- 1College of Engineering and Technology, Southwest University, Chongqing, China
- 2Chongqing Electric Power Research Institute, State Grid Chongqing Electric Power Company, Chongqing, China
SnO2 based sensors has received extensive attention in the field of toxic gas detection due to their excellent performances with high sensitivity, fast response, long-term stability. Volatile organic compounds (VOCs), originate from industrial production, fuel burning, detergent, adhesives, and painting, are poisonous gases with significant effects on air quality and human health. This mini-review focuses on significant improvement of SnO2 based sensors in VOCs detection in recent years. In this review, the sensing mechanism of SnO2-based sensors detecting VOCs are discussed. Furthermore, the improvement strategies of the SnO2 sensor from the perspective of nanomaterials are presented. Finally, this paper summarizes the sensing performances of these SnO2 nanomaterial sensors in VOCs detection, and the future development prospect and challenges is proposed.
Introduction
Volatile Organic Compounds (VOCs) are the most crucial cause of indoor air pollution and harm to human health, including a variety of toxic compounds and carcinogens (Shrubsole et al., 2019). For example, organic waste gases such as formaldehyde and polycyclic aromatic hydrocarbons have strong carcinogenicity, when the human body is in this environment for a long time, the possibility of carcinogenesis will greatly increase. There are also some organic waste gas shows a strong toxic effect, the human body in the excessive inhalation, will lead to coma or even death (Li G. et al., 2019). In addition, VOCs exhaust gas may also cause environmental problems such as acid rain, ozone layer damage, and atmospheric warming (Meng et al., 2018). Therefore, it is very essential to analyze the composition and concentration of VOCs in the air. Current methods for detecting VOCs include Gas Chromatography (GC), Gas Chromatography-mass spectrometry (GC-ms) and gas sensor detection (Vesely et al., 2003; Teixeira et al., 2004). GC determination of a single sample requires reference to known standards, and GC-ms requires a high time cost and complicated process. More importantly, both methods are offline and cannot detect the content and change of VOCs in real-time. In recent years, gas sensors has been widely noticed because of its fast detection speed, small volume, simple measurement and on-line monitoring.
Carbon materials and metal oxide semiconductor materials like SnO2, ZnO, WO3, and In2O3 have received scientific and technological importance and are widely used to detect VOCs gases (Luo et al., 2016; Lin et al., 2019; Zhao et al., 2019b). SnO2 gas sensor has been extensively studied for its applications in air quality detection, flammable and explosive gas detection, and environmental monitoring (Zhang Q. Y. et al., 2018; Zhou et al., 2018c). Nanomaterials have become the focus of the best sensing materials in recent years. Nanomaterials have many natural advantages such as large specific surface area, small size, and lightweight (Lu et al., 2018a; Zhou et al., 2018a). At present, there are many kinds of structures such as nanowires, nanofilaments, nanowires hollow spheres, nanofilaments flowers, and nanotubes (Mirzaei et al., 2016; Zhang Q. Y. et al., 2017). Different nanostructures and morphologies have different effects on the properties of materials. In order to change the nanostructure of a single material, there are other ways to improve the gas sensitivity of the sensor. This mini-review summaries the gas-sensing performances of SnO2 based sensor, which were influenced by the microstructure, doping, oxide composite and noble metal modification, toward toluene (C6H5CH3), formaldehyde (HCHO), and acetone (C3H6O).
Sensing Mechanism of SnO2 Gas Sensor
SnO2 sensor is a surface-controlled gas sensor. The gas-sensing reaction can only cause changes in parameters such as surface conductivity of the semiconductor (Ducere et al., 2012; Korotcenkov and Cho, 2017). When exposed to air, oxygen molecules would be adsorbed on the surface of the SnO2 nanostructures and capture electrons from the conduction band of SnO2 to generate chemisorbed oxygen species [, O−, and O2−, depending on temperatures; (Shahabuddin et al., 2017; Zhou et al., 2019)]. The chemical adsorption process can be explained by the following reactions:
When SnO2 sensor contacts with the measured gas, its resistance will change according to the oxidation or reduction characteristics of the gas. Toluene, formaldehyde and acetone tested in this paper are reductive gases. When SnO2 material surface comes into contact with a reducing gas, the reducing gas will react with oxygen anions to produce carbon dioxide and water, and the resulting electrons will return to the conduction band of the semiconductor. Therefore, this process will increase the carrier concentration on the surface of SnO2 material, resulting in a decrease in the resistance value. When finally restored to the air environment, the sensor returns to its original state (Lu et al., 2018b; Al-Hashem et al., 2019; Mahajan and Jagtap, 2019). The sensing mechanism of the SnO2 sensor reacting with these gases can be represented by the following path, where O− is taken as an example (Lian et al., 2017; Zhu et al., 2019):
Optimization of SnO2 Gas-sensing Materials
With the development of semiconductor gas-sensing materials, it has been the focus of research to enhance their gas-sensing properties for gas detection. The most common preparation methods of SnO2 sensing materials include electrostatic spinning and hydrothermal methods, as shown in Figures 1A,B. Different preparation methods will change the structure and morphology of SnO2 sensing materials and further enhance the gas sensitivity (Long et al., 2018; Zhang Y. J. et al., 2018; Zhou et al., 2018b). This section mainly reflects the changes in the gas-sensing properties of SnO2 nanomaterials from the aspects of structure and morphology design, ion doping, oxide composite and noble metal modification (Chen et al., 2013; Das and Jayaraman, 2014).
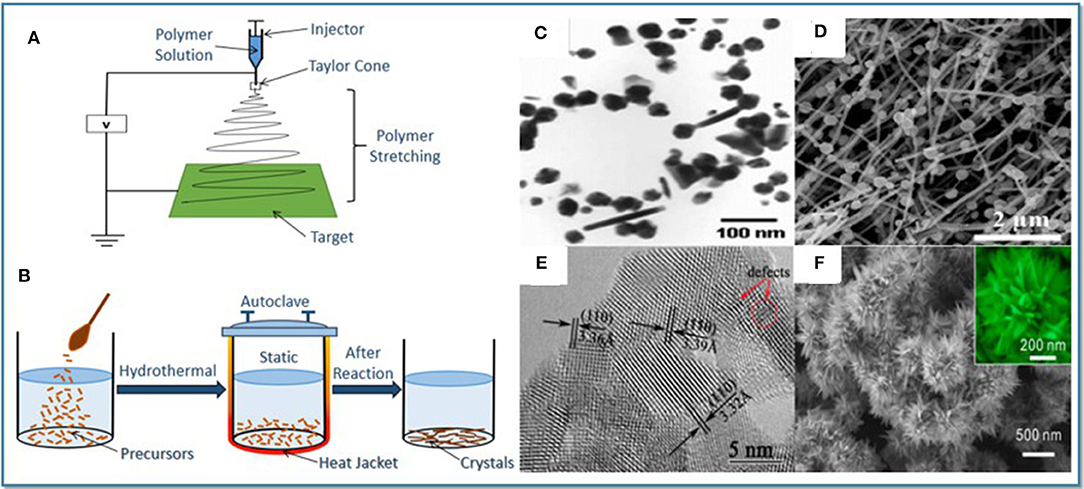
Figure 1. (A) A schematic of electrospinning method. (B) A schematic of hydrothermal method. (C) SnO2 nanoparticles. Reprinted with permission from Matussin et al. (2020). (D)SnO2/ZnO nanofibers. Reprinted with permission from Li H. et al. (2019). (E) SnO2 nanosheets. Reprinted with permission from Zhu et al. (2015). (F) SnO2 tapered layered nanostructures. Reprinted with permission from Li et al. (2017).
Different nanostructures and morphologies cause various effects on the properties of materials. In this respect, various morphologies from 0-D to 3-D with unique physical and chemical properties have been successfully synthesized. High dispersivity, ultra-small diameter 0-D SnO2 nanoparticles (Figure 1C) have highly effective surface areas and sufficient oxygen vacancies, which can improve the performance of nanoparticle based sensors (Matussin et al., 2020). 1-D SnO2 structure, such as nanofibers (Figure 1D), has excellent sensitivity and stability due to its large specific surface area, high porosity and good permeability (Li H. et al., 2019). Compared with low dimensional structure, 2-D structure possesses larger special surface area. In comparison to the 3-D structure, freestanding 2-D structures such as SnO2 nanosheets (Figure 1E) can provide better optimization including the modulation of the materials activity, surface polarization, and rich oxygen vacancies (Zhu et al., 2015). 3-D structures, such as microspheres, microflowers, and mesoporous structures, are assembled by diverse lower dimension fundamental blocks. Microstructured analyses suggest that the favorable gas sensitivity of SnO2 tapered layered nanostructures (Figure 1F) are mainly ascribed to the formation of more active surface defects and mismatches (Li et al., 2017).
Ion doping can change the cell parameters of the material, the number of suspensions on the surface of the material and the richness of defects, thereby enhance the gas sensitivity of the sensor (Korotcenkov and Cho, 2014). The Y-doped SnO2 three-dimensional flower-like nanostructure prepared by one-step hydrothermal method has a large number of rough nanoflakes, which increases the specific surface area and is more conducive to the adsorption and desorption of oxygen and formaldehyde gas. It is a highly sensitive formaldehyde detection material (Zhu et al., 2019). The doping of Ce ions into the SnO2 lattice results in the smaller size of nanoparticles and the formation of a porous structure. Therefore, Ce ions can provide more active sites for the adsorption and reaction of acetone (Lian et al., 2017).
The combination of two metal oxides can improve the gas sensitivity of semiconductor materials. The dispersion of functional components is the key factor to realize good gas sensitivities (Wei et al., 2020). Moreover, the heterostructure formed by SnO2 and another metal oxide can promote the transfer of carriers between materials and change the conductivity and energy band structure of composite materials (Gusain et al., 2019; Wei et al., 2019). In a recent research, a facile solvent EIOC has been demonstrated for the synthesis of novel hierarchical branched mesoporous TiO2-SnO2 semiconducting heterojunctions. The uniform distribution of SnO2 NCs in the pore walls of TiO2 forms numerous n-n heterojunctions which are extremely useful for surface catalytic reaction. Owing to the rational combination of a hierarchical mesoporous structure, a high crystallinity, and well-defined n-n heterojunctions, the SHMT-based gas sensor shows an excellent sensing performance with a fast response and recovery dynamics, ultralow limit of detection and a superior selectivity (Zhao et al., 2019a). The cactus-like WO3-SnO2 nanocomposite was prepared by one-step hydrothermal method by attaching many tiny SnO2 nanospheres to large WO3 nanospheres, which provided many active sites for the acetone molecule and provided heterojunctions between WO3 and SnO2. The synergistic effect between them improves the sensing performance of the composite nanomaterial to acetone gas (Zhu et al., 2018).
Precious metal modification usually uses Au, Ag, Pt, and Pd or their oxides to improve the sensitivity and response speed of gas sensing materials and reduce the working temperature. Ag modified SnO2 nanoparticles prepared by hydrothermal in situ reduction improved the sensor's ability to detect formaldehyde. This is due to the charge transfer between Ag and SnO2, which increases the absorption band on the composite by 20 nm, thus improving the gas sensitivity (Liu et al., 2019). When the acetone is detected by Ag/SnO2 porous tubular nanostructures prepared by electrospinning, the sensor resistance changed rapidly and significantly. On the one hand, the p-n hybrid interface of p-type Ag2O and n-type SnO2 causes the energy band of the depletion layer to bend, increasing the initial resistance. On the other hand, the hollow nanostructure promotes the adsorption and electron transfer of acetone, which makes the resistance change rapidly (Xu et al., 2017). The bimetal PdAu modified SnO2 nanosheet showed excellent selectivity and responsiveness to low concentrations of acetone, which is due to the chemical sensitization of Au, electronic sensitization of Pd and synergism of PdAu bimetal nanosheet (Li G. et al., 2019).
Sensing Performance of VOCs Based On SnO2 Nanomaterials
For VOCs, this review mainly introduces toluene, formaldehyde and acetone. This section summarizes the gas-sensitive characteristics of SnO2-based nanomaterials for the above gases, as shown in Table 1. In the detection of common VOCs, the lower detection limit, response value and detection temperature of SnO2 based nanomaterials are different.
Toluene, a colorless volatile liquid, is one of the most widely used aromatic hydrocarbons and is considered as a biomarker of cancer. Occupational Safety and Health Administration (OSHA) stipulates that the permissible exposure limit for toluene is 100 ppm for 8 h (Sui et al., 2017). The Pd-doped SnO2 hollow spheres prepared by hydrothermal method measured a response value of 52.9 for toluene at 20 ppm and a lower temperature of 230°C (Zhang K. et al., 2017). The Pd-loaded SnO2 cubic nanocages are also an ideal choice for toluene detection, with a minimum detection concentration of 100 ppb, a response to 20 ppm of toluene of 41.4, and an optimal reaction temperature of 250°C (Qiao et al., 2017). Formaldehyde is a colorless and pungent gas. Due to the toxicity of formaldehyde, OSHA has established the Threshold Limit Value (TLV) as a concentration of 0.75 ppm for 8 h. The SnO/SnO2 nano-flowers prepared by hydrothermal method have a minimum detection concentration of 8.15 for formaldehyde, an optimal response temperature of 120°C, and a response value of 80.9 at 50 ppm. It is an ideal material for formaldehyde detection (Li N. et al., 2019). The hydrothermal Ni doping of SnO2 nanoparticles also had a good response value of 130–100 ppm of formaldehyde at 200°C (Hu et al., 2018). In addition, the Ag-doped Zn2SnO4/SnO2 hollow nanospheres responded to 50 ppm of formaldehyde with a value of 62.2 and a lower detection temperature of 140°C (Zhang et al., 2019). Acetone is a colorless and irritant liquid. Long term exposure to acetone can stimulate human sensory organs and lead to inflammation. Therefore, the quantitative detection of acetone is of great significance (Cheng et al., 2015; Lian et al., 2017). PdAu decorated SnO2 nanosheets sensor was able to detect acetone at 45 ppb and to respond to acetone at 50 ppm to 109 (Li G. et al., 2019). The detection limit of Au @ WO3-SnO2 corrugated nanofibers prepared by hydrothermal treatment was 200 ppb acetone, and the best response to 0.5 ppm acetone at 150°C was 79.6. The Au@WO3-SnO2 corrugated nanofibers is an ideal low concentration acetone gas sensor with low detection limit and high response (Shao et al., 2019). La2O3-doped SnO2 nanoparticle thick films has an amazing response value of 3,626 −400 ppm at 350°C, which is suitable for the detection of high concentration acetone (Tammanoon et al., 2018).
Conclusion and Perspective
This review discusses the performance improvements of SnO2-based nanomaterials and the comparison of gas sensitivity in VOCs in recent years. SnO2-based nanostructures provide a larger specific surface area and more active sites, which is conducive to VOCs adsorption. Ion doping can reduce the size of nanomaterials and make the surface of the material rougher, thereby increasing the specific surface area. Metal oxide composite can not only achieve functional dispersion, but also form heterojunctions to promote the movement of charge carriers. Precious metals have excellent catalytic activity for SnO2 nanomaterials. These optimization methods make SnO2-based gas sensors operate at lower temperatures, higher sensitivity, and better stability. Despite great progress has been made in the application of SnO2 nanomaterials, there is still much room for further development. First of all, cross sensitivity is a huge challenge for the preparation of high-performance sensors. In the future, SnO2 gas sensor will be able to detect a single gas in the mixture. Secondly, most of the SnO2 sensors currently used work at high temperature, which limits their wide application in detecting VOCs at room temperature. In addition, long-term stability is also one of the research hotspots of SnO2 sensors in the future. Due to the influence of external environment and other factors, the stability of the sensor can not be guaranteed. Therefore, it is of great significance to develop more stable gas sensors. It has become a research hotspot to optimize the existing gas sensing materials by chemical modification and develop new gas sensing materials such as composite and hybrid semiconductor materials and polymer gas sensing materials. In addition, new sensors, such as optical waveguide gas sensor, quartz resonant gas sensor and microbial gas sensor, developed with advanced processing technology and microstructure, can make the sensor more stable, and versatility. Finally, we hope our work will be helpful for the further exploration of metal oxide nanomaterials in the detection of VOCs.
Author Contributions
All authors listed have made a substantial, direct and intellectual contribution to the work, and approved it for publication.
Funding
This work has been supported in part by the National Natural Science Foundation of China (No. 51507144), Fundamental Research Funds for the Central Universities (No. XDJK2019B021), the Chongqing Science and Technology Commission (CSTC) (No. cstc2016jcyjA0400), and the project of China Scholarship Council (CSC).
Conflict of Interest
The authors declare that the research was conducted in the absence of any commercial or financial relationships that could be construed as a potential conflict of interest.
References
Al-Hashem, M., Akbar, S., and Morris, P. (2019). Role of oxygen vacancies in nanostructured metal-oxide gas sensors: a review. Sensor. Actuat. B Chem. 301:126845. doi: 10.1016/j.snb.2019.126845
Chen, W. G., Zhou, Q., Gao, T. Y., Su, X. P., and Wan, F. (2013). Pd-doped SnO2-based sensor detecting characteristic fault hydrocarbon gases in transformer oil. J. Nanomater. 2013:127345. doi: 10.1155/2013/127345
Cheng, L., Ma, S. Y., Wang, T. T., and Luo, J. (2015). Synthesis and enhanced acetone sensing properties of 3D porous flower-like SnO2 nanostructures. Mater. Lett. 143, 84–87. doi: 10.1016/j.matlet.2014.12.062
Das, S., and Jayaraman, V. (2014). SnO2: a comprehensive review on structures and gas sensors. Prog. Mater. Sci. 66, 112–255. doi: 10.1016/j.pmatsci.2014.06.003
Ducere, J. M., Hemeryck, A., Esteve, A., Rouhani, M. D., Landa, G., Menini, P., et al. (2012). A computational chemist approach to gas sensors: modeling the response of SnO2 to CO, O2, and H2O gases. J. Comput. Chem. 33, 247–258. doi: 10.1002/jcc.21959
Gusain, R., Gupta, K., Joshi, P., and Khatri, O. P. (2019). Adsorptive removal and photocatalytic degradation of organic pollutants using metal oxides and their composites: a comprehensive review. Adv. Colloid. Interface. 272:102009. doi: 10.1016/j.cis.2019.102009
Hermawan, A., Asakura, Y., Inada, M., and Yin, S. (2019). One-step synthesis of micro-/mesoporous SnO2 spheres by solvothermal method for toluene gas sensor. Ceram. Int. 45, 15435–15444. doi: 10.1016/j.ceramint.2019.05.043
Hu, J., Wang, T., Wang, Y., Huang, D., He, G., Han, Y., et al. (2018). Enhanced formaldehyde detection based on Ni doping of SnO2 nanoparticles by one-step synthesis. Sensor. Actuat. B Chem. 263, 120–128. doi: 10.1016/j.snb.2018.02.035
Jiang, Z., Yin, M., and Wang, C. (2017). Facile synthesis of Ca2+/Au co-doped SnO2 nanofibers and their application in acetone sensor. Mater. Lett. 194, 209–212. doi: 10.1016/j.matlet.2017.02.031
Korotcenkov, G., and Cho, B. K. (2014). Bulk doping influence on the response of conductometric SnO2 gas sensors: understanding through cathodoluminescence study. Sensor. Actuat. B Chem. 196, 80–98. doi: 10.1016/j.snb.2014.01.108
Korotcenkov, G., and Cho, B. K. (2017). Metal oxide composites in conductometric gas sensors: achievements and challenges. Sensor. Actuat. B Chem. 244, 182–210. doi: 10.1016/j.snb.2016.12.117
Li, G., Cheng, Z., Xiang, Q., Yan, L., Wang, X., and Xu, J. (2019). Bimetal PdAu decorated SnO2 nanosheets based gas sensor with temperature-dependent dual selectivity for detecting formaldehyde and acetone. Sensor. Actuat. B Chem. 283, 590–601. doi: 10.1016/j.snb.2018.09.117
Li, H., Chu, S. S., Ma, Q., Li, H., Che, Q. D., Wang, J. P., et al. (2019). Multilevel effective heterojunctions based on SnO2/ZnO 1D fibrous hierarchical structure with unique interface electronic effects. Acs. Appl. Mater. Inter. 11, 31551–31561. doi: 10.1021/acsami.9b10410
Li, N., Fan, Y., Shi, Y., Xiang, Q., Wang, X., and Xu, J. (2019). A low temperature formaldehyde gas sensor based on hierarchical SnO/SnO2 nano-flowers assembled from ultrathin nanosheets: Synthesis, sensing performance and mechanism. Sensor. Actuat. B Chem. 294, 106–115. doi: 10.1016/j.snb.2019.04.061
Li, Y. X., Guo, Z., Su, Y., Jin, X. B., Tang, X. H., Huang, J. R., et al. (2017). Hierarchical morphology-dependent gas-sensing performances of three-dimensional SnO2 nanostructures. Acs. Sens. 2, 102–110. doi: 10.1021/acssensors.6b00597
Lian, X., Li, Y., Tong, X., Zou, Y., Liu, X., An, D., et al. (2017). Synthesis of Ce-doped SnO2 nanoparticles and their acetone gas sensing properties. Appl. Surf. Sci. 407, 447–455. doi: 10.1016/j.apsusc.2017.02.228
Lin, T., Lv, X., Hu, Z., Xu, A., and Feng, C. (2019). Semiconductor metal oxides as chemoresistive sensors for detecting volatile organic compounds. Sensors Basel. 19:233. doi: 10.3390/s19020233
Liu, D., Pan, J., Tang, J., Liu, W., Bai, S., and Luo, R. (2019). Ag decorated SnO2 nanoparticles to enhance formaldehyde sensing properties. J. Phys. Chem. Solids. 124, 36–43. doi: 10.1016/j.jpcs.2018.08.028
Long, H., Zeng, W., Wang, H., Qian, M., Liang, Y., and Wang, Z. (2018). Self-assembled biomolecular 1D nanostructures for aqueous sodium-ion battery. Adv. Sci. 5:1700634. doi: 10.1002/advs.201700634
Lu, Z. R., Zhou, Q., Wang, C. S., Wei, Z. J., Xu, L. N., and Gui, Y. G. (2018a). Electrospun ZnO-SnO2 composite nanofifibers and enhanced sensing properties to SF6 decomposition byproduct H2S. Front. Chem. 6:540. doi: 10.3389/fchem.2018.00540
Lu, Z. R., Zhou, Q., Xu, L. N., Gui, Y. G., Zhao, Z. Y., Tang, C., et al. (2018b). Synthesis and characterization of highly sensitive hydrogen (H2) sensing device based on Ag doped SnO2 nanospheres. Materials. 11:492. doi: 10.3390/ma11040492
Luo, W., Zhao, T., Li, Y. H., Wei, J., Xu, P. C., Li, X. X., et al. (2016). A micelle fusion-aggregation assembly approach to mesoporous carbon materials with rich active sites for ultra-sensitive ammonia. Sensing. J. Am. Chem. Soc. 138, 12586–12595. doi: 10.1021/jacs.6b07355
Mahajan, S., and Jagtap, S. (2019). Metal-oxide semiconductors for carbon monoxide (CO) gas sensing: a review. Appl. Mater. Today. 18:100483. doi: 10.1016/j.apmt.2019.100483
Matussin, S., Harunsani, M. H., Tan, A. L., and Khan, M. M. (2020). Plant-extract-mediated SnO2 nanoparticles: synthesis and applications. Acs. Sustain. Chem. Eng. 8, 3040–3054. doi: 10.1021/acssuschemeng.9b06398
Meng, D., Liu, D., Wang, G., Shen, Y., San, X., Li, M., et al. (2018). Low-temperature formaldehyde gas sensors based on NiO-SnO2 heterojunction microflowers assembled by thin porous nanosheets. Sensor. Actuat. B Chem. 273, 418–428. doi: 10.1016/j.snb.2018.06.030
Mirzaei, A., Leonardi, S. G., and Neri, G. (2016). Detection of hazardous volatile organic compounds (VOCs) by metal oxide nanostructures-based gas sensors: a review. Ceram. Int. 42, 15119–15141. doi: 10.1016/j.ceramint.2016.06.145
Qiao, L., Bing, Y., Wang, Y., Yu, S., Liang, Z., and Zeng, Y. (2017). Enhanced toluene sensing performances of Pd- loaded SnO2 cubic nanocages with porous nanoparticle-assembled shells. Sensor. Actuat. B Chem. 241, 1121–1129. doi: 10.1016/j.snb.2016.10.024
Shahabuddin, M., Umar, A., Tomar, M., and Gupta, V. (2017). Custom designed metal anchored SnO2 sensor for H2 detection. Int. J. Hydrogen Energ. 42, 4597–4609. doi: 10.1016/j.ijhydene.2016.12.054
Shao, S., Chen, X., Chen, Y., Lai, M., and Che, L. (2019). Ultrasensitive and highly selective detection of acetone based on Au@WO3-SnO2 corrugated nanofibers. Appl. Surf. Sci. 473, 902–911. doi: 10.1016/j.apsusc.2018.12.208
Shrubsole, C., Dimitroulopoulou, S., Foxall, K., Gadeberg, B., and Doutsi, A. (2019). IAQ guidelines for selected volatile organic compounds (VOCs) in the UK. Build. Environ. 165:106382. doi: 10.1016/j.buildenv.2019.106382
Sui, L. L., Zhang, X. F., Cheng, X. L., Wang, P., Xu, Y. M., Gao, S., et al. (2017). Au-loaded hierarchical MoO3 hollow spheres with enhanced gas-sensing performance for the detection of BTX (benzene, toluene, and xylene) and the sensing mechanism. Acs. Appl. Mater. Inter. 9, 1661–1670. doi: 10.1021/acsami.6b11754
Tammanoon, N., Wisitsoraat, A., Phokharatkul, D., Tuantranont, A., Phanichphant, S., Yordsri, V., et al. (2018). Highly sensitive acetone sensors based on flame-spray-made La2O3-doped SnO2 nanoparticulate thick films. Sensor. Actuat. B Chem. 262, 245–262. doi: 10.1016/j.snb.2018.01.238
Teixeira, L. S., Leao, E. S., Dantas, A. F., Pinheiro, H. L., Costa, A. C., and de Andrade, J. B. (2004). Determination of formaldehyde in Brazilian alcohol fuels by flow-injection solid phase spectrophotometry. Talanta 64, 711–715. doi: 10.1016/j.talanta.2004.03.047
Vesely, P., Lusk, L., Basarova, G., Seabrooks, J., and Ryder, D. (2003). Analysis of aldehydes in beer using solid-phase microextraction with on-fiber derivatization and gas chromatography/mass spectrometry. J. Agr. Food Chem. 51, 6941–6944. doi: 10.1021/jf034410t
Wang, D., Zhang, M., Chen, Z., Li, H., Chen, A., Wang, X., et al. (2017). Enhanced formaldehyde sensing properties of hollow SnO2 nanofibers by graphene oxide. Sensor. Actuat. B Chem. 250, 533–542. doi: 10.1016/j.snb.2017.04.164
Wei, Z. J., Zhou, Q., Wang, J. X., Lu, Z. R., Xu, L. N., and Zeng, W. (2019). Hydrothermal synthesis of SnO2 nanoneedle-anchored NiO microsphere and its gas sensing performances. Nanomaterials Basel. 9:1015. doi: 10.3390/nano9071015
Wei, Z. J., Zhou, Q., and Zeng, W. (2020). Hierarchical WO3-NiO microflower for high sensitivity detection of SF6 decomposition byproduct H2S. Nanotechnology 31:215701. doi: 10.1088/1361-6528/ab73bd
Xie, N., Guo, L. L., Chen, F., Kou, X. Y., Wang, C., Ma, J., et al. (2018). Enhanced sensing properties of SnO2 nanofibers with a novel structure by carbonization. Sensor. Actuat. B Chem. 271, 44–53. doi: 10.1016/j.snb.2018.05.039
Xu, X., Chen, Y., Zhang, G., Ma, S., Lu, Y., Bian, H., et al. (2017). Highly sensitive VOCs-acetone sensor based on Ag-decorated SnO2 hollow nanofibers. J. Alloy. Compd. 703, 572–579. doi: 10.1016/j.jallcom.2017.01.348
Yu, H., Yang, T., Wang, Z., Li, Z., Xiao, B., Zhao, Q., et al. (2017). Facile synthesis cedar-like SnO2 hierarchical micro-nanostructures with improved formaldehyde gas sensing characteristics. J. Alloy. Compd. 724, 121–129. doi: 10.1016/j.jallcom.2017.07.017
Zhang, K., Yang, X., Wang, Y., Bing, Y., Qiao, L., Liang, Z., et al. (2017). Pd-loaded SnO2 ultrathin nanorod-assembled hollow microspheres with the significant improvement for toluene detection. Sensor. Actuat. B Chem. 243, 465–474. doi: 10.1016/j.snb.2016.11.153
Zhang, Q. Y., Zhou, Q., Lu, Z. R., Wei, Z. J., Xu, L. N., and Gui, Y. G. (2018). Recent advances of SnO2-based sensors for detecting fault characteristic gases extracted from power transformer oil. Front. Chem. 6:364. doi: 10.3389/Fchem.2018.00364
Zhang, Q. Y., Zhou, Q., Yin, X. T., Liu, H. C., Xu, L. N., Tan, W. M., et al. (2017). The effect of PMMA pore-forming on hydrogen sensing properties of porous SnO2 thick film sensor. Adv. Sci. 9, 1350–1355. doi: 10.1166/sam.2017.3111
Zhang, R., Ma, S. Y., Zhang, Q. X., Zhu, K. M., Tie, Y., Pei, S. T., et al. (2019). Highly sensitive formaldehyde gas sensors based on Ag doped Zn2SnO4/SnO2 hollow nanospheres. Mater. Lett. 254, 178–181. doi: 10.1016/j.matlet.2019.07.065
Zhang, Y. J., Zeng, W., and Li, Y. Q. (2018). The hydrothermal synthesis of 3D hierarchical porous MoS2 microspheres assembled by nanosheets with excellent gas sensing properties. J. Alloy. Compd. 749, 355–362. doi: 10.1016/j.jallcom.2018.03.307
Zhao, R., Zhang, X., Peng, S., Hong, P., Zou, T., Wang, Z., et al. (2020). Shaddock peels as bio-templates synthesis of Cd-doped SnO2 nanofibers: a high performance formaldehyde sensing material. J. Alloy. Compd. 813:152170. doi: 10.1016/j.jallcom.2019.152170
Zhao, T., Qiu, P. P., Fan, Y. C., Yang, J. P., Jiang, W., Wang, L. J., et al. (2019a). Hierarchical branched mesoporous TiO2-SnO2 nanocomposites with well-defined n-n heterojunctions for highly efficient ethanol sensing. Adv. Sci. 6:1902008. doi: 10.1002/advs.201902008
Zhao, T., Ren, Y., Jia, G. Y., Zhao, Y. Y., Fan, Y. C., Yang, J. P., et al. (2019b). Facile synthesis of mesoporous WO3@graphene aerogel nanocomposites for low-temperature acetone sensing. Chin. Chem. Lett. 30, 2032–2038. doi: 10.1016/j.cclet.2019.05.006
Zhou, Q., Chen, W. G., Xu, L. N., Kumar, R., Gui, Y. G., Zhao, Z. Y., et al. (2018a). Highly sensitive carbon monoxide (CO) gas sensors based on Ni and Zn doped SnO2 nanomaterials. Ceram. Int. 44, 4392–4399. doi: 10.1016/j.ceramint.2017.12.038
Zhou, Q., Umar, A., Sodki, E., Amine, A., Xu, L. N., Gui, Y. G., et al. (2018b). Fabrication and characterization of highly sensitive and selective sensors based on porous NiO nanodisks. Sensor. Actuat. B Chem. 259, 604–615. doi: 10.1016/j.snb.2017.12.050
Zhou, Q., Xu, L. N., Umar, A., Chen, W. G., and Kumar, R. (2018c). Pt nanoparticles decorated SnO2 nanoneedles for effificient CO gas sensing applications. Sensor. Actuat. B Chem. 256, 656–664. doi: 10.1016/j.snb.2017.09.206
Zhou, Q., Zeng, W., Chen, W. G., Xu, L. N., Kumar, R., and Umar, A. (2019). High sensitive and low-concentration sulfur dioxide (SO2) gas sensor application of heterostructure NiO-ZnO nanodisks. Sensor. Actuat. B Chem. 298:126870. doi: 10.1016/j.snb.2019.126870
Zhu, K., Ma, S., Tie, Y., Zhang, Q., Wang, W., Pei, S., et al. (2019). Highly sensitive formaldehyde gas sensors based on Y-doped SnO2 hierarchical flower-shaped nanostructures. J. Alloy. Compd. 792, 938–944. doi: 10.1016/j.jallcom.2019.04.102
Zhu, L., Zeng, W., and Li, Y. (2018). A novel cactus-like WO3-SnO2 nanocomposite and its acetone gas sensing properties. Mater. Lett. 231, 5–7. doi: 10.1016/j.matlet.2018.08.007
Keywords: SnO2 based sensor, gas detection, VOCs, nanomaterials, improvement strategies
Citation: Li B, Zhou Q, Peng S and Liao Y (2020) Recent Advances of SnO2-Based Sensors for Detecting Volatile Organic Compounds. Front. Chem. 8:321. doi: 10.3389/fchem.2020.00321
Received: 29 January 2020; Accepted: 30 March 2020;
Published: 05 May 2020.
Edited by:
Weiwei Wu, Xidian University, ChinaCopyright © 2020 Li, Zhou, Peng and Liao. This is an open-access article distributed under the terms of the Creative Commons Attribution License (CC BY). The use, distribution or reproduction in other forums is permitted, provided the original author(s) and the copyright owner(s) are credited and that the original publication in this journal is cited, in accordance with accepted academic practice. No use, distribution or reproduction is permitted which does not comply with these terms.
*Correspondence: Qu Zhou, emhvdXF1QHN3dS5lZHUuY24=