- 1School of Chemical and Environmental Engineering, Shanghai Institute of Technology, Shanghai, China
- 2Department of Chemistry, Center for Supramolecular Chemistry and Catalysis, Shanghai University, Shanghai, China
- 3Tianjin Key Laboratory of Structure and Performance for Functional Molecules, College of Chemistry, Tianjin Normal University, Tianjin, China
Self-assembly of polypseudorotaxanes in high-polar organic solvents is difficult due to remarkably weak interactions between macrocycles and axles. Reported here is a novel metal-coordinated poly[2]pseudorotaxane constructed by pillar[5]arene, 1,4-bis(4-pyridyl pyridinium)butane, and [PdCl2(PhCN)2] in highly polar organic solvent of dimethyl sulfoxide (DMSO). Utilizing a combination of 1H NMR, NOESY, DOSY, DLS, SEM, and viscosity measurements, the formation of polypseudorotaxane was shown to be dependent on the concentration of [2]pseudorotaxanes/[PdCl2(PhCN)2] and temperature. Furthermore, a temperature-responsive supramolecular gel with reversibly gel–sol transformation was obtained via spontaneous assembly of the polypseudorotaxanes at high concentrations.
Introduction
Over the past 20 years, supramolecular architectures of (pseudo)rotaxanes and catenanes have played a significant role in supramolecular topology and the fabrication of mechanically interlocked molecules (Loeb, 2007; Serreli et al., 2007; Hunter, 2011; Lehn, 2017). Poly(pseudo)rotaxanes constructed by threading repeated macrocyclic rings onto linear-chain polymeric backbones have attracted tremendous attention for their specific and unique molecular recognition structures and diverse potential applications in various fields (Forgan et al., 2011; Du et al., 2012; Rambo et al., 2012; Rotzler and Mayor, 2013; Guo and Liu, 2014; Ma and Tian, 2014; Hou et al., 2016; Lefebvre et al., 2016; Kato et al., 2018; Hashidzume et al., 2019; Xiao et al., 2020).
Macrocycles are the basic building blocks in the construction of pseudorotaxanes because of the strong binding ability between macrocyclic hosts and guests. Therefore, there is no doubt that introducing new macrocycles and novel non-covalent interactions into polypseudorotaxanes will expand the applications of polypseudorotaxanes. Furthermore, variations in supramolecular structures allow them to show unique responsivity to stimuli. Pillar[n]arenes, the fifth generation of host macrocycles, have been applied to the formation of various functional supramolecular materials, owing to their rigid pillar architecture, easy functionalization, and outstanding binding properties in host–guest chemistry (Cao et al., 2009; Xue et al., 2012; Li, 2014; Ogoshi et al., 2016; Li et al., 2017; Hua et al., 2018, 2019; Chen et al., 2019; Xia et al., 2019; Shao et al., 2020; Wang et al., 2020). To date, a variety of supramolecular poly(pseudo)rotaxanes based on pillar[n]arenes have been investigated (Hu et al., 2012; Eichstaedt et al., 2016; Cui et al., 2017; Zeng et al., 2018; Li B. et al., 2019; Yang et al., 2019).
Metal coordination interactions, as a class of non-covalent interactions possessing remarkable stability and unique properties, can be used to effectively and conveniently generate polypseudorotaxane (Lee et al., 2001; Liu et al., 2003; Harada et al., 2009; Wei et al., 2014; Yan et al., 2014; Krogsgaard et al., 2016; Tian et al., 2016; Winter and Schubert, 2016; Wu et al., 2016; Huang et al., 2017; Wang et al., 2018 Xia et al., 2018; Wang L. et al., 2019; Zhu et al., 2019). However, most of the (poly)rotaxanes and (poly)pseudorotaxanes are constructed in water, low polar organic solvents, or the crystalline state. Highly polar organic solvent such as dimethyl sulfoxide (DMSO) seems to be not working because, generally, the non-covalent interactions between the wheels and axles, which greatly depend on the sorts and polarity of solvents, are quite weak in DMSO. Aqueous solution and low polar organic solvents can maintain these non-covalent interactions well. But highly polar solvents such as DMSO inhibit non-covalent bonds involving hydrogen bonding and complementary π···π-stacking, through powerful solvation of the interacting components.
In the past 10 years, our group focused on the host–guest chemistry of pillararenes and biphenarenes (Li, 2014; Ma et al., 2016; Li H. et al., 2019; Wang Y. et al., 2019; Xu et al., 2020). The association constant, (7.4 ± 0.3) × 102 M−1, of P5A and bis(pyridinium)dicationic guest in DMSO is surprisingly high, leading to the formation of a [2]psdudorotaxane-type complex (Li et al., 2010). Herein, to provide new insight into supramolecular polypseudorotaxanes in highly polar solvents, we extended our research target to novel P5A-based polypseudorotaxane bridging by palladium(II)-containing coordination interactions [PdCl2(PhCN)2]. Therefore, a linear polypseudorotaxane was constructed by [2]pseudorotaxanes making up of P5A and bis(pyridinium)dicationic (1) via metal–coordination interactions in DMSO (Scheme 1). It was expected that the utilization of P5A-based [2]pseudorotaxanes and metal–ligand coordination would be quite suitable for fabricating polymeric assemblies in highly polar solvents due to their robust interactions. Interestingly, the obtained polypseudorotaxane could continuously self-assemble at higher concentrations to form a dynamic supramolecular gel, which responded to environmental stimuli.
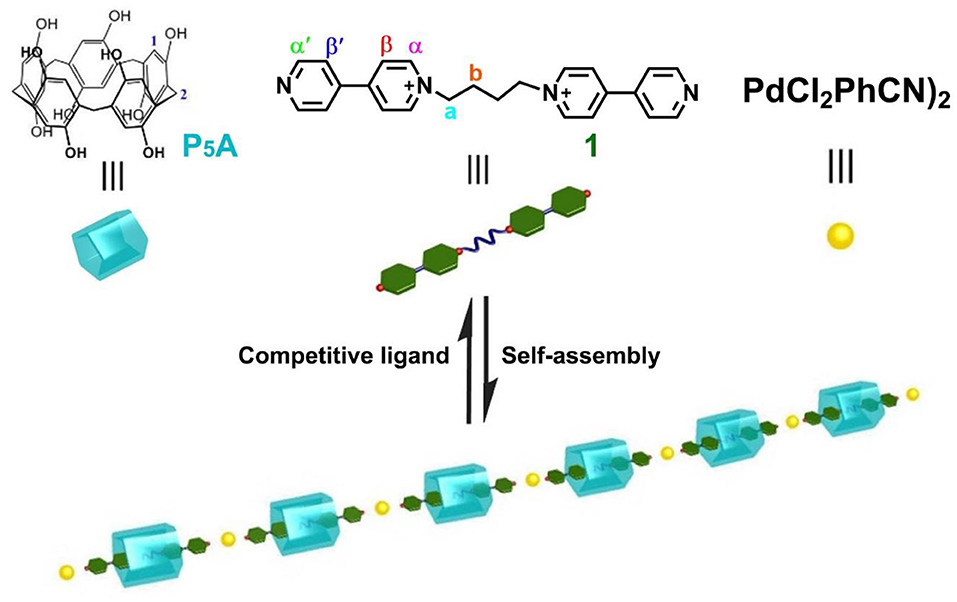
Scheme 1. Schematic representation of the conversion from [2]pseudorotaxanes to linear polypseudorotaxane.
Materials and Methods
All reagents and solvents were commercially available and used without further purification, unless otherwise noted. Compound (P5A) (Ogoshi et al., 2008; Cao et al., 2009) and bis(pyridinium)dicationic 1 (Joseph et al., 2003; Li et al., 2010) were synthesized according to literature procedures. 1H NMR and DOSY spectra were recorded on a Bruker AV500 instrument. Viscosity measurements were carried out with Ubbelohde micro dilution viscometers (Shanghai Liangjing Glass Instrument Factory, 0.40 mm inner diameter) at 298 K in DMSO. Dynamic light scattering (DLS) was analyzed on a Malvern Zetasizer 3000HSA at 298 K. Scanning electron microscopy (SEM) images were recorded on SHIMADZU SSX-550.
Results and Discussion
Initially, host–guest complexation of P5A and 1 was carried out in DMSO-d6 and investigated through 1H NMR spectroscopy. As shown in Figures 1A,E,F, the 1H NMR spectra of 1 were recorded in the absence and presence of the P5A host, where evident upfield chemical shifts and a broadening effect inside the pyridine motif and methylene protons (Ha, Hb, Hα, and Hβ) on guest 1 could be observed in the presence of P5A owing to the shielding effects in the cavity, while no apparent change was observed in the proton signals of and on guest 1. When comparing to the corresponding signals of the uncomplexed P5A and 1, new peaks were observed, demonstrating a slow exchange on the NMR timescale for this binding process. The results are in agreement with the spatial structure that the host P5A as a wheel was fully threaded by the axle of guest 1 and left pyridine moiety outside its cavity, indicating the formation of a [2]pseudorotaxane between P5A and 1 in DMSO (Scheme 1). Besides, distinct NOE correlation signals between the protons Ha, Hb, and Hα on 1 and H1−3 on P5A in a 2D NOESY spectrum further confirmed the formation of the [2]pseudorotaxanes (Supplementary Figure 1). As shown in the energy-minimized structure of the [2]pseudorotaxanes calculated by DFT (Materials Studio), multiple hydrogen bonding and C–H…π interactions between P5A and 1 provided enough non-covalent interactions and guaranteed the existence of [2]pseudorotaxanes in DMSO. And the pyridine moiety on 1 was located outside the cavity of P5A, which reserved indispensable sites for the coordination of metal (Figure 2).
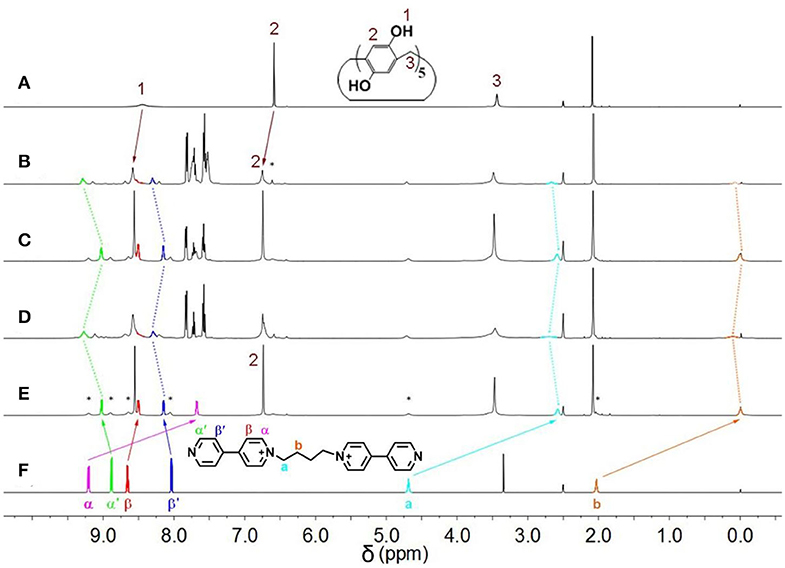
Figure 1. 1H NMR spectra (DMSO-d6, 298 K, 500 MHz) of polypseudorotaxane and building units. (A) P5A; (B) P5A + 1 + [PdCl2(PhCN)2] + PPh3 + [PdCl2(PhCN)2]; (C) P5A + 1 + [PdCl2(PhCN)2] + PPh3; (D) P5A + 1 + [PdCl2(PhCN)2]; (E) P5A + 1, (F) 1. ([P5A] = [1] = [PdCl2(PhCN)2] = 60.0 mM; *represent uncomplexed P5A and free 1).
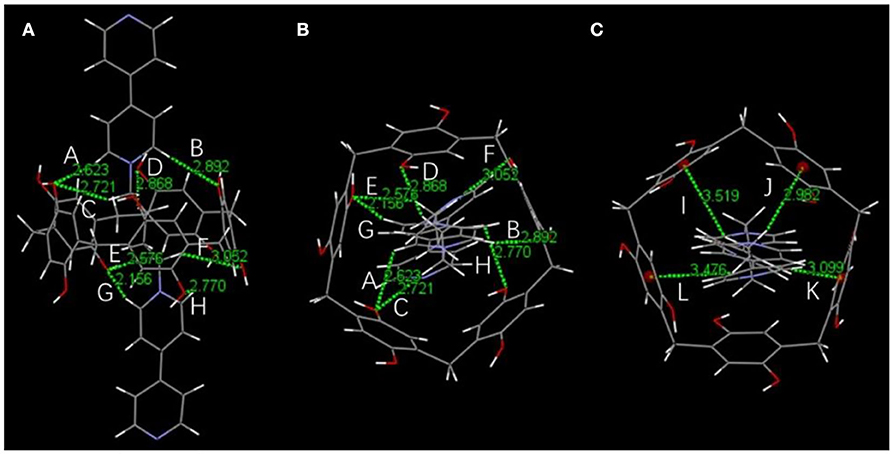
Figure 2. The energy-minimized structure of [2]pseudorotaxanes calculated by DFT (Materials Studio). The green dashed lines represent hydrogen bond interactions (A–H) and C–H…π interactions (I–L). (A) Front view and (B) top view of hydrogen bond parameters: H…O distance (Å), C(O)–H…O angle (°) A: 2.623, 122.75; B: 2.892, 169,46; C: 2.721, 147.14; D: 2.868, 144.38; E: 2.576, 144.90; F: 3.052, 148.60; G: 2.156, 161.67; H: 2.770, 101.37. (C) Top view of C–H…π interaction parameters: C–H…π distance (Å), C–H…π angle (°) I: 3.519, 138.60; J: 2.982, 156.63; K: 3.099, 142.02; L: 3.476, 133.24.
Subsequently, after dissolving 1.0 equiv of [PdCl2(PhCN)2] into 60 mM premixed solution of P5A and 1, all of the main peaks broadened remarkably, and the signals of and on guest 1 clearly shifted downfield (Figure 1D). These observations provided clear evidence of the complexation between pyridine nitrogen atoms and palladium(II) ligands. Furthermore, binding stoichiometry between [PdCl2(PhCN)2] and 1 was investigated. To a mixture of 1 and P5A ([1]: [P5A] = 1:5) in DMSO-d6, [PdCl2(PhCN)2] was added in different ratios and 1H NMR spectra were recorded. As shown in Supplementary Figure 2, upon increasing [PdCl2(PhCN)2], both the proton signals of and on the pyridine rings of 1 shifted downfield significantly, suggesting the coordination of metal to the pyridine rings. No obvious change was observed for the signals of and when the molar ratio of [1]: [PdCl2(PhCN)2] was increased to 1:1, indicating that the binding ratio between [PdCl2(PhCN)2] and 1 was 1:1 or n:n, which fitted well with the coordination characteristics between pyridine and [PdCl2(PhCN)2] (Kaminker et al., 2011). The 255 nm of the hydrodynamic radius measured by dynamic light scattering (DLS) manifested the formation of large aggregates, which excluded the 1:1 mode and confirmed that the binding ratio was n:n. The small amount of specie in several nanometers was deduced as unreacted [2]pseudorotaxanes (Figure 3). These results verified the formation of metal supramolecular polypseudorotaxane between [PdCl2(PhCN)2] and [2]pseudorotaxane.
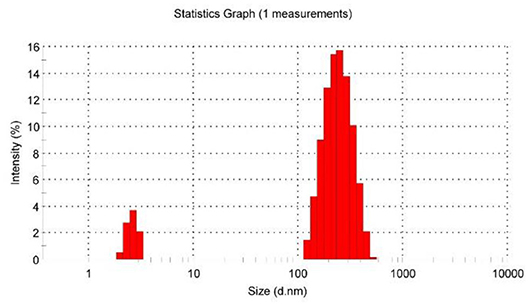
Figure 3. Dynamic light scattering (DLS) spectrum of polypseudorotaxane in DMSO ([PdCl2(PhCN)2]= [2]pseudorotaxanes = 60 mM, 298K).
Furthermore, the competitive ligand PPh3 was employed to bind palladium(II) ions to investigate the phase transition between polypseudorotaxane and [2]pseudorotaxane. Upon adding 1 equiv of PPh3 to the polypseudorotaxane, a precipitate formed at the bottom of the mixed system solution. As shown in Figure 1C, the 1H NMR spectrum was almost the same with the spectrum of [2]pseudorotaxane (Figure 1E), which indicated the formation of the more stable complex between PPh3 and palladium(II) ions, resulting in the disassembly of the polypseudorotaxane (Wang et al., 2010). After filtrating off the precipitate, one equiv of [PdCl2(PhCN)2] was added to the solution, and the peaks of protons on guest 1 returned to their original positions (Figure 1B). This result suggested that metallosupramolecular polypseudorotaxane was reconstructed. Therefore, the reversible transition between polypseudorotaxane and [2]pseudorotaxane can be realized.
Two-dimensional diffusion-ordered NMR experiments (DOSY) were adopted to explore the polypseudorotaxane. When the concentration of [2]pseudorotaxane/[PdCl2(PhCN)2] increased from 0.5 to 200 mM, the weight average diffusion coefficient (D) decreased significantly from 4.59 × 10−10 to 0.88 × 10−11 m2 s−1, suggesting an increase in the average size of the polymeric structure owing to the generation of polypseudorotaxane from the small oligomers (Figure 4A).
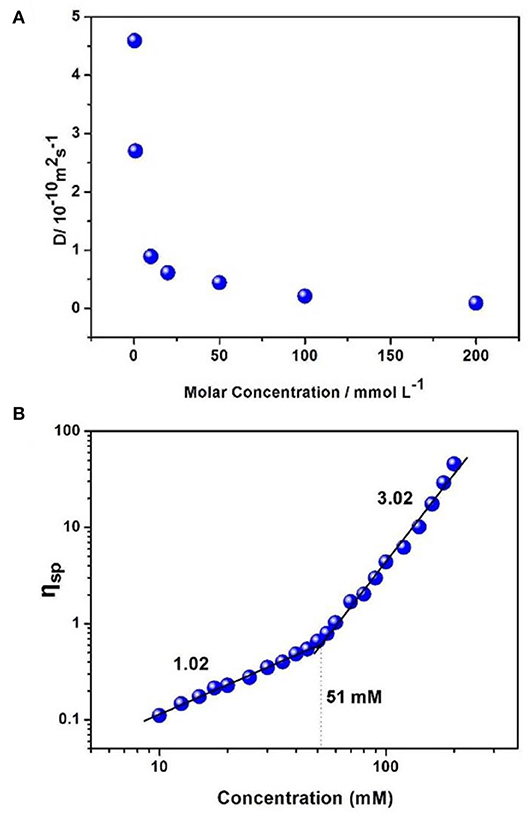
Figure 4. (A) Concentration dependence of diffusion coefficient D (500 MHz, CDCl3, 298 K) of [PdCl2(PhCN)2] and [2]pseudorotaxanes in a 1:1 molar ratio. (B) Specific viscosity of equimolar mixtures of [2]pseudorotaxanes and [PdCl2(PhCN)2] vs. the [PdCl2(PhCN)2]/[2]pseudorotaxanes concentration (DMSO, 298 K).
Viscosity is a characteristic property index for metallosupramolecular polypseudorotaxane. Therefore, viscosity measurements of an equimolar mixture of [2]pseudorotaxanes and [PdCl2(PhCN)2] were carried out in DMSO at 298 K. A double-logarithmic plot of specific viscosity vs. the initial concentrations of [2]pseudorotaxanes is shown in Figure 4B. The slopes of the curves in the low-concentration region tended to 1 (1.02 for [PdCl2(PhCN)2]/[2]pseudorotaxanes), implying that no linear polypseudorotaxane formed (Söntjens et al., 2001; Xiao et al., 2012). When the concentrations of the mixture of [2]pseudorotaxanes and [PdCl2(PhCN)2] increased above the critical polymerization concentration (CPC, approximately 51 mM), a sharp increase in the viscosity was obtained (slope = 3.02), which indicated the formation of linear polypseudorotaxane resulting from strong interactions between [2]pseudorotaxanes and [PdCl2(PhCN)2] (Söntjens et al., 2001; Xiao et al., 2012). This result is also in agreement with the above NMR experiments.
Interestingly, when the concentration exceeded 500 mM, a cross-linked supramolecular gel was observed. That was, upon increasing the concentration of [2]pseudorotaxane and [PdCl2(PhCN)2], metallosupramolecular polypseudorotaxane transformed into a supramolecular gel. Notably, the metal-coordinated polypseudorotaxane gel was sensitive to temperature and could transform into sol reversibly by heating to 60°C and cooling to room temperature (25°C) (Figure 5A). A possible reason for the reversible gel–sol transition is reversible entanglement among linear polypseudorotaxane and the coordination interaction between [2]pseudorotaxane and [PdCl2(PhCN)2]. Heating decreased that interaction and decomposed the polypseudorotaxane, and therefore, gel changed to sol. However, upon cooling, the intermolecular entanglement restored, resulting in the recovery of the supramolecular gel. The morphology of polypseudorotaxane xerogels prepared by freeze-drying was investigated by scanning electron microscopy (SEM). Regular long and fine fiber structures were observed, and the diameter was determined to be 0.2–0.3 μm (Figure 5B). These observations provided further proof that the metallosupramolecular gel was constructed by polypseudorotaxane fibers resulting from [2]pseudorotaxanes and bridging palladium(II).
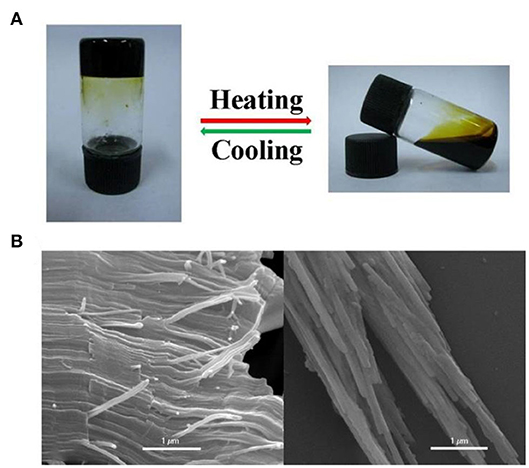
Figure 5. (A) The reversible gel–sol transformation of the polypseudorotaxane gel (500 mM) induced by temperature. (B) SEM images of the supramolecular xerogels.
Conclusions
In summary, a novel metallosupramolecular polypseudorotaxane was successfully fabricated from pillar[5]arene-based [2]pseudorotaxanes and [PdCl2(PhCN)2] in a highly polar solvent of DMSO, which was comprehensively confirmed by various techniques, such as 1H NMR, NOESY, DOSY, DLS, Viscometry, and SEM. The formation of polypseudorotaxane was shown to be dependent on the concentration of [2]pseudorotaxanes/[PdCl2(PhCN)2] and temperature. Moreover, the reversal transition between polypseudorotaxane and [2]pseudorotaxanes can be realized by the successive addition of metal linker [PdCl2(PhCN)2] and competitive ligand PPh3. Significantly, the metal polypseudorotaxane could transform into supramolecular gel when the concentration was above 500 mM, which showed reversibly temperature-induced gel–sol transformation. This study provides a new insight into the construction of macrocycles-based polypseudorotaxane in highly polar organic solvent and benefits to the fabrication of smart materials.
Data Availability Statement
The raw data supporting the conclusions of this article will be made available by the authors, without undue reservation.
Author Contributions
CL, BL, and LL conceived of this project and designed the experiments. HS and WC contributed to most of the experimental work. All authors analyzed the data. CL, WC, and Z-YZ co-wrote the paper. All authors discussed and commented on the paper.
Funding
This work was supported by the National Natural Science Foundation of China (21772118 and 21971192).
Conflict of Interest
The authors declare that the research was conducted in the absence of any commercial or financial relationships that could be construed as a potential conflict of interest.
Supplementary Material
The Supplementary Material for this article can be found online at: https://www.frontiersin.org/articles/10.3389/fchem.2020.00579/full#supplementary-material
References
Cao, D., Kou, Y., Liang, J., Chen, Z., Wang, L., and Meier, H. (2009). A facile and efficient preparation of pillararenes and a pillarquinone. Angew. Chem. Int. Ed. 48, 9721–9723. doi: 10.1002/anie.200904765
Chen, J., Ni, H., Meng, Z., Wang, J., Huang, X., Dong, Y., et al. (2019). Supramolecular trap for catching polyamines in cells as an anti-tumor strategy. Nat. Commun. 10:3546. doi: 10.1038/s41467-019-11553-7
Cui, W., Tang, H., Xu, L., Wang, L., Meier, H., and Cao, D. (2017). Pillar[5]arene-diketopyrrolopyrrole fluorescent copolymer: a promising recognition and adsorption material for adiponitrile by selective formation of a conjugated polypseudorotaxane. Macromol. Rapid Commun. 38:1700161. doi: 10.1002/marc.201700161
Du, G., Moulin, E., Jouault, N., Buhler, E., and Giuseppone, N. (2012). Muscle-like supramolecular polymers: integrated motion from thousands of molecular machines. Angew. Chem. Int. Ed. 51, 12504–12508. doi: 10.1002/anie.201206571
Eichstaedt, K., Wicher, B., Gdaniec, M., and Połonski, T. (2016). Halogen bonded polypseudorotaxanes based on a pillar[5]arene host. Cryst. Eng. Comm. 18, 5807–5810. doi: 10.1039/C6CE01416J
Forgan, R. S., Sauvage, J.-P., and Stoddart, J. F. (2011). Chemical topology: complex molecular knots, links, and entanglements. Chem. Rev. 111, 5434–5464. doi: 10.1021/cr200034u
Guo, D.-S., and Liu, Y. (2014). Supramolecular chemistry of p-sulfonatocalix[n]arenes and Its biological applications. Acc. Chem. Res. 47, 1925–1934. doi: 10.1021/ar500009g
Harada, A., Hashidzume, A., Yamaguchi, H., and Takashima, Y. (2009). Polymeric rotaxanes. Chem. Rev. 109, 5974–6023. doi: 10.1021/cr9000622
Hashidzume, A., Yamaguchi, H., and Harada, A. (2019). Cyclodextrin-based rotaxanes: from rotaxanes to polyrotaxanes and further to functional materials. Eur. J. Org. Chem. 2019, 3344–3357. doi: 10.1002/ejoc.201900090
Hou, X., Ke, C., and Fraser Stoddart, J. (2016). Cooperative capture synthesis: yet another playground for copper-free click chemistry. Chem. Soc. Rev. 45, 3766–3780. doi: 10.1039/C6CS00055J
Hu, X.-Y., Wu, X., Duan, Q., Xiao, T., Lin, C., and Wang, L. (2012). Novel Pillar[5]arene-based dynamic polyrotaxanes interlocked by the quadruple hydrogen bonding ureidopyrimidinone motif. Org. Lett. 14, 4826–4829. doi: 10.1021/ol302149t
Hua, B., Shao, L., Zhang, Z., Liu, J., and Huang, F. (2019). Cooperative silver ion-pair recognition by peralkylated pillar[5]arenes. J. Am. Chem. Soc. 141, 15008–15012. doi: 10.1021/jacs.9b08257
Hua, B., Zhou, W., Yang, Z., Zhang, Z., Shao, L., Zhu, H., et al. (2018). Supramolecular solid-state microlaser constructed from pillar[5]arene-based host–guest complex microcrystals. J. Am. Chem. Soc. 140, 15651–15654. doi: 10.1021/jacs.8b11156
Huang, C.-B., Xu, L., Zhu, J.-L., Wang, Y.-X., Sun, B., Li, X., et al. (2017). Real-time monitoring the dynamics of coordination-driven self-assembly by fluorescence-resonance energy transfer. J. Am. Chem. Soc. 139, 9459–9462. doi: 10.1021/jacs.7b04659
Joseph, J., Eldho, N. V., and Ramaiah, D. (2003). Design of photoactivated DNA oxidizing agents: synthesis and study of photophysical properties and DNA interactions of novel viologen-linked acridines. Chem. Eur. J. 9, 5926–5935. doi: 10.1002/chem.200304936
Kaminker, R., Popovitz-Biro, R., and van der Boom, M. E. (2011). Coordination-polymer nanotubes and spheres: a ligand-structure effect. Angew. Chem. Int. Ed. 50, 3224–3226. doi: 10.1002/anie.201008193
Kato, K., Hori, A., and Ito, K. (2018). An efficient synthesis of low-covered polyrotaxanes grafted with poly(ε-caprolactone) and the mechanical properties of its cross-linked elastomers. Polymer 147, 67–73. doi: 10.1016/j.polymer.2018.05.072
Krogsgaard, M., Nue, V., and Birkedal, H. (2016). Mussel-inspired materials: self-healing through coordination chemistry. Chem. Eur. J. 22, 844–857. doi: 10.1002/chem.201503380
Lee, E., Kim, J., Heo, J., Whang, D., and Kim, K. (2001). A two-dimensional polyrotaxane with large cavities and channels: a novel approach to metal–organic open-frameworks by using supramolecular building blocks. Angew. Chem. Int. Ed. 40, 399–402. doi: 10.1002/1521-3773(20010119)40:2<399::aid-anie399>3.0.co;2-w
Lefebvre, H., Bheda, M., and Gibson, H. W. (2016). Main chain polyamide rotaxanes from aliphatic crown ethers. Polymer 90, 317–330. doi: 10.1016/j.polymer.2016.02.048
Lehn, J.-M. (2017). Supramolecular chemistry: where from? Where to? Chem. Soc. Rev. 46, 2378–2379. doi: 10.1039/C7CS00115K
Li, B., Meng, Z., Li, Q., Huang, X., Kang, Z., Dong, H., et al. (2017). A pH responsive complexation-based drug delivery system for oxaliplatin. Chem. Sci. 8, 4458–4464. doi: 10.1039/C7SC01438D
Li, B., Wang, B., Huang, X., Dai, L., Cui, L., Li, J., et al. (2019). Terphen[n]arenes and quaterphen[n]arenes (n=3–6): one-pot synthesis, self-assembly into supramolecular gels, and iodine capture. Angew. Chem. Int. Ed. 58, 3885–3889. doi: 10.1002/anie.201813972
Li, C. (2014). Pillararene-based supramolecular polymers: from molecular recognition to polymeric aggregates. Chem. Commun. 50, 12420–12433. doi: 10.1039/C4CC03170A
Li, C., Xu, Q., Li, J., Feina, Y., and Jia, X. (2010). Complex interactions of pillar[5]arene with paraquats and bis(pyridinium) derivatives. Org. Biomol. Chem. 8, 1568–1576. doi: 10.1039/b920146g
Li, H., Yang, Y., Xu, F., Liang, T., Wen, H., and Tian, W. (2019). Pillararene-based supramolecular polymers. Chem. Commun. 55, 271–285. doi: 10.1039/C8CC08085B
Liu, Y., Zhao, Y.-L., Zhang, H.-Y., and Song, H.-B. (2003). Polymeric rotaxane constructed from the inclusion complex of β-cyclodextrin and 4,4′-dipyridine by coordination with nickel(II) ions. Angew. Chem. Int. Ed. 42, 3260–3263. doi: 10.1002/anie.200351128
Loeb, S. J. (2007). Rotaxanes as ligands: from molecules to materials. Chem. Soc. Rev. 36, 226–235. doi: 10.1039/B605172N
Ma, J., Meng, Q., Hu, X., Li, B., Ma, S., Hu, B., et al. (2016). Synthesis of a water-soluble carboxylatobiphen[4]arene and its selective complexation toward acetylcholine. Org. Lett. 18, 5740–5743. doi: 10.1021/acs.orglett.6b03005
Ma, X., and Tian, H. (2014). Stimuli-responsive supramolecular polymers in aqueous solution. Acc. Chem. Res. 47, 1971–1981. doi: 10.1021/ar500033n
Ogoshi, T., Kanai, S., Fujinami, S., Yamagishi, T.-A., and Nakamoto, Y. (2008). Para-bridged symmetrical pillar[5]arenes: their lewis acid catalyzed synthesis and host–guest property. J. Am. Chem. Soc. 130, 5022–5023. doi: 10.1021/ja711260m
Ogoshi, T., Yamagishi, T.-A., and Nakamoto, Y. (2016). Pillar-shaped macrocyclic hosts pillar[n]arenes: new key players for supramolecular chemistry. Chem. Rev. 116, 7937–8002. doi: 10.1021/acs.chemrev.5b00765
Rambo, B. M., Gong, H.-Y., Oh, M., and Sessler, J. L. (2012). The “texas-sized” molecular box: a versatile building block for the construction of anion-directed mechanically interlocked structures. Acc. Chem. Res. 45, 1390–1401. doi: 10.1021/ar300076b
Rotzler, J., and Mayor, M. (2013). Molecular daisy chains. Chem. Soc. Rev. 42, 44–62. doi: 10.1039/C2CS35217F
Serreli, V., Lee, C.-F., Kay, E. R., and Leigh, D. A. (2007). A molecular information ratchet. Nature 445, 523–527. doi: 10.1038/nature05452
Shao, L., Hua, B., Hu, X., Stalla, D., Kelley, S. P., and Atwood, J. L. (2020). Construction of polymeric metal–organic nanocapsule networks via supramolecular coordination-driven self-assembly. J. Am. Chem. Soc. 142, 7270–7275. doi: 10.1021/jacs.0c00640
Söntjens, S. H. M., Sijbesma, R. P., van Genderen, M. H. P., and Meijer, E. W. (2001). Selective formation of cyclic dimers in solutions of reversible supramolecular polymers. Macromolecules 34, 3815–3818. doi: 10.1021/ma002010q
Tian, J., Xu, Z.-Y., Zhang, D.-W., Wang, H., Xie, S.-H., Xu, D.-W., et al. (2016). Supramolecular metal-organic frameworks that display high homogeneous and heterogeneous photocatalytic activity for H2 production. Nat. Commun. 7:11580. doi: 10.1038/ncomms11580
Wang, F., Zhang, J., Ding, X., Dong, S., Liu, M., Zheng, B., et al. (2010). Metal coordination mediated reversible conversion between linear and cross-linked supramolecular polymers. Angew. Chem. Int. Ed. 49, 1090–1094. doi: 10.1002/anie.200906389
Wang, L., Xia, D., Chao, J., Zhang, J., Wei, X., and Wang, P. (2019). A dimethoxypillar[5]arene/azastilbene host–guest recognition motif and its applications in the fabrication of polypseudorotaxanes. Org. Biomol. Chem. 17, 6038–6042. doi: 10.1039/C9OB00862D
Wang, P., Wang, R., and Xia, D. (2020). pH-induced transition between single-chain macrocyclic amphiphile and [c2]daisy chain-based bola-type amphiphile and the related self-assembly behavior in water. Front. Chem. 7:894. doi: 10.3389/fchem.2019.00894
Wang, X.-Q., Wang, W., Li, W.-J., Chen, L.-J., Yao, R., Yin, G.-Q., et al. (2018). Dual stimuli-responsive rotaxane-branched dendrimers with reversible dimension modulation. Nat. Commun. 9, 3190. doi: 10.1038/s41467-018-05670-y
Wang, Y., Xu, K., Li, B., Cui, L., Li, J., Jia, X., et al. (2019). Efficient separation of cis- and trans-1,2-dichloroethene isomers by adaptive biphen[3]arene crystals. Angew. Chem. Int. Ed. 58, 10281–10284. doi: 10.1002/anie.201905563
Wei, P., Li, J., Yan, X., and Zhou, Q. (2014). Metallosupramolecular poly[2]pseudorotaxane constructed by metal coordination and crown-ether-based molecular recognition. Org. Lett. 16, 126–129. doi: 10.1021/ol403111e
Winter, A., and Schubert, U. S. (2016). Synthesis and characterization of metallo-supramolecular polymers. Chem. Soc. Rev. 45, 5311–5357. doi: 10.1039/C6CS00182C
Wu, X., Yu, Y., Gao, L., Hu, X.-Y., and Wang, L. (2016). Stimuli-responsive supramolecular gel constructed by pillar[5]arene-based pseudo[2]rotaxanes via orthogonal metal–ligand coordination and hydrogen bonding interaction. Org. Chem. Front. 3, 966–970. doi: 10.1039/C6QO00197A
Xia, D., Lv, X., Chen, K., and Wang, P. (2019). A [2]pseudorotaxane based on a pillar[6]arene and its application in the construction of a metallosupramolecular polymer. Dalton. Trans. 48, 9954–9958. doi: 10.1039/C9DT01713E
Xia, D., Wang, L., Lv, X., Chao, J., Wei, X., and Wang, P. (2018). Dual-responsive [2]pseudorotaxane on the basis of a pH-sensitive pillar[5]arene and its application in the fabrication of metallosupramolecular polypseudorotaxane. Macromolecules 51, 2716–2722. doi: 10.1021/acs.macromol.8b00354
Xiao, T., Li, S.-L., Zhang, Y., Lin, C., Hu, B., Guan, X., et al. (2012). Novel self-assembled dynamic [2]catenanes interlocked by the quadruple hydrogen bonding ureidopyrimidinone motif. Chem. Sci. 3, 1417–1421. doi: 10.1039/c2sc01004f
Xiao, T., Zhou, L., Sun, X.-Q., Huang, F., Lin, C., and Wang, L. (2020). Supramolecular polymers fabricated by orthogonal self-assembly based on multiple hydrogen bonding and macrocyclic host–guest interactions. Chin. Chem. Lett. 31, 1–9. doi: 10.1016/j.cclet.2019.05.011
Xu, K., Zhang, Z.-Y., Yu, C., Wang, B., Dong, M., Zeng, X., et al. (2020). A modular synthetic strategy for functional macrocycles. Angew. Chem. Int. Ed. 59, 7214–7218. doi: 10.1002/anie.202000909
Xue, M., Yang, Y., Chi, X., Zhang, Z., and Huang, F. (2012). Pillararenes, a new class of macrocycles for supramolecular chemistry. Acc. Chem. Res. 45, 1294–1308. doi: 10.1021/ar2003418
Yan, X., Xu, J.-F., Cook, T. R., Huang, F., Yang, Q.-Z., Tung, C.-H., et al. (2014). Photoinduced transformations of stiff-stilbene-based discrete metallacycles to metallosupramolecular polymers. Proc. Natl. Acad. Sci. U.S.A. 111, 8717–8722. doi: 10.1073/pnas.1408620111
Yang, K., Chao, S., Zhang, F., Pei, Y., and Pei, Z. (2019). Recent advances in the development of rotaxanes and pseudorotaxanes based on pillar[n]arenes: from construction to application. Chem. Commun. 55, 13198–13210. doi: 10.1039/C9CC07373F
Zeng, X., Deng, H., Jia, X., Cui, L., Li, J., Li, C., et al. (2018). Construction of [2]rotaxane-based supramolecular polymers driven by wheel-stopper π…π interactions. Chem. Commun. 54, 11634–11637. doi: 10.1039/C8CC07188H
Keywords: pillararenes, host-guest interactions, coordination polymers, polypseudorotaxanes, supramolecular chemistry
Citation: Su H, Chen W, Li L, Li B, Zhang Z-Y and Li C (2020) Coordination-Driven Poly[2]Pseudorotaxanes in Highly Polar Organic Solvent. Front. Chem. 8:579. doi: 10.3389/fchem.2020.00579
Received: 16 May 2020; Accepted: 04 June 2020;
Published: 30 July 2020.
Edited by:
Yong Yao, Nantong University, ChinaReviewed by:
Bin Hua, Zhejiang University, ChinaPeifa Wei, Anhui University, China
Lin Xu, East China Normal University, China
Xiao-Yu Hu, Nanjing University of Aeronautics and Astronautics, China
Copyright © 2020 Su, Chen, Li, Li, Zhang and Li. This is an open-access article distributed under the terms of the Creative Commons Attribution License (CC BY). The use, distribution or reproduction in other forums is permitted, provided the original author(s) and the copyright owner(s) are credited and that the original publication in this journal is cited, in accordance with accepted academic practice. No use, distribution or reproduction is permitted which does not comply with these terms.
*Correspondence: Liang Li, bGlsaWFuZ2xjeCYjeDAwMDQwO3NpdC5lZHUuY24=; Zhi-Yuan Zhang, enp5JiN4MDAwNDA7dGpudS5lZHUuY24=
†These authors have contributed equally to this work