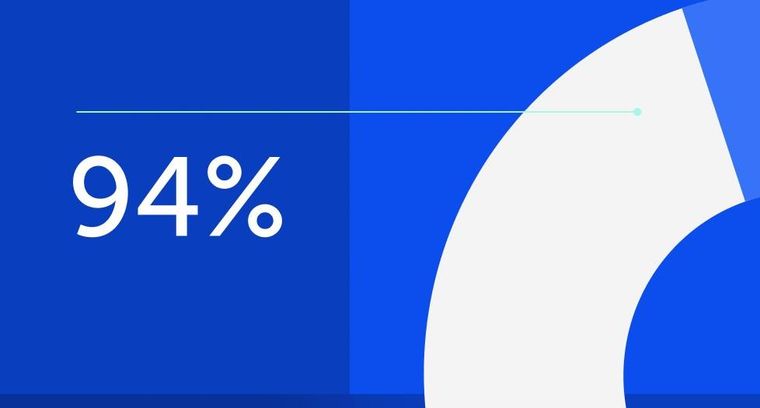
94% of researchers rate our articles as excellent or good
Learn more about the work of our research integrity team to safeguard the quality of each article we publish.
Find out more
ORIGINAL RESEARCH article
Front. Chem., 21 April 2020
Sec. Nanoscience
Volume 8 - 2020 | https://doi.org/10.3389/fchem.2020.00238
This article is part of the Research TopicNanoscience Editor’s Pick 2021View all 17 articles
We report a new sensor for the specific detection of lead ions (Pb2+) in contaminated water based on fluorescence resonance energy transfer (FRET) between upconversion nanoparticles (UCNPs) as donors and gold nanoparticles (Au NPs) as receptors. The UCNPs modified with Pb2+ aptamers could bind to Au NPs, which were functionalized with complementary DNA through hybridization. The green fluorescence of UCNPs was quenched to a maximum rate of 80% due to the close proximity between the energy donor and the acceptor. In the presence of Pb2+, the FRET process was broken because Pb2+ induced the formation of G-quadruplexes from aptamers, resulting in unwound DNA duplexes and separated acceptors from donors. The fluorescence of UCNPs was restored, and the relative intensity had a significant linear correlation with Pb2+ concentration from 0 to 50 nM. The sensor had a detection limit as low as 4.1 nM in a buffer solution. More importantly, the sensor exhibited specific detection of Pb2+ in complex metal ions, demonstrating high selectivity in practical application. The developed FRET prober may open up a new insight into the specific detection of environmental pollution.
Lead ion (Pb2+), one of the most serious metallic toxicants, can damage cardiovascular, reproductive, neurological, and developmental systems of the human body at low concentrations in the blood (Yoosaf et al., 2007; Zhou et al., 2011; Kim et al., 2012; Li et al., 2013). Although traditional methods including inductively coupled plasma mass spectrometry (Xia et al., 2008; Gao et al., 2009), atomic absorption spectroscopy (Bravo-Sanchez et al., 2001), and high-performance liquid chromatography (Yang et al., 2003) are highly sensitive and accurate, complicated pretreatment, and implementation limit their applicability for on-site rapid detection. Therefore, it is of important significance to develop analytical strategies with facile and straightforward features.
The detection assay composed of fluorescence and DNA molecules has attracted more and more attention mainly due to sensitive fluorescence signal, stable DNA molecules, and highly specific binding ability between specific sequences and target detectors (e.g., protein, ions, virus, and nucleic acid aptamers) (Hamaguchi et al., 2001; Pavlov et al., 2004; Xiao et al., 2005; Chang et al., 2010; Saha et al., 2012). Zhou and co-workers labeled 6-carboxyfluorescein on G-rich DNA strands and monitored the reduction of fluorescence for Pb2+ detection (Zhan et al., 2013). Shi and co-workers developed a new strategy based on DNA-templated silver nanoclusters with elevated fluorescence for L-histidine detection (Zheng et al., 2015). However, reported substances or quenchers in the actual environment might weaken the fluorescence and lead to false-positive results. Therefore, the “turn-off–on” detection system based on fluorescence resonance energy transfer (FRET) is introduced to avoid external interferences.
Upconversion nanoparticles (UCNPs) have rapidly emerged owing to their unique luminescent properties (Wang et al., 2005; Mader et al., 2010; Haase and Schaefer, 2011; Chen et al., 2014). Compared to traditional fluorescent markers, the UCNPs presented low auto-fluorescence, narrow emission width, no flicker, and strong light stability, leading to a wide application in biological and environmental monitoring and sensing (Chen and Zhao, 2012; Chen et al., 2013, 2014; Dacosta et al., 2014). Therefore, upconversion luminescence, as sensing signal under excitation of the near-infrared ray (NIR) light, effectively diminished the background noise in a complicated detection system (Wu et al., 2014). Meanwhile, gold nanoparticles (Au NPs) are superior fluorescence quenching agents due to the large extinction coefficient and a wide absorption band in the UV–visible region (Peng et al., 2011; Lin et al., 2013; Liu et al., 2013). The FRET systems were established based on upconversion nanoparticles and gold nanoparticles for the detection of avidin and Cr3+ (Wang et al., 2005; Liu et al., 2013).
In this paper, NaYF4: Yb, Er @NaYF4 UCNPs as energy donors and Au NPs as energy receptors are employed as FRET system for the sensitive detection of Pb2+. The donors and receptors are paired by two complementary DNA strands with good quenching ability for UCNPs. Single-stranded DNA for modifying UCNPs is rich in G base, which can fold to G-quadruplex structure in the presence of Pb2+. DNA duplex is then disrupted and the FRET system between UCNPs and Au NPs is cleaved, resulting in the restoration of fluorescence. The concentration of Pb2+ can be detected by monitoring the fluorescence recovery.
Anhydrous yttrium trichloride (YCl3, 99.99%), anhydrous ytterbium trichloride (YbCl3, 99.9%), anhydrous erbium chloride (ErCl3, 99.99%), 1-octadecene (ODE, 90%), oleic acid (OA, 90%), sodium hydroxide (NaOH, 96%), and ammonium fluoride (NH4F, 96%) were purchased from Sigma-Aldrich. Trihydroxy methyl aminomethane (Tris), HCl, NaCl, KCl, CaCl2, MgCl2, CuCl2, ZnCl2, and FeCl3 were obtained from Sinopharm. All chemicals were used directly without any further purification. Deionized water was purified by a Milli-Q system (Millipore, Bedford, MA, USA). The lead standard solution (1,000 mg/L) was purchased from Aladdin Industrial Inc. All nucleic acid molecules were prepared by Bioengineering Co., Ltd. (Shanghai). The sequences were as follows:
DNA1: 5′>AAGGGT GGGT GGGT <3′
DNA2: 5′>AAAAA AAAAA AAAAA AAAAA TTTTT CACCC TCCC AC <3′
The NaYF4: 18% Yb, 2% Er UCNPs were prepared according to the previous report (Li and Zhang, 2008). Typically, YCl3 (0.80 mmol), YbCl3 (0.18 mmol), ErCl3 (0.02 mmol), OA (6.0 ml), and ODE (15 ml) were mixed and heated to 140°C under vacuum for 1 h before cooling down to room temperature. Thereafter, NH4F (4.0 mmol) and NaOH (2.5 mmol) in methanol (10 ml) was added to the resulting solution and stirred for 30 min. The mixture was then transferred to a vacuum oven at 70°C for 30 min and heated at 300°C under argon flow for 1 h. NaYF4: 18% Yb, 2% Er cores were obtained as final product. As-prepared nanoparticles were washed with ethanol for several times and dispersed in 10 ml of cyclohexane.
To prepare NaYF4: Yb, Er @NaYF4 UCNPs, YCl3 (0.25 mmol), OA (6.0 ml), and ODE (15 ml) were mixed and transferred to a vacuum oven at 140°C for 1 h. The solution was added with NaYF4: Yb, Er initial core solution (5 ml) after cooling down to room temperature and heated at 70°C in a vacuum oven for 30 min to remove cyclohexane. Subsequently, the obtained mixture was further maintained at 280°C under argon atmosphere for 1 h. The NaYF4: Yb, Er @NaYF4 UCNPs were washed before dispersing in cyclohexane.
Ligand-free UCNPs were synthesized ahead of DNA modification following the method reported by Bogdan et al. (2011). The oleic acid-capped UCNPs in cyclohexane were centrifuged by adding ethanol as precipitant. Then, 100 mg of UCNPs was mixed with 10 ml water, and the pH of the solution was adjusted to 4 with 0.1 M hydrochloric acid solution. The solution was extracted three times with diethyl ether after stirring for 2 h, and the nanoparticles obtained were transferred to the aqueous layer and precipitated with acetone. Afterward, the oleic acid-ligand layer was removed and the ligand-free UCNPs were dispersed in water (5 ml).
DNA1 (200 nmol) was added to the solution of ligand-free UCNPs (20 μmol Ln3+). Following stirring overnight at room temperature, the mixture was centrifuged to remove excessive DNA1. The resulting DNA1-modified UCNPs were dispersed in Tris-HCl buffer solution (20 mM, 1 mM MgCl2, 2 mM KCl, and 100 mM NaCl, pH 7.4) and stored at 4°C.
Au NPs were prepared based on the previously reported method (Chen et al., 2012; Pei et al., 2012). The boiling HAuCl4 (HAuCl4·4H2O, 99.99%) solution was added with trisodium citrate solution (1%) with 20 min of stirring and then cooled down to room temperature to obtain Au NPs.
DNA2 was added to citrate-stabilized Au NPs with a stoichiometric ratio of 1:50, and a tiny amount of sodium citrate-hydrochloric acid buffer solution (500 mM, pH 3.0) was then rapidly added to the said mixture to make a final concentration of 10 mM. The solution was centrifuged for several times to remove excessive DNA2. DNA2-modified Au NPs were yielded and dispersed in Tris-HCl buffer solution (20 mM, pH 7.4).
The concentration of DNA1-modified UCNPs was immobilized, and DNA2-modified AuNPs with varied concentrations were added to the system. The mixture was incubated for 90 min at 37°C. The optimal concentration of AuNPs-DNA2 was determined by the fluorescence quenching efficiency of UCNPs-DNA1.
The Pb2+ standard solution with different concentrations was added to a mixed solution including UCNPs-DNA1 and AuNPs-DNA2, which had been incubated for 90 min. The said solution was further incubated for 30 min to measure its fluorescence spectra.
Transmission electron microscopy (TEM) images were taken with a JEM-2100F microscope (200 kV, with a Gatan imaging system). The upconversion fluorescence spectra were performed on a Hitachi F4500 fluorescence spectrometer (xenon lamp excitation source with a 980-nm laser). The UV–vis absorption spectra were characterized by a Lambda 750S UV/Vis/NIR spectrometer.
The sensor for Pb2+ detection was based on FRET from modified UCNPs to Au NPs by an aptamer matching its complement (Figure 1). Firstly, UCNPs were treated with hydrochloric acid to remove the hydrophobic surface ligands and modified with the aptamers (DNA1). Au NPs were functionalized with the complementary DNA (DNA2). Secondly, the FRET system was established with UCNPs as donors and Au NPS as receptors. The distance between UCNPs and Au NPs was shortened to <10 nm because of complementary DNA hybridization, leading to quenched fluorescence of UCNPs. Thirdly, the aptamers preferred to bind with metal ions and turned into intermolecular G-quadruplexes in the presence of Pb2+. Therefore, the DNA duplexes were unwound and upconversion fluorescence was restored to determine the concentration of Pb2+.
Figure 1. The schematic illustration of sensing assay constructed by UCNPs and Au NPs for Pb2+ detection.
The NaYF4: 18% Yb, 2% Er with a diameter of 45 nm was fabricated by the solvent–thermal method in Figure 2A (Li et al., 2014) and further coated with the NaYF4 passivation shell for the formation of NaYF4: 18% Yb, 2% Er @NaYF4 core/shell-structured UCNPs with a diameter of about 50 nm (Figure 2B). The OA ligands capped on UCNPs were treated with hydrochloric acid to form the water-soluble ligand-free UCNPs (Figure 2C). The TEM image shows that the obtained water-soluble ligand-free UCNPs remained uniform and monodispersed. Upon DNA1 modification, the UCNPs retained good dispersion with negligible morphological and size alteration (Figure 2D). The fluorescence of UCNPs was slightly quenched by water after being modified with DNA1 (Figure 2E). A strong absorption peak at 260 nm from the DNA was clearly observed in the spectrum of the DNA1-modified UCNPs, confirming that the UCNPs were successfully modified with DNA-1 (Figure 2F).
Figure 2. TEM images of (A) NaYF4: 18% Yb and 2% Er, (B) NaYF4: 18% Yb and 2% Er @NaYF4, (C) ligand-free UCNPs, and (D) DNA1-modified UCNPs (UCNPs-DNA1). (E) The upconversion fluorescence spectra of UCNPs with OA surface ligand (UCNPs-OA) and DNA aptamers (UCNPs-DNA1) under excitation of 980 nm. (F) The UV–vis absorption spectra of UCNPs-DNA1.
Au NPs with a diameter of 10 nm (Figure 3A) were modified with DNA2 molecules, which were partially complementary with DNA1. The DNA2-modified Au NPs remained of good dispersion (Figure 3B). The significant DNA absorption peak was observed at 260 nm, while the maximum absorption peak of Au NPs at 520 nm presented no significant variation (Figure 3C). A spectral overlap was illustrated in the range of 510–570 nm between UCNPs and Au NPs (Figure 3D).
Figure 3. TEM images of (A) Au NPs and (B) DNA2-modified Au NPs (Au NPs-DNA2). (C) The UV–vis absorption spectra of Au NPs and Au NPs-DNA2. (D) The upconversion fluorescence spectra of UCNPs-DNA1 and the UV–vis absorption spectra of Au NPs-DNA2.
The fluorescence intensity of UCNPs gradually decreases along with the elevation of DNA2-modified Au NPs (Figure 4A). The concentration of UCNPs was fixed at 6.56 mg/L accompanied by adjusting the concentration of Au NPs. The quenching efficiency of upconversion fluorescence climbed up to 80% at a concentration of 99.75 nM and remained stable at higher concentrations (Figure 4B). Therefore, the optimal concentration of the DNA2-modified Au NPs is 99.75 nM. In Figure 4C, the UCNPs are surrounded by Au NPs, indicating the construction of the FRET system between UCNPs and Au NPs. Moreover, the fluorescence quenching efficiency reached a plateau (80%) after 90 min of incubation (Figure 4D). Therefore, 90 min of incubation is adopted to achieve a stable fluorescence signal.
Figure 4. (A) The upconversion fluorescence spectra and (B) quenching efficiency of UCNPs-DNA1 after incubation at various concentrations of AuNPs-DNA2 in FRET system. (C) TEM image of FRET array fabricated from UCNPs-DNA1 and AuNPs-DNA2. (D) The fluorescence quenching efficiency with increasing incubation time.
The optimal concentrations of UCNPs (6.56 mg/L) and Au NPs (99.75 nM) were chosen for the detection system. After adding the lead standard solution to the system, the DNA aptamers on the surface of the UCNPs were induced to form G-quadruplexes by Pb2+, leading to the double-stranded DNA unwinding. Subsequently, the energy transfer system between the UCNP energy donors and Au NP receptors was broken, and the upconversion fluorescence was regained (Figures 5A,B). The fluorescence is restored gradually along with the elevation of concentration (Figure 5C). Linear correlation was demonstrated between the recovery of fluorescence and the concentration of Pb2+ in the range of 0 and 50 nM (Figure 5D). Determined to be 3σ, the detection limit of the sensor is 4.1 nM. According to the Guidelines for Drinking-water Quality in 2017, the Pb2+ concentration was recommended to be no more than 10 ppb or 48 nM (World Health Organization, 2017). Therefore, this study elucidated a great potential of the developed FRET-based assay for in situ detection of Pb2+ in practice. The effect of Pb2+ on upconversion fluorescence was demonstrated by adding different concentrations of heavy metal ions. There is no significant effect on upconversion fluorescence, even if the Pb2+concentration was increased up to 1,000 nM (Figure 6A).
Figure 5. (A) The restoration of fluorescence after incubation at different concentrations of Pb2+ in FRET system. (B) TEM image of FRET array fabricated from UCNPs-DNA1 and AuNPs-DNA2 after Pb2+ incorporation. (C) The relationship between relative fluorescence intensity (F–F0)/F0 and Pb2+ concentration. (D) Linear correlation between relative fluorescence intensity (F–F0)/F0 and Pb2+ concentration in the range of 0–50 nM.
Figure 6. (A) The fluorescence intensity of FRET array after incubation at different concentrations of Pb2+. (B) The fluorescence intensity of detection sensor after the incorporation of Pb2+, K+, Na+, Ca2+, Mg2+, Hg2+, Cu2+, Zn2+, and Fe3+.
The selectivity of the sensor for the detection of Pb2+ was also tested with different metal ions (Figure 6B, Figure S1). Pb2+ ions led to a dramatic fluorescence recovery (177.9%), while metal ions including K+, Na+, Ca2+, Mg2+, Hg2+, Cu2+, Zn2+, and Fe3+ presented little restoration in the fluorescence spectrum. The result demonstrated the high selectivity of the FRET system for Pb2+ detection.
In summary, a highly sensitive Pb2+ detection sensor was constructed based on FRET between UCNPs as donors and Au NPs as receptors. The hydrophobic surface ligands of NaYF4: 18% Yb, 2% Er @NaYF4 were removed by hydrochloric acid, resulting in enhanced water-dispersible UCNPs which were further modified with DNA1. Au NPs prepared and modified with DNA2, which was partially complementary with DNA1. The FRET assay was fabricated by hybridizing two complementary DNA strands; thus, the green fluorescence of UCNPs was quenched. The specific Pb2+ detection was due to the formation of G-quadruplexes derived from the preferred binding between aptamers of UCNPs and Pb2+, leading to unwound DNA for the recovery of fluorescence. There was a distinct linear correlation between the relative fluorescence intensity and the concentration of Pb2+ from 0 to 50 nM. The developed sensor also presented superior sensitivity (4.1 nM) and selectivity, indicating a promising perspective for Pb2+ detection.
All datasets generated for this study are included in the article/Supplementary Material.
YW: formal analysis, investigation. ML: validation. ZC: software. ZD: data analysis, overall planning, and revision of the manuscript. NL: methodology. JF: conceptualization and methodology. WZ: supervision. All authors wrote and reviewed the manuscript.
This work was supported by the National Natural Science Foundation of China (51878472), and the State Key Laboratory of Pollution Control and Resource Reuse Foundation (No. PCRRK 18001).
The authors declare that the research was conducted in the absence of any commercial or financial relationships that could be construed as a potential conflict of interest.
The Supplementary Material for this article can be found online at: https://www.frontiersin.org/articles/10.3389/fchem.2020.00238/full#supplementary-material
Bogdan, N., Vetrone, F., Ozin, G. A., and Capobianco, J. A. (2011). Synthesis of ligand-free colloidally stable water dispersible brightly luminescent lanthanide-doped upconverting nanoparticles. Nano Lett. 11, 835–840. doi: 10.1021/nl1041929
Bravo-Sanchez, L. R., De La Riva, B. S., Costa-Fernandez, J. M., Pereiro, R., and Sanz-Medel, A. (2001). Determination of lead and mercury in sea water by preconcentration in a flow injection system followed by atomic absorption spectrometry detection. Talanta 55, 1071–1078. doi: 10.1016/S0039-9140(01)00523-9
Chang, H., Tang, L., Wang, Y., Jiang, J., and Li, J. (2010). Graphene fluorescence resonance energy transfer aptasensor for the thrombin detection. Anal. Chem. 82, 2341–2346. doi: 10.1021/ac9025384
Chen, G., Qju, H., Prasad, P. N., and Chen, X. (2014). Upconversion nanoparticles: design, nanochemistry, and applications in theranostics. Chem. Rev. 114, 5161–5214. doi: 10.1021/cr400425h
Chen, G., Song, F., Xiong, X., and Peng, X. (2013). Fluorescent nanosensors based on Fluorescence Resonance Energy Transfer (FRET). Industr. Eng. Chem. Res. 52, 11228–11245. doi: 10.1021/ie303485n
Chen, J., and Zhao, J. X. (2012). Upconversion nanomaterials: synthesis, mechanism, and applications in sensing. Sensors 12, 2414–2435. doi: 10.3390/s120302414
Chen, Z., Ren, X., Meng, X., Zhang, Y., Chen, D., and Tang, F. (2012). Novel fluorescence method for detection of alpha-L-fucosidase based on CdTe quantum dots. Anal. Chem. 84, 4077–4082. doi: 10.1021/ac300166n
Dacosta, M. V., Doughan, S., Han, Y., and Krull, U. J. (2014). Lanthanide upconversion nanoparticles and applications in bioassays and bioimaging: a review. Anal. Chim. Acta 832, 1–33. doi: 10.1016/j.aca.2014.04.030
Gao, R., Hu, Z., Chang, X., He, Q., Zhang, L., Tu, Z., et al. (2009). Chemically modified activated carbon with 1-acylthiosemicarbazide for selective solid-phase extraction and preconcentration of trace Cu(II), Hg(II) and Pb(II) from water samples. J. Hazard. Mater. 172, 324–329. doi: 10.1016/j.jhazmat.2009.07.014
Haase, M., and Schaefer, H. (2011). Upconverting nanoparticles. Angew. Chem. Int. Edn. 50, 5808–5829. doi: 10.1002/anie.201005159
Hamaguchi, N., Ellington, A., and Stanton, M. (2001). Aptamer beacons for the direct detection of proteins. Anal. Biochem. 294, 126–131. doi: 10.1006/abio.2001.5169
Kim, H. N., Ren, W. X., Kim, J. S., and Yoon, J. (2012). Fluorescent and colorimetric sensors for detection of lead, cadmium, and mercury ions. Chem. Soc. Rev. 41, 3210–3244. doi: 10.1039/c1cs15245a
Li, X., Wang, R., Zhang, F., and Zhao, D. (2014). Engineering homogeneous doping in single nanoparticle to enhance upconversion efficiency. Nano Lett. 14, 3634–3639. doi: 10.1021/nl501366x
Li, X., Xu, B., Lu, H., Wang, Z., Zhang, J., Zhang, Y., et al. (2013). Label-free fluorescence turn-on detection of Pb2+ based on AIE-active quaternary ammonium salt of 9,10-distyrylanthracene. Anal. Methods 5, 438–441. doi: 10.1039/c2ay26202a
Li, Z., and Zhang, Y. (2008). An efficient and user-friendly method for the synthesis of hexagonal-phase NaYF(4):Yb, Er/Tm nanocrystals with controllable shape and upconversion fluorescence. Nanotechnology 19, 345606–345606. doi: 10.1088/0957-4484/19/34/345606
Lin, F., Yin, B., Li, C., Deng, J., Fan, X., Yi, Y., et al. (2013). Fluorescence resonance energy transfer aptasensor for platelet-derived growth factor detection based on upconversion nanoparticles in 30% blood serum. Anal. Methods 5, 699–704. doi: 10.1039/C2AY25519G
Liu, B., Tan, H., and Chen, Y. (2013). Upconversion nanoparticle-based fluorescence resonance energy transfer assay for Cr(III) ions in urine. Anal. Chim. Acta 761, 178–185. doi: 10.1016/j.aca.2012.11.035
Mader, H. S., Kele, P., Saleh, S. M., and Wolfbeis, O. S. (2010). Upconverting luminescent nanoparticles for use in bioconjugation and bioimaging. Curr. Opin. Chem. Biol. 14, 582–596. doi: 10.1016/j.cbpa.2010.08.014
Pavlov, V., Xiao, Y., Shlyahovsky, B., and Willner, I. (2004). Aptamer-functionalized Au nanoparticles for the amplified optical detection of thrombin. J. Am. Chem. Soc. 126, 11768–11769. doi: 10.1021/ja046970u
Pei, H., Li, F., Wan, Y., Wei, M., Liu, H., Su, Y., et al. (2012). Designed diblock oligonucleotide for the synthesis of spatially isolated and highly hybridizable functionalization of DNA-gold nanoparticle nanoconjugates. J. Am. Chem. Soc. 134, 11876–11879. doi: 10.1021/ja304118z
Peng, J., Wang, Y., Wang, J., Zhou, X., and Liu, Z. (2011). A new biosensor for glucose determination in serum based on up-converting fluorescence resonance energy transfer. Biosens. Bioelectr. 28, 414–420. doi: 10.1016/j.bios.2011.07.057
Saha, K., Agasti, S. S., Kim, C., Li, X., and Rotello, V. M. (2012). Gold nanoparticles in chemical and biological sensing. Chem. Rev. 112, 2739–2779. doi: 10.1021/cr2001178
Wang, L. Y., Yan, R. X., Hao, Z. Y., Wang, L., Zeng, J. H., Bao, J., et al. (2005). Fluorescence resonant energy transfer biosensor based on upconversion-luminescent nanoparticles. Angew. Chem. Int. Edn. 44, 6054–6057. doi: 10.1002/ange.200501907
World Health Organization (2017). Guidelines for Drinking-Water Quality: Fourth Edition, Incorporating The First Addendum. World Health Organization.
Wu, S., Duan, N., Shi, Z., Fang, C., and Wang, Z. (2014). Dual fluorescence resonance energy transfer assay between tunable upconversion nanoparticles and controlled gold nanoparticles for the simultaneous detection of Pb2+ and Hg2+. Talanta 128, 327–336. doi: 10.1016/j.talanta.2014.04.056
Xia, L., Li, X., Wu, Y., Hu, B., and Chen, R. (2008). Ionic liquids based single drop microextraction combined with electrothermal vaporization inductively coupled plasma mass spectrometry for determination of Co, Hg and Pb in biological and environmental samples. Spectrochim. Acta B 63, 1290–1296. doi: 10.1016/j.sab.2008.09.018
Xiao, Y., Lubin, A. A., Heeger, A. J., and Plaxco, K. W. (2005). Label-free electronic detection of thrombin in blood serum by using an aptamer-based sensor. Angew. Chem. Int. Edn. 44, 5456–5459. doi: 10.1002/ange.200500989
Yang, G., Zhang, C. M., Hu, Q. F., and Yin, J. Y. (2003). Simultaneous determination of four heavy metal ions in tobacco and tobacco additive by online enrichment followed by RP-HPLC and microwave digestion. J. Chromatogr. Sci. 41, 195–199. doi: 10.1093/chromsci/41.4.195
Yoosaf, K., Ipe, B. I., Suresh, C. H., and Thomas, K. G. (2007). In situ synthesis of metal nanoparticles and selective naked-eye detection of lead ions from aqueous media. J. Phys. Chem. C 111, 12839–12847. doi: 10.1021/jp073923q
Zhan, S., Wu, Y., Liu, L., Xing, H., He, L., Zhan, X., et al. (2013). A simple fluorescent assay for lead(II) detection based on lead(II)-stabilized G-quadruplex formation. Rsc Adv. 3, 16962–16966. doi: 10.1039/C3RA42621A
Zheng, X., Yao, T., Zhu, Y., and Shi, S. (2015). Cu2+ modulated silver nanoclusters as an on-off-on fluorescence probe for the selective detection of L-histidine. Biosens. Bioelectr. 66, 103–108. doi: 10.1016/j.bios.2014.11.013
Keywords: upconversion nanoparticles, gold nanoparticles, fluorescence resonance energy transfer, DNA, lead ions
Citation: Wang Y, Lv M, Chen Z, Deng Z, Liu N, Fan J and Zhang W (2020) A Fluorescence Resonance Energy Transfer Probe Based on DNA-Modified Upconversion and Gold Nanoparticles for Detection of Lead Ions. Front. Chem. 8:238. doi: 10.3389/fchem.2020.00238
Received: 06 January 2020; Accepted: 12 March 2020;
Published: 21 April 2020.
Edited by:
Xiaomin Li, Fudan University, ChinaReviewed by:
Peiyuan Wang, Fujian Institute of Research on the Structure of Matter (CAS), ChinaCopyright © 2020 Wang, Lv, Chen, Deng, Liu, Fan and Zhang. This is an open-access article distributed under the terms of the Creative Commons Attribution License (CC BY). The use, distribution or reproduction in other forums is permitted, provided the original author(s) and the copyright owner(s) are credited and that the original publication in this journal is cited, in accordance with accepted academic practice. No use, distribution or reproduction is permitted which does not comply with these terms.
*Correspondence: Ningtao Liu, bmluZ3RfbGl1QDE2My5jb20=; Jianwei Fan, ZmFuamlhbndlaUB0b25namkuZWR1LmNu
Disclaimer: All claims expressed in this article are solely those of the authors and do not necessarily represent those of their affiliated organizations, or those of the publisher, the editors and the reviewers. Any product that may be evaluated in this article or claim that may be made by its manufacturer is not guaranteed or endorsed by the publisher.
Research integrity at Frontiers
Learn more about the work of our research integrity team to safeguard the quality of each article we publish.