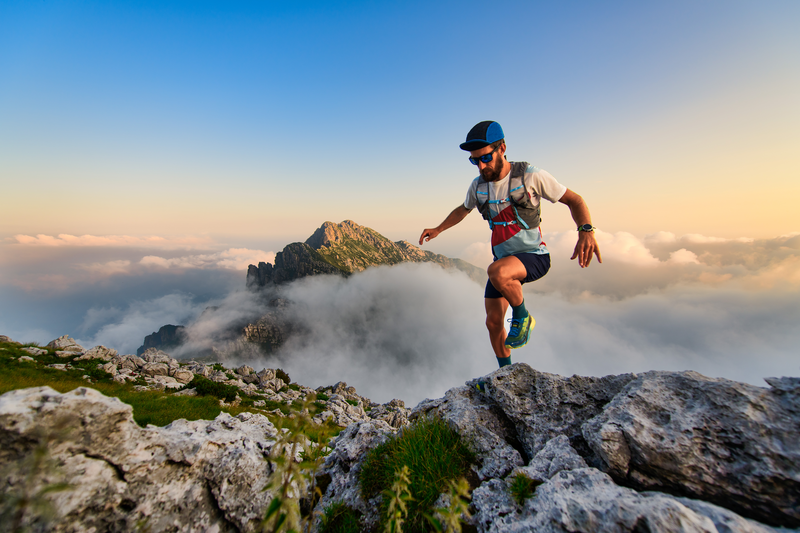
94% of researchers rate our articles as excellent or good
Learn more about the work of our research integrity team to safeguard the quality of each article we publish.
Find out more
REVIEW article
Front. Chem. , 24 July 2020
Sec. Electrochemistry
Volume 8 - 2020 | https://doi.org/10.3389/fchem.2020.00635
This article is part of the Research Topic Low-Cost Electrochemical Energy Storage Devices: Zinc-/Sodium-Ion Batteries View all 9 articles
Sodium ion batteries (SIBs) are one of the most potential alternative rechargeable batteries because of their low cost, high energy density, high thermal stability, and good structure stability. The cathode materials play a crucial role in the cycling life and safety of SIBs. Among reported cathode candidates, Na3V2(PO4)3 (NVP), a representative electrode material for sodium super ion conductor, has good application prospects due to its good structural stability, high ion conductivity and high platform voltage (~3.4 V). However, its practical applications are still restricted by comparatively low electronic conductivity. In this review, recent progresses of Na3V2(PO4)3 are well summarized and discussed, including preparation and modification methods, electrochemical properties. Meanwhile, the future research and further development of Na3V2(PO4)3 cathode are also discussed.
Lithium-ion battery (LIB), a kind of rechargeable battery, has been designed and modified to power portable electronic equipment, electric vehicle and even energy storage power station because of its high energy density, high voltage, and environmentally friendly (Goodenough and Park, 2013; Song et al., 2014a; Han et al., 2018; Yi T. F. et al., 2018; Fang R. et al., 2019; Li et al., 2019; Fang et al., 2020; Nie et al., 2020a,b; Wang et al., 2020). However, large-scale energy storage applications of LIBs could be hindered by the high cost of lithium minerals (Jiang et al., 2020; Yi et al., 2020). Hence, finding an alternative and sustainable electrochemical battery is necessary (Yang et al., 2011; Yabuuchi et al., 2014; Liu et al., 2018; Mao et al., 2018). Among the optional energy storage systems, sodium ion batteries (SIBs) have strongly caught researcher's attentions on account of abundant sodium resources, high energy storage capacities and high electrochemical activity (Komaba et al., 2011; Lee et al., 2011; Ponrouch et al., 2012; Tepavcevic et al., 2012; Chang et al., 2013; Slater et al., 2013; Farbod et al., 2014; Li W. et al., 2014; Xie et al., 2014; Zhong et al., 2016; Zhu et al., 2016; Li and Zhou, 2018; Nayak et al., 2018; Song et al., 2018; Vaalma et al., 2018). Though SIBs have similar structure with lithium ion batteries (Figure 1) (Li et al., 2013; Palomares et al., 2013; Pan et al., 2013; Kundu et al., 2015; Hwang et al., 2017; Zhao, 2019), Na ion has lager radium than lithium ion and easily coordinate in crystalline materials. Therefore, exploring appropriate host materials or other high energy density cathode materials are necessary for the developing of SIBs.
Suitable cathode materials should allow rapid Na-ion transport but also maintain structural stability and against the structural distortion/volume change in the process of Na ion extraction/insertion (Qi et al., 2015; Fang et al., 2016; Lee et al., 2016; Liu et al., 2016). The cathode materials of SIBs mainly include lamellar materials (Komaba et al., 2010; Kim et al., 2011, 2012; Liao et al., 2013; Tu et al., 2017; Xiao Y. et al., 2018), polyanionic materials (Ong et al., 2011; Fang et al., 2017) and polymer materials (Hwang et al., 2017; Deng et al., 2018). Among them, layered NaxMnO2 (Caballero et al., 2002) and NaxCoO2 (Samin et al., 2012), as well as phosphates-based NaMPO4 (M = Fe, Co, Ni, Mn) (Oh et al., 2012; Zhu et al., 2012; Hasa et al., 2014) have been deeply studied. Especially, sodium super ion conductor (NASICON) structured composite, such as Na3V2(PO4)3 (NVP) is likely to be the best candidate, because it has high theoretical energy storage capacities (117.6 mAh g−1), and rich Na-ion transport channels resulting from its open three-dimensional (3D) framework. However, the NVP has low electronic conductivity, which is not good for the migration of electrons. In order to solve this issue, many efforts have been done. For example, preparing nano-scaled Na3V2(PO4)3 to reduce the diffusion path of Na+ and accelerate its transportation. In addition, coating NVP with conductive carbon/polymer materials, or modifying Na3V2(PO4)3 with heterogeneous elements are also valid means to improve the electric conductivity of NVP.
In this minireview, the recent progresses of NVP are well summarized and discussed, including preparation methods, modification means (nanostructure, carbon coating, element doping) and their effects on the electrochemical property of NVP cathode.
Served as a greatly hopeful cathode material of SIBs, NVP crystallizes have trigonal system and belong to R-3c space group. As shown in Figure 2, VO6 octahedra and PO4 tetrahedra interlink mutually to construct a 3D [V2(PO4)3] frame via sharing corners (Kabbour et al., 2011; Kang et al., 2012; Shen et al., 2015a; Lavela et al., 2018), in which sodium ion occupies two different positions of Na(1) and Na(2), respectively. The desorption process of sodium ion relates to the transformation from Na3V2(PO4)3 to NaV2(PO4)3, it is generally considered that all the outer sodium ions come from the Na(2) position while the Na(1) position is unchanged. Though two sodium are stripped, the frame of NVP can still be maintained due to the strong covalent effect of (PO4)3−, leading to a high capacity of 117.6 mAh g−1 (Zheng et al., 2018).
Synthetic methods play an important role in controlling the morphology and particle size of electrode materials, which will further affect their electrochemical performance. Several approaches to synthesize Na3V2(PO4)3 electrode materials for SIBs, such as sol-gel method (Lim et al., 2012; Pivko et al., 2012; Li et al., 2015a; Wang S. Y. et al., 2015; Klee et al., 2016a; Song et al., 2016), hydrothermal method (Li H. et al., 2014; Nie et al., 2014; Ren et al., 2016), solid-state method (Gopalakrishnan and Rangan, 1992; Zatovsk, 2010; Du et al., 2013; Zhu et al., 2014; Klee et al., 2016b), and electrospinning method (Kajiyama et al., 2014; Li et al., 2015b) are summarized as follow.
Sol-gel method is the most common method to synthesize Na3V2(PO4)3, which converts colloidal suspension (sol) into a whole 3D network (gel) with submicron scale pores. Compared with other approaches, Sol-gel method has lower operating temperature and the preparation process is easy to control. However, it usually needs high cost and complex preparation routes. Hence, it does not always meet the industrial demands (Zhou et al., 2009; Rui et al., 2014; Wang D. X. et al., 2015).
Wang et al. (2019) have successfully prepared a Na3V2(PO4)3 cathode material by a typical sol-gel method using citric acid as complexant. The as-obtained Na3V2(PO4)3 sample exhibits a high initial discharge capacity of 107 mAh g−1 and high reversible capacity (97.1 mAh g−1) after 150 cycles at 0.2 C. A Na3V2(PO4)3 cathode material coated by carbon has been prepared by standard sol-gel method, which shows particles size range from 10 to 20 μm (Figures 3A,B) (Böckenfeld and Balducci, 2014). Results from galvanostatic intermittent titration technique (GITT) and cyclic voltammetry (CV) test reveal the apparent diffusion coefficient of sodium ions in the rhombohedral NVP. It ranges from 6 × 10−13 to 2 × 10−15 cm2 s−1, following an alike tendency that lithium ions behave in monoclinic Li3V2(PO4)3, indicating that the potential in ion extraction/insertion is minimum. Figure 3C shows the cycling performance over 100 cycles at 1 C. As described, the cathode material displays a discharge capacity of 92 mAh g−1 at the first cycle and 85 mAh g−1 at the 100th cycle, corresponding to a capacity retention rate of 92%. It also presents good rate behavior (Figure 3D). When cycled from 0.1 to 0.5 C (corresponding to currents of 11.9 to 59.5 mAh g−1), and values in the order of 95 mAh g −1.
Figure 3. (A,B) The SEM images of NVP/C, (C) constant-current charge/discharge of NVP-based electrodes material at 1 C, (D) C-rate test of Na3V2(PO4)3-based electrodes.
Hydrothermal method is a liquid chemical synthesis approach that can guarantee a homogeneous particle size distribution and high purity. Therefore, the hydrothermal method becomes one of the most common methods to synthesize the electrode materials. However, it should be noted that the hydrothermal method is not easily detected because the reactions are carried out in a kettle, thus making the process difficult to monitor (Liu et al., 2004; Gao et al., 2013).
Wang (2019) have obtained Na3V2(PO4)3 by one-step hydrothermal method. According to their report, the as-synthesized Na3V2(PO4)3 has a discharge specific capacity of 89.3 mAh g−1 at the first cycle, and after 30 cycles, the capacity increases to 91 mAh g−1, signifying a good cycling performance. Ruan (Ruan et al., 2017) also successfully synthesizes a new kind of chrysanthemum structure Na3V2(PO4)3 and carbon composite (NVP/C) cathode material, and corresponding fabrication process is presented in Figure 4A. During the sodium ion diffusion process, scattering nanosheets in chrysanthemum petals are good for reducing energy consumption, while the carbon-coated layer can obviously boost the entirety electrochemical behavior. As a result, the sample shows an excellent electrochemical property because of its characteristic structure. It processes a premier discharge capacity of 117.4 mAh g−1 at 0.05 C and an ultra-high specific capacity of 101.3 mAh g−1 at 10 C (Figure 4B). Furthermore, it can maintain a high discharge capacity of 87.5 mAh g−1 after 1,000 cycles at 10 C, as displayed in Figure 4C. Figure 4D shows the scanning electron microscope(SEM) image of the NVP/C after cycling, it clearly exhibits that the chrysanthemum structure can still be maintained even after 1,000 cycles at 10 C. Therefore, the improved electrochemical property of NVP/C can be attributed to its excellent structure stability.
Figure 4. (A) Schematic illustration ofthe fabrication of NVP/C materials, (B) rate performance of NVP/C, (C) cycle performance of the NVP/C cycled at 10 C for 1,000 cycles, (D) SEM image of the NVP/C at 10 C for 1,000 cycles.
Solid-state method, a traditional method of preparing electrode materials, is widely used in large-scale industrial application because of controllable reaction conditions, low cost and simple operation process. It should be noted that solid-state method still faces many challenges, such as anomalous morphologies and inhomogeneity of products.
A carbon-coated Na3V2(PO4)3 cathode material (NVP/C) is prepared through the simple and easy-to-operate solid-state method (Zhu, 2019). The as-prepared NVP/C composite exhibits some porous network structure (Figure 5A), which are good for increasing specific surface area, promoting electrolyte infiltration, and facilitating transmissions of sodium ions. As a consequence, the electrochemical performances of NVP after modification are significantly enhanced. NVP/C cathode material shows an initial discharge capacity of 95.6 mAh g−1 at 0.5 C (Figure 5B), signifying a good cycling performance. When cycled at 5 C, it can still deliver a high capacity of 71.39 mAh g−1 and go through 1,000 cycles with a capacity retention of 72.3% (Figure 5C). Jian (2012) also reports a carbon-coated Na3V2(PO4)3 material made by one-step solid phase method, which manifests a discharge capacity 107.1 mAh g−1 at 0.1 C and obtains a lifespan over 80 cycles with a retention of 92.9%.
Figure 5. (A) SEM images of NVP/C, (B) cycle performance of the NVP/C, (C) cycle performance of the NVP/C cycled at a rate of 5 C for 1,000 cycles.
Except for the technologies mentioned above, electrospinning is a method to fabricate fiber structure electrode materials. Compared with other methods, electrospinning can satisfy the large-scale industrial preparation and able to prepare uniform materials. Liu et al. (2014) use 20–30 nm NVP nanoparticles and citric acid as reactants to prepared a framework Na3V2(PO4)3/carbon (NVP/C) composite material by a simple electrostatic spinning and subsequent carbonization strategy. Figure 6A shows the SEM image of the final composite, it is obvious that NVP/C nanofibers interweave each other to form a 3D network. Figure 6B shows the transmission electron microscopy(TEM) images of NVP/C, where NVP are coated uniformly by thin carbon layer and form composite fiber with diameters about 200 nm. Ascribing to the 3D crosslinked conductive network, the as-obtained cathode material exhibits high charge (discharge)capacity of 103(101) mAh g−1 at 0.1 C (Figure 6C) and manifests a stable discharge capacities of 77, 58, 39, and 20 mAh g−1 at 2, 5, 10, and 20 C, respectively (Figure 6C).
Figure 6. (A) SEM image of NVP/C, (B) low-magnification TEM image, (C) the first Charge-discharge curve at 0.1 C rate, (D) cycling performance of the NVP/C cathode at different current densities.
In summary, synthesis methods can obviously affect the structure and morphology of NVP cathode materials and further determine their electrochemical performance. Sol-gel method is widely used for obtaining electrode materials with homogeneous particle size. Hydrothermal treatment can guarantee high specific surface area and high purity. Solid-state method has the merits of low cost and simple operation process. Electrospinning is suitable to large-scale industrial preparation and able to prepare the uniform materials. Herein, the electrochemical performances of NVP prepared by different methods are compared and listed in Table 1.
As shown, Na3V2(PO4)3 with excellent electrochemical property should have homogeneous particle size distribution and high specific surface area, which are convenient to the diffusion of Na+. In this respect, hydrothermal method behavior much better than other preparing technologies, however, considering the industrial application, this methods need to be improved or coordinated with other approaches.
In other way, modification approaches including element doping (Aragón et al., 2015a,b; Fang et al., 2015; Shen et al., 2015b; Xu and Sun, 2016; Zhou W. D. et al., 2016; Chen L. F. et al., 2017; Zheng Q. et al., 2017; Li et al., 2018; Xiao H. et al., 2018; Zhao et al., 2018; Zhu et al., 2018; Fang J. Q. et al., 2019), and nanostructures (Huang et al., 2002; Li S. et al., 2014; Li et al., 2015c; Chu and Yue, 2016; Chen S. Q. et al., 2017; Wei et al., 2017; Zhang C. Z. et al., 2017) can also deeply influence the cycling life and rate performance of NVP.
Adding heterogenous ions with larger ionic radius into the Na3V2(PO4)3 crystaline is an effective way to increase crystal volume, thereby expanding the tunnel for the diffusion of Na+. Furthermore, this method can also increase active sites. Hence, element doping is usually considered as a useful way to improve the performance of electrode materials.
Lim et al. (2014) use K as heterogeneous element to enlarge the diffusion accesses of Na+ and maintain the NASICON framework during repeated cycling process, obtaining a significant improvement of electrochemical performance. The XRD patterns in Figure 7A reveal that the addition of K+ cannot change the crystal structure of NVP. Even there are no obvious difference in initial charge/discharge capacity between undoped NVP/C and doped NVP/C (Figure 7B), the cycling stability and rate performance of doped NVP/C cathode material with doping content of 0.09 and 0.12 increase significantly (Figures 7C,D).
Figure 7. (A) XRD patterns of NVP/C doped with K+, (B) initial charge-discharge curve, (C) cycle property of NVP/C doped with K+, (D) rate capabilities of NVP/C doped with K+.
Shen et al. (2015a) utilize B doped carbon to coat Na3V2(PO4)3 with different B source (BC3, B4C, BCO2, and BC2O). It shows that the more BC2O and BCO2 in the Na3V2(PO4)3/carbon composite, the best electrochemical property can be achieved, especially high-rate capability and cyclic stability. This can be attributed to the increased external defects and active sites derived from BC2O and BCO2 doping, which accelerate the migration of Na+ in the carbon layer.
Chen et al. (2018) synthesize F-doping and V-defect Na3V1.98(PO4)3−xF3x/C composites by solid-state reaction route. F-doping is advanced to short the pathway of Na+ diffusion. The Na3V1.98(PO4)2.9F0.3/C composite delivers an initial charge capacity as high as 143.5 mAh g−1 at 0.1 C. After 100 cycles at 1 C, a reversible capacity is 100.6 mAh g−1.
Besides, transition metal elements are also commonly used to doping NVP to improve the electrochemical performance. Liu et al. (2019) use Fe element to prepare Fe-doped Na3V2(PO4)3@C cathode material. The as-obtained Na3V1.85Fe0.15(PO4)3@C shows a high capacity of 103.69 mAh g−1 and retain a capacity of 94.45 mAh g −1 after 1,200 cycles at 20 C.
Reducing particle size of NVP to nanometer scale is another way to shorten Na+ diffusion distance, and expand the effective contact area between electrolyte and the active material (Wu et al., 2019). Therefore, cycling performance as well as rate capabilities of Na3V2(PO4)3 can be enhanced. However, nanomaterials are easy to agglomerate, leading to an irreversible capacity loss.
As Figure 8A presented, Yang et al. (2015) have embed parts of NVP nanoparticles into carbon nanofibers to obtain Na3V2(PO4)3/carbon nanofibers composite (NVP-CNF), which has ultra-high power and excellent cycle performances due to rapid migration of sodium ions along with the conductive CNF. The XRD patterns of the prepared NVP-CNF composites are displayed in Figure 8B. It shows that all the composites have NASICON structure, and belong to the R-3c space group. Figures 8C,D reveal that the NVP nanoparticles have uniformly embedded in the carbon nanofiber. As seen in Figures 8E,F, the NVP-CNF composites have superior rate performances and high capacity retention of ~93% at 1 C after 300 cycles.
Figure 8. (A) The formation process of the NVP-CNF composite by schematic diagram, (B) X-ray diffraction patterns of NVP-CNF-4 h and NVP-CNF-6 h, (C) SEM image of NVP-CNF composite, (D) TEM image of NVP-CNF composite, (E) rate property plots of NVP-CNF-4 h and NVP-CNF-6 h, (F) cycle property of NVP-CNF-4 h and NVP-CNF-6 h.
As demonstrated in Figure 9, nitrogen doped grapheme (N-graphene) has been used as coating layer to modify Na3V2(PO4)3 nanocrystal (NVP/C@N-graphene) (Liu and Guo, 2017). Figures 10A,B display the SEM images of NVP/C@N-graphene, in which the NVP/C particles are anchored on the surface of N-graphene and construct an ideal 3D conductive network. Benefiting from this unique structure, the as-obtained cathode material has an initial specific capacity of 115.2 mAh g−1, amounting to about 97.6% of the theoretical capacity of NVP (Figure 10C). In addition, Figure 10D reveals that the composite material still have a high capacity after 1,000 cycles at 15 C, which can be ascribed to the enhanced conductivity by the cross-linked network consisting of carbon-coating layer and N-graphene.
Figure 10. (A,B) SEM image of the NVP/C@N-graphene, (C) charge-discharge curve of NVP/C@N-graphene, (D) cycle property of the NVP/C@N-graphene at 15 C.
In general, Nano-structure is a promising method to decrease the Na+ and electrons diffusion distance inside the NVP cathode materials, thereby improving the electrochemical properties of NVP materials.
Except for the technologies mentioned above, some other strategies can also enhance cycling stability as well as rate capability of NVP, such as encapsulating NVP into conductive materials (Jung et al., 2013; Tao et al., 2016) or coating it with conductive materials (Hultman et al., 2003; Xiong et al., 2012; Shen et al., 2013, 2016; Song et al., 2013, 2014b,c; Li G. et al., 2014; Si et al., 2014; Guo et al., 2015; Rui et al., 2015; Li et al., 2016, 2017; Xu et al., 2016; Zhou X. S. et al., 2016; Chen L. et al., 2017; Liang et al., 2017; Zhang H. et al., 2017; Gu et al., 2018; Kim et al., 2018; Yi H. M. et al., 2018).
A porous Na3V2(PO4)3/carbon(NVP/C) has been synthesized by a new solution-based method (Saravanan et al., 2013). As we can see from the field emission scanning electron microscope (FESEM) images in Figures 11A,B, NVP/C particles with irregular morphologies and uneven sizes ranging from 500 to 900 nm build up interlinked networks. The composite exhibits initial charge capacity of 50 mAh g−1 at 0.2 C, and retains 85% of its primal capacity at 10 C. The first charge-discharge curves of NVP/C at various current rates are shown in Figure 11C, which implies low over potential and good rate behavior. As illustrated in Figure 11D, the composite go through 30,000 cycles and just lose 50% of its original capacity.
Figure 11. (A) FESEM images at low, and (B) high magnification of NVP/C, (C) galvanostatic cycling of NVP/C at different current rates, (D) cycle property of the material cycled at a rate of 40 C for 30,000 cycles.
Jiang et al. (2015) have impregnated NVP nanoparticles coated by carbon into a ordered 3D mesoporous interconnected CMK-3(NVP@C@CMK-3). Figure 12A displays that NVP@C@CMK-3 has a rod-like shape, NVP@C particles are encapsulated in the pores of CMK-3. The outer carbon layer could greatly enhance the conductivity of the NVP. As a result, the NVP@C@CMK-3 demonstrates long lifespan over 2,000 cycles and high remaining capacity of 78 mAh g−1 at 5 C (Figure 12B), indicating good cycling performances. Compared with bare NVP, faster diffusion of Na+ owing to rich porous structure of the NVP@C@CMK-3 improves the cycle capability.
Figure 12. (A) SEM image of the NVP@C@CMK-3, (B) cycle properties of the NVP,NVP@C, NVP@CMK-3, and NVP@C@CMK-3 at 5 C.
The electrochemical performances of aforementioned NVP cathode materials modified by different methods are compared and listed in Table 2. It is clearly that nanostructured NVP present much better electrochemical performance because they can provide fast Na+/electrons migration pathway and more active sites for electrochemical reactions.
As one kind of SIBs cathode material, Na3V2(PO4)3 has many merits including high energy storage capacities and excellent structural stability. Unfortunately, its large scale applications are impeded by some obstacles. The main challenge of Na3V2(PO4)3 is the poor electron conductivity. Besides, as similar as other inserting materials, the volume of Na3V2(PO4)3 will change during charge and discharge process. In addition, the crystal structure of Na3V2(PO4)3 may change in low temperature. Corresponding expressions have been added in the last part of the revised manuscript.
This review summarizes some common preparing approaches of Na3V2(PO4)3 cathode materials and analyzes their effects on the electrochemical of NVP. Sol-gel method is widely used for obtaining electrode materials with homogeneous particle size. Hydrothermal treatment can guarantee high specific surface area and high purity of products. Solid-state method occupies merits of low cost and simple operation process. Electrospinning is suitable to large-scale industrial preparation and able to prepare the uniform materials. NVP cathode materials prepared by all of these methods have various properties, which make the NVP have good prospects for development.
Many kinds of conductive carbon materials are used as coating layer to improve the conductivity of NVP. Various of nanostructured NVP are prepared to shorten the diffusion distance of Na+/electrons. Some elements with larger ionic radium are applied to replace Na+, for the sake of enlarging volume of NVP and proving more reaction sites. All of these approaches indeed help enhancing the electrochemical performances of Na3V2(PO4)3 cathode materials
It is believed that low cost and large-scale material preparation can be achieved by continuous in-depth researches, and Na3V2(PO4)3 integrating stable structure, high surface specific are, fast Na+/electrons migration channels will be developed. By doing that, the industrial application of high performance NVP cathode materials will be within reach.
XZ, JP, and YG contributed the conception and design of the study. XH and HZ organized the database. JP wrote the first draft of the manuscript. XZ and YG revised the whole manuscript.
This work was financially supported by Sichuan Youth Science and Technology Innovation Research Team Project (2016TD0024) and Key Projects of Zigong Science and Technology Bureau (2018CXJD07).
HZ was employed by the company Zigong Langxingda Technology Co., Ltd.
The remaining authors declare that the research was conducted in the absence of any commercial or financial relationships that could be construed as a potential conflict of interest.
Aragón, M. J., Lavela, P., Alcántara, R., and Tirado, J. L. (2015a). Effect of aluminium doping on carbon loaded Na3V2(PO4)3 as cathode material for sodium-ion batteries. Electrochim. Acta 180, 824–830. doi: 10.1016/j.electacta.2015.09.044
Aragón, M. J., Lavela, P., Ortiz, G. F., and Tirado, P. J. L. (2015b). Benefits of chromium substitution in Na3V2(PO4)3 as a potential candidate for sodium-ion batteries. ChemElectroChem 2, 995–1002. doi: 10.1002/celc.201500052
Böckenfeld, N., and Balducci, A. (2014). Determination of sodium ion diffusion coefficients in sodium vanadium phosphate. J. Solid State Electrochem. 18, 959–964. doi: 10.1007/s10008-013-2342-6
Caballero, A., Hernán, L., Morales, J., Sánchez, L., Santos Peña, J., and Aranda, M. A. G. (2002). Synthesis and characterization of high-temperature hexagonal P2-Na0.6MnO2 and its electrochemical behaviour as cathode in sodium cells. J. Mater. Chem. 12, 1142–1147. doi: 10.1039/b108830k
Chang, Y. L., Song, Y., Wang, Z. B., Helander, M. A., Qiu, J., Chai, L., et al. (2013). Highly efficient warm white organic light-emitting diodes by triplet exciton conversion. Adv. Funct. Mater. 23, 705–712. doi: 10.1002/adfm.201201858
Chen, L., Zhao, Y. M., Liu, S. H., and Zhao, L. (2017). Hard carbon wrapped Na3V2(PO4)3@C porous composite extending cycling lifespan for sodium-ion batteries. ACS Appl. Mater. Interfaces 9, 44485–44493. doi: 10.1021/acsami.7b14006
Chen, L. F., Lu, Y., Yu, L. D., and Lou, X. W. (2017). Designed formation of hollow particle-based nitrogen-doped carbon nanofibers for high-performance supercapacitors. Energy Environ. Sci. 10, 1777–1783. doi: 10.1039/C7EE00488E
Chen, S. Q., Wu, C., Shen, L. F., Zhu, C. B., Huang, Y. Y., Xi, K., et al. (2017). Challenges and perspectives for nasicon-type electrode materials for advanced sodium-ion batteries. Adv. Mater. 29:1700431. doi: 10.1002/adma.201700431
Chen, Y. J., Xu, Y. L., Sun, X. F., Zhang, B. F., He, S. N., and Wang, C. (2018). F-doping and V-defect synergetic effects on Na3V2(PO4)3/C composite: a promising cathode with high ionic conductivity for sodium ion batteries. J. Power Sources 397, 307–317. doi: 10.1016/j.jpowsour.2018.07.006
Chu, Z. L., and Yue, C. B. (2016). Core-shell structured Na3V2(PO4)3/C nanocrystals embedded in multi-walled carbon nanotubes: a high-performance cathode for sodium-ion batteries. Solid State Ionics 287, 36–41. doi: 10.1016/j.ssi.2015.07.024
Deng, J., Luo, W. B., Chou, S. L., Liu, H. K., and Dou, S. X. (2018). Sodium-ion batteries: from academic research to practical commercialization. Adv. Energy Mater. 8:1701428. doi: 10.1002/aenm.201701428
Du, K., Guo, H., Hu, G., Peng, Z., and Cao, Y. (2013). Na3V2(PO4)3 as cathode material for hybrid lithium ion batteries. J. Power Sources 223, 284–288. doi: 10.1016/j.jpowsour.2012.09.069
Fang, J. Q., Wang, S. Q., Yao, X., Hu, X. C., Wang, Y., and Wang, H. H. (2019). Ration design of porous Mn-doped Na3V2(PO4)3 cathode for high rate and super stable sodium-ion batteries. Electrochim. Acta 295, 262–269. doi: 10.1016/j.electacta.2018.10.150
Fang, R., Miao, C., Mou, H. Y., and Xiao, W. (2019). Facile synthesis of Si@TiO2@rGO composite with sandwich-like nanostructure as superior performance anodes for lithium ion batteries. J. Alloys Compd. 818:152884. doi: 10.1016/j.jallcom.2019.152884
Fang, R., Xiao, W., Miao, C., Mei, P., Yan, X. M., Zhang, Y., et al. (2020). Improved lithium storage performance of pomegranate-like Si@NC/rGO composite anodes by facile in-situ nitrogen doped carbon coating and freeze drying processes. J. Alloys Compd. 834:155230. doi: 10.1016/j.jallcom.2020.155230
Fang, Y., Xiao, L., Qian, J., Cao, Y., and Yang, H. (2016). 3D graphene decorated NaTi2(PO4)3 microspheres as a superior high-rate and ultracycle-stable anode material for sodium ion batteries. Adv. Energy Mater. 6:1502197. doi: 10.1002/aenm.201502197
Fang, Y. J., Xiao, L. F., Ai, X. P., Cao, Y. L., and Yang, H. X. (2015). Hierarchical carbon framework wrapped Na3V2(PO4)3 as a superior high-rate and extended lifespan cathode for sodium-ion batteries. Adv. Mater. 27, 5895–5900. doi: 10.1002/adma.201502018
Fang, Y. J., Yu, X. Y., and Lou, X. W. (2017). A practical high-energy cathode for sodium-ion batteries based on uniform P2-Na0.7CoO2 microspheres. Angew. Chem. Int. Ed. 56, 5801–5805. doi: 10.1002/anie.201702024
Farbod, B., Cui, K., Kalisvaart, W. P., Kupsta, M., Zahiri, B., Kohandehghan, A., et al. (2014). Anodes for sodium ion batteries based on tin-germanium-antimony alloys. ACS Nano 8, 4415–4429. doi: 10.1021/nn4063598
Gao, M. R., Xu, Y. F., Jiang, J., and Yu, S. H. (2013). Nanostructured metal chalcogenides: synthesis, modification, and applications in energy conversion and storage devices. Chem. Soc. Rev. 42, 2986–3017. doi: 10.1039/c2cs35310e
Goodenough, J. B., and Park, K. S. (2013). The Li-ion rechargeable battery: a perspective. J. Am. Chem. Soc. 135, 1167–1176. doi: 10.1021/ja3091438
Gopalakrishnan, J., and Rangan, K. K. (1992). ChemInform abstract: V2(PO4)3: a novel NASICON-type vanadium phosphate synthesized by oxidative deintercalation of sodium from Na3V2(PO4)3. ChemInform 23, 745–747. doi: 10.1002/chin.199244030
Gu, E., Liu, S. H., Zhang, Z. Z., Fang, Y. Y., Zhou, X. S., and Bao, J. C. (2018). An efficient sodium-ion battery consisting of reduced graphene oxide boned Na3V2(PO4)3 in a composite carbon network. J. Alloys Compd. 767, 131–140. doi: 10.1016/j.jallcom.2018.07.082
Guo, J. Z., Wu, X. L., Wan, F., Wang, J., Zhang, X. H., and Wang, R. S. (2015). A superior Na3V2(PO4)3-based nanocomposite enhanced by both N-doped coating carbon and graphene as the cathode for sodium-ion batteries. Chem. Eur. J. 21, 17371–17378. doi: 10.1002/chem.201502583
Han, X., Gui, X., Yi, T. F., Li, Y. W., and Yue, C. B. (2018). Recent progress of NiCo2O4-based anodes for high-performance lithium-ion batteries. Curr. Opin. Solid State Mater. Sci. 22, 109–126. doi: 10.1016/j.cossms.2018.05.005
Hasa, I., Hassoun, J., Sun, Y. K., and Scrosati, B. (2014). Sodium-ion battery based on an electrochemically converted NaFePO4 cathode and nanostructured tin-carbon anode. ChemPhysChem 15, 2152–2155. doi: 10.1002/cphc.201400088
Huang, H., Yin, S. C., Kerr, T., Taylor, N., and Nazar, L. F. (2002). Nanostructured composites: a high capacity, fast rate Li3V2(PO4)3/carbon cathode for rechargable lithium batteries. Adv. Mater. 14, 1525–1528. doi: 10.1002/1521-4095(20021104)14:21<1525::AID-ADMA1525>3.0.CO;2-3
Hultman, L., Neidhardt, J., Hellgren, N., Sjöström, H., and Sundgren, J. E. (2003). Fullerene-like carbon nitride: a resilient coating material. MRS Bull. 28, 194–202. doi: 10.1557/mrs2003.62
Hwang, J. Y., Myung, S. T., and Sun, Y. K. (2017). Sodium-ion batteries: present and future. Chem. Soc. Rev. 46, 3529–3614. doi: 10.1039/C6CS00776G
Jian, Z. L. (2012). Novel Electrode Materials for Stationary Batteries. Wuhan: Wuhan University of Technology.
Jiang, Y., Yang, Z. Z., Li, W. H., Zeng, L. C., Yu, Y., et al. (2015). Nanoconfined carbon-coated Na3V2(PO4)3 particles in mesoporous carbon enabling ultralong cycle life for sodium-ion batteries. Adv. Energy Mater. 5:1402104. doi: 10.1002/aenm.201402104
Jiang, Z., Li, Y. H., Han, C., Huang, Z. Q., Wu, X. W., He, Z. X., et al. (2020). Raising lithium storage performances of NaTi2(PO4)3 by nitrogen and sulfur dual-doped carbon layer. Electrochem. Soc. 167:020550. doi: 10.1149/1945-7111/ab6c5c
Jung, Y. H., Lim, C. H., and Kim, D. K. (2013). Graphene-supported Na3V2(PO4)3 as a high rate cathode material for sodium-ion batteries. J. Mater. Chem. A 1, 11350–11354. doi: 10.1039/c3ta12116j
Kabbour, H., Coillot, D., Colmont, M., Masquelier, C., and Mentré, O. (2011). α-Na3M2(PO4)3 (M=Ti, Fe): absolute cationic ordering in NASICON-type phases. J. Am. Chem. Soc. 133, 11900–11903. doi: 10.1021/ja204321y
Kajiyama, S., Kikkawa, J., Hoshino, J., Okubo, M., and Hosono, E. (2014). Assembly of Na3V2(PO4)3 nanoparticles confined in a one-dimensional carbon sheath for enhanced sodium-ion cathode properties. Chem. Eur. J. 20, 12636–12640. doi: 10.1002/chem.201403126
Kang, J. W., Baek, S., Mathew, V., Gim, J., Song, J. J., Park, H., et al. (2012). High rate performance of a Na3V2(PO4)3/C cathode prepared by pyro-synthesis for sodium-ion batteries. J. Mater. Chem. 22, 20857–20860. doi: 10.1039/c2jm34451c
Kim, D., Kang, S. H., Slater, M., Rood, S., Vaughey, J. T., Karan, N., et al. (2011). Enabling sodium batteries using lithium-substituted sodium layered transition metal oxide cathodes. Adv. Energy Mater. 1, 333–336. doi: 10.1002/aenm.201000061
Kim, H., Lim, H., Kim, H. S., Kim, K. J., Byun, D. J., Choi, W. (2018). Polydopamine-derived N-doped carbon-wrapped Na3V2(PO4)3 cathode with superior rate capability and cycling stability for sodium-ion batteries. Nano Res. 12, 397–404. doi: 10.1007/s12274-018-2229-z
Kim, S. W., Seo, D. H., Ma, X., Ceder, G., and Kang, K. (2012). Electrode materials for rechargeable sodium-ion batteries: potential alternatives to current lithium-ion batteries. Adv. Energy Mater. 2, 710–721. doi: 10.1002/aenm.201200026
Klee, R., Aragón, M. J., Alcántara, R., Tirado, J. L., and Lavela, P. (2016b). High-performance Na3V2(PO4)3/C cathode for sodium-ion batteries prepared by a ball-milling-assisted method. Eur. J. Inorg. Chem. 2016, 3212–3218. doi: 10.1002/ejic.201600241
Klee, R., Aragón, M. J., Lavela, P., Alcántara, R., and Tirado, J. L. (2016a). Na3V2(PO4)3/C nanorods with improved electrode-electrolyte interface as cathode material for sodium-ion batteries. ACS Appl. Mater. Interfaces 8, 23151–23159. doi: 10.1021/acsami.6b07950
Komaba, S., Murata, W., Ishikawa, T., Yabuuchi, N., Ozeki, T., Nakayama, T., et al. (2011). Electrochemical Na insertion and solid electrolyte interphase for hard-carbon electrodes and application to Na-ion batteries. Adv. Funct. Mater. 21, 3859–3867. doi: 10.1002/adfm.201100854
Komaba, S., Takei, C., Nakayama, T., Ogata, A., and Yabuuchi, N. (2010). Electrochemical intercalation activity of layered NaCrO2 vs. LiCrO2. Electrochem. Commun. 12, 355–358. doi: 10.1016/j.elecom.2009.12.033
Kundu, D., Talaie, E., Duffort, V., and Nazar, L. F. (2015). The emerging chemistry of sodium ion batteries for electrochemical energy storage. Angew. Chem. Int. Ed. 46, 3431–3448. doi: 10.1002/anie.201410376
Lavela, P., Aragón, M. J., Ortiz, G. F., Alcántara, R., and Tirado, J. L. (2018). On the effect of silicon substitution in Na3V2(PO4)3 on the electrochemical behavior as cathode for sodium-ion batteries. ChemElectroChem 5, 367–374. doi: 10.1002/celc.201700933
Lee, J. W., Shin, H. S., Lee, C. W., and Jung, K. N. (2016). Carbon-and binder-free NiCo2O4 nanoneedle array electrode for sodium-ion batteries: electrochemical performance and insight into sodium storage reaction. Nanoscale Res. Lett. 11:45. doi: 10.1186/s11671-016-1271-6
Lee, K. T., Ramesh, T. N., Nan, F., Botton, G., and Nazar, L. F. (2011). Cheminform abstract: topochemical synthesis of sodium metal phosphate olivines for sodium-ion batteries. ChemInform 42. doi: 10.1002/chin.201144019
Li, F., and Zhou, Z. (2018). Micro/nanostructured materials for sodium ion batteries and capacitors. Small 14:1702961. doi: 10.1002/smll.201702961
Li, F., Zhu, Y. E., Sheng, J., Yang, L. P., Zhang, Y., and Zhou, Z. (2017). GO-induced preparation of flake-shaped Na3V2(PO4)3@rGO as high-rate and long-life cathodes for sodium-ion batteries. J. Mater. Chem. A 5, 25276–25281. doi: 10.1039/C7TA07943E
Li, G., Jiang, D., Wang, H., Lan, X., Zhong, H., and Jiang, Y. (2014). Glucose-assisted synthesis of Na3V2(PO4)3/C composite as an electrode material for high-performance sodium-ion batteries. J. Power Sources 265, 325–334. doi: 10.1016/j.jpowsour.2014.04.054
Li, H., Bai, Y., Wu, F., Li, Y., and Wu, C. (2015b). Budding willow branches shaped Na3V2(PO4)3/C nanofibers synthesized via an electrospinning technique and used as cathode material for sodium ion batteries. J. Power Sources 273, 784–792. doi: 10.1016/j.jpowsour.2014.09.153
Li, H., Bai, Y., Wu, F., Ni, Q., and Wu, C. (2015c). Na3V2(PO4)3/C nanorods as advanced cathode material for sodium ion batteries. Solid State Ionics 278, 281–286. doi: 10.1016/j.ssi.2015.06.026
Li, H., Wu, C., Bai, Y., Wu, F., and Wang, M. Z. (2016). Controllable synthesis of high-rate and long cycle-life Na3V2(PO4)3 for sodium-ion batteries. J. Power Sources 326, 14–22. doi: 10.1016/j.jpowsour.2016.06.096
Li, H., Yu, X. Q., Bai, Y., Wu, F., and Yang, X. Q. (2015a). Effects of Mg doping on the remarkably enhanced electrochemical performance of Na3V2(PO4)3 cathode materials for sodium ion batteries. J. Mater. Chem. A 3, 9578–9586. doi: 10.1039/C5TA00277J
Li, H., Zhu, Z. Q., and Duan, W. (2014). Na3V2(PO4)3@C core-shell nanocomposites for rechargeable sodium-ion batteries. J. Mater. Chem. A Mater. Energy Sustain. 2, 8668–8675. doi: 10.1039/C4TA00106K
Li, L., Xu, Y. L., Sun, X. F., He, S. N., and Li, L. (2018). High capacity-favorable tap density cathode material based on three-dimensional carbonous framework supported Na3V2(PO4)2F3 nanoparticles. Chem. Eng. J. 331, 712–719. doi: 10.1016/j.cej.2017.09.012
Li, R., Xiao, W., Miao, C., Fang, R., Wang, Z. Y., and Zhang, M. Q. (2019). Sphere-like SnO2/TiO2 composites as high-performance anodes for lithium ion batteries. Ceramics Int. 45, 13530–13535. doi: 10.1016/j.ceramint.2019.04.059
Li, S., Dong, Y. F., Xu, L., Xu, X., He, L., and Mai, L. Q. (2014). Effect of carbon matrix dimensions on the electrochemical properties of Na3V2(PO4)3 nanograins for high-performance symmetric sodium-ion batteries. Adv. Mater. 26, 3358–3358. doi: 10.1002/adma.201470139
Li, W., Chou, S. L., Wang, J. Z., Kim, J. H., and Dou, S. X. (2014). Sn4+xP3 @ amorphous Sn-P composites as anodes for sodium-ion batteries with low cost, high capacity, long life, and superior rate capability. Adv. Mater. 26, 4037–4042. doi: 10.1002/adma.201400794
Li, Z., Young, D., Xiang, K., Carter, W. C., and Chiang, Y. M. (2013). Towards high power high energy aqueous sodium-ion batteries: the NaTi2(PO4)3/Na0.44MnO2 system. Adv. Energy Mater. 3, 290–294. doi: 10.1002/aenm.201200598
Liang, X. H., Ou, X., Zheng, F. H., Pan, Q. C., Xiong, X. H., Hu, R. Z., et al. (2017). Surface modification of Na3V2(PO4)3 by nitrogen and sulfur Dual-doped carbon layer with advanced sodium storage property. ACS Appl. Mater. Interfaces 9, 13151–13162. doi: 10.1021/acsami.7b00818
Liao, Y., Park, K. S., Xiao, P., Henkelman, G., Li, W., and Goodenough, J. B. (2013). Sodium intercalation behavior of layered NaxNbS2 (0 ≤ x ≤ 1). Chem. Mater. 25, 1699–1705. doi: 10.1021/cm400150u
Lim, S. J., Han, D. W., Nam, D. H., Hong, K. S., Eom, J. Y., Ryu, W. H., et al. (2014). Structural enhancement of Na3V2(PO4)3/C composite cathode materials by pillar ion doping for high power and long cycle life sodium-ion batteries. J. Mater. Chem. A 2, 19623–19632. doi: 10.1039/C4TA03948C
Lim, S. Y., Kim, H. J., Shakoor, R. A., Jung, Y. S., and Choi, J. W. (2012). Electrochemical and thermal properties of NASICON structured Na3V2(PO4)3 as a sodium rechargeable battery cathode: acombined experimental and theoretical study. J. Electrochem. Soc. 159, A1393–A1397. doi: 10.1149/2.015209jes
Liu, H., Rahm, E., Holze, R., and Wu, H. Q. (2004). Cathode materials for lithium ion batteries prepared by sol-gel methods. J. Solid State Electrochem. 8, 450–466. doi: 10.1007/s10008-004-0521-1
Liu, H. X., and Guo, Y. (2017). Novel design and preparation of N-doped graphene decorated Na3V2(PO4)3/C composite for sodium-ion batteries. Solid State Ionics 307, 65–72. doi: 10.1016/j.ssi.2017.04.015
Liu, J., Tang, K., Song, K. P., Van Aken, P. A., Yu, Y., and Maier, J. (2014). Electrospun Na3V2(PO4)3/C nanofibers as stable cathode materials for sodium-ion batteries. Nanoscale 6, 5081–5086. doi: 10.1039/c3nr05329f
Liu, X. H., Feng, G. L., Wang, E. H., Chen, H., Wu, Z. G., Xiang, W., et al. (2019). Insight into preparation of Fe-doped Na3V2(PO4)3@C from aspects of particle morphology design. Crystal structure modulation, and carbon graphitization regulation. ACS Appl. Mater. Interfaces 11, 12421–12430. doi: 10.1021/acsami.8b21257
Liu, Y. H., Yu, X. Y., Fang, Y. J., Zhu, X. S., Bao, J. C., Zhou, X. S., et al. (2018). Confining SnS2 ultrathin nanosheets in hollow carbon nanostructures for efficient capacitive sodium storage. Joule 2, 725–735. doi: 10.1016/j.joule.2018.01.004
Liu, Z., Yu, X. Y., Lou, X. W., and Paik, U. (2016). Sb@C coaxial nanotubes as a superior long-life and high-rate anode for sodium ion batteries. Energy Environ. Sci. 9, 2314–2318. doi: 10.1039/C6EE01501H
Mao, M., Cui, C., Wu, M., Zhang, M., Gao, T., Fan, X., et al. (2018). Flexible ReS2 nanosheets/N-doped carbon nanofibers-based paper as a universal anode for alkali (Li, Na, K) ion battery. Nano Energy 45, 346–352. doi: 10.1016/j.nanoen.2018.01.001
Nayak, P. K., Yang, L. T., Brehm, W., and Adelhelm, P. (2018). From lithium-ion to sodium-ion batteries: advantages, challenges, and surprises. Angew. Chem. 57, 102–120. doi: 10.1002/anie.201703772
Nie, P., Zhu, Y. Y., Shen, L. F., Pang, G., Xu, G. Y., Dong, S. Y., et al. (2014). From biomolecule to Na3V2(PO4)3/nitrogen-decorated carbon hybrids: highly reversible cathodes for sodium-ion batteries. J. Mater. Chem. A 2, 18606–18612. doi: 10.1039/C4TA03922J
Nie, Y., Xiao, W., Miao, C., Fang, R., Kou, Z. Y., Wang, D. M., et al. (2020b). Boosting the electrochemical performance of LiNi0.8Co0.15Al0.05O2 cathode materials in-situ modified with Li1.3Al0.3Ti1.7(PO4)3 fast ion conductor for lithium-ion batteries. Electrochim. Acta 353:136477. doi: 10.1016/j.electacta.2020.136477
Nie, Y., Xiao, W., Miao, C., Xu, M. B., and Wang, C. J. (2020a). Effect of calcining oxygen pressure gradient on properties of LiNi0.8Co0.15Al0.05O2 cathode materials for lithium ion batteries. Electrochim. Acta 334:135654. doi: 10.1016/j.electacta.2020.135654
Oh, S. M., Myung, S. T., Hassoun, J., Scrosati, B., and Sun, Y. K. (2012). Reversible NaFePO4 electrode for sodium secondary batteries. Electrochem. Commun. 22, 149–152. doi: 10.1016/j.elecom.2012.06.014
Ong, S. P., Chevrier, V. L., Hautier, G., Jain, A., Moore, C., Kim, S., et al. (2011). Voltage, stability and diffusion barrier differences between sodium-ion and lithium-ion intercalation materials. Energy Environ. Sci. 4, 3680–3688. doi: 10.1039/c1ee01782a
Palomares, V., Casas-Cabanas, M., Castillo-Martinez, E., Han, M. H., and Rojo, T. (2013). Update on Na-based battery materials. Energy Environ. Sci. 6, 2312–2337. doi: 10.1039/c3ee41031e
Pan, H., Hu, Y. S., and Chen, L. (2013). Room-temperature stationary sodium-ion batteries for large-scale electric energy storage. Energy Environ. Sci. 6, 2338–2360. doi: 10.1039/c3ee40847g
Pivko, M., Arcon, I., Bele, M., Dominko, R., and Gaberscek, M. (2012). A3V2(PO4)3 (A=Na or Li) probed by in situ X-ray absorption spectroscopy. J. Power Sources 216, 145–151. doi: 10.1016/j.jpowsour.2012.05.037
Ponrouch, A., Marchante, E., Courty, M., Tarascon, J. M., and Palacin, M. R. (2012). In search of an optimized electarolyte for Na-ion batteries. Energy Environ. Sci. 5, 8572–8583. doi: 10.1039/c2ee22258b
Qi, Y., Mu, L., Zhao, J., Hu, Y. S., Liu, H., and Dai, S. (2015). Superior Na-storage performance of low-temperature-synthesized Na3(VO1−xPO4)2F1+2x(0 ≤ x ≤ 1) nanoparticles for Na-ion batteries. Angew. Chem. Int. Ed. 54, 9911–9916. doi: 10.1002/anie.201503188
Ren, W. H., Zheng, Z. P., Xu, C., Niu, C. J., Wei, Q. L., An, Q. Y., et al. (2016). Self-sacrificed synthesis of three-dimensional Na3V2(PO4)3 nanofiber network for high-rate sodium-ion full batteries. Nano Energy 25, 145–153. doi: 10.1016/j.nanoen.2016.03.018
Ruan, Y. L., Liu, J. J., Song, S. D., Jiang, N. Y., and Battaglia, V. (2017). Multi-hierarchical nanosheet-assembled chrysanthemum-structured Na3V2(PO4)3/C as electrode materials for high-performance sodium-ion batteries. Ionics 24, 1663–1673. doi: 10.1007/s11581-017-2342-0
Rui, X. H., Sun, W. P., Wu, C., Yu, Y., and Yan, Q. Y. (2015). An advanced sodium-ion battery composed of carbon coated Na3V2(PO4)3 in a porous graphene network. Adv. Mater. 27, 6670–6676. doi: 10.1002/adma.201502864
Rui, X. H., Yan, Q. Y., Skyllas-Kazacos, M., and Lim, T. M. (2014). Li3V2(PO4)3 cathode materials for lithium-ion batteries: a review. J. Power Sources 258, 19–38. doi: 10.1016/j.jpowsour.2014.01.126
Samin, N. K., Rusdi, R., Kamarudin, N., and Kamarulzaman, N. (2012). Synthesis and battery studies of sodium cobalt oxides, NaCoO2 cathodes. Adv. Mater. Res. 545, 185–189. doi: 10.4028/www.scientific.net/AMR.545.185
Saravanan, K., Mason, C. W., Rudola, A., Wong, K. H., and Balaya, P. (2013). The first report on excellent cycling stability and superior rate capability of Na3V2(PO4)3 for sodium ion batteries. Adv. Energy Mater. 3, 444–450. doi: 10.1002/aenm.201200803
Shen, W., Li, H., Guo, Z. Y., Wang, C., Li, Z. H., Xu, Q. J., et al. (2016). Double-nanocarbon synergistically modified Na3V2(PO4)3: an advanced cathode for high-rate and long-life sodium-ion batteries. ACS Appl. Mater. Interfaces 8, 15341–15351. doi: 10.1021/acsami.6b03410
Shen, W., Li, H., Wang, C., Li, Z. H., Xu, Q. J., Liu, H. M., et al. (2015a). Improved electrochemical performance of the Na3V2(PO4)3 cathode by B-doping of the carbon coating layer for sodium-ion batteries. J. Mater. Chem. A 3, 15190–15201. doi: 10.1039/C5TA03519H
Shen, W., Wang, C., Liu, H., and Yang, W. S. (2013). Towards highly stable storage of sodium ions: a porous Na3V2(PO4)3/C cathode material for sodium-ion batteries. Chem. Eur. J. 19, 14712–14718. doi: 10.1002/chem.201300005
Shen, W., Wang, C., Xu, Q. J., Liu, H. M., and Wang, Y. G. (2015b). Nitrogen-doping-induced defects of a carbon coating layer facilitate Na-storage in electrode materials. Adv. Energy Mater. 5:1400982. doi: 10.1002/aenm.201400982
Si, L. L., Yuan, Z. Q., Hu, L., Zhu, Y. C., and Qian, Y. T. (2014). Uniform and continuous carbon coated sodium vanadium phosphate cathode materials for sodium-ion battery. J. Power Sources 272, 880–885. doi: 10.1016/j.jpowsour.2014.09.046
Slater, M. D., Kim, D., Lee, E., and Johnson, C. S. (2013). Sodium-ion batteries. Adv. Funct. Mater. 23, 947–958. doi: 10.1002/adfm.201200691
Song, J., Park, S., Mathew, V., Gim, J., and Kim, J. (2016). An enhanced high-rate Na3V2(PO4)3-Ni2P nanocomposite cathode with stable lifetime for sodium-ion batteries. ACS Appl. Mater. Interfaces 8, 35235–35242. doi: 10.1021/acsami.6b11629
Song, T. B., Ye, S. C., Liu, H. M., and Wang, Y. G. (2018). Self-doping of Ti3+ into Na2Ti3O7 increases both ion and electron conductivity as a high-performance anode material for sodium-ion batteries. J. Alloys Compd. 767, 820–828. doi: 10.1016/j.jallcom.2018.07.186
Song, W., Ji, X., Wu, Z., Zhu, Y., Yang, Y., Chen, J., et al. (2014a). First exploration of Na-ion migration pathways in the NASICON structure Na3V2(PO4)3. J. Mater. Chem. A 2, 5358–5362. doi: 10.1039/c4ta00230j
Song, W. X., Cao, X. Y., Wu, Z. P., Chen, J., Huangfu, K., Wang, X. W., et al. (2014c). A study into the extracted ion number for NASICON structured Na3V2(PO4)3 in sodium-ion batteries. Phys. Chem. Chem. Phys. 16, 17681–17687. doi: 10.1039/C4CP01821D
Song, W. X., Ji, X. B., Pan, C. C., Zhu, Y. R., Chen, Q. Y., and Banks, C. E. (2013). A Na3V2(PO4)3 cathode material for use in hybrid lithium ion batteries. Phys. Chem. Chem. Phys. 15, 14357–14363. doi: 10.1039/c3cp52308j
Song, W. X., Ji, X. B., Yao, Y. P., Zhu, H. J., Chen, Q. Y., Sun, Q. Q., et al. (2014b). A promising Na3V2(PO4)3 cathode for use in the construction of high energy batteries. Phys. Chem. Chem. Phys. 16, 3055–3061. doi: 10.1039/c3cp54604g
Tao, S., Wang, X. B., Cui, P. X., Wang, Y., Haleem, Y. A., Wei, S. H., et al. (2016). Fabrication of graphene-encapsulated Na3V2(PO4)3 as high-performance cathode materials for sodium-ion batteries. RSC Adv. 6, 43591–43597. doi: 10.1039/C6RA04237F
Tepavcevic, S., Xiong, H., Stamenkovic, V. R., Zuo, X., Balasubramanian, M., Prakapenka, V. B., et al. (2012). Nanostructured bilayered vanadium oxide electrodes for rechargeable sodium-ion batteries. ACS Nano 6, 530–538. doi: 10.1021/nn203869a
Tu, F. Z., Xu, X., Wang, P. Z., Si, L., Zhou, X. S., Bao, J. C. (2017). A few-layer SnS2/reduced graphene oxide sandwich hybrid for efficient sodium storage. J. Phys. Chem. C 121, 3261–3269. doi: 10.1021/acs.jpcc.6b12692
Vaalma, C., Buchholz, D., Weil, M., and Passerini, S. (2018). A cost and resource analysis of sodium-ion batteries. Nat. Rev. Mater. 3:18013. doi: 10.1038/natrevmats.2018.13
Wang, D. (2019). Synthesis and Properties of Na3V2(PO4)3 Cathode. Chengdu: University of Electronic Science and Technology of China.
Wang, D. X., Chen, N., Li, M., Wang, C. Z., Ehrenberg, H., Bie, X. F., et al. (2015). Na3V2(PO4)3/C composite as the intercalation-type anode material for sodium-ion batteries with superior rate capability and long-cycle life. J. Mater. Chem. A 3, 8636–8642. doi: 10.1039/C5TA00528K
Wang, K., Huang, X. B., Zhou, T., Wang, H. Y., Xie, H. S., and Ren, Y. R. (2020). Boosted electrochemical properties of porous Li2FeSiO4/C based on Fe-MOFs precursor for lithium ion batteries. Vacuum 171:108997. doi: 10.1016/j.vacuum.2019.108997
Wang, Q., Cheng, B. M., Zhong, H. Y., Wang, Q. H., and Zhong, S. W. (2019). Effect of sol-gel method on crystal growth and electrochemical properties of Na3V2(PO4)3. Chin. J. Power Sources 43, 559–561. doi: 10.3969/j.issn.1002-087X.2019.04.005
Wang, S. Y., Zhao, J. C., Wang, L. L., Liu, X. J., Wu, Y. D., Xu, J. L. (2015). High performance Na3V2(PO4)3/C composite electrode for sodium-ion capacitors. Ionics 21, 2633–2638. doi: 10.1007/s11581-015-1428-9
Wei, T. Y., Yang, G. Z., and Wang, C. X. (2017). Bottom-up assembly of strongly-coupled Na3V2(PO4)3/C into hierarchically porous hollow nanospheres for high-rate and stable Na-ion storage. Nano Energy 39, 363–370. doi: 10.1016/j.nanoen.2017.07.019
Wu, Y. R., Pan, J., Ren, S., Xie, Y., Yue, C., and Yi, T. F. (2019). Review and prospect of Li2ZnTi3O8-based anode materials for li-ion battery. Ionics 25, 373–397. doi: 10.1007/s11581-018-2818-6
Xiao, H., Huang, X. B., Ren, Y. R., Wang, H. Y., Ding, J. N., Zhou, S. B., et al. (2018). Enhanced sodium ion storage performance of Na3V2(PO4)3 with N-doped carbon by folic acid as carbon-nitrogen source. J. Alloys Compd. 732, 454–459. doi: 10.1016/j.jallcom.2017.10.195
Xiao, Y., Wang, P. F., Yin, Y. X., Zhu, Y. F., Yang, X. N., Zhang, X. D., et al. (2018). A layered-tunnel intergrowth structure for high-performance sodium-ion oxide cathode. Adv. Energy Mater. 8:1800492. doi: 10.1002/aenm.201800492
Xie, X. Q., Su, D. W., Chen, S. Q., Zhang, J. Q., Dou, S. X., and Wang, G. X. (2014). SnS2 nanoplatelet@graphene nanocomposites as high-capacity anode materials for sodium-ion batteries. Chem. Asian J. 9, 1611–1617. doi: 10.1002/asia.201400018
Xiong, S. L., Chen, J. S., Lou, X. W., and Zeng, H. C. (2012). Mesoporous Co3O4 and CoO@C topotactically transformed from chrysanthemum-like Co(CO3)0.5(OH)0.11H2O and their lithium-storage properties. Adv. Funct. Mater. 22, 861–871. doi: 10.1002/adfm.201102192
Xu, G. Y., and Sun, G. G. (2016). Mg2+-doped Na3V2(PO4)3/C decorated with graphene sheets: An ultrafast Na-storage cathode for advanced energy storage. Ceramics Int. 42, 14774–14781. doi: 10.1016/j.ceramint.2016.06.107
Xu, Y. N., Wei, Q. L., Xu, C., Li, Q. D., An, Q. Y., Zhang, P. F., et al. (2016). Layer-by-layer Na3V2(PO4)3 embedded in reduced graphene oxide as superior rate and ultralong-life sodium-ion battery cathode. Adv. Energy Mater. 6:1600389. doi: 10.1002/aenm.201600389
Yabuuchi, N., Kubota, K., Dahbi, M., and Komaba, S. (2014). Research development on sodium-ion batteries. Chem. Rev. 114, 11636–11682. doi: 10.1021/cr500192f
Yang, J., Han, D. W., Jo, M. R., Song, K., Kim, Y. I., Chou, S. L., et al. (2015). Na3V2(PO4)3 particles partly embedded in carbon nanofibers with superb kinetics for ultra-high power sodium ion batteries. J. Mater. Chem. A 3, 1005–1009. doi: 10.1039/C4TA06001F
Yang, Z., Zhang, J., Kintner-Meyer, M. C. W., Lu, X., Choi, D., Lemmon, J. P., et al. (2011). Electrochemical energy storage for green grid. Chem. Rev. 111, 3577–3613. doi: 10.1021/cr100290v
Yi, H. M., Ling, M. X., Xu, W. B., Li, X. F., Zheng, Q., and Zhang, H. M. (2018). VSC-doping and VSU-dof Na3V2−xTix(PO4)2F3 compounds for sodium ion battery cathodes: analysis of electrochemical performance and kinetic properties. Nano Energy 47, 340–352. doi: 10.1016/j.nanoen.2018.02.053
Yi, T. F., Wei, T. T., Li, Y., He, Y. B., and Wang, Z. N. (2020). Efforts on enhancing the Li-ion diffusion coefficient and electronic conductivity of titanate-based anode materials for advanced Li-ion batteries. Energy Storage Mater. 26, 165–197. doi: 10.1016/j.ensm.2019.12.042
Yi, T. F., Zhu, Y. R., Tao, W., Luo, S. H., Xie, Y., and Li, X. F. (2018). Recent advances in the research of MLi2Ti6O14 (M = 2Na, Sr, Ba, Pb) anode materials for Li-ion batteries. J. Power Sources 399, 26–41. doi: 10.1016/j.jpowsour.2018.07.086
Zatovsk, I. V. (2010). NASICON-type Na3V2(PO4)3. Acta Crystallogr. Sect. E 66:i12. doi: 10.1107/S1600536810002801
Zhang, C. Z., Guo, D. L., Qin, J. W., Mao, B. G., and Cao, M. H. (2017). Rational construction of Na3V2(PO4)3, nanoparticles encapsulated in 3D honeycomb carbon network as a cathode for sodium-ion batteries. Mater. Lett. 195, 205–208. doi: 10.1016/j.matlet.2017.02.121
Zhang, H., Hasa, I., Buchholz, D., Qin, B. S., and Passerini, S. (2017). Effects of nitrogen doping on the structure and performance of carbon coated Na3V2(PO4)3, cathodes for sodium-ion batteriesm. Carbon 124, 334–341. doi: 10.1016/j.carbon.2017.08.063
Zhao, L. N. (2019). Structure and Electrochemical Properties of Na3V2(PO4)3 Electrodes for Sodium-Ion Batteries. Beijing: University of Science and Technology Beijing.
Zhao, L. N., Zhao, H. L., Du, Z. H., Chen, N., Chang, X. W., Zhang, Z. J., et al. (2018). Computational and experimental understanding of Al-doped Na3V2−xAlx(PO4)3 cathode material for sodium ion batteries: electronic structure, ion dynamics and electrochemical properties. Electrochim. Acta 282, 510–519. doi: 10.1016/j.electacta.2018.06.074
Zheng, Q., Yi, H. M., Li, X. F., and Zhang, H. M. (2018). Progress and prospect for NASICON-type Na3V2(PO4)3 for electrochemical energy storage. J. Energy Chem. 27, 1597–1617. doi: 10.1016/j.jechem.2018.05.001
Zheng, Q., Yi, H. M., Liu, W. Q., Li, X. F., and Zhang, H. M. (2017). Improving the electrochemical performance of Na3V2(PO4)3, cathode in sodium ion batteries through Ce/V substitution based on rational design and synthesis optimization. Electrochim. Acta 238, 288–297. doi: 10.1016/j.electacta.2017.04.029
Zhong, X., Yang, Z. Z., Jiang, Y., Li, W., and Yu, Y. (2016). Carbon coated Na3V2(PO4)3 anchored on freestanding graphite foam for high performance sodium-ion cathode. Appl. Mater. Interfaces 8, 32360–32365. doi: 10.1021/acsami.6b11873
Zhou, W. D., Xue, L. G., Lü, X. J., Gao, H. C., and Goodenough, J. B. (2016). NaxMV(PO4)3(M=Mn, Fe, Ni) structure and properties for sodium extraction. Nano Letters 16, 7836–7841. doi: 10.1021/acs.nanolett.6b04044
Zhou, X. C., Liu, Y. M., and Guo, Y. L. (2009). Effect of reduction agent on the performance of Li3V2(PO4)3/C positive material by one-step solid-state reaction. Electrochim. Acta 54, 2253–2258. doi: 10.1016/j.electacta.2008.10.062
Zhou, X. S., Yu, L., and Lou, X. W. D. (2016). Formation of uniform N-doped carbon-coated SnO2 submicroboxes with enhanced lithium storage properties. Adv. Energy Mater. 6:1600451. doi: 10.1002/aenm.201600451
Zhu, C., Kopold, P., Van Aken, P. A., Maier, J., and Yu, Y. (2016). High power-high energy sodium battery based on threefold interpenetrating network. Adv. Mater. 28, 2409–2416. doi: 10.1002/adma.201505943
Zhu, C. B., Song, K. P., Aken, P. A. V., Yu, Y., and Maier, J. (2014). Carbon-coated Na3V2(PO4)3 embedded in porous carbon matrix: an ultrafast Na-storage cathode with the potential of outperforming Li cathodes. Nano Lett. 14, 2175–2180. doi: 10.1021/nl500548a
Zhu, H. G. (2019). Preparation and Properties of Na3V2(PO4)3/C Cathode Material for Sodium-Ion Batteries. Xuzhou: China University of Mining and Technology.
Zhu, Q., Cheng, H., Zhang, X. M., He, L. Q., Hu, L. Z., Yang, J. W., et al. (2018). Improvement in electrochemical performance of Na3V2(PO4)3/C cathode material for sodium-ion batteries by K-Ca co-doping. Electrochim. Acta 281, 208–217. doi: 10.1016/j.electacta.2018.05.174
Keywords: Na3V2(PO4)3, cathode material, research progress, sodium ion batteries, prepare and modification
Citation: Zeng X, Peng J, Guo Y, Zhu H and Huang X (2020) Research Progress on Na3V2(PO4)3 Cathode Material of Sodium Ion Battery. Front. Chem. 8:635. doi: 10.3389/fchem.2020.00635
Received: 13 May 2020; Accepted: 18 June 2020;
Published: 24 July 2020.
Edited by:
Zhangxing He, North China University of Science and Technology, ChinaReviewed by:
Wei Xiao, Yangtze University, ChinaCopyright © 2020 Zeng, Peng, Guo, Zhu and Huang. This is an open-access article distributed under the terms of the Creative Commons Attribution License (CC BY). The use, distribution or reproduction in other forums is permitted, provided the original author(s) and the copyright owner(s) are credited and that the original publication in this journal is cited, in accordance with accepted academic practice. No use, distribution or reproduction is permitted which does not comply with these terms.
*Correspondence: Xianguang Zeng, aG56eGcxOTc5QDEyNi5jb20=
Disclaimer: All claims expressed in this article are solely those of the authors and do not necessarily represent those of their affiliated organizations, or those of the publisher, the editors and the reviewers. Any product that may be evaluated in this article or claim that may be made by its manufacturer is not guaranteed or endorsed by the publisher.
Research integrity at Frontiers
Learn more about the work of our research integrity team to safeguard the quality of each article we publish.