- 1Department of Chemistry, University at Buffalo, The State University of New York, Buffalo, NY, United States
- 2College of Chemistry, Beijing Normal University, Beijing, China
- 3Department of Chemistry, Hofstra University, Hempstead, NY, United States
Factors responsible for the persistent adoption of hairpin conformations by hybrid oligopeptides, each having a central β/α dipeptide segment flanked by aromatic γ-amino acid (γAr) residues, are probed. Our recent studies revealed that tetrapeptide 1 and 2, having central dipeptide segments consisting of β-alanine (β-Ala) and glycine (Gly), and L-β-homophenylalanine (L-β-homoPhe) and Gly residues, respectively, that are flanked by γAr residues, fold into well-defined, expanded β-turns with doubly H-bonded γAr residues. Replacing the γAr residues of 1 and 2 with L-Val and L-Leu residues results in tetrapetides 1′ and 2′ that fail to fold into defined conformations, which confirms the decisive role played by the H-bonded γAr residues in the promoting folding of 1 and 2. Attaching L-Val and L-Leu residues to the termini of 1 affords hexapeptide 1a. With an additional H-bond between its L-Val and L-Leu residues, peptide 1a folds into a hairpin with higher stability than that of 1, indicating that the expanded β-turn can nucleate and stabilize β-hairpin with longer β-strands. Attaching L-Val and L-Leu residues to the termini of 2 affords hexapeptide 2a. Substituting the L-β-homoPhe residue of 2a with a D-β-homoPhe residue gives hexapeptide 2b. Surprisingly, hexapeptide 2a fold into a hairpin showing the similar stability as those of tetrapeptides 1 and 2. Hexapeptide 2b, with its combination of a D-β-homoPhe residue and the L-Val/L-Leu pair, fold into a hairpin that is significantly more stable than the other hybrid peptides, demonstrating that a combination of hetero-chirality between the β-amino acid residue of the dipeptide loop and the α-amino acid residues of the β-strands enhances the stability of the resultant β-hairpin.
Introduction
As a major class of protein secondary structure, reverse turns provide sites of chain reversal, which results in the globular character of a protein (Smith and Pease, 1980; Milner-White and Poet, 1987). Reverse turns include the widely occurring two-residue β-turns (Wilmot and Thornton, 1988), along with the less prevalent γ-turns (Némethy and Printz, 1972) and α-turns (Pavone et al., 1996). β-Turns and β-hairpins are frequently found in hairpin loops of globular proteins and play a key role in protein folding (Marcelino and Gierasch, 2008). The design of discrete β-hairpins relies on the availability of type II′ β-turn of D-Pro-Gly (Haque et al., 1996; Karle et al., 1996; Haque and Gellman, 1997; Espinosa and Gellman, 2000; Syud et al., 2001; Aravinda et al., 2004) segment and type I′ β-turns of Asn-Gly (de Alba et al., 1997; Maynard and Searle, 1997; Simpson et al., 2005) and Aib-D-Ala (Aravinda et al., 2002) segments. Hairpins and reverse turns including β-turns play crucial roles in initiating the folding of peptides and proteins (Jäger et al., 2001; Rotondi and Gierasch, 2003; Du et al., 2004; Marcelino and Gierasch, 2008), and also possess in important biological functions, for example, as epitopes in protein–protein (Ripoll, 1992; Wilson and Stanfield, 1994; DeLano et al., 2000; Tyndall et al., 2005; Shukla and Sasidhar, 2015) and protein–nucleic acid (Churchill and Suzuki, 1989; Erard et al., 1990; Maynard and Searle, 1997; Shi et al., 1998; Leon et al., 2008) interactions. Our recent studies (Zhang et al., 2019; Tang et al., 2020) led to the discovery of a series of expanded β-turns sharing a β/α loop, i.e., a central dipeptide segment consisting of a β and α amino acid residue that is flanked by doubly H-bonded aromatic γ-amino acid (γAr) residues. This expanded β-turn represents a surprisingly resilient turn motif that allows the incorporation of different α and β amino acid residues (Tang et al., 2020). It was found that introducing various α amino acid residues into the β/α dipeptide loop results in β-hairpins with the same or slightly lower stabilities, while incorporating β amino acid residues enhances the stabilities of the resultant β-hairpins. In this study, we explore the role of the γAr residues in the folding of this series of hybrid peptides. The effects of additional α-amino acid residues added to the N- and C-termini of the hybrid tetrapeptides to the stabilities of the resultant folded structures. The combinations of chirality between the β-amino acid residue in the dipeptide loop and the terminal α-amino acid residues are also examined for its influence on the folding of the corresponding hybrid peptides.
Materials and Methods
Chemistry
Reagents and solvents were purchased from commercial sources and used without further purification. Column chromatography was carried out on silica gel (300~400 mesh). 1H NMR spectra were recorded at 400 MHz and 600 MHz on a Bruker-400 spectrometer and JEOL-400 and 600 spectrometers at ambient temperature. 13C NMR spectra were measured at 100 MHz and 150 MHz on the same spectrometers. Chemical shifts are reported in parts per million downfield from TMS (tetramethylsilane). Coupling constants in 1H NMR are expressed in Hertz. Electrospray ionization high resolution mass spectra (ESI-HRMS) were acquired using a waters LCT Premier XE spectrometer (Waters, Milford, MA, USA).
Computational Methods
The models for 2a and 2b were optimized with the revPBE-D3 (Zhang and Yang, 1998; Grimme et al., 2010) functional and dispersion correction using the Amsterdam Density Functional (ADF) (Fonseca Guerra et al., 1998; te Velde et al., 2001)1 software package. The triple-zeta with polarization functions (TZP) basis set was used while keeping the core 1s electrons fixed in the oxygen, nitrogen, and carbon atoms (van Lenthe and Baerends, 2003). The revPBE-D3 functional and dispersion correction were used due to its previous treatment of similar tetrapeptides (Zhang et al., 2019).
Results and Discussion
Synthesis
The synthesis of tetrapetides 1 and 2 has been reported by us (Zhang et al., 2019; Tang et al., 2020). Peptides 1′ and 2′ were prepared based on standard amide/peptide coupling.
Figure 1 shows the general steps and conditions for synthesizing hexapeptides 1a, 2a, and 2b. Coupling I and II, which were prepared by coupling the methyl ester of D- or L-β-homo-phenyalanine with 2-isopentyloxy-5-nitrobenzoic acid, and Boc-protected glycine with the methyl ester of 5-amino-2-isopentyloxybenzoic acid, results in oligomer III. Hydrolyzing the methyl ester gives IV, which is coupled with the L-leucine derived amide to give V. Subjecting V to catalytic hydrogenation results in VI, followed by coupling with acetyl-L-valine to give peptides 1a, 2a, and 2b. The detailed synthetic steps for preparing the intermediates and final products, along with the corresponding analytical data are included in the Supplementary Materials.
The Critical Role of Aromatic γ-Amino Acid Residues
Results from our recent study indicate that hybrid tetrapeptides 1 and 2, along with eight other homologous hybrid peptides (Tang et al., 2020), persistently fold into a doubly H-bonded hairpin conformation containing an expanded β-turn that is very resilient toward incorporating different α- and β-amino acid residues into the central β/α dipeptide loop. Such a turn motif is capable of accommodating a variety of β/α dipeptide sequences that otherwise could not be introduced into a β-turn. For example (Figure 2A), glycine and β-alanine, i.e., homoglycine, two conformationally most flexible α- and β-amino acid residues, are found in tetrapeptide 1 which adopts a well-defined hairpin conformation. Replacing the β-alanine residue of 1 with other β-amino acid residues having side chains results in hybrid tetrapeptides such as 2 that adopts a hairpin conformation with enhanced stability.
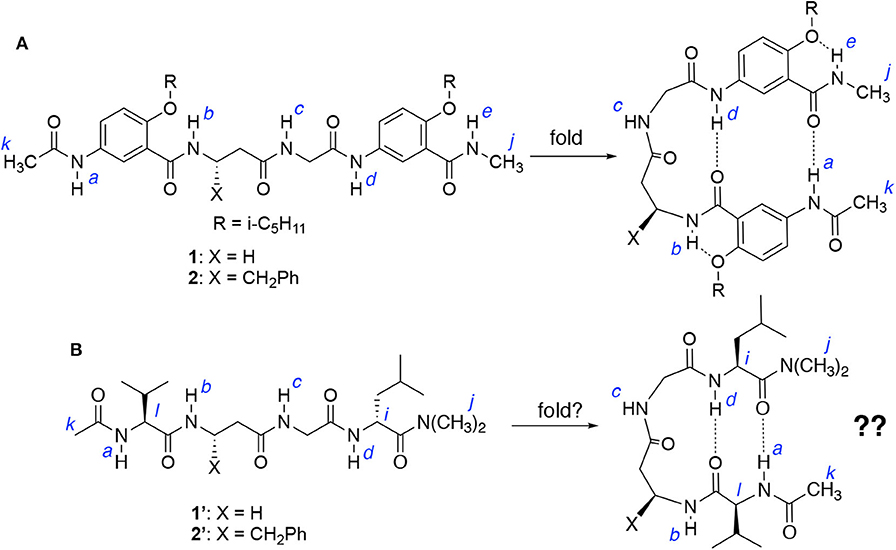
Figure 2. (A) Hybrid tetrapeptides 1 and 2 were found to fold into a hairpin conformation as shown. (B) Hybrid tetrapeptides 1′ and 2′ are designed to probe whether folded (hairpin) conformations could also be adopted.
Each of tetrapeptides 1 and 2, like other hybrid tetrapeptides of this series, has two aromatic γ-amino acid (γAr) residues flanking the central β/α dipeptide segment. The vital role of the γAr residues in driving the folding of 1 and 2 is further demonstrated by examining the folding of tetrapeptides 1′ and 2′ (Figure 2B), which share the same β/α dipeptide segments with 1 and 2, respectively, but the latter two have two α-amino acid residues, i.e., L-Val and L-Leu, that flank the β/α dipeptide segment. If folded, tetrapeptides 1′ and 2′ could also adopt doubly H-bonded hairpin conformations as shown in Figure 2B.
The 1H NMR spectra of 1 and 1′, and 2 and 2′ recorded at 25 mM were compared to those recorded at 1 mM in CDCl3. Table 1 shows the difference in the chemical shifts (ΔδNH) of the amide protons of the four peptides at the two concentrations. In the folded conformations of 1 and 2, protons a and d, like b and e, are intramolecularly H-bonded and exhibit either small upfield shifts or insignificant downfield shifts at high vs. low concentrations; while the signals of protons c, which are not intramolecularly H-bonded, shift noticeably downfield with increasing concentration (Tang et al., 2020). In contrast, all of the amide proton resonances of peptides 1′ and 2′ recorded at 25 mM show downfield shifts relative to those at 1 mM, with the signals of protons a, c, and d showing significant shifts, while those of protons b undergoing small shifts. These observations suggest that among the amide protons of 1′ and 2′, only protons b are intramolecularly H-bonded. The fact that protons a and d of 1′ and 2′ are not intramolecularly H-bonded indicates that 1′ and 2′ do not fold into the hairpin conformation as shown in Figure 2B.
The conformations of tetrapeptides 1′ and 2′ were examined with 2D (NOESY) spectroscopy (Supplementary Figures S4, S5). Except for NOEs between protons b and c, and c and d, the spectrum of 1′ reveals no NOEs between protons a and d, i and l, or j and k, which would exist if a hairpin conformation existed. The spectrum of 2′ contains an NOE between protons b and c, with no NOEs between protons a and d, i and l, or j and k being observed. The fact that only proton b of 1′ or 2′ is intramolecularly H-bonded, along with the absence of NOEs between other remote protons, suggests that 1′ and 2′, being derived from replacing the aromatic γ-amino acid residues 1 and 2 with L-Val and L-Leu residues, cannot fold into hairpin conformations.
The above observations indicate that the doubly H-bonded γAr residues are indispensable in driving the folding of 1 and 2 into hairpin conformations. Without the γAr residues, peptides 1′ and 2′, although capable of forming intramolecular H-bonds involving protons a and d, fail to adopt hairpin conformations. The critical role played by the γAr residues on stabilizing these novel hairpins relies on the effective H-bonding capabilities offered by these structural units (Gong, 2007), which provides the energetic driving force for the observed persistent folding of these hybrid peptides.
Triply H-Bonded β-Hairpins: The Folding of Hexapeptide 1a
Comparing tetrapeptides 1′ with 1, and 2′ with 2 revealed the critical importance of the doubly H-bonded γAr residues in ensuring the adoption of hairpin conformations by 1 and 2. Attaching additional amino acid residues to 1 or 2 results in a longer peptide that may fold into a hairpin with an enhanced stability due to the energetic contribution of added H-bond(s). Hexapeptide 1a (Figure 3), which is resulted from adding a pair of amino acid residues, L-Val and L-Leu, to the N and C termini of 1, respectively, were examined and compared to tetrapeptide 1.
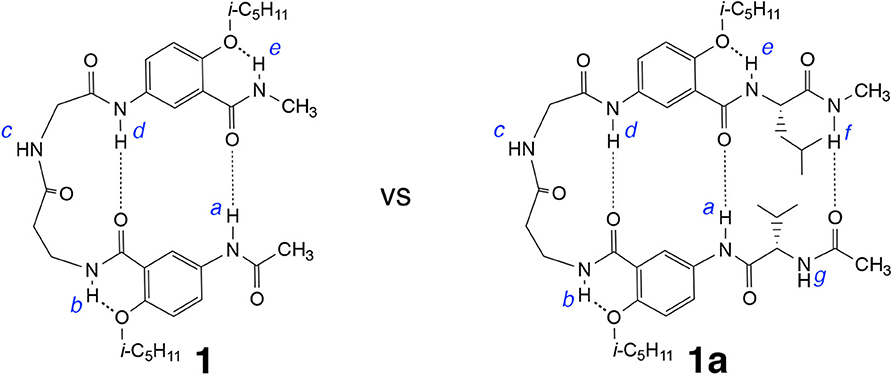
Figure 3. Hybrid hexapeptide 1a, derived from tetrapeptide 1, is expected to fold into a triply H-bonded hairpin conformation.
The 1H NMR spectrum of 1a recorded in CDCl3 at 25 °C contains well-dispersed signals (Supplementary Figure 1), indicating that 1a, like 1, exists as a single discrete species with a defined conformation. At 1 mM in CDCl3, the signals of protons a and d of 1a appear at 10.06 and 9.53 ppm, respectively, while the same protons of 1 are found at 9.65 and 9.39 ppm. The downfield shifts of protons a and d of 1a relative to those of 1 at the same concentration indicate that the H-bonds involving protons a and d of the former are stronger than those of the latter, which suggests that, hexapeptide 1a, with one additional H-bond contributed by the L-Val/L-Leu pair, may very likely fold into a triply H-bonded conformation that is more stable than the doubly H-bonded hairpin of 1.
Comparing the difference in the chemical shifts of amide protons at 25 mM and 1 mM in CDCl3 reveals that the amide proton resonances of 1a follow the same trend as shown by those of 1 (Supplementary Table 1). The signal of proton c of 1 or 1a undergoes the largest downfield shift (~0.7 ppm) upon increasing the concentration from 1 to 25 mM, suggesting that proton c of 1a, like that of 1 (Tang et al., 2020), is intermolecularly H-bonded. In contrast, the signals of amide protons a, b, d, and e of 1a exhibit very small (<0.01 ppm) upfield shifts, indicating that protons a and d, like b and e, are intramolecularly H-bonded. These observations suggest that hexapeptide 1a, like tetrapeprtide 1, folds into a hairpin conformation that is stabilized by H-bonds involving protons a and d, and further reinforced by a H-bond involving proton f. Indeed, proton f undergoes an upfield shift of 0.274 ppm from 1 to 25 mM, suggesting that it is intramolecularly H-bonded.
The H-bonding interactions involving protons a and d were further examined by monitoring the chemical shifts of amide protons a and d of peptides 1 and 1a in CDCl3 containing DMSO-d6. Interestingly, the signals of protons a and d shift differently with increasing ratios of DMSO (Figure 4). The resonances of protons a of both 1 and 1a first shift upfield and then move downfield with as the ratio of DMSO increases (Figure 4A). In contrast, the signals of protons d of both 1 and 1a show overall linear downfield shifts with increasing DMSO ratio (Figure 4B).
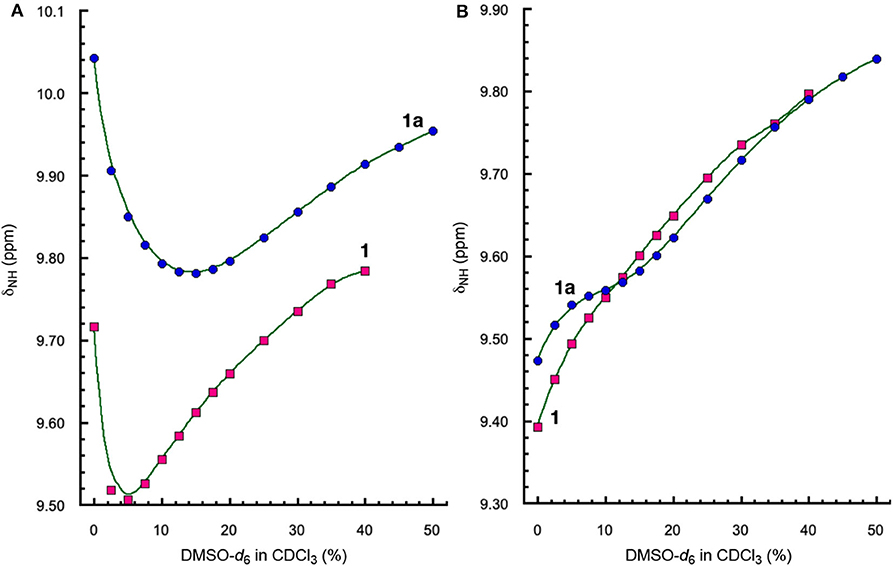
Figure 4. Plots of chemical shifts (δNH) of amide protons (A) a and (B) d, of 1 (5 mM) and 1a (5 mM) versus percent of DMSO-d6 in CDCl3.
The different shifts of amide protons a and d toward increasing solvent polarity can be explained by the folded conformations of 1 and 1a (Figure 3). Proton d is involved in an “intraturn”, N–H(i) → O=C(i −3) hydrogen bond that is part of the 11-atom, intramolecularly H-bonded ring that constitutes the expanded β-turn in the hairpin conformation of 1 or 1a. Such a H-bond, with a H•••O distance of over 2.0 Å, is slightly longer and thus weaker than typical H-bonds. As a result, proton d of 1 or 1d is more accessible to solvent molecules than proton a. With increasing proportion of DMSO, proton d becomes increasingly H-bonded with DMSO molecules, which results in the destabilization of the folded (hairpin) conformation. Such an overall destabilization of the hairpin conformation in turn weakens the H-bond involving proton a, which is reflected by the initial upfield shift of the signal of proton a. As the H-bond becomes further weakened, proton a also becomes more exposed to solvent molecules and engages in increasing H-bonding interaction with DMSO molecules, which leads to the downfield shift of its signal.
Comparing the shifts of amide protons a of 1 and 1a reveals another interesting trend. As show in Figure 4A, the upfield shift shown by the signal of proton a of 1 is reversed at ~5% DMSO, while that of 1a is reversed at 15% DMSO, indicating that proton a of 1a is more resistant toward increasing solvent polarity than that of 1, i.e., the H-bond involving proton a in 1a is stronger than that in 1. The stronger H-bond involving proton a of 1a is mostly like due to the higher overall stability of the hairpin conformation of 1a than that of 1. The enhanced stability shown by the folded structure of 1a can be explained by the energetic contribution of H-bonding involving proton f and the terminal amide C = O group.
The folded conformation of hybrid peptide 1a is confirmed by 2D NOESY spectra. As shown in Figure 5, the NOEs observed with 1a include those between protons a and m, and h and n, which indicate the H-bonded alignment of the two aromatic γ-amino acid residues and the L-Val and L-Leu residues. In addition, NOEs between protons c and j, c and k, c and d, and d and l demonstrate the presence of a well-defined loop. These multiple NOEs confirm that hybrid peptide 1a folds into a hairpin conformation similar to those observed with hybrid tetrapeptides 1 and 2 (Tang et al., 2020).
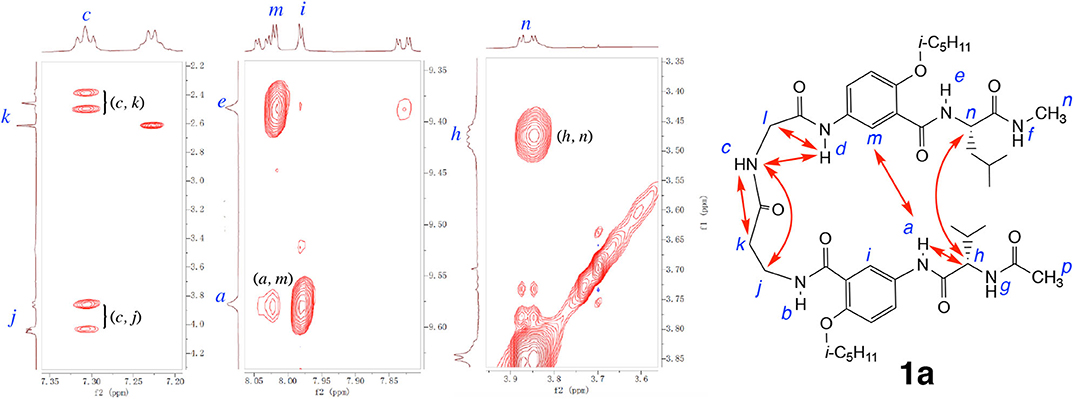
Figure 5. Partial NOESY spectra of hexapeptide 1a (5 mM) in 1, 1, 2, 2-tetrachloroethane-d2 containing 5% DMSO-d6 (600 MHz, 298 K, mixing time: 300 ms). Major NOEs are indicated by double-headed arrows in the structure. Partial NOESY spectra showing NOEs between protons a and h, c and d, and d and l are included in the Supplementary Materials.
Role of Residue Chirality on Hairpin Folding
Results from our recent study demonstrate that replacing the β-alanine residue of 1 with other β-amino acid residues such as β-homoPhe gives hybrid tetrapeptides including 2 that fold into expanded β-turns with enhanced stabilities (Tang et al., 2020). It is expected that the β-turn of 2, like that of 1, should also accommodate additional amino acid residues, resulting in longer β-hairpins. Attaching L-Val and L-Leu residues to the N and C termini of 2 results in hexapeptide 2a. Replacing the L-β-homoPhe residue of 2a with D-β-homoPhe gives hexapeptide 2b (Figure 6). By comparing the folding of 2, 2a, and 2b, and the stabilities of the folded structures, we intend to probe the compatibility of the chirality of the β-homoPhe residue in the dipeptide segment of the expanded β-turn with that of the two terminal L-α-amino acid residues.
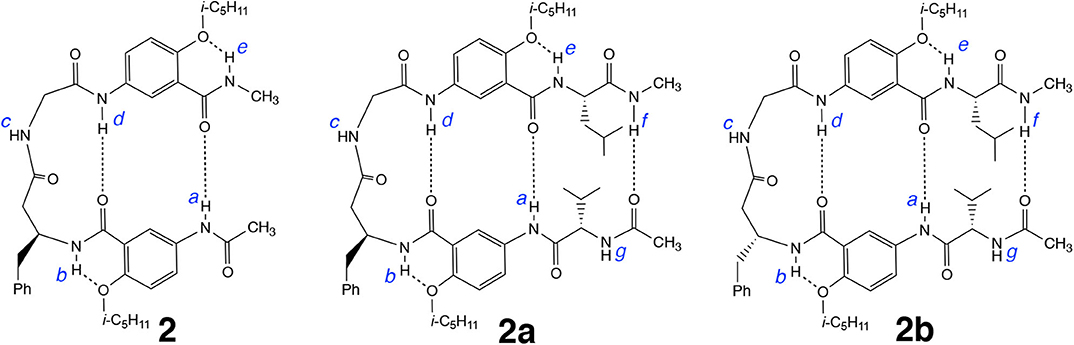
Figure 6. Hexapeptide 2a, derived from tetrapeptide 2, and hexapeptide 2b, derived from 2a, are expected to also adopt hairpin conformations.
Table 2 lists the difference in the chemical shifts of amide protons a, b, c, d, and e of peptides 2, 2a, and 2b, along with the chemical shifts of amide protons f and g of 2a and 2b, measured at 25 mM and 1 mM. The amide protons of 2 and 2b show the same overall change in their chemical shifts measured at high and low concentrations, suggesting that 2b, like 2, also folds into a well-defined hairpin conformation. In contrast, the amide proton resonances of 2a show noticeable difference in that proton c does not undergo as large a downfield shift as those shown by protons c of 2 and 2b. In addition, proton d of 2a shows a significant downfield shift from 1 to 25 mM, which contrasts the negligible shifts observed with protons d of 2 and 2b. These observations imply that, compared to those of 2 and 2b, proton d of 2a is more exposed and is available for intermolecular H-bonding, which in turn suggests that the expanded β-turn of 2a involving H-bonded proton d might be partially twisted. Such a partial twisting or deformation may be resulted from the incompatibility of the L-homoPhe with the terminal L-Val and L-Leu residues of 2a.
To assess the relative stabilities of the folded conformations of 2, 2a, and 2b, the chemical shifts of protons a and d of these three hybrid peptides in CDCl3 containing DMSO-d6 were compared. Similar to what is observed with 1 and 1a (Figure 4), the signals of protons a of 2, 2a and 2b also first shift upfield with increasing proportion of DMSO, followed by shifting downfield as the ratio of DMSO further increases (Figure 7A). The resonances of protons a of the three peptides, however, undergo transitions from upfield to downfield shifts at different percent of DMSO, with peptide 2 showing its transition at ~6% DMSO, 2a at ~8% DMSO, and 2b at ~25% DMSO. Thus, proton a of 2b is the least responsive toward increasing solvent polarity, which indicates that the folded conformation of 2b is the most stable among those of the three peptides. The high stability of folded 2b is also demonstrated by the upfied and then down field shift of proton d of this peptide (Figure 7B), which contrasts the consistent downfield shifts observed with the resonances of protons d of the other hybrid peptides, indicating that the H-bond involving proton d is greatly enhanced in the folded conformation of 2b.
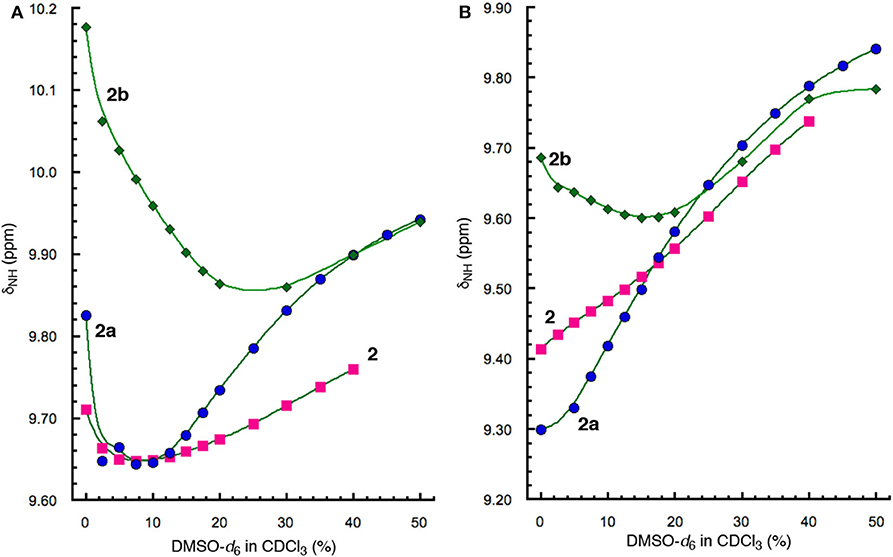
Figure 7. Plots of chemical shifts (δNH) of amide protons (A) a and (B) d, of 2 (5 mM), 2a (5 mM), and 2b (5 mM) vs. percent of DMSO-d6 in CDCl3.
In contrast, the stability of folded hexapeptide 2a is similar to that of tetrapeptide 2 and is much less stable than those of hexapeptides 2b and 1a (Figures 4A, 7A). The strong H-bonding and high stability of 2b, and the much lower stability of 2a, suggest that D-homoPhe residue in the H-bonded loop of 2b is more compatible with the L-Val and L-Leu residues, while L-homoPhe of 2a is much less compatible. Therefore, a hetero-chiral combination of the β-amino acid residue in the expanded β-turn and the α-amino acid residues in the β-strands seems to favor the nucleation and stabilization of β-hairpins. For example, an expanded β-strand with a D-β-amino acid residue should promote oligopeptides of L-α-amino acids to pair into a β-sheet.
NOESY spectra of 2a and 2b provide additional insights into the folding of these two hexapeptides. As shown in Figure 8A, the NOEs observed with 2a include those between protons d and i, and h and n, which indicate the alignment of the two γAr residues and the L-Val and L-Leu residues. In addition, NOEs between protons c and j, c and k, c and d, and d and l demonstrate the presence of the H-bonded loop. The observed NOEs suggest that hybrid peptide 2a adopts an overall hairpin conformation that includes a H-bonded loop along with the expected alignment of γAr and α-amino acid residues. In comparison to those of 2a, NOEs with significantly stronger intensities are revealed by the NOESY spectrum of 2b (Figure 8B). Strong NOEs that indicate the H-bonded alignment of the two γAr residues and the L-Val/L-Leu residues are clearly observed between protons a and m, a and n, d and i, and h and n. NOEs between protons c and j, c and k, c and d, d and j, and d and l are consistent with the presence of the 11-atom H-bonded ring that constitutes the expanded β-turn. The different numbers and strengths of the NOEs detected for 2a and 2b are consistent with the above conclusion on the different stabilities of the two folded structures. The numerous strong NOEs observed with 2b is consistent with a compact, tightly folded conformation.
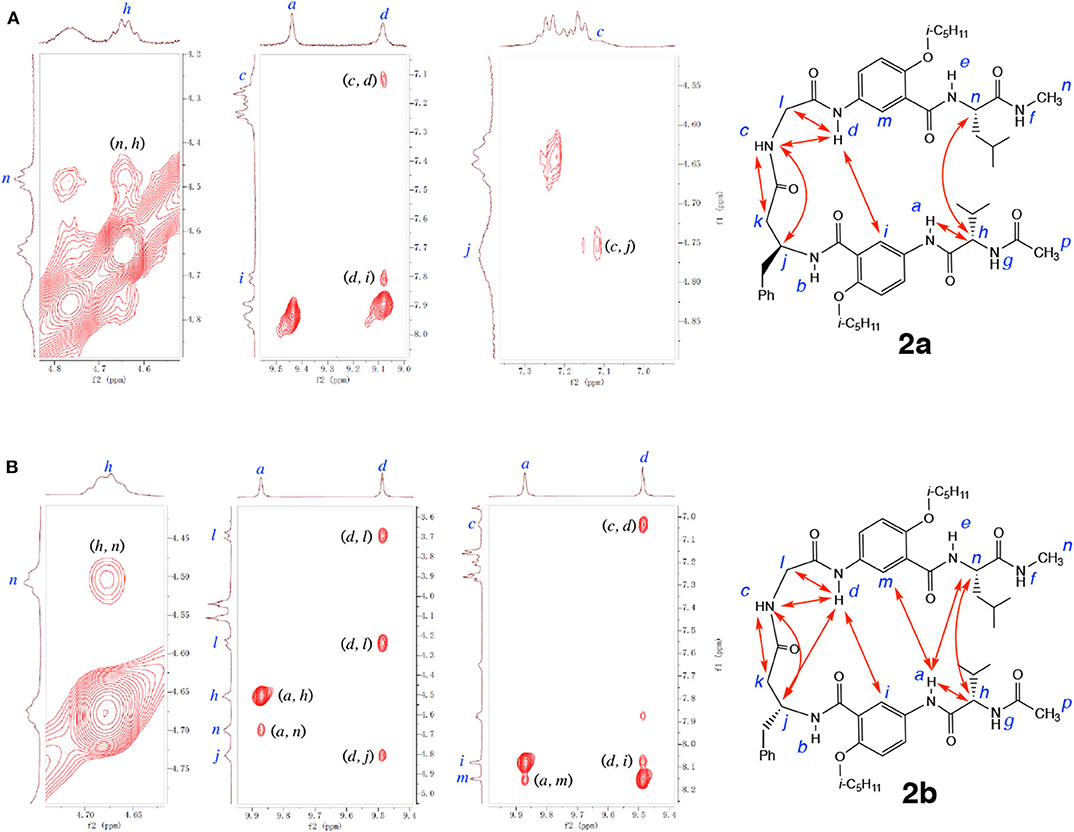
Figure 8. Partial NOESY spectra of (A) hexapeptide 2a (5 mM), and (B) hexapeptide 2b (5 mM) in 1, 1, 2, 2-tetrachloroethane-d2 containing 5% DMSO-d6 (600 MHz, 298 K, mixing time: 300 ms). Major NOEs are indicated by double-headed arrows in the structures. Partial NOESY spectra showing NOEs between protons a and h, c and k, and d and l of 2a, and between protons c and k, and c and j of 2b, are included in the Supplementary Materials.
Finally, hexapeptides 2a and 2b were computationally optimized and compared. The optimized structures are shown in Figure 9. Modeling of 2a with revPBE-D3 revealed N-H•••O hydrogen bond distances for protons a, d, and f to be 1.79, 2.08, and 1.91 Å, respectively. Measurements of the N-H•••O hydrogen bond distances in 2b show that H-bonds involving protons a, d, and f shorten to 1.78, 1.98, and 1.88 Å, respectively, which suggest strengthened H-bonds. The strengthening of the hydrogen bonds in 2b relative to 2a, assessed through the N-H•••O hydrogen bond distances, coupled with 2a having a larger buckling within the turnabout of the L-homoPhe residue relative to 2b supports the experimental findings that 2b holds a greater overall stability than 2a.
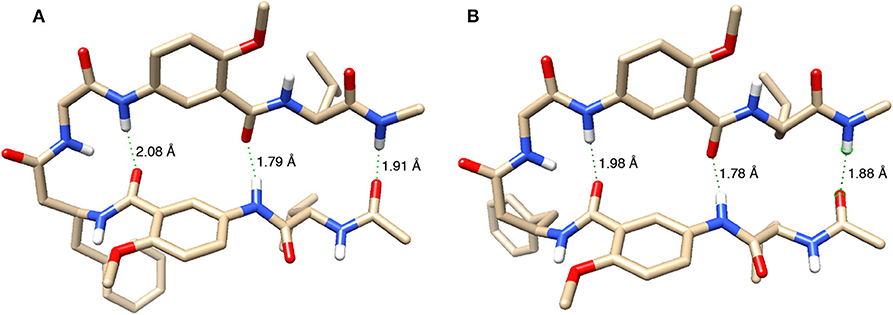
Figure 9. Energy-minimized structures of the hairpin conformations of (A) 2a and (B) 2b. Except for amide hydrogens, all other hydrogen atoms are removed for clarity. Three N-H•••O distances are indicated for each structure.
Conclusion
Results from this study have demonstrated that, by comparing with tetrapeptides consisting of α-amino acid residues that fail to fold into hairpin conformations, hybrid tetrapeptides sharing a general structure with a central β/α dipeptide segment flanked by doubly H-bonded γAr residues reliably fold into hairpins, which demonstrate the decisive role played by the γAr residues in driving the folding of these short peptides. Adding additional α-amino acid residues to the hybrid tetrapeptides results hexapeptides that fold into hairpins with enhanced stabilities, indicating that the expanded β-turns formed by the tetrapetides can effectively nucleate and stabilize longer hairpins. A combination of hetero-chirality between the β-amino acid residue, i.e., L- and D-homoPhe residues, in the dipeptide loop and the terminal α-amino acid residues, i.e., L-Val and L-Leu, strongly promotes the folding of the hexapeptides, based on which longer peptide strands should be aligned into defined β-sheets.
Data Availability Statement
The raw data supporting the conclusions of this article will be made available by the authors, without undue reservation, to any qualified researcher.
Author Contributions
The project was designed, coordinated, and supervised by BG with assistance from YZ. Synthesis of the hybrid peptides was performed by QT and YZ, under supervision of BG, Z-LL, and RL. The measurement of spectroscopic data was performed and analyzed by YZ and QT. The molecular modeling study was designed by DM and YZ, supervised by EZ and BG, and executed mainly by DM. BG analyzed and compiled the data and prepared the manuscript with support of YZ, and also of QT, DM, and EZ. The final manuscript was read and approved by all authors.
Funding
We are gratefully acknowledge financial support from the American Chemical Society—Petroleum Research Fund (PRF# 70641-ND, to BG), the Silbert Fellowship from the University at Buffalo, SUNY (to DM), the Center of Computational Research, CCR, at the University at Buffalo (to EZ and DM), and the Natural Science Foundation of China (91227109 and 21778012 to Z-LL, 21801020 to RL).
Conflict of Interest
The authors declare that the research was conducted in the absence of any commercial or financial relationships that could be construed as a potential conflict of interest.
Acknowledgments
This work was supported by the Center of Computational Research, CCR, at the University at Buffalo (http://hdl.handle.net/10477/79221).
Supplementary Material
The Supplementary Material for this article can be found online at: https://www.frontiersin.org/articles/10.3389/fchem.2020.530083/full#supplementary-material
Footnotes
1. ^ADF, SCM, Theoretical Chemistry, Vrije Universiteit, Amsterdam, Netherlands. Available online at: http://www.scm.com.
References
Aravinda, S., Harini, V. V., Shamala, N., Das, C., and Balaram, P. (2004). Structure and assembly of designed β-hairpin peptides in crystals as models for β-sheet aggregation. Biochemistry 43, 1832–1846. doi: 10.1021/bi035522g
Aravinda, S., Shamala, N., Rajkishore, R., Gopi, H. N., and Balaram, P. (2002). A crystalline beta-hairpin peptide nucleated by a type I' Aib-D-Ala beta-turn: evidence for cross-strand aromatic interactions. Angew. Chem. Int. Ed. Engl. 41, 3863–3865. doi: 10.1002/1521-3773(20021018)41:20<3863::AID-ANIE3863>3.0.CO;2-A
Churchill, M. E. A., and Suzuki, M. (1989). 'SPKK' motifs prefer to bind to DNA at A/T-rich sites. EMBO J. 8, 4189–4195. doi: 10.1002/j.1460-2075.1989.tb08604.x
de Alba, E., Jiménez, M. A., and Rico, M. (1997). Turn residue sequence determines β-hairpin conformation in designed peptides. J. Am. Chem. Soc. 119, 175–183. doi: 10.1021/ja962325e
DeLano, W. L., Ultsch, M. H., de Vos, A. M., and Wells, J. A. (2000). Convergent solutions to binding at a protein–protein interface. Science 287, 1279–1283. doi: 10.1126/science.287.5456.1279
Du, D., Zhu, Y., Huang, C. Y., and Gai, F. (2004). Understanding the key factors that control the rate of β-hairpin folding. Proc. Natl. Acad. Sci. U.S.A. 101, 15915–15920. doi: 10.1073/pnas.0405904101
Erard, M., Lakhdar-Ghazal, F., and Amalric, F. (1990). Repeat peptide motifs which contain beta-turns and modulate DNA condensation in chromatin. Eur. J. Biochem. 191, 19–26. doi: 10.1111/j.1432-1033.1990.tb19088.x
Espinosa, J. F., and Gellman, S. H. (2000). A designed β-hairpin containing a natural hydrophobic cluster. Angew. Chem. Int. Ed. 39, 2330–2333. doi: 10.1002/1521-3773(20000703)39:13<2330::AID-ANIE2330>3.0.CO;2-C
Fonseca Guerra, C., Snijders, J. G., te Velde, G., and Baerends, E. J. (1998). Towards an order-N DFT method. Theor. Chem. Acc. 99, 391–403. doi: 10.1007/s002140050353
Gong, B. (2007). Engineering hydrogen-bonded duplexes. Polym. Int. 56, 436–443. doi: 10.1002/pi.2175
Grimme, S., Antony, J., Ehrlich, S., and Krieg, H. (2010). A consistent and accurate ab initio parametrization of density functional dispersion correction (DFT-D) for the 94 elements H-Pu. J. Chem. Phys. 132:154104. doi: 10.1063/1.3382344
Haque, T. S., and Gellman, S. H. (1997). Insights on β-hairpin stability in aqueous solution from peptides with enforced type I' and type II' β-turns. J. Am. Chem. Soc. 119, 2303–2304. doi: 10.1021/ja963653h
Haque, T. S., Little, J. S., and Gellman, S. H. (1996). Stereochemical requirements for β-hairpin formation: model studies with four-residue peptides and depsipeptides. J. Am. Chem. Soc. 118, 6975–6985. doi: 10.1021/ja960429j
Jäger, M., Nguyen, H., Crane, J. C., Kelly, J. W., and Gruebele, M. (2001). The folding mechanism of a β-sheet: the WW domain. J. Mol. Biol. 311, 373–393. doi: 10.1006/jmbi.2001.4873
Karle, I. L., Awasthi, S. K., and Balaram, P. (1996). A designed β-hairpin peptide in crystals. Proc. Natl. Acad. Sci. U.S.A. 93, 8189–8193. doi: 10.1073/pnas.93.16.8189
Leon, R. P., Tecklenburg, M., and Sclafani, R. A. (2008). Functional conservation of β-hairpin DNA binding domains in the Mcm protein of Methanobacterium thermoautotrophicum and the Mcm5 protein of Saccharomyces cerevisiae. Genetics 179, 1757–1768. doi: 10.1534/genetics.108.088690
Marcelino, A. M. C., and Gierasch, L. M. (2008). Roles of beta-turns in protein folding: from peptide models to protein engineering. Biopolymers 89, 380–391. doi: 10.1002/bip.20960
Maynard, A. J., and Searle, M. S. (1997). NMR structural analysis of a β-hairpin peptide designed for DNA binding. Chem. Commun. 1997, 1297–1298. doi: 10.1039/a702593i
Milner-White, E. J., and Poet, R. (1987). Loops, bulges, turns and hairpins in proteins. Trends Biochem. Sci. 12, 189–192. doi: 10.1016/0968-0004(87)90091-0
Némethy, G., and Printz, M. P. (1972). The γ turn, a possible folded conformation of the polypeptide chain. Comparison with the β turn. Macromolecules 5, 755–758. doi: 10.1021/ma60030a017
Pavone, V., Gaeta, G., Lombardi, A., Nastri, F., Maglio, O., Isernia, C., et al. (1996). Discovering protein secondary structures: classification and description of isolated α-turns. Biopolymers 38, 705–721. doi: 10.1002/(SICI)1097-0282(199606)38:6%3C705::AID-BIP3%3E3.0.CO;2-V
Ripoll, D. R. (1992). Conformational study of a peptide epitope shows large preferences for β-turn conformations. Int. J. Pept. Protein Res. 40, 575–581. doi: 10.1111/j.1399-3011.1992.tb00443.x
Rotondi, K. S., and Gierasch, L. M. (2003). Role of local sequence in the folding of cellular retinoic acid binding protein I: structural propensities of reverse turns. Biochemistry 42, 7976–7985. doi: 10.1021/bi034304k
Shi, Y. G., Wang, Y. F., Jayaraman, L., Yang, H. J., Massague, J., and Pavletich, N. P. (1998). Crystal structure of a Smad MH1 domain bound to DNA: insights on DNA binding in TGF-β signaling. Cell 94, 585–594. doi: 10.1016/S0092-8674(00)81600-1
Shukla, R. T., and Sasidhar, Y. U. (2015). Conformational dynamics of a short antigenic peptide in its free and antibody bound forms gives insight into the role of β-turns in peptide immunogenicity. Proteins 83, 1352–1367. doi: 10.1002/prot.24831
Simpson, E. R., Meldrum, J. K., Bofill, R., Crespo, M. D., Holmes, E., and Searle, M. S. (2005). Engineering enhanced protein stability through β-turn optimization: insights for the design of stable peptide β-hairpin systems. Angew. Chem. Int. Ed. 44, 4939–4944. doi: 10.1002/anie.200500577
Smith, J. A., and Pease, L. G. (1980). Reverse turns in peptides and proteins. CRC Crit. Rev. Biochem. 8, 315–399. doi: 10.3109/10409238009105470
Syud, F. A., Stanger, H. E., and Gellman, S. H. (2001). Interstrand side chain–side chain interactions in a designed β-hairpin: significance of both lateral and diagonal pairings. J. Am. Chem. Soc. 123, 8667–8677. doi: 10.1021/ja0109803
Tang, Q., Zhong, Y. L., Miller, D. P., Liu, R., Zurek, E., Lu, Z. L., et al. (2020). Reverse turn foldamers: an expanded β-turn motif reinforced by double hydrogen bonds. Org. Lett. 22, 1003–1007. doi: 10.1021/acs.orglett.9b04547
te Velde, G., Bickelhaupt, F. M., van Gisbergen, S. J. A., Fonseca Guerra, C., Baerends, E. J., Snijders, J. G., et al. (2001). Chemistry with ADF. J. Comp. Chem. 22, 931–967. doi: 10.1002/jcc.1056
Tyndall, J. D. A., Pfeiffer, B., Abbenante, G., and Fairlie, D. P. (2005). Over one hundred peptide-activated G protein-coupled receptors recognize ligands with turn structure. Chem. Rev. 105, 793–826. doi: 10.1021/cr040689g
van Lenthe, E., and Baerends, E. J. (2003). Optimized slater-type basis sets for the elements 1–118. J. Comp. Chem. 24, 1142–1156. doi: 10.1002/jcc.10255
Wilmot, C. M., and Thornton, J. M. (1988). Analysis and prediction of the different types of beta-turn in proteins. J. Mol. Biol. 203, 221–232. doi: 10.1016/0022-2836(88)90103-9
Wilson, I. A., and Stanfield, R. L. (1994). Antibody-antigen interactions: new structures and new conformational changes. Curr. Opin. Struct. Biol. 4, 857–867. doi: 10.1016/0959-440X(94)90267-4
Zhang, Y., and Yang, W. (1998). Comment on “Generalized gradient approximation made simple”. Phys. Rev. Lett. 80:890. doi: 10.1103/PhysRevLett.80.890
Keywords: hybrid peptide, foldamer, hydrogen bond, β-turn, unnatural amino acid, β-hairpin
Citation: Zhong Y, Tang Q, Miller DP, Zurek E, Liu R, Lu Z-L and Gong B (2020) Major Factors for the Persistent Folding of Hybrid α, β, γ-Hybrid Peptides Into Hairpins. Front. Chem. 8:530083. doi: 10.3389/fchem.2020.530083
Received: 29 January 2020; Accepted: 31 August 2020;
Published: 29 September 2020.
Edited by:
Juan Del Valle, University of South Florida, United StatesReviewed by:
Jianfeng Cai, University of South Florida, United StatesLijiang Yang, Peking University, China
Copyright © 2020 Zhong, Tang, Miller, Zurek, Liu, Lu and Gong. This is an open-access article distributed under the terms of the Creative Commons Attribution License (CC BY). The use, distribution or reproduction in other forums is permitted, provided the original author(s) and the copyright owner(s) are credited and that the original publication in this journal is cited, in accordance with accepted academic practice. No use, distribution or reproduction is permitted which does not comply with these terms.
*Correspondence: Bing Gong, YmdvbmcmI3gwMDA0MDtidWZmYWxvLmVkdQ==; Zhong-Lin Lu, bHV6bCYjeDAwMDQwO2JudS5lZHUuY24=
†These authors have contributed equally to this work