- 1Clinical Laboratory of BGI Health, BGI-Shenzhen, Shenzhen, China
- 2Joint International Research Laboratory of Glycobiology and Medical Chemistry, College of Life Sciences, Northwest University, Xi'an, China
- 3Institute of Interdisciplinary Integrative Medicine Research, Shanghai University of Traditional Chinese Medicine, Shanghai, China
The N-glycans of mammalian glycoproteins vary greatly in structure, and the biological importance of these variations is mostly unknown. It is widely acknowledged that the bisecting N-acetylglucosamine (GlcNAc) structure, a β1,4-linked GlcNAc attached to the core β-mannose residue, represents a special type of N-glycosylated modification, and it has been reported to be involved in various biological processes, such as cell adhesion, fertilization and fetal development, neuritogenesis, and tumor development. In particular, the occurrence of N-glycans with a bisecting GlcNAc modification on proteins has been proven, with many implications for immune biology. Due to the essential functions of bisecting GlcNAc structures, analytical approaches to this modification are highly required. The traditional approach that has been used for bisecting GlcNAc determinations is based on the lectin recognition of Phaseolus vulgaris erythroagglutinin (PHA-E); however, poor binding specificity hinders the application of this method. With the development of mass spectrometry (MS) with high resolution and improved sensitivity and accuracy, MS-based glycomic analysis has provided precise characterization and quantification for glycosylation modification. In this review, we first provide an overview of the bisecting GlcNAc structure and its biological importance in neurological systems, immune tolerance, immunoglobulin G (IgG), and tumor metastasis and development and then summarize approaches to its determination by MS for performing precise functional studies. This review is valuable for those readers who are interested in the importance of bisecting GlcNAc in cell biology.
Introduction
The monosaccharide-amino acid linkage of N-acetylglucosamine (GlcNAc) β1- asparagine (Asn) was originally discovered in biochemical analyses of abundant glycoproteins present in serum, e.g., immunoglobulins (Imperiali and Hendrickson, 1995; Cobb, 2020). Since then, glycans that covalently attached to proteins at Asn residues by an N-glycosidic bond have been termed N-glycans. This attachment usually occurs in a conserved sequence Asn-X-Ser/Thr, in which X can be any amino acid except proline (Pro) (Varki, 2009; Taylor and Drickamer, 2011; Chung et al., 2017).
A distinctive structural feature of N-glycans is the presence of several GlcNAc antennae (branches) that are sequentially synthesized by a series of Golgi-resident glycosyltransferases, N-acetylglucosaminyltransferases (GlcNAc-Ts) (Figure 1) (Schachter, 1991; Kizuka and Taniguchi, 2018). N-glycans can be divided into three categories: high-mannose, hybrid, and complex. Hybrid and complex N-glycans may carry a bisecting GlcNAc group, which forms a new subtype of glycan termed bisecting GlcNAc (Harpaz and Schachter, 1980; Varki, 2009; Nakano et al., 2019). The discovery of this structure lagged behind the detection of other glycan structures due to the limitations of the detection approaches and the peculiarity of its structure. This type of glycan was reported in the 1970s and was detected by a combination of sequential exoglycosidase digestion, methylation derivatization, acetolysis, and Smith degradation from ovalbumin (Yamashita et al., 1978; Nagae et al., 2020). GlcNAc transferred to the 4-position of the β-linked core mannose (Man) residue in complex or hybrid N-glycans by the β1,4-mannosyl-glycoprotein 4-β-N-acetylglucosaminyltransferase (GlcNAc-T III) is considered as a bisecting structure that is usually not considered as an antenna because it cannot be further extended by the proper enzymes (Narasimhan, 1982; Schachter, 1991; Varki, 2009; Miwa et al., 2012; Chen et al., 2016). GlcNAc-T III is encoded by the gene mgat3, which was initially discovered from hen oviducts in 1982 (Narasimhan, 1982; Miwa et al., 2012). It has been reported that its distribution in human tissues is mainly in the brain, liver, placenta, bone marrow, and kidney (Nishikawa et al., 1992; Yoshimura et al., 1995b; Taniguchi et al., 1999; Takamatsu et al., 2004; Schedin-Weiss et al., 2019). So far, there are no reports on any tissue specificity that is related to the functions of this subtype of glycan. The addition of this GlcNAc requires the prior action of GlcNAc-T I (Schachter, 1991; Nakano et al., 2019). The existence of a bisecting GlcNAc prevents α-mannosidase II from trimming and has been proved to inhibit the activities of GlcNAc-T II, GlcNAc-T IV, and GlcNAc-T V in vitro as well (Schachter, 1991, 2014; Varki, 2009; Nakano et al., 2019). The addition of bisecting GlcNAc confers unique lectin recognition properties to this new subtype of glycan (Miwa et al., 2012; Nagae et al., 2013; Link-Lenczowski et al., 2018). B16 mouse melanoma transfected by mgat3 that encodes GlcNAc-T III shows weaker binding to phytohemagglutinin-L (PHA-L) but stronger binding to Phaseolus vulgaris erythroagglutinin (PHA-E). The lectins of PHA-L and PHA-E show specific recognition to multiple antennary glycans and bisecting GlcNAc structures, respectively (Yoshimura et al., 1995c; Varki, 2009; Liu et al., 2016; Wu et al., 2019). This suggests that increased expression of GlcNAc-T III may result in a decrease in multiple branched N-glycan structures. The balance among different types of glycans may play an important role in controlling cell functions.
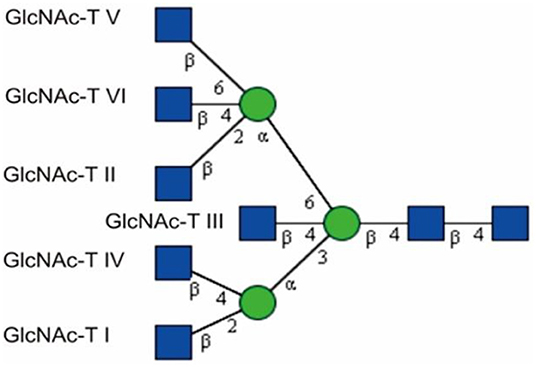
Figure 1. GlcNAc-Man branches catalyzed by GlcNAc-Ts, adapted from Chen (2015) with permission from Qiushi Chen. The first antenna is initiated via the enzyme GlcNAc-T I. GlcNAc-T II creates a biantennary glycan, and GlcNAc-T III yields a bisecting GlcNAc. More branches can be produced via the action of GlcNAc-T IV, V, and VI. GlcNAc,
Man.
The N-glycans of mammalian glycoproteins vary greatly in structure, but the biological importance of these variations is mostly unknown (Bhattacharyya et al., 2002; Reily et al., 2019). It is widely acknowledged that bisecting GlcNAc represents a special type of N-glycosylated modification that is involved in various biological processes, such as cell adhesion, fertilization and fetal development, neuritogenesis, and tumor metastasis and development (Bhattacharyya et al., 2002; Kariya et al., 2008; Akasaka-Manya et al., 2010; Gu et al., 2012; Allam et al., 2015; Zhang et al., 2015; Kizuka and Taniguchi, 2018). The clearly altered levels of bisecting GlcNAc on integrin β1 have been reported to be responsible for early spontaneous miscarriages in humans (Zhang et al., 2015). Tan et al. found that bisecting GlcNAc is able to inhibit hypoxia-induced epithelial-mesenchymal transition in breast cancer cells (Li et al., 2016; Tan et al., 2018). The presence of glycoproteins bearing complex N-glycans with bisecting GlcNAc, fucose (Fuc) and N,N-diacetyllactosamine (LacdiNAc) structures was detected in extracellular vehicles (EVs) from ovarian carcinoma cells; however, the prevention of N-glycosylation processing from high mannose to complex glycans by kifunensine resulted in alterations in the components of EVs and triggered a decrease in several glycoproteins (Gomes et al., 2015). It has also been reported that the occurrence of a bisecting GlcNAc on glycoproteins has many implications in immune biology (el Ouagari et al., 1995; Yoshimura et al., 1996; Takegawa et al., 2005; Pang et al., 2007; Clark, 2014; Chen et al., 2016; Shade et al., 2019). For instance, K562 cells are easily killed by natural killer (NK) cells; however, after being transfected with mgat3, K562 cells acquired NK-cell resistance (Yoshimura et al., 1996; Patankar et al., 1997; Miwa et al., 2012). Therefore, it is very important to take a step forward and review this type of N-glycan. Although the method that is usually used in many studies for bisecting GlcNAc determination is lectin recognition by PHA-E, there are drawbacks to this method. The first disadvantage is low specificity and sensitivity (Dang et al., 2019). This is quite common in most of the lectin-glycan recognition methods. For instance, Sambucus nigra (elderberry) agglutinin (SNA) IV prefers to bind with α2,6-linked sialic acid but also has some binding to α2,3-linked sialic acid (Chen, 2015; Shang et al., 2015; Lis-Kuberka et al., 2019). The second drawback is that lectin recognition could not tell the relative amount of the bisecting GlcNAc structure. Last but not least, this method is not able to reveal the glycosylation site or the glycan structure. Instead, approaches based on mass spectrometry (MS) have been revealed in recent studies to be a suitable tool for expeditiously and precisely investigating this type of glycan.
MS is a technique that measures the mass-to-charge ratios of ions and has been used for small-molecule analysis since World War I (Calvete, 2014). It has a history of playing an important role in glycan or glycan-related studies since the 1960s. In 1968, electron ionization was used in the structural elucidation of di- and tri-saccharides (Kochetkov et al., 1968; Chen, 2015). At that time, it was difficult to detect more complex oligosaccharides since only the molecules possessing higher volatility were analyzable; however, more oligosaccharides in the complex glycans led to decreased volatility. Additionally, the mass range of MS detection restricted study of the complex oligosaccharides with higher molecular weights. In the late 1970s, MS was used for the first time in the study of blood glycoproteins from Antarctic fish, in which a proline-containing glycopeptide that had a disaccharide was sequenced; the structure of this disaccharide was identified as galactosyl-N-acetylgalactosamine (Morris et al., 1978; Dell and Morris, 2001; Bielik and Zaia, 2010). Several years later, Dell and Morris performed the first structural analysis of glycans using a fast-atom-bombardment mass spectrometer (FAB MS) (Dell et al., 1983; Dell and Morris, 2001). With its rapid development, MS has now significantly improved in its analytical scope, speed, and depth. For glycopeptide or glycan structure analysis, the Orbitrap mass spectrometers with higher resolution and accuracy are a good choice under MSn (n > 2) mode coupled with or without liquid chromatography (LC). Matrix-assisted laser desorption ionization time-of-flight (MALDI-TOF/TOF) MS with higher collision energies is also a valuable choice for MS1/MS2 analysis of glycans (Chen, 2015; Chen et al., 2016). Electrospray ionization (ESI)-TOF MS has also been reported to be efficient for glycosylation analysis of intact IgG molecules (Wei et al., 2019).
The Functions of Bisecting GlcNAc Modification
In Neurological Systems
Akasaka-Manya et al. discovered that the mRNA levels of mgat3 were elevated in the temporal cortex of the brain in patients with Alzheimer's disease (AD) (Akasaka-Manya et al., 2010), which accelerated studies on the tissue distribution of GlcNAc-T III expression, with the conclusion that it was most highly expressed in the nervous system (Shimizu et al., 1993; Kizuka et al., 2016b; Kizuka and Taniguchi, 2018).
In 1993, Shimizu et al. reported that the main glycan structures detected in the mouse cerebrum, cerebellum, and brain stem are bisected, as is proposed in Figure 2 (Shimizu et al., 1993; Nagae et al., 2016). Later, Shigeta et al. found that GlcNAc-T III promoted β1 integrin-mediated neuritogenesis triggered by serum deprivation in Neuro2a cells and that the neuritogenesis induced by GlcNAc-T III was functionally blocked by anti-β1 integrin monoclonal antibody (DF5) (Shigeta et al., 2006). In addition, β1 integrin is found to be regulated as a target protein by GlcNAc-T III, and this could be supported by a study showing that the amount of β1 integrin in erythroagglutinating-phytohemagglutinin (E4-PHA)-associated complexes significantly increased in GlcNAc-T III transfectants compared with that in mock transfectants (Shigeta et al., 2006).
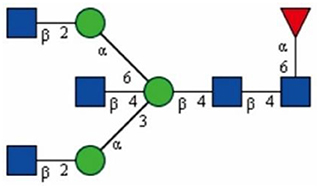
Figure 2. The bisecting GlcNAc structure proposed by Shimizu et al., drawn based on the information obtained from Shimizu et al. (1993). The proposed linkage between each monosaccharide is labeled. GlcNAc,
Man.
Fuc.
AD is a progressive, neurodegenerative disease in which there are deficits in memory and cognitive functions; more importantly, it is a global health problem (Abbott, 2011; Scheltens et al., 2016; Kizuka and Taniguchi, 2018; Schedin-Weiss et al., 2019). However, current treatments are still only symptomatic (Winblad et al., 2016; Schedin-Weiss et al., 2019). It is necessary to understand that many solid studies support connections between AD and aberrant protein glycosylation, considering the fact that glycoproteins including tau, Aβ-precursor protein (APP), and β-site APP-cleaving enzyme-1 (BACE-1) are involved in AD pathogenesis and have been found to show altered glycosylation patterns (Halim et al., 2011; Schedin-Weiss et al., 2014, 2019; Kizuka et al., 2015). More importantly, APP and BACE-1 contain glycosylation modifications with bisecting GlcNAc structures (Akasaka-Manya et al., 2008, 2010; Schedin-Weiss et al., 2014; Kizuka et al., 2015, 2016a). Bisecting GlcNAc modifications have shown the capacity to stabilize BACE1 protein under conditions of oxidative stress (Kizuka et al., 2016a), and the increased contents of bisecting GlcNAc in AD brains might function as an adaptive response, which protects the brains from the damage caused by additional beta-amyloid yields (Akasaka-Manya et al., 2010). One study showed that the lack of bisecting GlcNAc to BACE1 directed the transport of this protein to the lysosome and accelerated its degradation, which resulted in the less accumulation of β amyloid in AD (Kizuka et al., 2015; Kizuka and Taniguchi, 2018). These findings have highlighted the importance of bisecting GlcNAc modification in the nervous system. However, the underlying mechanism is still not clear.
AD is a chronic disease and begins to develop decades before the first symptoms appear, which suggests the importance of investigating early changes (e.g., glycan alteration) for improving early diagnosis. We have recently published our findings on glycosylation changes in AD research. An increase in bisecting N-GlcNAc modifications was observed in cerebrospinal fluid (CSF) from AD patients. The further investigation of CSF from 242 patients with subjective cognitive impairment (SCI), mild cognitive impairment (MCI), or AD revealed more glycoproteins binding to PHA-E in MCI and AD than in SCI (Schedin-Weiss et al., 2019). Therefore, these findings could be essential for developing early AD diagnosis biomarkers and understanding the early stages of AD development, which might be additionally beneficial for designing novel AD treatment strategies. The challenges in the future will be to perform comprehensive and detailed glycoproteomic and glycomic analysis of those glycoproteins with bisecting GlcNAc modification.
In Immune Tolerance
In 1996, Clark et al. proposed the human fetoembryonic defense system hypothesis (hu-FEDS) (Clark et al., 1996; Pang et al., 2016). The basic concept of this hypothesis is that glycoproteins expressed in the reproductive system and gametes can either inhibit immune responses or prevent rejection. Indeed, glycoproteins in human seminal plasma and the pregnant uterus have been shown to suppress immune responses in vitro, specifically for those glycoproteins containing bisecting GlcNAc structures (Bolton et al., 1987; Kelly and Critchley, 1997; Clark, 2014; Szczykutowicz et al., 2019).
Bisecting GlcNAc structures have been reported to possess immune suppression functions. For instance, K562 cells are easily killed by natural killer (NK) cells; however, after being transfected with the gene that encodes GlcNAc-T III, K562 cells possessing more bisecting GlcNAc attain NK cell resistance (Yoshimura et al., 1996; Patankar et al., 1997). Natural killer (NK) cells are the major type of immune cells found in the human uterus, which indicates that they potentially target sperm (King et al., 1991; Clark, 2014). Human sperm were found to express bisecting GlcNAc structures, which explains why sperm are not killed by the maternal immune system when entering the female as a foreign substrate and thus support hu-FEDS (Pang et al., 2007; Clark, 2014; Szczykutowicz et al., 2019). Additionally, abundant bisecting GlcNAc glycans were detected in human syncytiotrophoblasts (STB) and cytotrophoblasts (CTB) (Chen et al., 2016). It is most likely that the maternal immune system was suppressed due to the presence of bisecting GlcNAc glycans and that the fetus benefited from this suppression; the mother could nourish a fetus (similar to a foreign organ as the father contributes to its half genome) within her body for several months without rejection. The possible mechanism underlying this suppression could be that the glycoconjugates interacted with lectins that linked to particular signal transduction pathways modulating immune cell functions. For instance, α-2,3-linked sialic acid on soluble CD52, a glycoprotein of 12 amino acids anchored to glycosylphosphatidylinositol, could mediate T-cell suppression by binding to siglec-10 (Clark, 2014; Shathili et al., 2019). It is possible that bisecting GlcNAc can function in a similar way to suppress NK cells.
On Immunoglobulin G (IgG)
IgG is an important molecule in the immune system. IgG regulates its immune functions through complement and cellular IgG-Fc gamma receptors (FcγR) (Dekkers et al., 2016). It contains a highly conserved N-linked glycan at position Asn297 in the Fc region (Arnold et al., 2007; Dekkers et al., 2016; Kiyoshi et al., 2017). This glycan is composed of variable levels of fucose, galactose, and sialic acid and bisecting GlcNAc (Le et al., 2016; Gudelj et al., 2018; Lu and Holland, 2019; Shade et al., 2019). It is widely acknowledged that the Fc-glycan has an influence on the biological activities of IgG. For example, a lack of fucose in the Fc glycan significantly improves binding to the human FcγR III, and this result is applied to improve the efficacy of therapeutic monoclonal antibodies. Attachment of bisecting GlcNAc to the Fc glycan has been described to induce antibody-dependent cell-mediated cytotoxicity (ADCC) (Shields et al., 2002; Hodoniczky et al., 2005; Dekkers et al., 2016).
Studies focused on characterizing the IgG- and IgA-linked glycans have shown that glycans are differentially expressed in the setting of autoimmunity. For instance, patients <50 years old with Lambert-Eaton myasthenic syndrome (an autoimmune disease in which the immune system attacks the body's own tissues) show increased levels of bisecting GlcNAc on IgG1 and IgG2 (Selman et al., 2011; Maverakis et al., 2015). This suggests that particular glycan types may be potential biomarkers for certain diseases.
In Tumor Metastasis and Development
It is essential to understand the factors that affect tumor progression so as to determine how to control tumor growth and metastasis. It has been reported that more multiple branched N-glycan modifications occur in tumor cells due to the higher activity of GlcNAc-T V, which promotes tumor cell metastasis (Dennis et al., 1987; Gu et al., 2009; Kizuka and Taniguchi, 2016). A possible explanation for this is that β1,6-GlcNAc-branched N-glycans can be preferentially processed by β1,4 Gal-T, and β1,3 GlcNAc-T to form poly-N-acetyllacotosamine (poly-LacNAc) for elongation of N-glycans, which could be further modified into the motifs involved in cancer metastasis, such as sialyl Lewis X (Yamadera et al., 2018). As mentioned above, the increased expression of GlcNAc-T III prevented the formation of multiple branch glycans, as GlcNAc-T V could not extend the glycans beyond the bisecting GlcNAc structure and thus inhibited tumor cell metastasis (Dennis et al., 1987; Gu et al., 2009; Taniguchi and Korekane, 2011). Two years ago, it was reported that bisecting GlcNAc structures could inhibit hypoxia-induced epithelial-mesenchymal transition in breast cancer (Tan et al., 2018). However, the mechanism underlying is still not clear. It has been speculated that the addition of bisecting GlcNAc to the key glycoproteins of signaling transduction, e.g., growth factors, integrins, and cytokine receptors, has its special signaling strength under hypoxia. Actually, observations of non-solid tumors contradict this explanation. GlcNAc-T III was more activated in patients with chronic myelogeneous leukemia in blast crisis (CML-BC) and in patients with multiple myeloma (MM) (Yoshimura et al., 1995a).
Alterations in glycosylation are usually considered as a hallmark of cancer, and the protein with the most extensive studies of its glycosylation is E-cadherin (de-Freitas-Junior et al., 2013). In 2019, researchers found that E-cadherin was required for metastasis in multiple breast cancer models (Padmanaban et al., 2019) and that it contained bisecting GlcNAc modifications (Kitada et al., 2001; de-Freitas-Junior et al., 2013). The addition of bisecting GlcNAc to E-cadherin was found to negatively regulate the tyrosine phosphorylation of β-catenin (Kitada et al., 2001; Takahashi et al., 2009), and GlcNAc T-III knockdown cells displayed a membrane delocalization of E-cadherin, resulting in its cytoplasmic accumulation (Pinho et al., 2009). As a result, the deactivated β-catenin failed to enhance cell growth or oncogenesis as it formed a tight complex with E-cadherin and could not be translocated into the nuclei (Gu et al., 2009). These results suggest that bisecting GlcNAc plays important roles in tumor metastasis and development. Therefore, it is reasonable that certain aberrant glycosylation (e.g., bisecting) patterns could be used as biomarkers for the progression of particular diseases, including cancer metastasis and development (Dennis et al., 1999; Tan et al., 2018).
Others
It has been reported there are multiple functions of bisecting GlcNAc in other cell biology processes. The bisecting GlcNAc structure in N-glycans of adenylyl cyclase III was proved to be an enhancer of enzyme activity (Li et al., 2007). The bisecting GlcNAc structure has been found to inhibit stroma-dependent hemopoiesis in transgenic mice expressing GlcNAc-T III (Yoshimura et al., 1998).
The Detection of Bisecting GlcNAc Structures
The approaches reviewed here have been released and proved as efficient tools for bisecting GlcNAc modification studies. These bisecting GlcNAc determination approaches are reviewed based on two detection targets, namely, glycan and glycopeptide levels. Before the samples are subjected to glycan or glycopeptide analysis, cell or tissue samples need to be processed as previously described (North et al., 2010; Chen, 2015; Chen et al., 2016); the procedure for sample preparation will not be addressed here.
Approaches for Detecting Glycan Levels
β1,4-Galactosyltransferase Reaction
β1,4-galactosyltransferase is an enzyme that transfers a galactose (Gal) from UDP-Gal to GlcNAc and forms the disaccharide unit of Galβ1,4GlcNAc in the antenna of complex and hybrid glycans (Schwientek et al., 1996; Chen et al., 2016). However, if a GlcNAc is at the bisected position, it will not be processed by this enzyme, and there are no changes for glycans containing a bisecting GlcNAc (Pang et al., 2007; Qasba et al., 2008;Chen, 2015).
In our previous study, we adopted this method to prove the presence of a bisecting GlcNAc structure in glycans through the β1,4-galactosyltransferase reaction, as is shown in Figure 3 (Chen et al., 2016). Using this strategy, the glycan sample was treated by the enzyme at 37°C for 24 h to ensure a complete reaction (Chen, 2015). The glycans at m/z 2,489, 2,850, and 3,212 were chosen as the targets of observation because these glycans contain a potential substrate (an unmodified GlcNAc) for β1,4-galactosyltransferase. If the structures included a reactable GlcNAc group, a Gal residue would be incorporated into the structure, resulting in the glycans at m/z 2,489, 2,850, and 3,212 undergoing a shift in m/z to 2,693, 3,055, and 3,416, respectively. Figure 3 displays two typical spectra scanned by MALDI-TOF MS with or without β1,4-galactosyltransferase treatment. This figure clearly shows that after the enzyme treatment, there are no obvious changes in the three glycan comparison groups at m/z 2,489 and 2,693, 2,850 and 3,055, and 3,212 and 3,416. This result demonstrates that the GlcNAc in these three glycan structures cannot be extended by β1,4-galactosyltransferase and the bisecting GlcNAc present in these glycans.
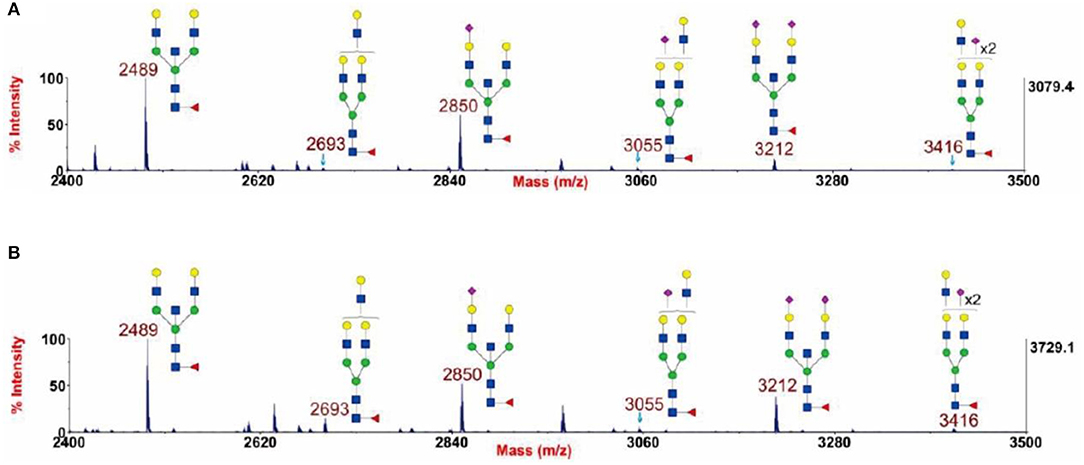
Figure 3. Annotated MALDI-TOF MS spectra of permethylated N-glycans (A) and permethylated β1,4-galactosyltransferase treated N-glycans (B) from human cytotrophoblasts (CTB), adapted from Chen et al. (2016) with permission from Qiushi Chen. GlcNAc,
Man,
Gal,
Fuc,
NeuAc.
The processing performed using this approach is quite simple, and the interpretation of the results is so direct that there is no need for any software for further data analysis. The signal alteration from glycans is basic and essential for this method. However, some exceptions have been observed in the application of this approach in vitro. A research article reported a successful galactosylation occurring beyond the bisecting GlcNAc in the structure of GlcNAcMan3GlcNAc2 in vitro (Zou et al., 2011). In addition, we found that a chemoenzymatically synthesized glycan structure (Galb1-4GlcNAcb1-2 Mana1-6(Galb1-4GlcNAcb1-4)(Galb1-4GlcNAcb1-2Mana1-3)Manb1-4GlcNAcb1-4(Fuca1-6)GlcNAc) containing galactosylated bisecting GlcNAc was clearly labeled for Functional Glycomics (CFG) array (CFG, 2012).
Therefore, it would be better to combine the approach of galactosyltransferase reaction with other methods listed in this review to confirm the presence of a bisecting GlcNAc structure in glycans. In 2016, we adopted this method together with gas chromatography-mass spectrometry (GC-MS) to study the bisecting GlcNAc modification (Chen et al., 2016), which will be described in the following section.
Gas Chromatography-Mass Spectrometry (GC-MS)
GC-MS methods adopted to detect bisecting GlcNAc have been described previously (Ciucanu, 2006; North et al., 2010). Considering the volatile analytes necessary for GC-MS detection, the glycan samples must be derivatized into partially methylated alditol acetates (PMAA) before being subjected to MS, as has been published in some reports (North et al., 2010; Chen, 2015): the permethylated glycan sample was treated with a NaBD4 solution and was then dried under nitrogen assistance, followed by acetylation treatment with acetic anhydride. As shown in Figure 1, the bisecting GlcNAc is directly attached to the C4 position of the β-linked Man, which possesses a characteristic component of 3,4,6-linked Man, which is a unique signal for the identification of bisecting GlcNAc by GC-MS.
Figure 4 shows the structural molecule of the PMAA derivative of a 3,4,6-linked-D-mannopyranosyl residue. The fragmentation of this molecule can yield two characteristic ions with m/z of 118 and 333.
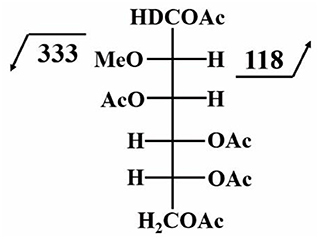
Figure 4. Characteristic fragment ions of the PMAA derivative of a 3,4,6-linked-D-mannopyranosyl residue; this figure is modified from CCRC (2020) with permission from CCRC.
We have adopted this permethylation method and combined GC-MS detection to prove the presence of bisecting GlcNAc in human CTB and STB (Chen et al., 2016). As shown in Table 1, the characteristic fragment ions of m/z 118 and 333 were simultaneously detected for the group of 3,4,6-linked Man, which supported the existence of bisecting GlcNAc.
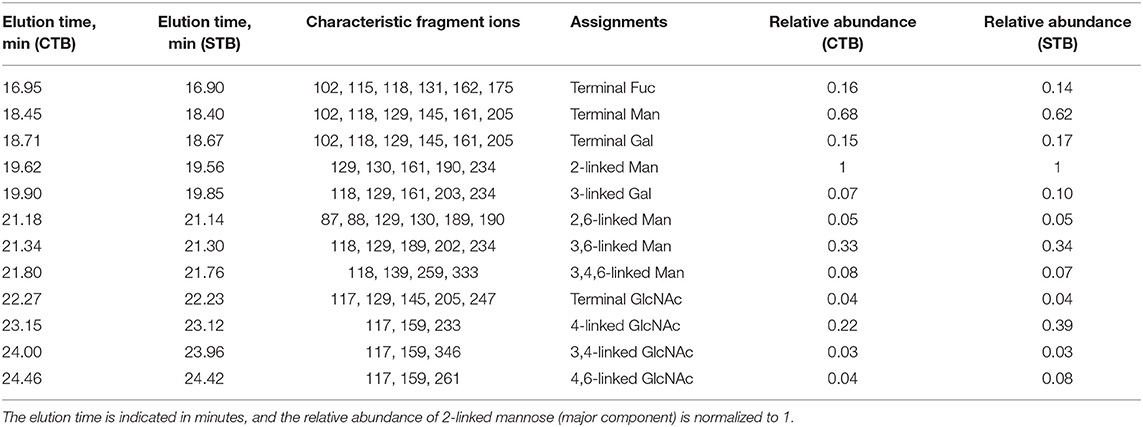
Table 1. Summary of the GC-MS linkage analysis of partially methylated alditol acetates derived from N-glycans of cytotrophoblasts (CTB) and syncytiotrophoblasts (STB), adapted from Chen et al. (2016) with permission from Qiushi Chen.
In this method, GC was used for the separation of analytes and it thus has higher resolution for complex small molecules; however, the glycan samples must be derivatized into PMAA for GC-MS analysis, and the reaction efficiency affects the quantification of the bisecting GlcNAc structures.
Multi-Stage Mass Spectrometry (MSn)
In principle, this method is quite similar to GC-MS detection because the detection of bisected glycan structures can be accomplished by identifying the presence of the 3,4,6-linked Man (Allam et al., 2015). In this method, the Obitrap MS was used for multiple fragmentation (Allam et al., 2015).
Figure 5 shows the logical order of the MS8 approach that was used for detecting bisecting GlcNAc in the glycan at m/z 2489.25, which is a bitennary, core-fucosylated glycan. Theoretically, the MS7 spectrum of the glycan at m/z 2489.25 should display the characteristic ion of the bisected glycan at m/z 444.18, which would support the presence of 3,4,6-linked Man. Additionally, MS8 analysis would be further carried out to show that the ion at m/z 444.18 is truly a glycan fragment ion indeed and is not noise or a contaminant.
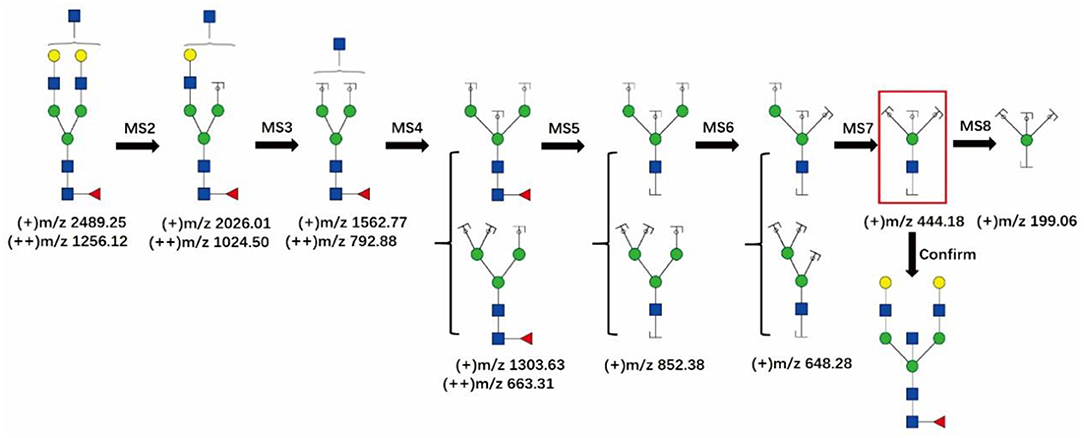
Figure 5. The MS8 approach for confirming the presence of bisecting GlcNAc structures. The fragment ion at m/z 444.18 in the red frame is the characteristic ion of the bisecting GlcNAc glycans. GlcNAc,
Man,
Gal,
Fuc.
This method is able to target the bisecting GlcNAc structure of interest. More importantly, it does not require additional sample processing. However, it is highly dependent on the MS analyzer, as well as on operator techniques. Usually, only glycans with higher abundances can provide good signals with multiple fragmentation under MSn mode.
Approach for Detecting Glycopeptide Levels
Due to the rapid development of MS techniques, it is possible to perform analysis of glycopeptides composed of the peptides together with their glycans. In addition to detecting bisecting GlcNAc, MS can also confirm the glycosylation sites as well as the glycan components. The method introduced here for bisecting GlcNAc detection references the paper published in Analytical Chemistry in 2019 (Dang et al., 2019), which was designed to detect bisecting GlcNAc in glycopeptides by their characteristic ion(s) in fragmented MS/MS spectra under low-energy collisions. The characteristic ion(s) are either [Pep+HexNAc3Hex] or [Pep+FucHexNAc3Hex] or both. In this paper, 25 glycoproteins (possessing bisecting GlcNAc) were identified from rat kidney tissue, four of which (Q01129 decorin, P17046 lysosome-associated membrane glycoprotein 2, P07861 neprilysin, and B5DFC9 nidogen-2) were found to be protein analogs of those identified in our human amnion samples. More importantly, one of these glycoproteins, neprilysin, has the same bisecting GlcNAc location (site N285) as the human neprilysin (P08473) (data not shown).
This method can simultaneously obtain precise information regarding the heterogeneity of glycosylation, including the modification sites and their linked glycan structures, which is useful for the functional study of target proteins. More importantly, this method does not require additional sample processing. However, as mentioned by Dang et al., the effectiveness of the method may be impacted by multiple parameters, such as glycopeptide structures (Dang et al., 2019). It also places greater requirements on the MS analyzer, and the profiling coverage of the glycosylation is limited because sufficient information regarding the peptides and the glycans in the MS2 spectra must be obtained for identification.
We summarize the advantages and disadvantages of each method mentioned above in Table 2 to help researchers to make appropriate choices according to the laboratory instrumentation and conditions.
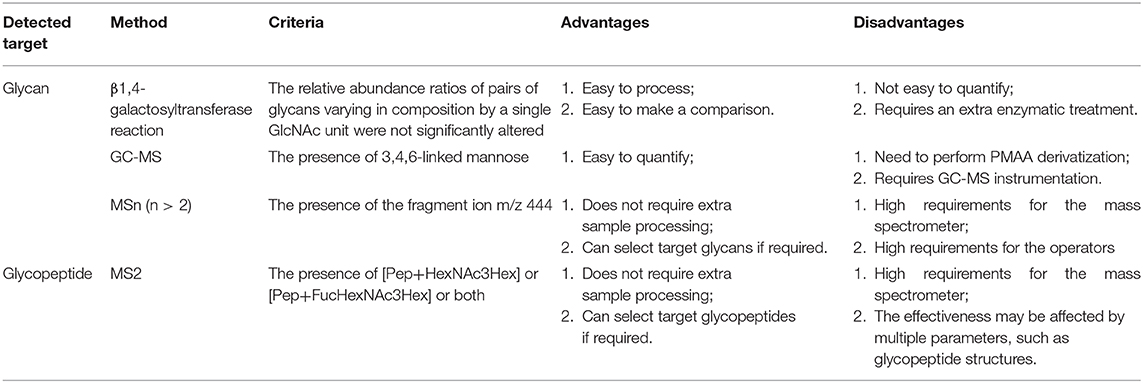
Table 2. A comparison of different approaches for bisecting GlcNAc characterization based on MS detection.
Synthesis of Bisecting Glycans
With more studies focusing on the special bisecting glycans, the importance of this type of glycan in cell biology has been discovered. Indeed, glycosylation modification plays an important role in protein functions due to participation in the functional domain of protein configuration (Luber et al., 2018). It has been reported that human IgG, an important immune system molecule, possesses glycans containing bisecting GlcNAc (Le et al., 2016; Lu and Holland, 2019; Shade et al., 2019). Thus, only synthesizing the sequence of proteins, but not the glycan chains, is insufficient for protein function. Syntheses of glycans or glycoproteins containing bisecting GlcNAc structures have been reported in many papers (Wang et al., 2009; Castilho et al., 2011; Luber et al., 2018; Manabe et al., 2018; Yang et al., 2018). Synthesis of glycans or glycoproteins containing bisecting GlcNAc structures is helpful for glycomic and glycoproteomic research and will improve the development of protein-based therapeutics and the generation of glycan-engineered therapeutic antibodies (Castilho et al., 2011, 2015).
In 2007, Unverzagt et al. reported the first chemical synthesis of highly branched pentaantennary N-glycans and derivatives with bisecting GlcNAc modifications (Eller et al., 2007). The chemical synthesis of a bisecting GlcNAc could also be achieved through [4+2] and [6+2] glycosylations. This synthetic method reduces the number of reaction steps but faces two difficulties, namely, low yields and poor synthesis selectivity for key glycosylations (Manabe et al., 2018). A modular synthesis of 16 cores of mammalian complex-type N-glycans with optional core fucose and bisecting GlcNAc has been established by Unverzagt et al., and core fucosylated and bisected N-glycans could be synthesized with unprecedented efficiency and purity by integrating a one-pot protocol (Luber et al., 2018).
Biosynthesis of glycoproteins with bisecting GlcNAc glycans has been performed in glycoengineered Nicotiana benthamiana, which lacks plant-specific N-glycosylation (Castilho et al., 2011) but expresses a modified version of human GlcNAc-T III. However, GlcNAc-T III is sometimes not very active when fused to the Golgi α-mannosidase II-cytoplasmic tail, transmembrane domain, and stem (GMII-CTS) region. Therefore, more studies are required to overcome these difficulties.
Conclusions
Researchers are now beginning to realize the importance of bisecting GlcNAc glycans. We reviewed its importance in neurological systems, immune tolerance, IgG, and tumor metastasis and development and then introduced a series of MS approaches for bisecting GlcNAc detection. Compared to the traditional lectin recognition method, MS-based methods can be quantifiable, can target the glycan and glycopeptide of interest, and can provide details of the glycosylation sites and glycan components. In addition, MS approaches are more sensitive, and limits on sample amounts are overcome in glycosylation studies. However, there are bottlenecks in the use of current MS technology to detect the bisecting GlcNAc. The sensitivity of MS detection to glycosylation modification is still limited, and thus specific enrichment of the glycans or glycopeptides is needed. Especially for the MSn analysis, only glycans with higher abundance could be interpreted in detail and with accuracy. In addition, the construction of high-quality MSn spectral databases as well as an understanding of fragmentation mechanisms are also vital for developing the in silico fragmentation tools. Precise prediction of bisecting GlcNAc will be achieved via developing a probabilistic generative model for the CID/HCD fragmentation by machine learning techniques. This review will be valuable for those researchers who are interested in the importance of bisecting GlcNAc in cell biology and can conduct studies in this field and will be helpful for advancing our understanding of bisecting GlcNAc.
Author Contributions
QC participated in the discussion and drafted the manuscript. YR participated in the discussion and made corrections to the manuscript. ZT and FG participated in the discussion and made corrections to the manuscript. All authors have checked and approved the final manuscript.
Funding
This work was supported by the National Key R&D Program of China (No. 2017YFC0908403) and the National Natural Science Foundation of China (No. 31500670).
Conflict of Interest
The authors declare that the research was conducted in the absence of any commercial or financial relationships that could be construed as a potential conflict of interest.
Acknowledgments
We thank the Complex Carbohydrate Research Center (CCRC); a figure is modified from the CCRC Spectral Database (https://www.ccrc.uga.edu/specdb/ms/pmaa/pframe.html).
References
Akasaka-Manya, K., Manya, H., Sakurai, Y., Wojczyk, B. S., Kozutsumi, Y., Saito, Y., et al. (2010). Protective effect of N-glycan bisecting GlcNAc residues on β-amyloid production in Alzheimer's disease. Glycobiology 20, 99–106. doi: 10.1093/glycob/cwp152
Akasaka-Manya, K., Manya, H., Sakurai, Y., Wojczyk, B. S., Spitalnik, S. L., and Endo, T. (2008). Increased bisecting and core-fucosylated N-glycans on mutant human amyloid precursor proteins. Glycoconj J. 25:12. doi: 10.1007/s10719-008-9140-x
Allam, H., Aoki, K., Benigno, B. B., McDonald, J. F., Mackintosh, S. G., Tiemeyer, M., et al. (2015). Glycomic analysis of membrane glycoproteins with bisecting glycosylation from ovarian cancer tissues reveals novel structures and functions. J. Proteome Res. 14, 434–446. doi: 10.1021/pr501174p
Arnold, J. N., Wormald, M. R., Sim, R. B., Rudd, P. M., and Dwek, R. A. (2007). The impact of glycosylation on the biological function and structure of human immunoglobulins. Annu. Rev. Immunol. 25, 21–50. doi: 10.1146/annurev.immunol.25.022106.141702
Bhattacharyya, R., Bhaumik, M., Raju, T. S., and Stanley, P. (2002). Truncated, inactive N-acetylglucosaminyltransferase III (GlcNAc-TIII) induces neurological and other traits absent in mice that lack GlcNAc-TIII. J. Biol. Chem. 277, 26300–26309. doi: 10.1074/jbc.M202276200
Bielik, A. M., and Zaia, J. (2010). Historical overview of glycoanalysis. Methods Mol. Biol. 600, 9–30. doi: 10.1007/978-1-60761-454-8_2
Bolton, A. E., Pockley, A. G., Clough, K. J., Mowles, E. A., Stoker, R. J., Westwood, O. M., et al. (1987). Identification of placental protein 14 as an immunosuppressive factor in human reproduction. Lancet 1, 593–595. doi: 10.1016/S0140-6736(87)90235-2
Calvete, J. J. (2014). The expanding universe of mass analyzer configurations for biological analysis. Methods Mol. Biol. 1072, 61–81. doi: 10.1007/978-1-62703-631-3_6
Castilho, A., Gattinger, P., Grass, J., Jez, J., Pabst, M., Altmann, F., et al. (2011). N-glycosylation engineering of plants for the biosynthesis of glycoproteins with bisected and branched complex N-glycans. Glycobiology 21, 813–823. doi: 10.1093/glycob/cwr009
Castilho, A., Gruber, C., Thader, A., Oostenbrink, C., Pechlaner, M., Steinkellner, H., et al. (2015). Processing of complex N-glycans in IgG Fc-region is affected by core fucosylation. MABS 7, 863–870. doi: 10.1080/19420862.2015.1053683
CCRC (2020). GC-EIMS of Partially Methylated Alditol Acetates Database [Online]. University of Georgia: Complex Carbohydrate Research Center. Available online at: https://www.ccrc.uga.edu/databases/index.php# (accessed February 16, 2020).
CFG (2012). CFG Mammalian-Type Glycan Microarray. (Boston, MA: Beth Israel Deaconess Medical Center: Harvard Medical School.
Chen, Q. (2015). Mass Spectrometric Investigation of Biomedically Important Glycosylation (Ph.D). London, UK: Imperial College London.
Chen, Q., Pang, P. C., Cohen, M. E., Longtine, M. S., Schust, D. J., Haslam, S. M., et al. (2016). Evidence for differential glycosylation of trophoblast cell types. Mol. Cell. Proteomics 15, 1857–1866. doi: 10.1074/mcp.M115.055798
Chung, C. Y., Majewska, N. I., Wang, Q., Paul, J. T., and Betenbaugh, M. J. (2017). SnapShot: N-glycosylation processing pathways across kingdoms. Cell 171, 258–258.e251. doi: 10.1016/j.cell.2017.09.014
Ciucanu, I. (2006). Per-O-methylation reaction for structural analysis of carbohydrates by mass spectrometry. Anal. Chim. Acta. 576, 147–155. doi: 10.1016/j.aca.2006.06.009
Clark, G. F. (2014). The role of glycans in immune evasion: the human fetoembryonic defence system hypothesis revisited. Mol. Hum. Reprod. 20, 185–199. doi: 10.1093/molehr/gat064
Clark, G. F., Oehninger, S., Patankar, M. S., Koistinen, R., Dell, A., Morris, H. R., et al. (1996). A role for glycoconjugates in human development: the human feto-embryonic defence system hypothesis. Hum. Reprod. 11, 467–473. doi: 10.1093/HUMREP/11.3.467
Cobb, B. A. (2020). The history of IgG glycosylation and where we are now. Glycobiology 30, 202–213. doi: 10.1093/glycob/cwz065
Dang, L., Shen, J., Zhao, T., Zhao, F., Jia, L., Zhu, B., et al. (2019). Recognition of bisecting N-glycans on intact glycopeptides by two characteristic ions in tandem mass spectra. Anal. Chem. 91, 5478–5482. doi: 10.1021/acs.analchem.8b05639
de-Freitas-Junior, J. C., Carvalho, S., Dias, A. M., Oliveira, P., Cabral, J., Seruca, R., et al. (2013). Insulin/IGF-I signaling pathways enhances tumor cell invasion through bisecting GlcNAc N-glycans modulation. An interplay with E-cadherin. PLoS ONE 8:e81579. doi: 10.1371/journal.pone.0081579
Dekkers, G., Plomp, R., Koeleman, C. A., Visser, R., von Horsten, H. H., Sandig, V., et al. (2016). Multi-level glyco-engineering techniques to generate IgG with defined Fc-glycans. Sci. Rep. 6, 36964. doi: 10.1038/srep36964
Dell, A., and Morris, H. R. (2001). Glycoprotein structure determination by mass spectrometry. Science 291, 2351–2356. doi: 10.1126/science.1058890
Dell, A., Morris, H. R., Egge, H., Vonnicolai, H., and Strecker, G. (1983). Fast-atom-bombardment mass-spectrometry for carbohydrate-structure determination. Carbohyd. Res. 115:12. doi: 10.1016/0008-6215(83)88133-6
Dennis, J. W., Granovsky, M., and Warren, C. E. (1999). Protein glycosylation in development and disease. Bioessays 21, 412-421 doi: 10.1002/(SICI)1521-1878(199905)21:5<412::AID-BIES8>3.0.CO;2-5
Dennis, J. W., Laferte, S., Waghorne, C., Breitman, M. L., and Kerbel, R. S. (1987). β1-6 branching of Asn-linked oligosaccharides is directly associated with metastasis. Science 236, 582–585. doi: 10.1126/science.2953071
el Ouagari, K., Teissie, J., and Benoist, H. (1995). Glycophorin A protects K562 cells from natural killer cell attack. Role of oligosaccharides. J. Biol. Chem. 270, 26970–26975. doi: 10.1074/jbc.270.45.26970
Eller, S., Schuberth, R., Gundel, G., Seifert, J., and Unverzagt, C. (2007). Synthesis of pentaantennary N-glycans with bisecting GlcNAc and core fucose. Angew. Chem. Int. Ed. Engl. 46, 4173–4175. doi: 10.1002/anie.200604788
Gomes, J., Gomes-Alves, P., Carvalho, S. B., Peixoto, C., Alves, P. M., Altevogt, P., et al. (2015). Extracellular vesicles from ovarian carcinoma cells display specific glycosignatures. Biomolecules 5, 1741–1761. doi: 10.3390/biom5031741
Gu, J., Isaji, T., Xu, Q., Kariya, Y., Gu, W., Fukuda, T., et al. (2012). Potential roles of N-glycosylation in cell adhesion. Glycoconjugate J. 29, 599–607. doi: 10.1007/s10719-012-9386-1
Gu, J., Sato, Y., Kariya, Y., Isaji, T., Taniguchi, N., and Fukuda, T. (2009). A mutual regulation between cell-cell adhesion and N-glycosylation: implication of the bisecting GlcNAc for biological functions. J. Proteome Res. 8:5. doi: 10.1021/pr800674g
Gudelj, I., Lauc, G., and Pezer, M. (2018). Immunoglobulin G glycosylation in aging and diseases. Cell Immunol. 333, 65–79. doi: 10.1016/j.cellimm.2018.07.009
Halim, A., Brinkmalm, G., Ruetschi, U., Westman-Brinkmalm, A., Portelius, E., Zetterberg, H., et al. (2011). Site-specific characterization of threonine, serine, and tyrosine glycosylations of amyloid precursor protein/amyloid beta-peptides in human cerebrospinal fluid. Proc. Natl. Acad. Sci. U.S.A. 108, 11848–11853. doi: 10.1073/pnas.1102664108
Harpaz, N., and Schachter, H. (1980). Control of glycoprotein synthesis. Processing of asparagine-linked oligosaccharides by one or more rat liver Golgi α-D-mannosidases dependent on the prior action of UDP-N-acetylglucosamine: α-D-mannoside β 2-N-acetylglucosaminyltransferase I. J. Biol. Chem. 255, 4894–4902.
Hodoniczky, J., Zheng, Y. Z., and James, D. C. (2005). Control of recombinant monoclonal antibody effector functions by Fc N-glycan remodeling in vitro. Biotechnol. Prog. 21, 1644–1652. doi: 10.1021/bp050228w
Imperiali, B., and Hendrickson, T. L. (1995). Asparagine-linked glycosylation: specificity and function of oligosaccharyl transferase. Bioorg. Med. Chem. 3, 1565–1578. doi: 10.1016/0968-0896(95)00142-5
Kariya, Y., Kato, R., Itoh, S., Fukuda, T., Shibukawa, Y., Sanzen, N., et al. (2008). N-Glycosylation of laminin-332 regulates its biological functions. A novel function of the bisecting GlcNAc. J. Biol. Chem. 283, 33036–33045. doi: 10.1074/jbc.M804526200
Kelly, R. W., and Critchley, H. O. (1997). Immunomodulation by human seminal plasma: a benefit for spermatozoon and pathogen? Hum. Reprod. 12, 2200–2207. doi: 10.1093/oxfordjournals.humrep.a019559
King, A., Balendran, N., Wooding, P., Carter, N. P., and Loke, Y. W. (1991). CD3- leukocytes present in the human uterus during early placentation: phenotypic and morphologic characterization of the CD56++ population. Dev. Immunol. 1, 169–190. doi: 10.1155/1991/83493
Kitada, T., Miyoshi, E., Noda, K., Higashiyama, S., Ihara, H., Matsuura, N., et al. (2001). The addition of bisecting N-acetylglucosamine residues to E-cadherin down-regulates the tyrosine phosphorylation of beta-catenin. J. Biol. Chem. 276, 475–480. doi: 10.1074/jbc.M006689200
Kiyoshi, M., Tsumoto, K., Ishii-Watabe, A., and Caaveiro, J. M. M. (2017). Glycosylation of IgG-Fc: a molecular perspective. Int. Immunol. 29, 311–317. doi: 10.1093/intimm/dxx038
Kizuka, Y., Kitazume, S., Fujinawa, R., Saito, T., Iwata, N., Saido, T. C., et al. (2015). An aberrant sugar modification of BACE1 blocks its lysosomal targeting in Alzheimer's disease. EMBO Mol. Med. 7, 175–189. doi: 10.15252/emmm.201404438
Kizuka, Y., Nakano, M., Kitazume, S., Saito, T., Saido, T. C., and Taniguchi, N. (2016a). Bisecting GlcNAc modification stabilizes BACE1 protein under oxidative stress conditions. Biochem. J. 473, 21–30. doi: 10.1042/BJ20150607
Kizuka, Y., Nakano, M., Miura, Y., and Taniguchi, N. (2016b). Epigenetic regulation of neural N-glycomics. Proteomics 16, 2854–2863. doi: 10.1002/pmic.201600053
Kizuka, Y., and Taniguchi, N. (2016). Enzymes for N-glycan branching and their genetic and nongenetic regulation in cancer. Biomolecules 6:25. doi: 10.3390/biom6020025
Kizuka, Y., and Taniguchi, N. (2018). Neural functions of bisecting GlcNAc. Glycoconj. J. 35, 345–351. doi: 10.1007/s10719-018-9829-4
Kochetkov, N. K., Chizhov, O. S., and Molodtsov, N. V. (1968). Mass spectrometry of oligosaccharides. Tetrahedron 24. doi: 10.1016/0040-4020(68)88156-6
Le, N. P., Bowden, T. A., Struwe, W. B., and Crispin, M. (2016). Immune recruitment or suppression by glycan engineering of endogenous and therapeutic antibodies. BBA-Gen. Subjects 1860, 1655–1668. doi: 10.1016/j.bbagen.2016.04.016
Li, W., Takahashi, M., Shibukawa, Y., Yokoe, S., Gu, J., Miyoshi, E., et al. (2007). Introduction of bisecting GlcNAc in N-glycans of adenylyl cyclase III enhances its activity. Glycobiology 17:8. doi: 10.1093/glycob/cwm022
Li, X., Wang, X., Tan, Z., Chen, S., and Guan, F. (2016). Role of glycans in cancer cells undergoing epithelial-mesenchymal transition. Front. Oncol. 6:33. doi: 10.3389/fonc.2016.00033
Link-Lenczowski, P., Bubka, M., Balog, C. I. A., Koeleman, C. A. M., Butters, T. D., Wuhrer, M., et al. (2018). The glycomic effect of N-acetylglucosaminyltransferase III overexpression in metastatic melanoma cells. GnT-III modifies highly branched N-glycans. Glycoconj. J. 35:217–231. doi: 10.1007/s10719-018-9814-y
Lis-Kuberka, J., Krolak-Olejnik, B., Berghausen-Mazur, M., and Orczyk-Pawilowicz, M. (2019). Lectin-based method for deciphering human milk IgG sialylation. Molecules 24:3797. doi: 10.3390/molecules24203797
Liu, T. H., Liu, D. H., Mo, C. J., Sun, L., Liu, X. X., Li, W., et al. (2016). Glycosylation patterns and PHA-E-associated glycoprotein profiling associated with early hepatic encephalopathy in Chinese hepatocellular carcinoma patients. Am. J. Transl. Res. 8, 4250–4264.
Lu, G., and Holland, L. A. (2019). Profiling the N-Glycan composition of IgG with lectins and capillary nanogel electrophoresis. Anal. Chem. 91, 1375–1383. doi: 10.1021/acs.analchem.8b03725
Luber, T., Niemietz, M., Karagiannis, T., Monnich, M., Ott, D., Perkams, L., et al. (2018). A single route to mammalian N-glycans substituted with core fucose and bisecting GlcNAc. Angew. Chem. Int. Ed. Engl. 57, 14543–14549. doi: 10.1002/anie.201807742
Manabe, Y., Shomura, H., Minamoto, N., Nagasaki, M., Takakura, Y., Tanaka, K., et al. (2018). Convergent synthesis of a bisecting N-acetylglucosamine (GlcNAc)-containing N-glycan. Chem. Asian J. 13, 1544–1551. doi: 10.1002/asia.201800367
Maverakis, E., Kim, K., Shimoda, M., Gershwin, M. E., Patel, F., Wilken, R., et al. (2015). Glycans in the immune system and the altered glycan theory of autoimmunity: a critical review. J. Autoimmun. 57, 1–13. doi: 10.1016/j.jaut.2014.12.002
Miwa, H. E., Song, Y., Alvarez, R., Cummings, R. D., and Stanley, P. (2012). The bisecting GlcNAc in cell growth control and tumor progression. Glycoconj. J. 29, 609–618. doi: 10.1007/s10719-012-9373-6
Morris, H. R., Thompson, M. R., Osuga, D. T., Ahmed, A. I., Chan, S. M., Vandenheede, J. R., et al. (1978). Antifreeze glycoproteins from the blood of an antarctic fish. The structure of the proline-containing glycopeptides. J. Biol. Chem. 253, 5155–5162.
Nagae, M., Kanagawa, M., Morita-Matsumoto, K., Hanashima, S., Kizuka, Y., Taniguchi, N., et al. (2016). Atomic visualization of a flipped-back conformation of bisected glycans bound to specific lectins. Sci. Rep. 6:22973. doi: 10.1038/srep22973
Nagae, M., Yamaguchi, Y., Taniguchi, N., and Kizuka, Y. (2020). 3D structure and function of glycosyltransferases involved in N-glycan maturation. Int. J. Mol. Sci. 21:437. doi: 10.3390/ijms21020437
Nagae, M., Yamanaka, K., Hanashima, S., Ikeda, A., Morita-Matsumoto, K., Satoh, T., et al. (2013). Recognition of bisecting N-acetylglucosamine: structural basis for asymmetric interaction with the mouse lectin dendritic cell inhibitory receptor 2. J. Biol. Chem. 288, 33598–33610. doi: 10.1074/jbc.M113.513572
Nakano, M., Mishra, S. K., Tokoro, Y., Sato, K., Nakajima, K., Yamaguchi, Y., et al. (2019). Bisecting GlcNAc is a general suppressor of terminal modification of N-glycan. Mol. Cell. Proteomics 18, 2044–2057. doi: 10.1074/mcp.RA119.001534
Narasimhan, S. (1982). Control of glycoprotein synthesis. UDP-GlcNAc:glycopeptide β4-N-acetylglucosaminyltransferase III, an enzyme in hen oviduct which adds GlcNAc in β1-4 linkage to the β-linked mannose of the trimannosyl core of N-glycosyl oligosaccharides. J. Biol. Chem. 257, 10235–10242.
Nishikawa, A., Ihara, Y., Hatakeyama, M., Kangawa, K., and Taniguchi, N. (1992). Purification, cDNA cloning, and expression of UDP-N-acetylglucosamine: β-D-mannoside β-1,4N-acetylglucosaminyltransferase III from rat kidney. J. Biol. Chem. 267, 18199–18204.
North, S. J., Jang-Lee, J., Harrison, R., Canis, K., Ismail, M. N., Trollope, A., et al. (2010). Mass spectrometric analysis of mutant mice. Methods Enzymol. 478, 27–77. doi: 10.1016/S0076-6879(10)78002-2
Padmanaban, V., Krol, I., Suhail, Y., Szczerba, B. M., Aceto, N., Bader, J. S., et al. (2019). E-cadherin is required for metastasis in multiple models of breast cancer. Nature 573, 439–444. doi: 10.1038/s41586-019-1526-3
Pang, P. C., Haslam, S. M., Dell, A., and Clark, G. F. (2016). The human fetoembryonic defense system hypothesis: twenty years on. Mol. Aspects Med. 51, 71–88. doi: 10.1016/j.mam.2016.06.002
Pang, P. C., Tissot, B., Drobnis, E. Z., Sutovsky, P., Morris, H. R., Clark, G. F., et al. (2007). Expression of bisecting type and Lewisx/Lewisy terminated N-glycans on human sperm. J. Biol. Chem. 282, 36593–36602. doi: 10.1074/jbc.M705134200
Patankar, M. S., Ozgur, K., Oehninger, S., Dell, A., Morris, H., Seppala, M., et al. (1997). Expression of glycans linked to natural killer cell inhibition on the human zona pellucida. Mol. Hum. Reprod. 3, 501–505. doi: 10.1093/molehr/3.6.501
Pinho, S. S., Reis, C. A., Paredes, J., Magalhães, A. M., Ferreira, A. C., Figueiredo, J., et al. (2009). The role of N-acetylglucosaminyltransferase III and V in the post-transcriptional modifications of E-cadherin. Hum. Mol. Genet. 18:10. doi: 10.1093/hmg/ddp194
Qasba, P. K., Ramakrishnan, B., and Boeggeman, E. (2008). Structure and Function of beta-1,4-Galactosyltransferase. Curr. Drug Targets 9:18. doi: 10.2174/138945008783954943
Reily, C., Stewart, T. J., Renfrow, M. B., and Novak, J. (2019). Glycosylation in health and disease. Nat. Rev. Nephrol. 15, 346–366. doi: 10.1038/s41581-019-0129-4
Schachter, H. (1991). The ‘yellow brick road’ to branched complex N-glycans. Glycobiology 1, 453–461. doi: 10.1093/glycob/1.5.453
Schachter, H. (2014). Complex N-glycans: the story of the “yellow brick road”. Glycoconj. J. 31, 1–5. doi: 10.1007/s10719-013-9507-5
Schedin-Weiss, S., Gaunitz, S., Sui, P., Chen, Q., Haslam, S. M., Blennow, K., et al. (2019). Glycan biomarkers for Alzheimer disease correlate with T-tau and P-tau in cerebrospinal fluid in subjective cognitive impairment. FEBS J. doi: 10.1111/febs.15197. [Epub ahead of print].
Schedin-Weiss, S., Winblad, B., and Tjernberg, L. O. (2014). The role of protein glycosylation in Alzheimer disease. FEBS J. 281, 46–62. doi: 10.1111/febs.12590
Scheltens, P., Blennow, K., Breteler, M. M., de Strooper, B., Frisoni, G. B., Salloway, S., et al. (2016). Alzheimer's disease. Lancet 388:13. doi: 10.1016/S0140-6736(15)01124-1
Schwientek, T., Narimatsu, H., and Ernst, J. F. (1996). Golgi localization and in vivo activity of a mammalian glycosyltransferase (human beta1,4-galactosyltransferase) in yeast. J. Biol. Chem. 271, 3398–3405. doi: 10.1074/jbc.271.7.3398
Selman, M. H., Niks, E. H., Titulaer, M. J., Verschuuren, J. J., Wuhrer, M., and Deelder, A. M. (2011). IgG fc N-glycosylation changes in Lambert-Eaton myasthenic syndrome and myasthenia gravis. J. Proteome Res. 10, 143–152. doi: 10.1021/pr1004373
Shade, K. T., Conroy, M. E., and Anthony, R. M. (2019). IgE glycosylation in health and disease. Curr. Top. Microbiol. Immunol. 423, 77–93. doi: 10.1007/82_2019_151
Shang, C., Chen, Q., Dell, A., Haslam, S. M., De Vos, W. H., and Van Damme, E. J. (2015). The cytotoxicity of elderberry ribosome-inactivating proteins is not solely determined by their protein translation inhibition activity. PLoS ONE 10:e0132389. doi: 10.1371/journal.pone.0132389
Shathili, A. M., Bandala-Sanchez, E., John, A., Goddard-Borger, E. D., Thaysen-Andersen, M., Everest-Dass, A. V., et al. (2019). Specific sialoforms required for the immune suppressive activity of human soluble CD52. Front. Immunol. 10:1967. doi: 10.3389/fimmu.2019.01967
Shields, R. L., Lai, J., Keck, R., O'Connell, L. Y., Hong, K., Meng, Y. G., et al. (2002). Lack of fucose on human IgG1 N-linked oligosaccharide improves binding to human Fcgamma RIII and antibody-dependent cellular toxicity. J. Biol. Chem. 277, 26733–26740. doi: 10.1074/jbc.M202069200
Shigeta, M., Shibukawa, Y., Ihara, H., Miyoshi, E., Taniguchi, N., and Gu, J. (2006). β1,4-N-Acetylglucosaminyltransferase III potentiates β1 integrin-mediated neuritogenesis induced by serum deprivation in Neuro2a cells. Glycobiology 16, 564–571. doi: 10.1093/glycob/cwj100
Shimizu, H., Ochiai, K., Ikenaka, K., Mikoshiba, K., and Hase, S. (1993). Structures of N-linked sugar chains expressed mainly in mouse brain. J. Biochem. 114:5. doi: 10.1093/oxfordjournals.jbchem.a124177
Szczykutowicz, J., Kaluza, A., Kazmierowska-Niemczuk, M., and Ferens-Sieczkowska, M. (2019). The Potential Role of Seminal Plasma in the Fertilization Outcomes. Biomed Res. Int. 2019:5397804. doi: 10.1155/2019/5397804
Takahashi, M., Kuroki, Y., Ohtsubo, K., and Taniguchi, N. (2009). Core fucose and bisecting GlcNAc, the direct modifiers of the N-glycan core: their functions and target proteins. Carbohydr Res 344, 1387–1390. doi: 10.1016/j.carres.2009.04.031
Takamatsu, S., Katsumata, T., Inoue, N., Watanabe, T., Fujibayashi, Y., and Takeuchi, M. (2004). Abnormal biantennary sugar chains are expressed in human chorionic gonadotropin produced in the choriocarcinoma cell line, JEG-3. Glycoconj. J. 20, 473–481. doi: 10.1023/B:GLYC.0000038293.37376.9f
Takegawa, Y., Deguchi, K., Nakagawa, H., and Nishimura, S. (2005). Structural analysis of an N-glycan with “β1-4 bisecting branch” from human serum IgG by negative-ion MSn spectral matching and exoglycosidase digestion. Anal. Chem. 77, 6062–6068. doi: 10.1021/ac050843e
Tan, Z., Wang, C., Li, X., and Guan, F. (2018). Bisecting N-acetylglucosamine structures inhibit hypoxia-induced epithelial-mesenchymal transition in breast cancer cells. Front. Physiol. 9:210. doi: 10.3389/fphys.2018.00210
Taniguchi, N., and Korekane, H. (2011). Branched N-glycans and their implications for cell adhesion, signaling and clinical applications for cancer biomarkers and in therapeutics. BMB Rep. 44, 772–781. doi: 10.5483/BMBRep.2011.44.12.772
Taniguchi, N., Miyoshi, E., Ko, J. H., Ikeda, Y., and Ihara, Y. (1999). Implication of N-acetylglucosaminyltransferases III and V in cancer: gene regulation and signaling mechanism. BBA-Mol. Basis Dis. 1455, 287–300. doi: 10.1016/S0925-4439(99)00066-6
Taylor, M. E., and Drickamer, K. (2011). Introduction to glycobiology. Oxford; New York, NY: Oxford University Press.
Varki, A. (2009). Essentials of Glycobiology. Cold Spring Harbor, N.Y.: Cold Spring Harbor Laboratory Press.
Wang, G., Zhang, W., Lu, Z., Wang, P., Zhang, X., and Li, Y. (2009). Convenient synthesis of an N-glycan octasaccharide of the bisecting type. J. Org. Chem. 74, 2508–2515. doi: 10.1021/jo900016j
Wei, B., Han, G., Tang, J., Sandoval, W., and Zhang, Y. T. (2019). Native hydrophobic interaction chromatography hyphenated to mass spectrometry for characterization of monoclonal antibody minor variants. Anal. Chem. 91, 15360–15364. doi: 10.1021/acs.analchem.9b04467
Winblad, B., Amouyel, P., Andrieu, S., Ballard, C., Brayne, C., Brodaty, H., et al. (2016). Defeating Alzheimer's disease and other dementias: a priority for European science and society. Lancet Neurol. 15, 455–532. doi: 10.1016/S1474-4422(16)00062-4
Wu, D., Li, J., Struwe, W. B., and Robinson, C. V. (2019). Probing N-glycoprotein microheterogeneity by lectin affinity purification-mass spectrometry analysis. Chem. Sci. 10, 5146–5155. doi: 10.1039/C9SC00360F
Yamadera, M., Shinto, E., Tsuda, H., Kajiwara, Y., Naito, Y., Hase, K., et al. (2018). Sialyl Lewis(x) expression at the invasive front as a predictive marker of liver recurrence in stage II colorectal cancer. Oncol. Lett. 15, 221–228. doi: 10.3892/ol.2017.7340
Yamashita, K., Tachibana, Y., and Kobata, A. (1978). The structures of the galactose-containing sugar chains of ovalbumin. J. Biol. Chem. 253, 3862–3869.
Yang, W., Ramadan, S., Orwenyo, J., Kakeshpour, T., Diaz, T., Eken, Y., et al. (2018). Chemoenzymatic synthesis of glycopeptides bearing rare N-glycan sequences with or without bisecting GlcNAc. Chem. Sci. 9, 8194–8206. doi: 10.1039/C8SC02457J
Yoshimura, M., Ihara, Y., Nishiura, T., Okajima, Y., Ogawa, M., Yoshida, H., et al. (1998). Bisecting GlcNAc structure is implicated in suppression of stroma-dependent haemopoiesis in transgenic mice expressing N-acetylglucosaminyltransferase III. Biochem. J. 331 (Pt 3), 733–742. doi: 10.1042/bj3310733
Yoshimura, M., Ihara, Y., Ohnishi, A., Ijuhin, N., Nishiura, T., Kanakura, Y., et al. (1996). Bisecting N-acetylglucosamine on K562 cells suppresses natural killer cytotoxicity and promotes spleen colonization. Cancer Res. 56, 412–418.
Yoshimura, M., Ihara, Y., and Taniguchi, N. (1995a). Changes of β-1,4-N-acetylglucosaminyltransferase III (GnT-III) in patients with leukaemia. Glycoconj. J. 12, 234–240. doi: 10.1007/BF00731325
Yoshimura, M., Nishikawa, A., Ihara, Y., Nishiura, T., Nakao, H., Kanayama, Y., et al. (1995b). High expression of UDP-N-acetylglucosamine: β-D mannoside β-1,4-N-acetylglucosaminyltransferase III (GnT-III) in chronic myelogenous leukemia in blast crisis. Int. J. Cancer 60, 443–449. doi: 10.1002/ijc.2910600404
Yoshimura, M., Nishikawa, A., Ihara, Y., Taniguchi, S., and Taniguchi, N. (1995c). Suppression of lung metastasis of B16 mouse melanoma by N-acetylglucosaminyltransferase III gene transfection. Proc. Natl. Acad. Sci. U. S. A. 92, 8754–8758. doi: 10.1073/pnas.92.19.8754
Zhang, M., Wang, M., Gao, R., Liu, X., Chen, X., Geng, Y., et al. (2015). Altered β1,6-GlcNAc and bisecting GlcNAc-branched N-glycan on integrin β1 are associated with early spontaneous miscarriage in humans. Hum. Reprod. 30, 2064–2075. doi: 10.1093/humrep/dev153
Zou, G., Ochiai, H., Huang, W., Yang, Q., Li, C., and Wang, L. X. (2011). Chemoenzymatic synthesis and Fcgamma receptor binding of homogeneous glycoforms of antibody Fc domain. Presence of a bisecting sugar moiety enhances the affinity of Fc to FcgammaIIIa receptor. J. Am. Chem. Soc. 133, 18975–18991. doi: 10.1021/ja208390n
Keywords: bisecting GlcNAc, mass spectrometry, glycosylation, N-glycan, GlcNAc-T III
Citation: Chen Q, Tan Z, Guan F and Ren Y (2020) The Essential Functions and Detection of Bisecting GlcNAc in Cell Biology. Front. Chem. 8:511. doi: 10.3389/fchem.2020.00511
Received: 12 March 2020; Accepted: 18 May 2020;
Published: 03 July 2020.
Edited by:
Zhongping Tan, Chinese Academy of Medical Sciences and Peking Union Medical College, ChinaReviewed by:
Wenjie Peng, Shanghai Jiao Tong University, ChinaHongzhi Cao, Shandong University, China
Copyright © 2020 Chen, Tan, Guan and Ren. This is an open-access article distributed under the terms of the Creative Commons Attribution License (CC BY). The use, distribution or reproduction in other forums is permitted, provided the original author(s) and the copyright owner(s) are credited and that the original publication in this journal is cited, in accordance with accepted academic practice. No use, distribution or reproduction is permitted which does not comply with these terms.
*Correspondence: Feng Guan, Z3VhbmZlbmdAbnd1LmVkdS5jbg==; Yan Ren, cmVueUBnZW5vbWljcy5jbg==
†These authors have contributed equally to this work