- Department of Chemistry, University of Southern California, Los Angeles, CA, United States
The structural, photophysical and electrochemical properties of three luminescent 2-coordinate coinage metal (i.e., M = Cu, Ag, Au) complexes bearing a sterically bulky benzimidazolyl carbene, 1,3-bis(2,6-diisopropylphenyl)-1-H-benzo[d]imidazol-2-ylidene (BZI), and carbazolide (Cz) as the anionic ligand were investigated. All the complexes emit in the deep blue region (~430 nm) with relatively narrow spectra (full width at half maximum = 44 nm, 2,300 cm−1) characterized by vibronic fine structure in nonpolar media (methylcyclohexane at room temperature), and with high photoluminescence quantum yields (ΦPL > 80%) and radiative rate constants (kr ~ 7.8 × 105 s−1). The luminescence is solvatochromic, undergoing a red-shift in a polar solvent (CH2Cl2) at room temperature that are accompanied by a decrease in quantum yields (ΦPL < 23%) and radiative rate constants (kr < 4.0 × 104 s−1), whereas the non-radiative rate constants remain nearly constant (knr ~ 1.0 × 105 s−1). The radiative rate is controlled via thermally assisted delayed fluorescence (TADF) and temperature-dependent luminescence studies of the gold complex (AuBZI) in methylcyclohexane solution reveal an energy difference between the lowest singlet and triplet excited states of 920 cm−1. An organic light-emitting diode (OLED) fabricated using AuBZI as a luminescent dopant has an external quantum efficiency of 12% and narrow, deep-blue emission (CIE = 0.16, 0.06).
Introduction
Highly luminescent, neutral two-coordinate, linear d10 metal complexes of coinage metals i.e., Cu(I), Ag(I), Au(I) have recently been reported (Di et al., 2017; Romanov et al., 2018, 2019; Hamze et al., 2019a,b; Shi et al., 2019). These complexes have redox active carbene (acceptor) and amide (donor) ligands connected by a metal in a linear fashion i.e., (carbene)M(I)(amide). Their photoluminescence efficiencies are close to 100% in solution and in thin films, with phosphorescent lifetimes in the range of 1–3 μs. These (carbene)M(I)(amide) complexes emit from an amide-N (donor) to carbene-C (acceptor) intramolecular charge transfer (ICT) state, a transition also referred to as ligand-to-ligand charge transfer (LLCT). Because of their excellent photophysical properties, these complexes are potential candidates for application in photocatalysis (Kalyanasundaram, 1982), chemo- and biosensing (Keefe et al., 2000; Lo et al., 2010), dye-sensitized solar cells (Grätzel, 2005) and organic electronics (Lamansky et al., 2001). In particular, their fast radiative lifetimes make them promising candidates as dopants in organic light emitting diodes (OLEDs) (Di et al., 2017; Romanov et al., 2018, 2019; Hamze et al., 2019a,b; Shi et al., 2019). Unlike phosphors like iridium and platinum complexes, which rely on strong spin-orbit coupling (SOC) to induce what is principally triplet metal ligand charge transfer (3MLCT) emission (Yersin et al., 2011), the two-coordinate coinage metal complexes emit via E-type fluorescence or thermally activated delayed fluorescence (TADF). Their fast radiative lifetimes are due to two factors; the small energy separation between their lowest singlet and triplet excited states (ΔEST) and spin orbit coupling via the metal ion. Together these two parameters lead to rapid endothermic intersystem crossing from the long-lived triplet to the faster radiating singlet state. Recent work has shown that ΔEST in these complexes is dependent on the identity of the metal atom. The copper and gold complexes have similar values for ΔEST, whereas the ΔEST for the silver complexes is smaller (Romanov et al., 2018; Hamze et al., 2019b). The small ΔEST in the silver analogs leads to extremely fast radiative rate constants (kr > 106 s−1), faster than the Ir and Pt phosphorescent emitters. The emission energy of complexes, although nearly independent of the metal, can be altered using different carbenes with varying electrophilicity or amides with different nucleophilicity, allowing the luminescence color to be varied from deep blue to deep red (Hamze et al., 2019a; Shi et al., 2019). Developing alternative blue dopants is crucial in tackling the long-standing problem of stability in blue OLEDs. Complexes based on the CAAC ligand (MCAAC, Figure 1) are reported to have efficient blue photoluminescence and give good efficiencies as dopants in OLEDs (Hamze et al., 2019a). Unfortunately, the emission spectra of these complexes are broad, which is not ideal for display applications. Additionally, the ability to vary the physical and electronic properties of CAAC ligands is limited and therefore inconvenient for modifying the characteristics needed for OLEDs. Complexes based on the MAC ligand (MMAC) can be used to fabricate high efficiency OLEDs; however, emission in these derivatives is bathochromically shifted to green owing to the electrophilic MAC ligand (Hamze et al., 2019b). Substitution of the carbazole ligand with cyano groups was therefore used to stabilize the HOMO and blue-shift the emission (Shi et al., 2019). Alternatively, carbenes based on benzoimidazoles (BZI), originally used in luminescent two-coordinate Au complexes (Wang et al., 1999), lead to metal complexes with LUMO energies similar to CAAC (Krylova et al., 2014; Hamze et al., 2017, 2020), suggesting that replacing CAAC with benzoimidazolyl-carbene ligands should give similar photophysical and electrochemical properties as MCAAC. Herein, we examine monovalent, linear, 2-coordinate coinage metal (i.e., M = Cu, Ag, Au) complexes (MBZI) bearing a sterically bulky benzimidazolyl carbene, 1,3-bis(2,6-diisopropylphenyl)-1-H-benzo[d]imidazol-2-ylidene (BZI), and carbazolide (Cz) as the anionic ligand. We have investigated the structural and photophysical properties of the MBZI derivatives to elucidate the role of the carbene and the metal ion in the excited-state properties. The MBZI complexes have structures, redox potentials and photoluminescent efficiencies (ΦPL = 0.8–1.0) similar to the MCAAC analogs, but different excited-state dynamics. Analysis of the luminescence at low temperature reveals that the triplet carbazole (3Cz) state and the singlet/triplet intramolecular charge transfer (1/3ICT) manifold of the MBZI complexes are near degenerate, resulting in photophysical properties that are distinct from the MCAAC complexes. The AuBZI complex has also been successfully employed a dopant to fabricate efficient blue OLEDs.
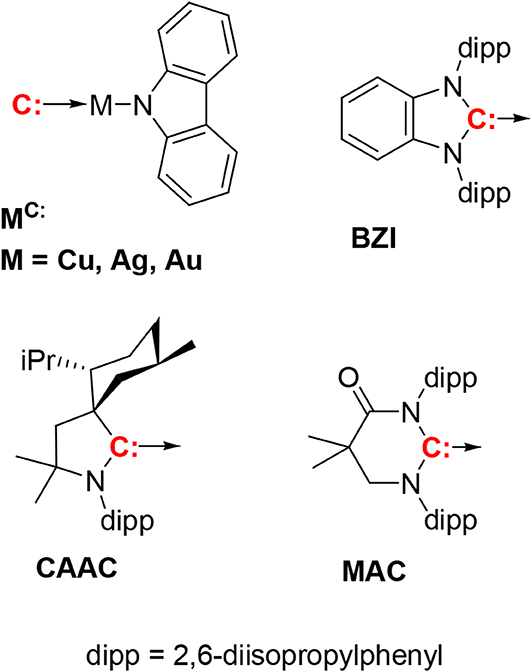
Figure 1. The compounds discussed in this paper have the general formula (carbene)M(N-carbazolyl), with three carbenes, i.e., CAAC, MAC, and BZI. The BZI compounds are new and the CAAC and MAC derivatives have been reported previously (Hamze et al., 2019a,b; Shi et al., 2019). Compounds are abbreviated using the symbol for the metal ion and superscripted carbene, e.g., CuCAAC or AuBZI.
Results and Discussion
Synthesis
The synthesis of MBZI complexes is depicted in Figure 2. Cyclization of the dianiline derivative (a) to the benzoimidazolium salt is reported to be extremely challenging, due to steric bulk imposed by the isopropyl groups, with a reaction yield of only 16% (Grieco et al., 2015). We modified the literature procedure, using excess triethyl orthoformate [HC(OEt)3] and distilling the excess off during the reaction, which increases the yield of the reaction to 76%. Similar to MCAAC and MMAC complexes, the synthesis of MBZI complexes starts with addition of Ag2O to the benzoimidazolium salt or CuCl to BZI carbene generated in situ with base to form the respective benzoimidazole silver(I) or copper(I) chloride complexes. The isolated BZIAgCl is transmetallated with (Me2S)AuCl to form the BZIAuCl complex. Reaction of the chloride complexes with carbazole in the presence of NaOtBu forms the MBZI complexes in good yields (70–85%).
X-Ray Analysis
The structures were determined for CuBZI, AgBZI, and AuBZI by single crystal X-ray diffraction (details can be found in the Supplementary Information and the datasets generated for this study can be found in the Cambridge Crystallographic Data Center, https://www.ccdc.cam.ac.uk/structures/, under the identifiers CuBZI: 1984269, AgBZI: 1984268 and AuBZI: 1984267). CuBZI and AuBZI show only a single conformer, with bond distances and interligand torsion angles similar to our previously reported linear coinage metal complexes (summarized in the Supporting Information) (Di et al., 2017; Romanov et al., 2018, 2019; Hamze et al., 2019a,b; Shi et al., 2019). In contrast, the unit cell for the AgBZI complex contains two conformers (Figure 3). The first, like its copper and gold analogs, has a coplanar conformation of its carbene and amide ligands (dihedral angle = 0°), whereas the second displays an orthogonal conformation (dihedral angle = 95°). The Ccarbene···NCz distances of MBZI fall in the order Cu (~3.73 Å) < Au (~4.00 Å) < Ag (~4.11 Å). The C–M–N bond angles are all close to 180° (range = 174–180°).
Electrochemistry
The electrochemical properties of the complexes were determined using cyclic voltammetry (CV) and differential pulse voltammetry (DPV). The copper and the silver complexes show irreversible reduction, whereas the gold analog shows a quasi-reversible reduction (see section Supporting Information). The reduction potentials for the MBZI series are identical (Ered = −2.84 ± 0.02 V) and greater (more negative) than those of MCAAC (Ered = −2.78 ± 0.06 V) and MMAC (Ered = −2.45 ± 0.06 V) complexes. The reduction potentials of MBZI relative to their MCAAC and MMAC analogs indicates that the electrophilicity of the coordinated BZI carbene is lower than the CAAC and MAC ligands in similar complexes. All the complexes undergo irreversible oxidation (Table 1) and, unlike the MCAAC and MMAC complexes where the oxidation potential is the same across the series, the potential of the MBZI complexes increases from Cu (Eox = 0.11 ± 0.06 V) to Au (Eox = 0.32 ± 0.06 V), suggesting participation of the metal in the oxidation process. Thus, values for the redox gap (ΔEredox) are greater for the silver and gold complexes than the copper analog.
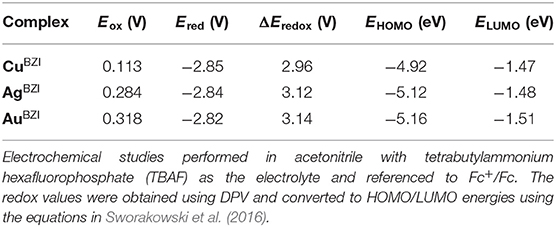
Table 1. Redox potentials of complexes MBZI and the associated experimental frontier orbital energies.
Computational Analysis
The structure calculated using density function theory (DFT) for the ground state of AuBZI is shown in Figure 4. The HOMO density is localized largely on the carbazolide (Cz) ligand, whereas the LUMO is primarily confined to the carbene ligand, with smaller contributions from the metal d-orbitals to both MOs. Time dependent DFT (TD-DFT) calculations find that 3Cz is the lowest-energy state and lies <0.09 eV below the manifold of the 1/3ICT states (Table S8). Additionally, the oscillator strength calculated for the silver complex is weaker than that of its Cu and Au analogs (Table S8), consistent with the lower molar absorptivity observed for the ICT transition in the absorption spectra of the silver complex (see below). Large molecular dipole moments calculated for the ground state are directed along the metal-ligand bond axis toward the carbazolide ligand (μcalc ≈ −12.5 debye), whereas the moments for the excited 1ICT state is comparable in magnitude but directed toward the BZI ligand (μcalc ≈ 13.5 debye).
Photophysical Characterization
Absorption spectra of the MBZI complexes in polar (2-methyltetrahydrofuran, 2-MeTHF) and nonpolar (methylcyclohexane, MeCy) solvents are shown in Figure 5. Absorption bands between 300 and 375 nm of MBZI are assigned to π-π* transitions localized on the carbazolyl ligand (Hamze et al., 2019a,b; Shi et al., 2019). The band at lower energy (>375 nm) is assigned to an intramolecular ligand-to-ligand charge transfer (ICT/LLCT) transition from Cz to BZI. The energy of the ICT band of MBZI is higher than in the MCAAC and MMAC complexes, consistent with the order of reduction potentials in these complexes. The ICT band extends to 410 nm in MeCy and has two features separated by 1100 cm−1 indicative of vibronic coupling. Similar to MCAAC and MMAC complexes, the ICT band of the MBZI complexes displays negative solvatochromism and merges into the higher lying ligand π-π* transitions in polar solvents. These shifts with solvent polarity are due to the large change in the molecular dipole moments between the ground and excited ICT states. The molar absorptivities of the MBZI complexes decrease in the order Au > Cu > Ag in all media. The same trend was observed in the MCAAC and MMAC analogs and attributed to a decrease in the overlap integrals between orbitals on the donor Cz and acceptor ligands mediated by the metal center (Hamze et al., 2019b).
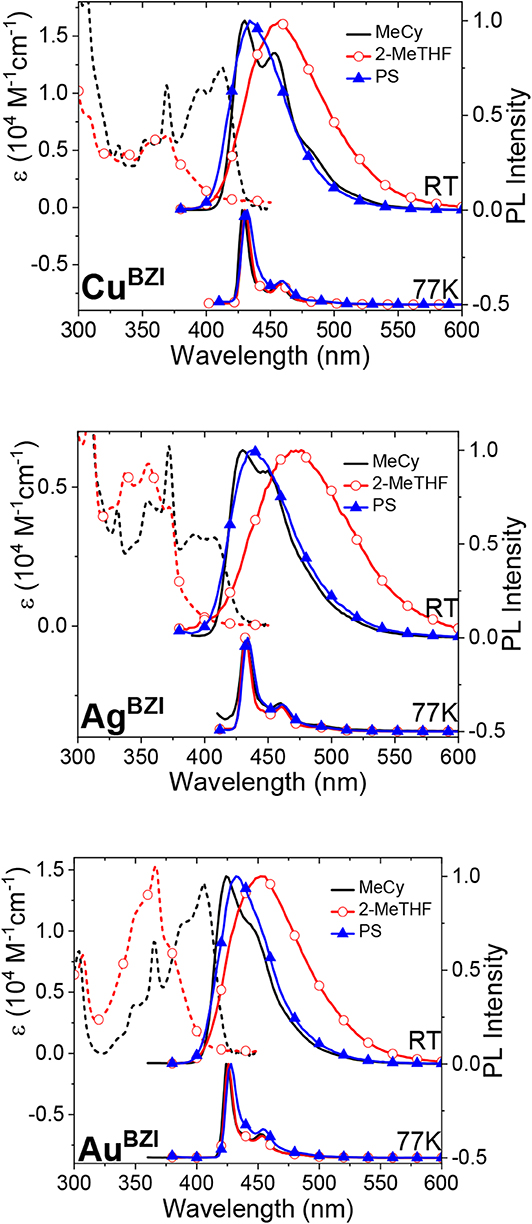
Figure 5. Absorption (dashed lines) and photoluminescence (solid line) spectra of MBZI complexes in MeCy, 2-MeTHF and polystyrene at room temperature (RT) and 77 K.
Emission spectra of the MBZI compounds in MeCy, 2-MeTHF solution and polystyrene (PS) films at room temperature and 77 K are shown in Figure 5 and tabulated in Table 2. The spectra from the MBZI complexes are blue-shifted relative to their MCAAC and MMAC counterparts, yet display similar solvatochromic behavior, undergoing red shifts in polar solvents. Spectra recorded in MeCy are relatively narrow (FWHM = 44 nm, 2,300 cm−1) and show underlying vibronic features. The photoluminescence quantum yields (ΦPL) of MBZI complexes are close to unity in MeCy and PS films but decrease with increasing solvent polarity (Table 2). Furthermore, increasing solvent polarity is correlated with increased spectral width and loss of the vibronic features, suggesting structural distortion in the excited states. To explain the blue-shift in absorption spectra and red-shift in emission spectra observed in these complexes with increasing solvent polarity, a diagram representing the potential energy surfaces for the ground state (S0) and excited states (3Cz and 1,3ICT) as a function of nuclear coordinates in MeCy and CH2Cl2 is proposed (Figure 6). The vibronically structured absorption and emission spectra in nonpolar solvents (MeCy) indicate that the potential energy surfaces are well-nested, such that ICT transitions are induced with small reorganization energies. In contrast, the blue-shifted absorption and broad, featureless red-shifted emission observed in polar solvents (CH2Cl2) indicate that significant reorganization occurs within the metal complex and its surrounding media (to a larger extent) as a result of the large change in dipole moment upon excitation (Δμcalc > 24 debye). Unlike MCAAC and MMAC complexes, where the radiative rate constant (kr) is fastest for the silver analog (Romanov et al., 2018; Hamze et al., 2019b), AuBZI has the fastest kr in accord with gold having the largest SOC constant.
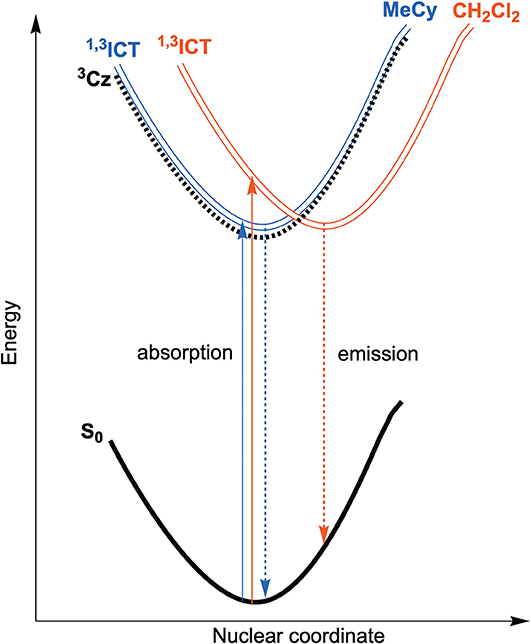
Figure 6. Qualitative energy diagram representing the ground state (S0) and both excited state potential energy surfaces (3Cz and 1,3ICT) as a function of nuclear coordinate in MeCy (blue) and CH2Cl2 (red) solution along with absorption (solid) and emission (dashed) transitions.
The biexponential character of the emission decay in PS thin films could be due to the presence of MBZI complexes in different conformations, one where the carbene and carbazole are in a coplanar orientation and another where the two ligands are twisted relative to each other. In our previous studies of (CAAC)Cu(carbazole) complexes we found that while the two forms display similar emission spectra the twisted form has a markedly longer excited state lifetime and lower oscillator strength than the coplanar form (Hamze et al., 2019a). In solution the excited MBZI can effectively rotate to the coplanar form prior to relaxing to the ground state. However, the rigid PS matrix will prevent the conformers from equilibrating in the excited state and thus they are expected to emit independently with different individual emission lifetimes.
The emission spectra display a pronounced rigidochromic shift upon cooling to 77 K and become extremely narrow and vibronically structured, with luminescence lifetimes in the millisecond regime. Thus, emission at low temperatures is consistent with a triplet transition localized on the carbazolide ligand (3Cz). This change in emission properties with temperature is attributed to the close energy separation between the 3Cz and 1/3ICT manifolds, making the ICT manifold thermally accessible at room temperature, but inaccessible in frozen MeCy and 2-MeTHF at 77 K. The fact that the MBZI complexes display 3Cz emission in PS (as well as MeCy and 2-MeTHF) at 77 K is different from the behavior observed in MCAAC and MMAC complexes. Emission from the latter complexes remains broad and featureless in a polystyrene matrix at all temperatures, even down to 4 K (Hamze et al., 2019b). Thus, in the case of the MCAAC and MMAC complexes, the 3ICT state lies below the energy of the 3Cz state in PS at all temperatures (Hamze et al., 2019b). However, for the MBZI complexes in PS films, it is evident that the lowest excited triplet state is indeed 3Cz at all temperatures. This difference suggests that the 3Cz and 3ICT states in the MBZI complexes are near degenerate in energy, and TADF emission occurs via thermal activation from the 3Cz to 1ICT states, not just within the ICT manifold as in the case of the MCAAC and MMAC complexes.
Another difference in the properties of the MBZI complexes compared to the MCAAC and MMAC analogs is the pronounced decrease in luminescence efficiency with increasing solvent polarity. For example, the quantum yield of CuBZI is severely diminished in CH2Cl2 relative to that recorded in MeCy (ΦPL = 0.03 in the former and 0.80 in the latter), whereas this decrease in efficiency is less pronounced for CuCAAC (ΦPL = 0.4 in CH2Cl2 and 0.92 in MeCy) and CuMAC (ΦPL = 0.5 in CH2Cl2 and 0.90 in MeCy). To better understand the origin of this decrease in ΦPL with solvent polarity, photophysical properties of AuBZI were characterized in mixtures of MeCy and CH2Cl2 at various ratios. The ICT band in the absorption spectra gradually blue shifts with increasing CH2Cl2 concentration and the vibronic fine structure observed in MeCy disappears in mixtures with ≥ 5 vol% CH2Cl2 (Figure 7A and Figure S6). Figure 7B shows that the radiative rate constant of AuBZI decreases with increasing solvent polarity, whereas the non-radiative rate constant (knr) remains near constant, consequently decreasing the ΦPL. The fact that the non-radiative rate constant of AuBZI is largely independent of CH2Cl2 concentration, despite the similar reduction potentials for the AuBZI excited state (E0/+* = −2.67 V) and CH2Cl2 (E0/− = −2.73 V), indicates that there is no oxidative quenching of excited AuBZI by CH2Cl2. Therefore, the lower ΦPL of AuBZI in CH2Cl2 comes about from a decrease in the radiative rate constant. This change is likely due to a decrease in the Franck-Condon factors for the ICT transition caused by the shift of the excited state surface in the polar solvent, as illustrated in Figure 6.
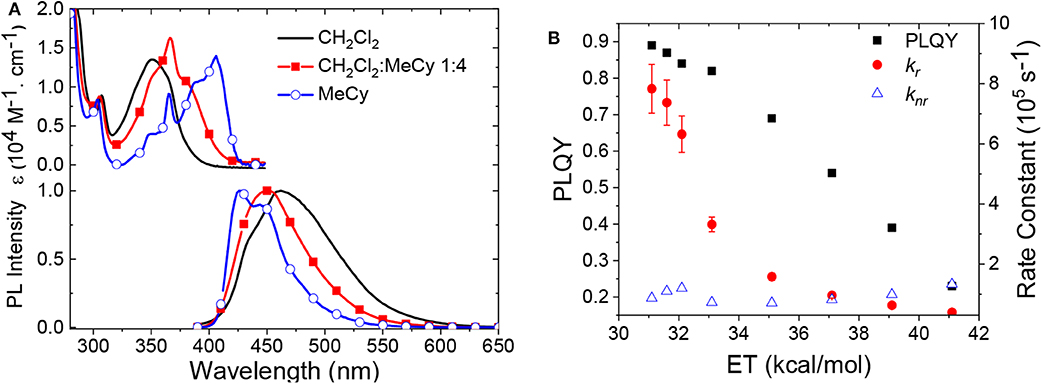
Figure 7. (A) Absorption and emission spectra of AuBZI in CH2Cl2, MeCy, and a mixture of both solvents. (B) Change in ΦPL and radiative and non-radiative rate constants of AuBZI as a function of solvent polarity.
Emission studies of AuBZI at variable temperature were conducted in MeCy and CH2Cl2 to investigate the parameters controlling TADF (Figure 8). Emission in MeCy slightly increases in intensity and displays limited changes in line shape with increasing temperature. In contrast, spectra recorded in CH2Cl2 reveal a drop in intensity with increasing temperature. Vibronic features resolved at low temperatures (190–230 K) are found to broaden abruptly at 240 K. The krvalues calculated from the quantum yields measured at various temperatures (see Supplementary Information) were fit to a two level model using Equation 1 (Figure 9A) (Hamze et al., 2019b).
Where, kB is the Boltzmann constant, kTADF and kfl are the radiative rate constants of the TADF and fluorescence, respectively and is the intersystem crossing rate (see Figure 9A). The best fit to the MeCy data (Figure 9B) give an energy difference between the triplet and emitting singlet state of AuBZI of 920 cm−1. However, the Arrhenius plot of the radiative rate constant of AuBZI recorded in CH2Cl2 at variable temperature is decidedly non-linear (Figure S10). Variable temperature NMR spectra indicate that an aquo species is formed with residual water below 240 K (Figure S11). The formation of this aquo complex likely leads to the anomalous behavior observed in CH2Cl2 at lower temperatures, making the analysis using the simple two-level model problematic.
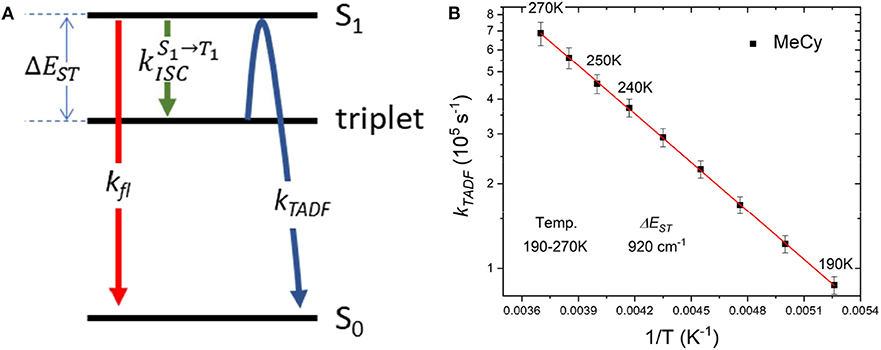
Figure 9. (A) Kinetic scheme for emission from AuBZI. (B) Arrhenius plot of the temperature-dependent lifetime data for AuBZI in MeCy recorded from 190 to 300 K, along with fits to the data according to Equation 1.
OLED Characterization
The AuBZI complex is stable to sublimation and was thus used as dopant to fabricate OLEDs by thermal evaporation. Device optimization and details are shown in the Supplementary Information. Considering the high triplet energy of AuBZI (ET = 3.1 eV), 1,3-bis(triphenylsilyl)benzene (UGH3, ET = 3.5 eV) was employed as the host matrix. Devices were fabricated using different doping levels (5, 10 and 15 wt%) and the best performance was obtained with 5 wt% AuBZI (see Supplementary Information). Optimized devices achieved reasonably high efficiencies (maximum EQE = 12%, Figure 10) and electroluminescence (EL) spectra (λmax = 430 nm, FWHM = 45 nm) identical to the PL spectrum in PS, demonstrating efficient exciton confinement on the complex. The color coordinates of the EL spectrum (CIE = 0.16, 0.06) make AuBZI an efficient deep blue dopant for phosphorescent OLEDs.
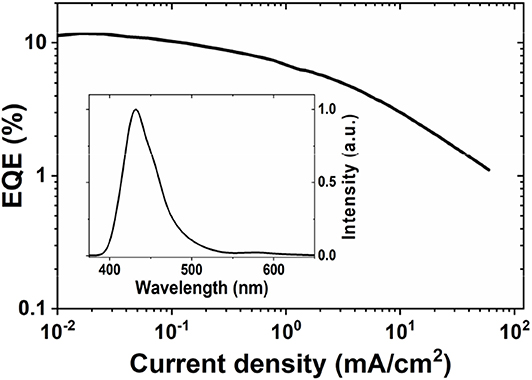
Figure 10. EQE vs. current density curve of 5 vol% doped devices of AuBZI. The EL spectrum is shown in the inset. Device architecture: ITO/10 nm HATCN/40 nm TAPC/40 nm UGH3:5 wt % AuBZI /40 nm TPBI/1 nm LiF/100 nm Al.
Conclusion
A series of 2-coordinate coinage metal (i.e., M = Cu, Ag, Au) complexes bearing a sterically bulky benzimidazolyl-carbene, 1,3-bis(2,6-diisopropylphenyl)-1-H-benzo[d]imidazol-2-ylidene (BZI), and carbazolide (Cz) as the anionic ligand were investigated. X-ray analysis reveals a linear geometry at the metal center with the ligands in a coplanar conformation, as well as orthogonal for AgBZI. The redox gap of all the complexes is large (ΔEredox > 3 V), in agreement with the high-energy absorption band (> 400 nm in CH2Cl2) corresponding to the carbazolide-to-carbene ICT transition. The complexes have high luminescence efficiencies (ΦPL >80%) and display deep blue narrow emission in MeCy and PS films. Their absorption spectra display negative solvatochromism, whereas their emission spectra undergo bathochromic shifts in polar solvents that is accompanied by decrease in quantum yields (ΦPL < 23%) and radiative rate constants (kr < 4.0 × 104 s−1). The non-radiative rate constants, however, are unaffected by the medium, remaining nearly the same in polar and nonplar media (knr ~ 1 × 105 s−1). Temperature-dependent studies reveal that the energy difference between the singlet and triplet excited states in methylcyclohexane is 920 cm−1. Vapor-deposited OLEDs fabricated using AuBZI as an emissive dopant have high efficiency (EQE = 12%) and a narrow and deep blue emission (CIE = 0.16, 0.06). These two-coordinate complexes present new opportunities for use as dopants in blue OLEDs. Lifetime studies on devices will need to be carried out to determine if these coinage metal-based emitters could serve as alternatives to state-of-the-art Ir(III) complexes commonly used in OLEDs.
Supporting Information
Synthesis of precursors and complexes, differential pulse voltammetry, cyclic voltammetry curves, photophysical data of the final complexes in various solvents, computational data and x-ray crystallographic data of the final complexes, OLED device fabrication procedure and device characteristics, 1H and 13C NMR of precursors and final complexes.
Data Availability Statement
The datasets generated for this study can be found in the Cambridge Crystallographic Data Center (https://www.ccdc.cam.ac.uk/structures/) under the identifiers CuBZI: 1984269, AgBZI: 1984268, and AuBZI: 1984267.
Author Contributions
RHam synthesized and measured the photophysical properties of the copper, silver, and gold compounds. MI measured and analyzed the temperature dependent photophysical properties of the gold complex. DM carried out the theoretical modeling. MJ prepared and characterized the OLEDs. RHai determined all of the crystal structures. RHam, PD, and MT conceived of the project. MI, PD, and MT wrote the manuscript.
Funding
This work was supported solely by the Universal Display Corporation. No funds have been received from Universal Displays, our institution or any other source to cover publication fees or open access fees.
Conflict of Interest
MT has a financial interest in the Universal Display Corporation. RHam is currently an employee of the Universal Display Corporation, however, all of her work in this paper was carried out when she was a graduate student at the University of Southern California.
The remaining authors declare that the research was conducted in the absence of any commercial or financial relationships that could be construed as a potential conflict of interest.
Acknowledgments
The authors wish to thank the Universal Display Corporation for their support of this work.
Supplementary Material
The Supplementary Material for this article can be found online at: https://www.frontiersin.org/articles/10.3389/fchem.2020.00401/full#supplementary-material
References
Di, D., Romanov, A. S., Yang, L., Richter, J. M., Rivett, J. P. H., Jones, S., et al. (2017). High-performance light-emitting diodes based on carbene-metal-amides. Science 356:159. doi: 10.1126/science.aah4345
Grätzel, M. (2005). Solar energy conversion by dye-sensitized photovoltaic cells. Inorganic Chem. 44, 6841–6851. doi: 10.1021/ic0508371
Grieco, G., Blacque, O., and Berke, H. (2015). A facile synthetic route to benzimidazolium salts bearing bulky aromatic N-substituents. Beilstein J. Organ. Chem. 11, 1656–1666. doi: 10.3762/bjoc.11.182
Hamze, R., Kapper, S. C., Ravinson, D. S. M., Haiges, R., Djurovich, P. I., and Thompson, M. E. (2020). Molecular dynamics of four-coordinate carbene-Cu(I) complexes employing tris(pyrazolyl)borate ligands. Polyhedron. 180:114381. doi: 10.1016/j.poly.2020.114381
Hamze, R., Jazzar, R., Soleilhavoup, M., Djurovich, P. I., Bertrand, G., and Thompson, M. E. (2017). Phosphorescent 2-, 3- and 4-Coordinate Cyclic (Alkyl)(Amino)Carbene (Caac) Cu(I) Complexes. Chem. Commun. 53, 9008–9011. doi: 10.1039/C7CC02638B
Hamze, R., Peltier, J. L., Sylvinson, D., Jung, M., Cardenas, J., Haiges, R., et al. (2019a). Eliminating Nonradiative Decay in Cu(I) Emitters: >99% quantum efficiency and microsecond lifetime. Science 363:601. doi: 10.1126/science.aav2865
Hamze, R., Shi, S., Kapper, S. C., Muthiah Ravinson, D. S., Estergreen, L., Jung, M.-C., et al. (2019b). “Quick-Silver” from a systematic study of highly luminescent, two-coordinate, D10 coinage metal complexes. J. Am. Chem. Soc. 141, 8616–8626. doi: 10.1021/jacs.9b03657
Kalyanasundaram, K. (1982). Photophysics, photochemistry and solar energy conversion with Tris(Bipyridyl)Ruthenium(Ii) and its analogues. Coord. Chem. Rev. 46, 159–244. doi: 10.1016/0010-8545(82)85003-0
Keefe, M. H., Benkstein, K. D., and Hupp, J. T. (2000). Luminescent sensor molecules based on coordinated metals: a review of recent developments. Coord. Chem. Rev. 205, 201–228. doi: 10.1016/S0010-8545(00)00240-X
Krylova, V. A., Djurovich, P. I., Conley, B. L., Haiges, R., Whited, M. T., Williams, T. J., et al. (2014). Control of emission colour with N-heterocyclic carbene (Nhc) ligands in phosphorescent three-coordinate Cu(I) Complexes. Chem. Commun. 50, 7176–7179. doi: 10.1039/C4CC02037E
Lamansky, S., Djurovich, P., Murphy, D., Abdel-Razzaq, F., Lee, H.-E., Adachi, C., et al. (2001). Highly phosphorescent bis-cyclometalated iridium complexes: synthesis, photophysical characterization, and use in organic light emitting diodes. J. Am. Chem. Soc. 123, 4304–4312. doi: 10.1021/ja003693s
Lo, K. K.-W., Louie, M.-W., and Zhang, K. Y. (2010). Design of Luminescent Iridium(III) and Rhenium(I) polypyridine complexes as in vitro and in vivo ion, molecular and biological probes. Coord. Chem. Rev. 254, 2603–2622. doi: 10.1016/j.ccr.2010.01.014
Romanov, A. S., Jones, S. T. E., Yang, L., Conaghan, P. J., Di, D., Linnolahti, M., et al. (2018). Mononuclear silver complexes for efficient solution and vacuum-processed oleds. Adv. Opt. Mater. 6:1801347. doi: 10.1002/adom.201801347
Romanov, A. S., Yang, L., Jones, S. T. E., Di, D., Morley, O. J., Drummond, B. H., et al. (2019). Dendritic carbene metal carbazole complexes as photoemitters for fully solution-processed oleds. Chem. Mater. 31, 3613–3623. doi: 10.1021/acs.chemmater.8b05112
Shi, S., Jung, M. C., Coburn, C., Tadle, A., Sylvinson, M. R. D, Djurovich, P. I., et al. (2019). Highly efficient photo- and electroluminescence from two-coordinate Cu(I) complexes featuring nonconventional N-Heterocyclic carbenes. J. Am. Chem. Soc. 141, 3576–3588. doi: 10.1021/jacs.8b12397
Sworakowski, J., Lipinski, J., and Janus, K. (2016). On the reliability of determination of energies of homo and lumo levels in organic semiconductors from electrochemical measurements. A simple picture based on the electrostatic model. Organ. Electron. 33, 300–310. doi: 10.1016/j.orgel.2016.03.031
Wang, H. M. J., Chen, C. Y. L., and Lin, I. J. B. (1999). Synthesis, structure, and spectroscopic properties of Gold(I)–carbene complexes. Organometallics 18, 1216–1223. doi: 10.1021/om980718b
Keywords: OLED, TADF, high efficiency, deep blue, copper, silver, gold, short lifetime
Citation: Hamze R, Idris M, Muthiah Ravinson DS, Jung MC, Haiges R, Djurovich PI and Thompson ME (2020) Highly Efficient Deep Blue Luminescence of 2-Coordinate Coinage Metal Complexes Bearing Bulky NHC Benzimidazolyl Carbene. Front. Chem. 8:401. doi: 10.3389/fchem.2020.00401
Received: 27 February 2020; Accepted: 16 April 2020;
Published: 08 May 2020.
Edited by:
Chihaya Adachi, Kyushu University, JapanReviewed by:
Yun CHI, City University of Hong Kong, Hong KongShin-ya Takizawa, The University of Tokyo, Japan
Feng Li, Jilin University, China
Copyright © 2020 Hamze, Idris, Muthiah Ravinson, Jung, Haiges, Djurovich and Thompson. This is an open-access article distributed under the terms of the Creative Commons Attribution License (CC BY). The use, distribution or reproduction in other forums is permitted, provided the original author(s) and the copyright owner(s) are credited and that the original publication in this journal is cited, in accordance with accepted academic practice. No use, distribution or reproduction is permitted which does not comply with these terms.
*Correspondence: Mark E. Thompson, met@usc.edu
†These authors have contributed equally to this work
‡Present address: Rasha Hamze, Universal Display Corporation, Ewing, NJ, United States