E. M., Hladik, M. L., Kolpin, D. W., Cwiertny, D. M., et al. (2017). Occurrence of neonicotinoid insecticides in finished drinking water and fate during drinking water treatment. Environ. Sci. Technol. Lett. 4, 168–173. doi: 10.1021/acs.estlett.7b00081
Li, M., Zhao, H., and Lu, Z.-Y. (2020). Porphyrin-based porous organic polymer, Py-POP, as a multifunctional platform for efficient selective adsorption and photocatalytic degradation of cationic dyes. Microporous Mesoporous Mater. 292:109774. doi: 10.1016/j.micromeso.2019.109774
Li, W. K., and Shi, Y. P. (2019). Recent advances and applications of carbon nanotubes based composites in magnetic solid-phase extraction. Trends Analy. Chem. 118, 652–665. doi: 10.1016/j.trac.2019.06.039
Li, X., Chen, J., He, X., Wang, Z., Wu, D., Zheng, X., et al. (2019). Simultaneous determination of neonicotinoids and fipronil and its metabolites in environmental water from coastal bay using disk-based solid-phase extraction and high-performance liquid chromatography–tandem mass spectrometry. Chemosphere 234, 224–231. doi: 10.1016/j.chemosphere.2019.05.243
Main, A. R., Headley, J. V., Peru, K. M., Michel, N. L., Cessna, A. J., and Morrissey, C. A. (2014). Widespread use and frequent detection of neonicotinoid insecticides in wetlands of Canada's prairie pothole region. PLoS ONE 9:e92821. doi: 10.1371/journal.pone.0092821
Mashile, G. P., Mpupa, A., Nqombolo, A., Dimpe, K. M., and Nomngongo, P. N. (2020). Recyclable magnetic waste tyre activated carbon-chitosan composite as an effective adsorbent rapid and simultaneous removal of methylparaben and propylparaben from aqueous solution and wastewater. J. Water Proc. Eng. 33:101011. doi: 10.1016/j.jwpe.2019.101011
Mousavi, S. J., Parvini, M., and Ghorbani, M. (2018). Experimental design data for the zinc ions adsorption based on mesoporous modified chitosan using central composite design method. Carbohydr. Polym. 188, 197–212. doi: 10.1016/j.carbpol.2018.01.105
Moyakao, K., Santaladchaiyakit, Y., Srijaranai, S., and Vichapong, J. (2018). Preconcentration of trace neonicotinoid insecticide residues using vortex-assisted dispersive micro solid-phase extraction with montmorillonite as an efficient sorbent. Molecules 23:883. doi: 10.3390/molecules23040883
Munonde, T. S., Maxakato, N. W., and Nomngongo, P. N. (2018). Preparation of magnetic Fe3O4 nanocomposites modified with MnO2, Al2O3, Au and their application for preconcentration of arsenic in river water samples. J. Environ. Chem. Eng. 6, 1673–1681. doi: 10.1016/j.jece.2018.02.017
Nqombolo, A., Mpupa, A., Gugushe, A. S., Moutloali, R. M., and Nomngongo, P. N. (2019). Adsorptive removal of lead from acid mine drainage using cobalt-methylimidazolate framework as an adsorbent: kinetics, isotherm, and regeneration. Environ. Sci. Pollut. Res. 26, 3330–3339. doi: 10.1007/s11356-018-3868-z
Pan, Q., Xu, Z., Deng, S., Zhang, F., Li, H., Cheng, Y., et al. (2019). A mechanochemically synthesized porous organic polymer derived CQD/chitosan–graphene composite film electrode for electrochemiluminescence determination of dopamine. RSC Adv. 9, 39332–39337. doi: 10.1039/C9RA06912G
Pezoti, O., Cazetta, A. L., Bedin, K. C., Souza, L. S., Martins, A. C., Silva, T. L., et al. (2016). NaOH-activated carbon of high surface area produced from guava seeds as a high-efficiency adsorbent for amoxicillin removal: kinetic, isotherm and thermodynamic studies. Chem. Eng. J. 288, 778–788. doi: 10.1016/j.cej.2015.12.042
Queiroz, M. E. C., de Souza, I. D., and Marchioni, C. (2019). Current advances and applications of in-tube solid-phase microextraction. Trends Analy. Chem. 111, 261–278. doi: 10.1016/j.trac.2018.12.018
Rafati, L., Ehrampoush, M., Rafati, A., Mokhtari, M., and Mahvi, A. (2018). Removal of ibuprofen from aqueous solution by functionalized strong nano-clay composite adsorbent: kinetic and equilibrium isotherm studies. Int. J. Environ. Sci. Technol. 15, 513–524. doi: 10.1007/s13762-017-1393-0
Sánchez-Bayo, F., and Hyne, R. V. (2014). Detection and analysis of neonicotinoids in river waters–development of a passive sampler for three commonly used insecticides. Chemosphere 99, 143–151. doi: 10.1016/j.chemosphere.2013.10.051
Sánchez-Hernández, L., Hernández-Domínguez, D., Bernal, J., Neusüß, C., Martín, M. T., and Bernal, J. L. (2014). Capillary electrophoresis–mass spectrometry as a new approach to analyze neonicotinoid insecticides. J. Chromatogr. A 1359, 317–324. doi: 10.1016/j.chroma.2014.07.028
Schaafsma, A., Limay-Rios, V., Baute, T., Smith, J., and Xue, Y. (2015). Neonicotinoid insecticide residues in surface water and soil associated with commercial maize (corn) fields in southwestern Ontario. PLoS ONE 10:e0118139. doi: 10.1371/journal.pone.0118139
Shi, Z., Zhang, S., Huai, Q., Xu, D., and Zhang, H. (2017). Methylamine-modified graphene-based solid phase extraction combined with UPLC-MS/MS for the analysis of neonicotinoid insecticides in sunflower seeds. Talanta 162, 300–308. doi: 10.1016/j.talanta.2016.10.042
Starner, K., and Goh, K. S. (2012). Detections of the neonicotinoid insecticide imidacloprid in surface waters of three agricultural regions of California, USA, 2010–2011. Bull. Environ. Contam. Toxicol. 88, 316–321. doi: 10.1007/s00128-011-0515-5
Struger, J., Grabuski, J., Cagampan, S., Sverko, E., McGoldrick, D., and Marvin, C. H. (2017). Factors influencing the occurrence and distribution of neonicotinoid insecticides in surface waters of southern Ontario, Canada. Chemosphere 169, 516–523. doi: 10.1016/j.chemosphere.2016.11.036
Sultana, T., Murray, C., Kleywegt, S., and Metcalfe, C. D. (2018). Neonicotinoid pesticides in drinking water in agricultural regions of southern Ontario, Canada. Chemosphere 202, 506–513. doi: 10.1016/j.chemosphere.2018.02.108
Tan, S. C., and Lee, H. K. (2019). A hydrogel composite prepared from alginate, an amino-functionalized metal-organic framework of type MIL-101 (Cr), and magnetite nanoparticles for magnetic solid-phase extraction and UHPLC-MS/MS analysis of polar chlorophenoxy acid herbicides. Microchi. Acta 186:545. doi: 10.1007/s00604-019-3679-z
Vichapong, J., Burakham, R., Santaladchaiyakit, Y., and Srijaranai, S. (2016). A preconcentration method for analysis of neonicotinoids in honey samples by ionic liquid-based cold-induced aggregation microextraction. Talanta 155, 216–221. doi: 10.1016/j.talanta.2016.04.045
Vichapong, J., Burakham, R., and Srijaranai, S. (2015). In-coupled syringe assisted octanol–water partition microextraction coupled with high-performance liquid chromatography for simultaneous determination of neonicotinoid insecticide residues in honey. Talanta 139, 21–26. doi: 10.1016/j.talanta.2015.02.033
Vilian, A. E., Sivakumar, R., Huh, Y. S., Youk, J. H., and Han, Y.-K. (2018). Palladium supported on an amphiphilic triazine–urea-functionalized porous organic polymer as a highly efficient electrocatalyst for electrochemical sensing of rutin in human plasma. ACS Appl. Mater. Interfaces 10, 19554–19563. doi: 10.1021/acsami.8b00579
Wang, J., Jiao, C., Li, M., Wang, X., Wang, C., Wu, Q., et al. (2018a). Porphyrin based porous organic polymer modified with Fe 3 O 4 nanoparticles as an efficient adsorbent for the enrichment of benzoylurea insecticides. Microchim. Acta 185:36. doi: 10.1007/s00604-017-2542-3
Wang, J., Li, M., Jiao, C., Song, Y., Wang, C., Wu, Q., et al. (2017). Preparation of a magnetic porous organic polymer for the efficient extraction of phenylurea herbicides. J. Chromatogr. A 1519, 19–27. doi: 10.1016/j.chroma.2017.09.001
Wang, Q., Wu, J., Hao, L., Wu, Q., Wang, C., and Wang, Z. (2018b). Magnetic solid-phase extraction of benzoylurea insecticides by Fe3O4 nanoparticles decorated with a hyper-cross-linked porous organic polymer. J. Sep. Sci. 41, 3285–3293. doi: 10.1002/jssc.201800392
Wood, C. D., Tan, B., Trewin, A., Su, F., Rosseinsky, M. J., Bradshaw, D., et al. (2008). Microporous organic polymers for methane storage. Adv. Mater. 20, 1916–1921. doi: 10.1002/adma.200702397
Wu, Q., Li, Z., Wang, C., Wu, C., Wang, W., and Wang, Z. (2011). Dispersive solid-phase extraction clean-up combined with dispersive liquid–liquid microextraction for the determination of neonicotinoid insecticides in vegetable samples by high-performance liquid chromatography. Food Anal. Methods 4, 559–566. doi: 10.1007/s12161-011-9200-x
Xie, W., Han, C., Qian, Y., Ding, H., Chen, X., and Xi, J. (2011). Determination of neonicotinoid pesticides residues in agricultural samples by solid-phase extraction combined with liquid chromatography–tandem mass spectrometry. J. Chromatogr. A 1218, 4426–4433. doi: 10.1016/j.chroma.2011.05.026
Xiong, G., Wang, B.-B., You, L.-X., Ren, B.-Y., He, Y.-K., Ding, F., et al. (2019). Hypervalent silicon-based, anionic porous organic polymers with solid microsphere or hollow nanotube morphologies and exceptional capacity for selective adsorption of cationic dyes. J. Mater. Chem. A 7, 393–404. doi: 10.1039/C8TA07109H
Xiong, J., Li, H., Ma, X., and You, J. (2018). Synthesis and application of a novel solid-phase extraction adsorbent for multiresidue analysis of insecticides in water. J. Sep. Sci. 41, 525–533. doi: 10.1002/jssc.201700726
Xue, J., Zhu, X., Wu, X., Shi, T., Zhang, D., and Hua, R. (2019). Self-acidity induced effervescence and manual shaking-assisted microextraction of neonicotinoid insecticides in orange juice. J. Sep. Sci. 42, 2993–3001. doi: 10.1002/jssc.201900473
Yamamoto, A., Terao, T., Hisatomi, H., Kawasaki, H., and Arakawa, R. (2012). Evaluation of river pollution of neonicotinoids in Osaka City (Japan) by LC/MS with dopant-assisted photoionisation. J. Environ. Monitor. 14, 2189–2194. doi: 10.1039/c2em30296a
Zhang, C., Tian, D., Yi, X., Zhang, T., Ruan, J., Wu, R., et al. (2019). Occurrence, distribution and seasonal variation of five neonicotinoid insecticides in surface water and sediment of the Pearl Rivers, South China. Chemosphere 217, 437–446. doi: 10.1016/j.chemosphere.2018.11.024
Zhang, J., Wei, Y., Li, H., Zeng, E. Y., and You, J. (2017). Application of box–behnken design to optimize multi-sorbent solid phase extraction for trace neonicotinoids in water containing high level of matrix substances. Talanta 170, 392–398. doi: 10.1016/j.talanta.2017.04.031
Zhang, S., Yang, X., Yin, X., Wang, C., and Wang, Z. (2012). Dispersive liquid–liquid microextraction combined with sweeping micellar electrokinetic chromatography for the determination of some neonicotinoid insecticides in cucumber samples. Food Chem. 133, 544–550. doi: 10.1016/j.foodchem.2012.01.028
Zhang, Y., and Riduan, S. N. (2012). Functional porous organic polymers for heterogeneous catalysis. Chem. Soc. Rev. 41, 2083–2094. doi: 10.1039/C1CS15227K
Zhou, Y., Lu, X., Fu, X., Yu, B., Wang, D., Zhao, C., et al. (2018). Development of a fast and sensitive method for measuring multiple neonicotinoid insecticide residues in soil and the application in parks and residential areas. Anal. Chim. Acta 1016, 19–28. doi: 10.1016/j.aca.2018.02.047
Keywords: neonicotinoid insecticides, porphyrin based organic polymer, high performance liquid chromatography, adsorption mechanism surface water, magnetic solid-phase extraction
Citation: Selahle SK, Waleng NJ, Mpupa A and Nomngongo PN (2020) Magnetic Solid Phase Extraction Based on Nanostructured Magnetic Porous Porphyrin Organic Polymer for Simultaneous Extraction and Preconcentration of Neonicotinoid Insecticides From Surface Water. Front. Chem. 8:555847. doi: 10.3389/fchem.2020.555847
Received: 26 April 2020; Accepted: 17 August 2020;
Published: 16 September 2020.
Edited by:
Zhiyuan Xiong, The University of Melbourne, AustraliaReviewed by:
Mahendra Dashrath Shirsat, Dr. Babasaheb Ambedkar Marathwada University, IndiaDiego Centonze, University of Foggia, Italy
Copyright © 2020 Selahle, Waleng, Mpupa and Nomngongo. This is an open-access article distributed under the terms of the Creative Commons Attribution License (CC BY). The use, distribution or reproduction in other forums is permitted, provided the original author(s) and the copyright owner(s) are credited and that the original publication in this journal is cited, in accordance with accepted academic practice. No use, distribution or reproduction is permitted which does not comply with these terms.
*Correspondence: Philiswa N. Nomngongo, cG5ub21uZ29uZ29AdWouYWMuemE=