- 1School of Chemical Engineering & Technology, China University of Mining and Technology, Xuzhou, China
- 2Jiangsu Key Laboratory of Green Synthetic Chemistry for Functional Materials, School of Chemistry & Materials Science, Jiangsu Normal University, Xuzhou, China
- 3Collaborative Innovation Center of Chemistry for Life Sciences, Institute of Chemistry and BioMedical Sciences, Nanjing University, Nanjing, China
- 4Department of Chemistry and Biochemistry, Texas Tech University, Lubbock, TX, United States
A new Cu(II)-catalyzed annulation–cyanotrifluoromethylation of 1,6-enynes with Togni's reagent and trimethylsilyl cyanide (TMSCN) has been established, enabling the direct construction of trifluoromethylated 1-indanones with an all-carbon quaternary center in good yields. This reaction was performed by using low-cost Cu(OTf)2 as the catalyst and Togni's reagent as both the radical initiator and a CF3 source, providing an efficient protocol for building up an 1-indanone framework with wide functional group compatibility. The reaction mechanism was proposed through a radical triggered addition/5-exo-dig cyclization/oxidation/nucleophilic cascade.

Graphical Abstract. A new Cu(II)-catalyzed annulation-cyanotrifluoromethylation of 1,6-enynes with Togni's reagent and TMSCN has been established, enabling the direct construction of trifluoromethylated 1-indanones with an all-carbon quaternary center in good yields. This reaction was performed by using low-cost Cu(OTf)2 as the catalyst and Togni's reagent as both the radical initiator and a CF3 source, providing an efficieA new Cu(II)-catalyzed annulation-cyanotrifluoromethylation of 1,6-enynes with Togni's reagent and TMSCN has been established, enabling the direct construction of trifluoromethylated 1-indanones with an all-carbon quaternary center in good yields. This reaction was performed by using low-cost Cu(OTf)2 as the catalyst and Togni's reagent as both the radical initiator and a CF3 source, providing an efficient protocol for building up 1-indanone framework with wide functional group compatibility. The reaction mechanism was proposed through a radical triggered addition/5-exo-dig cyclization/oxidation/nucleophilic cascade. nt protocol for building up 1-indanone framework with wide functional group compatibility. The reaction mechanism was proposed through a radical triggered addition/5-exo-dig cyclization/oxidation/nucleophilic cascade.
Introduction
Trifluoromethylation of organic molecular skeletons has attracted considerable attention in pharmaceutical chemistry, agrochemicals, and functional materials, owing to the fact that incorporation of the trifluoromethyl group into organic molecules can modulate their abilities including lipophilicity, bioavailability, and metabolic stability (Umemoto, 1996; Müller et al., 2007; Hagmann, 2008; Studer, 2012; Yang et al., 2015). Therefore, many efforts have been done in the past few decades, which mainly depended on transition-metal-catalyzed trifluoromethylation reactions. Such reactions enable direct construction of the C–CF3 bond in an atom-economic manner and provide efficient and practical methods for the collection of trifluoromethyl-containing compounds, such as catalytic trifluoromethylation of alkane (Pan et al., 2011; Fu et al., 2012; Kuninobu et al., 2015; Wang et al., 2015; Xiao et al., 2019), alkenes (Chu and Qing, 2012; Shimizu et al., 2012; Zhu and Buchwald, 2013; Lin et al., 2016; He et al., 2018), and alkynes (Ge et al., 2014; Iqbal et al., 2014; Tomita et al., 2015; Wu et al., 2017; Huang et al., 2018). Among them, a vast majority of reports focused on the difunctionalization of alkenes or enynes (He et al., 2014a,b), such as hydrotrifluoromethylation (Wilger et al., 2013; Wu et al., 2013), carbotrifluoromethylation (Chen et al., 2013; Egami et al., 2013; Liu et al., 2013), and oxytrifluoromethylation (Egami et al., 2012; Janson et al., 2012; Li and Studer, 2012; Zhu and Buchwald, 2012) for their high utilization by incorporating trifluoromethyl groups into target molecules across the unsaturated π system. On the other hand, 1-indanones are privileged structural motifs commonly present in many bioactive molecules and natural products such as Pterosin B and C (Nagle et al., 2000; Wessig and Teubner, 2006), pauciflorol F (Dai et al., 1998; Nitta et al., 2002; Ito et al., 2004), and (+)-indacrinone (DeSolms et al., 1978) (Figure 1). Consequently, many chemists made their contributions to establish numerous elegant protocols for their synthesis including Friedel–Crafts acylation (Koelsch, 1932; Frank et al., 1944), Grignard reactions (Bergmann, 1956; Manning et al., 1981), and transition metal-catalyzed annulation of arylalkynes (Shintani et al., 2007; Chernyak et al., 2011; He et al., 2018; Song et al., 2019), radical addition–cyclization of 1,6-enynes (Shen et al., 2018a,b, 2019), and other methods (Zhu et al., 2017, 2018a,b; Shi et al., 2019a). To the best of our knowledge, introduction of a trifluoromethyl group into the 1-indanone framework via a radical-triggered annulation–difunctionalization strategy remains elusive.
Multicomponent reactions (MCRs) represent an attractive and powerful tool for building complex molecular architectures under usually mild conditions (Hao et al., 2016; Wang et al., 2016a,b; Ji et al., 2019; Liu et al., 2019; Qin et al., 2019; Shi et al., 2019b). Radical-triggered annulation–difunctionalization cascades, standing at the intersection of both radical and multicomponent transformations, constitute a unique reaction category, which enables direct assembly of difunctionalized cyclic systems containing both isocyclic and heterocyclic skeletons which are not available from other methods. As a result, lots of unsaturated compounds endowed with alkene and/or alkyne units are devised and prepared as radical acceptors to capture the various radical species (Chen et al., 2008; Liu et al., 2014; Kong et al., 2015; Wang F. et al., 2016; Zhang et al., 2019). Generally, the success of the radical annulation–difunctionalization relies on the radical continuous transfer across the unsaturated systems through a synergistic orientation process. Over the years, our group has been heavily involved in the development of new annulation–difunctionalization cascades for multiple ring formations. For example, we reported a copper-catalyzed annulation–halofluoroalkylation of 1,6-enynes, leading to the atom-economic and highly stereoselective protocol toward functionalized 1-indenones (Scheme 1, path a) (Shen et al., 2019). To continue our interest in this project, we approach a radical addition–cyclization strategy to install both trifluoromethyl and cyano moieties into the 1-indenone framework, due to the behaviors of trifluoromethyl and cyano groups in the wide application potentiality in assigning and discovering new biological lead compounds. An extensive literature survey revealed that the radical-triggered annulation–cyanotrifluoromethylation of 1,6-enynes toward 1-indanones remains unreported to date. For this reason, the copper-catalyzed annulation–cyanotrifluoromethylation of 1,6-enynes 1 with Togni's reagent 2a and trimethylsilyl cyanide (TMSCN) was carried out by 1,10-phenanthroline (phen) as the ligand, enabling a radical-induced three-component cascade to access trifluoromethylated 1-indanones 3 with generally good yields (Scheme 1, path b). Remarkably, some cases showed complete stereoselectivity, and only E-selectivity was observed. Herein, we report this copper-catalyzed radical transformation.
Results and Discussion
At the outset of our studies, we chose the preformed 1,6-enyne 1a, Togni's reagent 2a, and TMSCN as the model substrate (Table 1). To our delight, the reaction of 1a with 2a and TMSCN in a 1:2:2 mol ratio catalyzed by 10 mol% Cu(OAc)2 proceeded smoothly in acetonitrile at 50°C by using 1,10-phenanthroline (phen, L1) as a ligand, and the target product 3a as a sole (E)-stereoisomer was obtained in 36% yield. The following screening of solvents showed that the use of DMSO and DMF led to a slightly decreased yield of 3a compared with acetonitrile (entries 2 and 3 vs. entry 1), whereas both 1,4-dioxane and THF completely suppressed the formation of 3a (entries 4 and 5). Thus, acetonitrile was the best solvent for the reaction. An employment of NaOAc as the base facilitated the reaction process, delivering 40% yield of the desired product 3a (entry 6). After that, we conducted the screening of a variety of copper salts, such as Cu(CH3CN)4PF6, CuCN, CuI, and Cu(OTf)2, that are often utilized in catalytic transformations, for this addition–cyclization cascade by using acetonitrile as the reaction medium. All these catalysts could promote the conversion of 1a into 3a (entries 7–10), and the latter one showed the best catalytic performance in the current reaction, generating product 3a in 55% yield (entry 10). As the next optimization step, several ligands, such as 1,10-phenanthroline-5,6-dione (L2), 2,2′-bipyridine (L3), and 2,2′:6′,2″-terpyridine (L4), were investigated and anticipated to enhance the yield of product 3a. Disappointingly, ligands L2-L4 showed slightly weaker performance on the conversion of 1a into 3a as compared with L1 (entries 11–13). Using Togni's reagent 2b to replace 2a resulted in a lower conversion (42%, entry 14 vs. entry 10). Different bases such as potassium phosphate tribasic (K3PO4), trimethylamine (Et3N), and cesium carbonate (Cs2CO3) were then screened. The results indicated that K3PO4 could improve the reaction, providing product 3a in 64% yield. After careful optimizations, we found that fine-tuning the substrate ratio 1a/2a/TMSCN to 1:3:2 delivered product 3a in a higher 87% yield (entry 18).
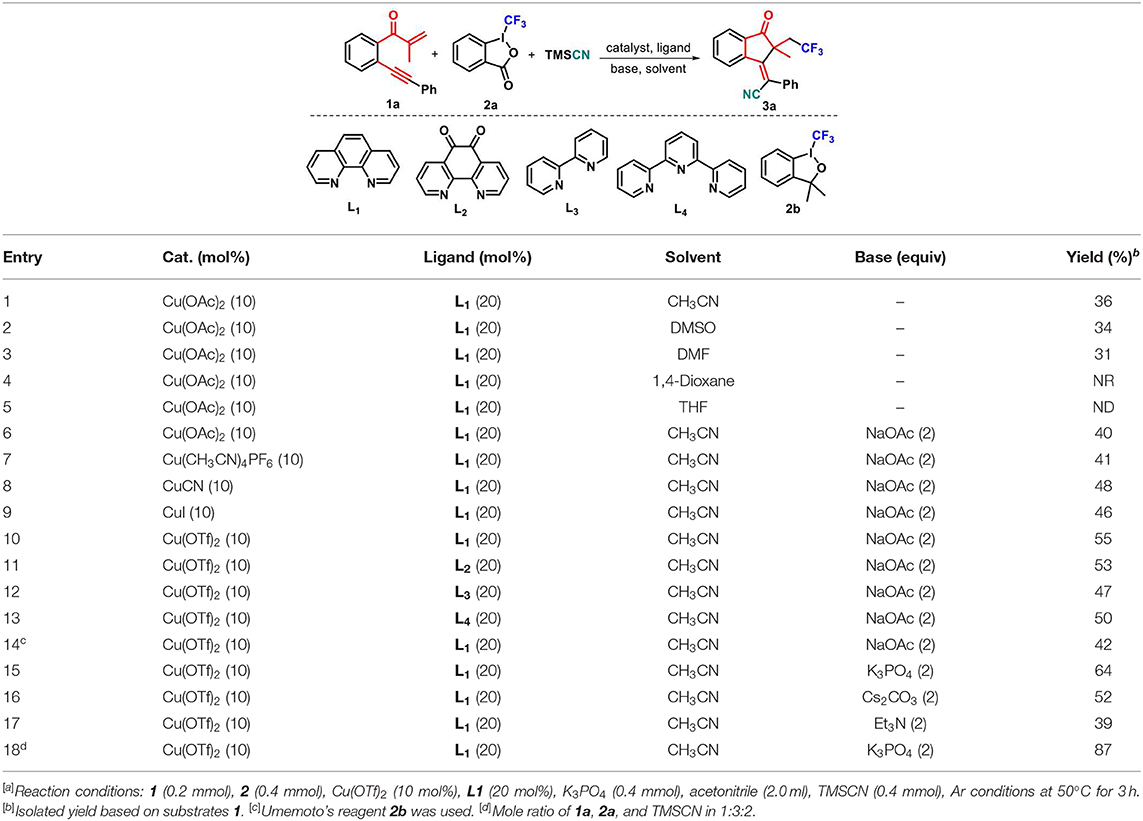
Table 1. Optimization of reaction conditions[a].
With the optimized conditions in hand (Table 1, entry 18), the substrate scope of this radical-triggered annulation–cyanotrifluoromethylation of 1,6-enynes was investigated. The results were presented in Scheme 2. Upon repeating the reaction with 2a and TMSCN, substrate 1 with diverse substituents such as fluoro (1b), chloro (1c and 1d), and bromo (1e) groups on the arylalkynyl moiety all work well, giving the corresponding functionalized (E)-1-indanones 3b–3e in 45–78% yields. Notably, substrates 1c–1e could completely orient the E-selectivity to the target products 3c–3e. Alternatively, both cyclopropyl 1f and n-butyl 1g counterparts were proven to be favorable, enabling radical-induced cyclization reactions to offer the corresponding (E)-1-indanones 3f and 3g with complete stereoselectivities, albeit with low yields. Due to the pharmacological significance of fluorine-containing molecules compared to their non-fluorinated analogs, we decided to prepare 1,6-enynes 1h–1j containing the fluoro group residing in the 5-position of the internal arene ring and employed them to react with 2a and TMSCN. The reaction worked well, accessing the corresponding polyfluoro products 3h–3j in 42–64% yields and 5:3 to 5:2 E/Z ratios. Other substituents including chloro (1k–1o, 1r, and 1s), methyl (1p and 1t–1x), and methoxy (1q) located at the C4- or C5-position on the internal arene ring did not hamper this copper-catalyzed reaction, and a range of new substituted 1-indanones 3k–3x can be isolated in synthetically useful yields, in which a complete diastereoselectivity was also observed in the cases of 3k, 3l, 3p, 3q, 3r, and 3t. However, unsatisfactory E/Z ratios were detected for the other products. Either electronically neutral (H), poor (fluoro, chloro, and bromo), or rich [methyl, ethyl, t-butyl, and methoxy (PMP = p-methoxyphenyl)] groups at the para-position of the arylalkynyl moiety (R1) are well-tolerated with the catalytic conditions. Unfortunately, 1,6-enyne 1y carrying a 2-thienyl group was an ineffective reaction partner in this transformation. The structures of these resulting 1-indanones were fully characterized by NMR spectroscopy and HRMS data (Data Sheet 1).
To gain mechanistic insight into this transformation, radical trapping experiments were performed. When 2,2,6,6-tetramethylpiperidine-1-oxyl (TEMPO) as a radical scavenger was subjected to the reaction conditions, the generation of 3a was completely suppressed (Scheme 3a). Similarly, BHT could inhibit the formation of 3a. These results showed that the reaction may include a radical process. Moreover, the developed transformation could be valorized through post-functionalization of indanone 3q (Scheme 3b). The combination of NaBH4 and I2 was found to be effective to reduce 3q to give 2,3-dihydro-1H-inden-1-ol 4 (51% yield) (He et al., 2015; Chen et al., 2018).
Mechanism
According to these results and related literature (Kamigata et al., 1990; Liu et al., 2012; Pair et al., 2013; Yasu et al., 2013; He et al., 2014b; Shen et al., 2019), a plausible mechanism was proposed (Scheme 4). The copper(II) catalyst activates Togni's reagent 2a to give the activated complex A, which releases a Cu(III) species and the trifluoromethyl radical. The latter rapidly adds to 1,6-enyne 1a to give the radical intermediate B. In the presence of ligands and TMSCN, Cu(III) species activates an alkyne unit of intermediate B to drive 5-exo-dig cyclization, giving favorable anti-Cu(III) species C (Shen et al., 2019), some of which is converted into syn-Cu(III) species C′, together with trimethylsilyl 2-iodobenzoate D. Finally, anti-Cu(III) species C undergoes reductive elimination to give the desired (E)-product 3a as a major isomer and regenerate a Cu(II) complex to complete a catalytic cycle through the release of ligands (He et al., 2014b), whereas syn-Cu(III) species C′ undergoes the same reductive elimination to access minor (Z)-product 3a.
Conclusion
In summary, we have established a copper-catalyzed annulation–cyanotrifluoromethylation of 1,6-enynes with easily available Togni's reagent and TMSCN, by which a wide range of 1-indanones with a quaternary carbon center were stereoselectively synthesized in generally good yields. Notably, a complete stereoselectivity could be detected in most cases. This approach is efficiently induced by Togni's reagent as a radical donor and ultimately terminated by TMSCN as the nucleophilic reagent. The transformation offered a new entry to prepare the CF3-containing 1-indanone skeleton via a complex radical addition–cyclization cascade. Further investigations into the mechanism and its application will be conducted in due course.
Materials and Methods
General
1H NMR (13C NMR, 19F NMR) spectra were measured on a Bruker DPX 400-MHz spectrometer in CDCl3 (DMSO-d6) with chemical shift (δ) given in ppm relative to TMS as internal standard [(s = singlet, d = doublet, t = triplet, brs = broad singlet, m = multiplet), coupling constant (Hz)]. HRMS (ESI) was done by using a micrOTOF-Q II HRMS/MS instrument (Bruker).
General Procedure for the Synthesis of 3
Example for the Synthesis of 3a
Under Ar conditions, a mixture of Togni's reagent 2 (0.6 mmol), Cu(OTf)2 (0.02 mmol), K3PO4 (0.4 mmol), and ligand L1 (0.04 mmol) was added in a Schlenk tube. Acetonitrile was added into the tube. Then, 2-methyl-1-[2-(phenylethynyl)phenyl]prop-2-en-1-one 1a (0.2 mmol) and TMSCN (0.4 mmol) were put in the system, stirred for 3 h at 50°C until thin-layer chromatography (TLC) revealed that conversion of the starting material 1a was complete. Next, the reaction mixture was concentrated in vacuum, and the resulting residue was purified by silica gel column chromatography (petroleum ether/ethyl acetate = 25:1, v/v) to afford the desired product 3a.
General Procedure for the Synthesis of 4
Under Ar conditions, 3q (0.05 mmol), NaBH4 (3.0 equiv), and I2 (1.0 equiv) were added in a Schlenk tube. THF was added, and the reaction mixture was stirred at room temperature for 10 h. The solution was treated with water and extracted with dichloromethane. The combined organic layers were washed with brine, dried over Na2SO4, concentrated in vacuum, and purified by preparative TLC (petroleum ether/ethyl acetate = 2/1) to afford product 4 (He et al., 2015; Chen et al., 2018).
(E)-2-[2-Methyl-3-oxo-2-(2,2,2-trifluoroethyl)-2,3-dihydro-1H-inden-1-ylidene]-2-phenylacetonitrile (3a)
Light yellow solid, 59 mg, 87% yield; mp 105.2–106.1°C. 1H NMR (400 MHz, CDCl3; δ, ppm): 8.96 (d, J = 8.0 Hz, 1H), 7.94 (d, J = 7.6 Hz, 1H), 7.90–7.85 (m, 1H), 7.73–7.67 (m, 1H), 7.54–7.49 (m, 3H), 7.46–7.41 (m, 2H), 2.66–2.53 (m, 1H), 2.25–2.12 (m, 1H), 1.19 (s, 3H). 13C NMR (100 MHz, CDCl3; δ, ppm): 202.3, 153.2, 144.5, 136.3, 135.5, 133.0, 132.4, 129.9, 129.5, 129.2, 125.0 (q, J = 85.1 Hz, CF3), 118.9, 109.1, 49.8, 40.4 (q, J = 27.6 Hz, CH2CF3), 25.1. 19F NMR (376 MHz, CDCl3; δ, ppm): −61.29 (s, 3F). IR (KBr, ν, cm−1): 2,200, 1,721, 1,577, 1,447, 1,361, 1,256, 1,138, 967, 775. HRMS (ESI, m/z): calcd for C20H14F3NONa [M + Na]+ 364.0919, found 364.0928.
(E)-2-(4-Fluorophenyl)-2-[2-methyl-3-oxo-2-(2,2,2-trifluoroethyl)-2,3-dihydro-1H-inden-1-ylidene]acetonitrile (3b, Major)
Light yellow solid, 56 mg, 78% yield; mp 148.9–150.9°C. 1H NMR (400 MHz, CDCl3; δ, ppm): 8.94 (d, J = 8.0 Hz, 1H), 7.94 (d, J = 7.6 Hz, 1H), 7.86 (d, J = 8.0 Hz, 1H), 7.73–7.67 (m, 1H), 7.46–7.42 (m, 2H), 7.27–7.22 (m, 2H), 2.67–2.58 (m, 1H), 2.18–2.09 (m, 1H), 1.20 (s, 3H). 13C NMR (100 MHz, CDCl3; δ, ppm): 202.0, 163.3 (d, 1J = 247.0 Hz, CF), 153.9, 144.3, 136.3, 135.3, 132.6, 131.6 (d, 3J = 8.3 Hz, CF), 128.9 (d, 4J = 3.7 Hz, CF), 126.6, 125.0 (q, J = 80.7 Hz, CF3), 117.4, 117.2, 116.4 (d, 2J = 21.8 Hz, CF), 108.0, 49.8, 40.3 (q, J = 27.8 Hz, CH2CF3), 25.2. 19F NMR (376 MHz, CDCl3; δ, ppm): −61.31 (s, 3F), −109.82 (s, 1F). IR (KBr, ν, cm−1): 2,202, 1,724, 1,599, 1,509, 1,361, 1,257, 1,142, 1,070, 776. HRMS (ESI, m/z): calcd for C20H13F4NONa [M + Na]+ 382.0825, found 382.0784.
(E)-2-(3-Chlorophenyl)-2-[2-Methyl-3-oxo-2-(2,2,2-Trifluoroethyl)-2,3-Dihydro-1H-Inden-1-Ylidene]Acetonitril (3c)
Light yellow solid, 34 mg, 45% yield; mp 174.6–177.1°C. 1H NMR (400 MHz, CDCl3; δ, ppm): 8.94 (d, J = 8.0 Hz, 1H), 7.96 (d, J = 7.6 Hz, 1H), 7.91–7.86 (m, 1H), 7.75–7.69 (m, 1H), 7.52–7.42 (m, 3H), 7.34 (d, J = 7.2 Hz, 1H), 2.69–2.61 (m, 1H), 2.21–2.12 (m, 1H), 1.21 (s, 3H). 13C NMR (100 MHz, CDCl3; δ, ppm): 201.9, 154.0, 144.2, 136.4, 135.6, 135.2, 134.6, 132.7, 130.5. 130.2, 129.8, 127.7, 125.1 (q, J = 78.5 Hz, CF3), 118.5, 107.6, 49.8, 40.2 (q, J = 55.5 Hz, CH2CF3), 25.2. 19F NMR (376 MHz, CDCl3; δ, ppm): −61.31 (s, 3F). IR (KBr, ν, cm−1): 2,204, 1,728, 1,595, 1,336, 1,260, 1,140, 1,069, 776. HRMS (ESI, m/z): calcd for C20H13ClF3NONa [M + Na]+ 398.0529, found 398.0520.
(E)-2-(4-Chlorophenyl)-2-[2-Methyl-3-oxo-2-(2,2,2-Trifluoroethyl)-2,3-Dihydro-1H-Inden-1-ylidene]Acetonitrile (3d)
Light yellow solid, 53 mg, 70% yield; mp 154.5–155.3°C. 1H NMR (400 MHz, CDCl3; δ, ppm): 8.94 (d, J = 8.4 Hz, 1H), 7.95 (d, J = 7.6 Hz, 1H), 7.91–7.85 (m, 1H), 7.75–7.69 (m, 1H), 7.50 (d, J = 8.4 Hz, 2H), 7.39 (d, J = 8.4 Hz, 2H), 2.69–2.59 (m, 1H), 2.20–2.11 (m, 1H), 1.20 (s, 3H). 13C NMR (100 MHz, CDCl3; δ, ppm): 201.9, 153.9, 144.2, 136.4, 136.3, 135.6, 132.7, 131.4, 131.0, 129.6, 125.1 (q, J = 78.5 Hz, CF3), 118.6, 107.8, 49.8, 40.7 (q, J = 27.7 Hz, CH2CF3), 25.2. 19F NMR (376 MHz, CDCl3; δ, ppm): −61.28 (s, 3F). IR (KBr, ν, cm−1): 2,204, 1,729, 1,593, 1,491, 1,360, 1,256, 1,143, 1,072, 835, 776. HRMS (ESI, m/z): calcd for C20H13ClF3NONa [M + Na]+ 398.0529, found 398.0569.
(E)-2-(4-Bromophenyl)-2-[2-Methyl-3-oxo-2-(2,2,2-Trifluoroethyl)-2,3-dihydro-1H-inden-1-ylidene]Acetonitrile (3e)
Light yellow solid, 53 mg, 63% yield; mp 103.9–104.7°C. 1H NMR (400 MHz, CDCl3; δ, ppm): 8.93 (d, J = 8.0 Hz, 1H), 7.95 (d, J = 7.6 Hz, 1H), 7.91–7.85 (m, 1H), 7.74–7.68 (m, 1H), 7.66 (d, J = 8.4 Hz, 2H), 7.32 (d, J = 8.4 Hz, 2H), 2.71–2.59 (m, 1H), 2.21–2.11 (m, 1H), 1.20 (s, 3H). 13C NMR (100 MHz, CDCl3; δ, ppm): 201.9, 153.9, 144.2, 136.4, 135.6, 132.7, 132.5, 131.9, 131.2, 125.1 (q, J = 77.7 Hz, CF3), 124.5, 118.5, 107.8, 49.8, 40.4 (q, J = 27.8 Hz, CH2CF3), 25.2. 19F NMR (376 MHz, CDCl3; δ, ppm): −61.27 (s, 3F). IR (KBr, ν, cm−1): 2,205, 1,728, 1,585, 1,486, 1,360, 1,255, 1,142, 1,069, 1,011, 968, 832, 723. HRMS (ESI, m/z): calcd for C20H13BrF3NONa [M + Na]+ 442.0024, found 442.0020.
(E)-2-Cyclopropyl-2-[2-Methyl-3-oxo-2-(2,2,2-trifluoroethyl)-2,3-dihydro-1H-inden-1-ylidene]Acetonitrile (3f)
Light yellow oil, 9 mg, 14% yield. 1H NMR (400 MHz, CDCl3; δ, ppm): 7.82 (d, J = 7.6 Hz, 1H), 7.72–7.61 (m, 2H), 7.46–7.39 (m, 1H), 5.99–5.89 (m, 1H), 2.86–2.77 (m, 1H), 2.65–2.58 (m, 1H), 2.58–2.46 (m, 4H), 1.33 (s, 3H). 13C NMR (100 MHz, CDCl3; δ, ppm): 203.5, 146.2, 135.9, 129.2, 124.4 (q, J = 28.8 Hz, CF3), 118.9, 110.0, 97.0, 48.1, 40.0 (q, J = 28.1 Hz, CH2CF3), 25.3, 25.2, 17.1. 19F NMR (376 MHz, CDCl3; δ, ppm): −61.78 (s, 3F). IR (KBr, ν, cm−1): 2,248, 1,964, 1,719, 1,602, 1,471, 1,362, 1,261, 1,142, 1,069, 799. HRMS (ESI, m/z): calcd for C17H14F3NONa [M + Na]+ 328.0920, found 328.0856.
(E)-2-[2-Methyl-3-oxo-2-(2,2,2-Trifluoroethyl)-2,3-dihydro-1H-inden-1-ylidene]hexanenitrile (3g)
Light yellow oil, 19 mg, 30% yield. 1H NMR (400 MHz, CDCl3; δ, ppm): 8.88 (d, J = 8.0 Hz, 1H), 7.90 (d, J = 7.6 Hz, 1H), 7.83–7.77 (m, 1H), 7.64–7.59 (m, 1H), 3.08–2.99 (m, 1H), 2.82–2.72 (m, 1H), 2.57–2.48 (m, 2H), 1.83–1.69 (m, 2H), 1.49–1.45 (m, 2H), 1.41 (s, 3H), 1.01 (t, J = 7.2 Hz, 3H). 13C NMR (100 MHz, CDCl3; δ, ppm): 202.7, 149.9, 144.8, 136.2, 134.8, 131.6, 124.8 (q, J = 49.1 Hz, CF3), 118.8, 110.3, 49.2, 40.3 (q, J = 25.2 Hz, CH2CF3), 31.8, 30.0, 23.7, 22.6, 13.9. 19F NMR (376 MHz, CDCl3; δ, ppm): −62.09 (s, 3F). IR (KBr, ν, cm−1): 2,210, 1,731, 1,596, 1,469, 1,365, 1,257, 1,142, 1,070, 777. HRMS (ESI, m/z): calcd for C18H18F3NONa [M + Na]+ 344.1213, found 344.1197.
(E)-2-[5-Fluoro-2-methyl-3-oxo-2-(2,2,2-trifluoroethyl)-2,3-dihydro-1H-inden-1-ylidene]-2-phenylacetonitrile (3h, Major)
Light yellow solid, 30 mg, 42% yield; mp 107.0–109.0°C. 1H NMR (400 MHz, CDCl3; δ, ppm): 9.00–8.94 (m, 1H), 7.59–7.55 (m, 2H), 7.51–7.48 (m, 3H), 7.44–7.42 (m, 2H), 2.62–2.53 (m, 1H), 2.24–2.15 (m, 1H), 1.19 (s, 3H). 13C NMR (100 MHz, CDCl3; δ, ppm): 201.3, 165.0 (d, 1J = 256.3 Hz, CF), 152.1, 140.6 (d, 5J = 2.5 Hz, CF), 132.7, 130.1, 129.2, 127.8 (d, 4J = 8.6 Hz, CF), 126.3, 123.5, 123.3 (d, 2J = 26.8 Hz, CF), 118.9, 110.6 (d, 3J = 22.2 Hz, CF), 50.4, 40.5 (q, J = 27.8 Hz, CH2CF3), 25.1. 19F NMR (376 MHz, CDCl3; δ, ppm): −61.30 (s, 3F), −104.65 (s, 1F). IR (KBr, ν, cm−1): 2,205, 1,732, 1,600, 1,488, 1,362, 1,257, 1,186, 1,141, 1,067, 949, 833. HRMS (ESI, m/z): calcd for C20H13F4NONa [M + Na]+ 382.0825, found 382.0832.
(E)-2[(5-Fluoro-2-methyl-3-oxo-2-(2,2,2-trifluoroethyl)-2,3-dihydro-1H-inden-1-ylidene]-2-(p-tolyl)acetonitrile (3i, Major)
Light yellow solid, 41 mg, 55% yield; mp 1,041–105.8°C. 1H NMR (400 MHz, CDCl3; δ, ppm): 8.98–8.93 (m, 1H), 7.58–7.52 (m, 2H), 7.35–7.30 (m, 4H), 2.62–2.52 (m, 1H), 2.43 (s, 3H), 2.27–2.18 (m, 1H), 1.20 (s, 3H). 13C NMR (100 MHz, CDCl3; δ, ppm): 201.5, 164.9 (d, 1J = 256.2 Hz, CF), 152.0, 140.2, 130.8, 129.9, 129.3, 127.7 (d, 4J = 8.6 Hz, CF), 126.3, 123.5, 123.2 (d, 2J = 23.6 Hz, CF), 119.0, 110.5 (d, 3J = 22.2 Hz, CF), 50.4, 40.4 (q, J = 27.7 Hz, CH2CF3), 25.1, 21.4. 19F NMR (376 MHz, CDCl3; δ, ppm): −61.29 (s, 3F), −104.91 (s, 1F). IR (KBr, ν, cm−1): 2,201, 1,729, 1,596, 1,447, 1,361, 1,256, 1,178, 1,138, 1,069, 967, 775, 712. HRMS (ESI, m/z): calcd for C21H15F4NONa [M + Na]+ 396.0982, found 396.0956.
(E)-2-[4-(tert-Butyl)phenyl]-2-[5-fluoro-2-methyl-3-oxo-2-(2,2,2-trifluoroethyl)-2,3-dihydro-1H-inden-1-ylidene]acetonitrile (3j, Major)
Light yellow oil, 53 mg, 64% yield. 1H NMR (400 MHz, CDCl3; δ, ppm): 8.99–8.92 (m, 1H), 7.53–7.48 (m, 3H), 7.36–7.32 (m, 3H), 2.63–2.53 (m, 1H), 2.31–2.18 (m, 1H), 1.37 (s, 9H), 1.20 (s, 3H). 13C NMR (100 MHz, CDCl3; δ, ppm): 201.5, 164.9 (d, 1J = 256.0 Hz, CF), 153.4, 140.8 (d, 5J = 2.6 Hz, CF), 129.7, 129.1, 128.3, 127.7 (d, 4J = 8.5 Hz, CF), 126.1, 123.5, 123.2 (d, 2J = 23.6 Hz, CF), 119.0, 110.5 (d, 3J = 22.2 Hz, CF), 50.4, 40.6 (q, J = 27.7 Hz, CH2CF3), 34.9, 31.3, 25.0. 19F NMR (376 MHz, CDCl3; δ, ppm): −61.26 (s, 3F), −104.94 (s, 1F). IR (KBr, ν, cm−1): 2,206, 1,734, 1,599, 1,487, 1,362, 1,258, 1,187, 1,141, 1,071, 949, 808. HRMS (ESI, m/z): calcd for C24H21F4NONa [M + Na]+ 438.1451, found 438.1458.
(E)-2-[5-Chloro-2-methyl-3-oxo-2-(2,2,2-trifluoroethyl)-2,3-dihydro-1H-inden-1-ylidene]-2-phenylacetonitrile (3k)
Light yellow solid, 36 mg, 48% yield; mp 144.7–146.9°C. 1H NMR (400 MHz, CDCl3; δ, ppm): 8.89 (d, J = 8.8 Hz, 1H), 7.89 (d, J = 2.0 Hz, 1H), 7.84–7.78 (m, 1H), 7.54–7.48 (m, 3H), 7.46–7.38 (m, 2H), 2.63–2.52 (m, 1H), 2.24–2.13 (m, 1H), 1.19 (s, 3H). 13C NMR (100 MHz, CDCl3; δ, ppm): 201.1, 152.1, 142.7, 139.1, 137.0, 136.3, 132.7, 130.1, 129.4, 129.2, 126.7, 124.4, 118.7, 109.6, 50.2, 40.5 (q, J = 27.8 Hz, CH2CF3), 25.1. 19F NMR (376 MHz, CDCl3; δ, ppm): −61.25 (s, 3F). IR (KBr, ν, cm−1): 2,205, 1,726, 1,588, 1,457, 1,419, 1,364, 1,264, 1,179, 1,142, 1,068, 836, 703. HRMS (ESI, m/z): calcd for C20H13ClF3NONa [M + Na]+ 398.0530, found 398.0491.
(E)-2-[5-Chloro-2-methyl-3-oxo-2-(2,2,2-trifluoroethyl)-2,3-dihydro-1H-inden-1-ylidene]-2-(4-fluorophenyl)acetonitrile (3l)
Light yellow solid, 48 mg, 61% yield; mp 195.2–197.2°C. 1H NMR (400 MHz, CDCl3; δ, ppm): 8.88 (d, J = 8.8 Hz, 1H), 7.89 (d, J = 2.0 Hz, 1H), 7.84–7.78 (m, 1H), 7.45–7.38 (m, 2H), 7.25–7.18 (m, 2H), 2.68–2.55 (m, 1H), 2.20–2.08 (m, 1H), 1.20 (s, 3H). 13C NMR (100 MHz, CDCl3; δ, ppm): 200.9, 163.4 (d, 1J = 250.2 Hz, CF), 152.8, 142.4, 139.4, 137.0, 136.4, 131.5 (d, 2J = 8.4 Hz, CF), 128.6 (d, 3J = 3.6 Hz, CF), 126.7, 124.5, 118.6, 116.7, 116.4, 108.5, 50.1, 40.4 (q, J = 27.8 Hz, CH2CF3), 25.1. 19F NMR (376 MHz, CDCl3; δ, ppm): −61.27(s, 3F), −109.49 (s, 1F). IR (KBr, ν, cm−1): 2,209, 1,727, 1,588, 1,507, 1,426, 1,361, 1,263, 1,139, 1,064, 835. HRMS (ESI, m/z): calcd for C20H12ClF4NONa [M + Na]+ 416.0436, found 416.0421.
(E)-2-[5-Chloro-2-methyl-3-oxo-2-(2,2,2-trifluoroethyl)-2,3-dihydro-1H-inden-1-ylidene]-2-(p-tolyl)acetonitrile (3m, Major)
Light yellow solid, 48 mg, 62% yield; mp 121.7–123.6°C. 1H NMR (400 MHz, CDCl3; δ, ppm): 8.88 (d, J = 8.8 Hz, 1H), 7.88 (d, J = 2.0 Hz, 1H), 7.81–7.78 (m, 1H), 7.30 (s, 4H), 2.61–2.52 (m, 1H), 2.43 (s, 3H), 2.26–2.18 (m, 1H), 1.20 (s, 3H). 13C NMR (100 MHz, CDCl3; δ, ppm): 201.3, 152.0, 142.8, 140.2, 139.0, 136.3, 135.4, 130.8, 129.9, 129.3, 126.7, 124.3, 118.8, 109.7, 50.2, 40.4 (q, J = 27.6 Hz, CH2CF3), 25.2, 21.4. 19F NMR (376 MHz, CDCl3; δ, ppm): −61.25 (s, 3F). IR (KBr, ν, cm−1): 2,205, 1,732, 1,589, 1,508, 1,457, 1,361, 1,263, 1,178, 1,144, 1,070, 942, 833. HRMS (ESI, m/z): calcd for C21H15ClF3NONa [M + Na]+ 412.0686, found 412.0657.
(E)-2-[5-Chloro-2-methyl-3-oxo-2-(2,2,2-trifluoroethyl)-2,3-dihydro-1H-inden-1-ylidene]-2-(4-ethylphenyl)acetonitrile (3n, Major)
Light yellow solid, 48 mg, 59% yield; mp 117.8–120.4°C. 1H NMR (400 MHz, CDCl3; δ, ppm): 8.88 (d, J = 8.8 Hz, 1H), 7.88 (d, J = 2.0 Hz, 1H), 7.82–7.78 (m, 1H), 7.32 (s, 4H), 2.75–2.68 (m, 2H), 2.61–2.53 (m, 1H), 2.27–2.18 (m, 1H), 1.30 (t, J = 7.6 Hz, 3H), 1.20 (s, 3H). 13C NMR (100 MHz, CDCl3; δ, ppm): 201.3, 152.0, 146.5, 142.8, 139.0, 136.9, 136.3, 129.6, 129.3, 128.7, 126.7, 124.3, 118.9, 109.8, 50.2, 40.4 (q, J = 27.76 Hz, CH2CF3), 28.7, 25.1, 15.3. 19F NMR (376 MHz, CDCl3; δ, ppm): −61.24 (s, 3F). IR (KBr, ν, cm−1): 2,203, 1,732, 1,587, 1,507, 1,457, 1,362, 1,254, 1,179, 1,145, 1,070, 942, 833. HRMS (ESI, m/z): calcd for C22H17ClF3NONa [M + Na]+ 426.0843, found 426.0824.
(E)-2-[5-Chloro-2-methyl-3-oxo-2-(2,2,2-trifluoroethyl)-2,3-dihydro-1H-inden-1-ylidene]-2-(4-methoxyphenyl)acetonitrile (3o, Major)
Light yellow solid, 56 mg, 69% yield; mp 118.4–120.8°C. 1H NMR (400 MHz, CDCl3; δ, ppm): 8.87 (d, J = 8.8 Hz, 1H), 7.87 (d, J = 2.0 Hz, 1H), 7.83–7.79 (m, 1H), 7.36–7.33 (m, 2H), 7.02–6.98 (m, 2H), 3.87 (s, 3H), 2.62–2.54 (m, 1H), 2.27–2.18 (m, 1H), 1.20 (s, 3H). 13C NMR (100 MHz, CDCl3; δ, ppm): 201.3, 160.7, 152.1, 142.8, 138.9, 136.9, 136.3, 130.8, 126.7, 125.5, 124.3, 118.9, 114.6, 109.5, 55.4, 50.2, 40.3 (q, J = 27.8 Hz, CH2CF3), 25.2. 19F NMR (376 MHz, CDCl3; δ, ppm): −61.26 (s, 3F). IR (KBr, ν, cm−1): 2,203, 1,732, 1,602, 1,508, 1,457, 1,362, 1,255, 1,177, 1,144, 1,069, 833. HRMS (ESI, m/z): calcd for C21H15ClF3NO2Na [M + Na]+ 428.0636, found 428.0623.
(E)-2-[2,5-Dimethyl-3-oxo-2-(2,2,2-trifluoroethyl)-2,3-dihydro-1H-inden-1-ylidene]-2-(p-tolyl)acetonitrile (3p)
Light yellow solid, 48 mg, 65% yield; mp 174.5–176.6°C. 1H NMR (400 MHz, CDCl3; δ, ppm): 8.83 (d, J = 8.8 Hz, 1H), 7.41–7.37 (m, 1H), 7.31 (d, J = 2.4 Hz, 1H), 7.29 (s, 3H), 7.26 (s, 1H), 3.94 (s, 3H), 2.60–2.50 (m, 1H), 2.42 (s, 3H), 2.27–2.16 (m, 1H), 1.19 (s, 3H). 13C NMR (100 MHz, CDCl3; δ, ppm): 202.4, 163.0, 152.7, 139.8, 138.0, 137.7, 130.2, 129.8, 129.6, 126.8, 125.2, 119.5, 106.5, 105.6, 56.0, 50.3, 40.3 (q, J = 27.6 Hz, CH2CF3), 25.2, 21.4. 19F NMR (376 MHz, CDCl3; δ, ppm): −61.38 (s, 3F). IR (KBr, ν, cm−1): 2,201, 1,725, 1,594, 1,486, 1,362, 1,296, 1,231, 1,146, 1,069, 832. HRMS (ESI, m/z): calcd for C22H18F3NONa [M + Na]+ 392.1233, found 392.1257.
(E)-2-(4-Chlorophenyl)-2-[5-methoxy-2-methyl-3-oxo-2-(2,2,2-trifluoroethyl)-2,3-dihydro-1H-inden-1-ylidene]acetonitrile (3q)
Light yellow solid, 52 mg, 64% yield; mp 130.5–131.2°C. 1H NMR (400 MHz, CDCl3; δ, ppm): 8.83 (d, J = 8.8 Hz, 1H), 7.48 (d, J = 8.4 Hz, 2H), 7.40 (d, J = 6.4 Hz, 1H), 7.39–7.35 (m, 2H), 7.32 (d, J = 2.4 Hz, 1H), 3.94 (s, 3H), 2.68–2.56 (m, 1H), 2.20–2.08 (m, 1H), 1.19 (s, 3H). 13C NMR (100 MHz, CDCl3; δ, ppm): 201.9, 163.3, 153.5, 137.8, 137.5, 136.1, 131.6, 131.2, 129.5, 126.0 (q, J = 160.5 Hz, CF3), 119.0, 105.8, 105.0, 56.0, 50.3, 40.3 (q, J = 27.7 Hz, CH2CF3), 25.2. 19F NMR (376 MHz, CDCl3; δ, ppm): −61.38 (s, 3F). IR (KBr, ν, cm−1): 2,202, 1,727, 1,595, 1,488, 1,364, 1,295, 1,143, 1,019, 845. HRMS (ESI, m/z): calcd for C21H15ClF3NO2Na [M + Na]+ 428.0636, found 428.0616.
(E)-2-[6-Chloro-2-methyl-3-oxo-2-(2,2,2-trifluoroethyl)-2,3-dihydro-1H-inden-1-ylidene]-2-phenylacetonitrile (3r)
Light yellow solid, 53 mg, 71% yield; mp 161.6–163.8°C. 1H NMR (400 MHz, CDCl3; δ, ppm): 8.94 (d, J = 1.2 Hz, 1H), 7.88 (d, J = 8.0 Hz, 1H), 7.69–7.64 (m, 1H), 7.54–7.50 (m, 3H), 7.45–7.39 (m, 2H), 2.62–2.54 (m, 1H), 2.22–2.13 (m, 1H), 1.19 (s, 3H). 13C NMR (100 MHz, CDCl3; δ, ppm): 201.0, 151.9, 145.7, 143.3, 133.8, 133.0, 132.6, 130.1, 129.4, 129.3, 125.6, 125.5, 118.4, 110.5, 50.0, 40.4 (q, J = 27.7 Hz, CH2CF3), 25.2. 19F NMR (376 MHz, CDCl3; δ, ppm): −61.25 (s, 3F). IR (KBr, ν, cm−1): 2,202, 1,724, 1,589, 1,489, 1,361, 1,271, 1,139, 1,072, 835, 712. HRMS (ESI, m/z): calcd for C20H13ClF3NONa [M + Na]+ 398.0530, found 398.0556.
(E)-2-[6-Chloro-2-methyl-3-oxo-2-(2,2,2-trifluoroethyl)-2,3-dihydro-1H-inden-1-ylidene]-2-(p-tolyl)acetonitrile (3s, Major)
Light yellow solid, 48 mg, 62% yield; mp 121.5–123.1°C. 1H NMR (400 MHz, CDCl3; δ, ppm): 8.92 (s, 1H), 7.86 (d, J = 8.0 Hz, 1H), 7.65 (d, J = 8.4 Hz, 1H), 7.36 (d, J = 7.6 Hz, 1H), 7.30 (s, 3H), 2.61–2.52 (m, 1H), 2.43 (s, 3H), 2.26–2.17 (m, 1H), 1.19 (s, 3H). 13C NMR (100 MHz, CDCl3; δ, ppm): 201.1, 151.7, 145.8, 143.2, 140.3, 133.8, 132.9, 130.8, 129.9, 126.8, 125.6, 118.5, 110.7, 50.1, 40.4 (q, J = 27.7 Hz, CH2CF3), 25.2, 21.4. 19F NMR (376 MHz, CDCl3; δ, ppm): −61.24 (s, 3F). IR (KBr, ν, cm−1): 2,205, 1,731, 1,588, 1,509, 1,456, 1,362, 1,255, 1,145, 1,072, 825. HRMS (ESI, m/z): calcd for C21H15ClF3NONa [M + Na]+ 412.0686, found 412.0686.
(E)-2-[2,6-Dimethyl-3-oxo-2-(2,2,2-trifluoroethyl)-2,3-dihydro-1H-inden-1-ylidene]-2-phenylacetonitrile (3t)
Light yellow solid, 41 mg, 58% yield; mp 147.9–150.4°C. 1H NMR (400 MHz, CDCl3; δ, ppm): 8.74 (s, 1H), 7.83 (d, J = 8.0 Hz, 1H), 7.53–7.48 (m, 4H), 7.45–7.41 (m, 2H), 2.62–2.54 (m, 4H), 2.21–2.11 (m, 1H), 1.17 (s, 3H). 13C NMR (100 MHz, CDCl3; δ, ppm): 201.7, 153.3, 147.9, 144.8, 133.7, 133.5, 133.1, 129.8, 129.6, 129.2, 125.5 (q, J = 112.6 Hz, CF3), 119.0, 108.7, 50.0, 40.3 (q, J = 27.6 Hz, CH2CF3), 25.2, 22.6. 19F NMR (376 MHz, CDCl3; δ, ppm): −61.33 (s, 3F). IR (KBr, ν, cm−1): 2,202, 1,716, 1,613, 1,489, 1,455, 1,360, 1,253, 1,136, 1,072, 831, 767. HRMS (ESI, m/z): calcd for C21H16F3NONa [M + Na]+ 378.1076, found 378.1054.
(E)-2-(4-Chlorophenyl)-2-[2,6-dimethyl-3-oxo-2-(2,2,2-trifluoroethyl)-2,3-dihydro-1H-inden-1-ylidene]acetonitrile (3u, Major)
Light yellow solid, 66 mg, 85% yield; mp 108.1–110.8°C. 1H NMR (400 MHz, CDCl3; δ, ppm): 8.72 (s, 1H), 7.84 (d, J = 8.0 Hz, 1H), 7.52–7.48 (m, 3H), 7.40 (d, J = 6.8 Hz, 2H), 2.66–2.58 (m, 4H), 2.17–2.08 (m, 1H), 1.18 (s, 3H). 13C NMR (100 MHz, CDCl3; δ, ppm): 201.3, 154.0, 148.0, 144.6, 136.2, 134.0, 133.8, 132.3, 131.0, 129.5, 126.9, 125.0 (q, J = 106.5 Hz, CF3), 124.6, 118.6, 107.4, 50.0, 40.4 (q, J = 27.6 Hz, CH2CF3), 25.2, 22.6. 19F NMR (376 MHz, CDCl3; δ, ppm): −61.33 (s, 3F). IR (KBr, ν, cm−1): 2,208, 1,727, 1,595, 1,489, 1,360, 1,253, 1,180, 1,142, 1,071, 832. HRMS (ESI, m/z): calcd for C21H15ClF3NONa [M + Na]+ 412.0686, found 412.0637.
(E)-2-(4-Bromophenyl)-2-[2,6-dimethyl-3-oxo-2-(2,2,2-trifluoroethyl)-2,3-dihydro-1H-inden-1-ylidene]acetonitrile (3v, Major)
Light yellow solid, 39 mg, 45% yield; mp 136.5–138.6°C. 1H NMR (400 MHz, CDCl3; δ, ppm): 8.71 (s, 1H), 7.83 (d, J = 8.0 Hz, 1H), 7.65 (d, J = 8.4 Hz, 2H), 7.52 (d, J = 8.0 Hz, 1H), 7.31 (d, J = 8.4 Hz, 2H), 2.66–2.61 (m, 1H), 2.58 (s, 3H), 2.17–2.09 (m, 1H), 1.18 (s, 3H). 13C NMR (100 MHz, CDCl3; δ, ppm): 201.3, 154.0, 145.0, 144.6, 134.0, 133.8, 133.2, 132.5, 131.2, 126.9, 125.5 (q, J = 105.5 Hz, CF3), 124.6, 118.5, 107.4, 50.0, 40.4 (q, J = 27.7 Hz, CH2CF3), 25.2, 22.6. 19F NMR (376 MHz, CDCl3; δ, ppm): −61.31 (s, 3F). IR (KBr, ν, cm−1): 2,206, 1,731, 1,593, 1,456, 1,362, 1,255, 1,141, 1,070, 1,011, 831. HRMS (ESI, m/z): calcd for C21H15BrF3NONa [M + Na]+ 456.0181, found 456.0137.
(E)-2-[2,6-Dimethyl-3-oxo-2-(2,2,2-trifluoroethyl)-2,3-dihydro-1H-inden-1-ylidene]-2-(p-tolyl)acetonitrile (3w, Major)
Light yellow solid, 54 mg, 73% yield; mp 127.8–129.9°C. 1H NMR (400 MHz, CDCl3; δ, ppm): 8.73 (s, 1H), 7.82 (d, J = 8.0 Hz, 1H), 7.49 (d, J = 8.0 Hz, 1H), 7.30 (s, 4H), 2.61–2.53 (m, 4H), 2.43 (s, 3H), 2.24–2.17 (m, 1H), 1.18 (s, 3H). 13C NMR (100 MHz, CDCl3; δ, ppm): 201.9, 153.2, 147.8, 144.9, 140.0, 133.6, 133.3, 130.5, 129.8, 129.4, 127.1, 125.4 (q, J = 114.4 Hz, CF3), 119.1, 108.9, 50.0, 40.3 (q, J = 27.5 Hz, CH2CF3), 25.2, 22.6, 21.4. 19F NMR (376 MHz, CDCl3; δ, ppm): −61.32 (s, 3F). IR (KBr, ν, cm−1): 2,204, 1,719, 1,609, 1,590, 1,510, 1,456, 1,361, 1,254, 1,144, 1,071, 830. HRMS (ESI, m/z): calcd for C22H18F3NONa [M + Na]+ 392.1233, found 392.1223.
(E)-2-[2,6-Dimethyl-3-oxo-2-(2,2,2-trifluoroethyl)-2,3-dihydro-1H-inden-1-ylidene]-2-(4-methoxyphenyl)acetonitrile (3x, Major)
Light yellow solid, 63 mg, 82% yield; mp 120.8–122.4°C. 1H NMR (400 MHz, CDCl3; δ, ppm): 8.72 (s, 1H), 7.82 (d, J = 8.0 Hz, 1H), 7.49 (d, J = 8.0 Hz, 1H), 7.36 (d, J = 8.4 Hz, 2H), 7.00 (d, J = 8.8 Hz, 2H), 3.87 (s, 3H), 2.62–2.55 (m, 4H), 2.24–2.19 (m, 1H), 1.19 (s, 3H). 13C NMR (100 MHz, CDCl3; δ, ppm): 201.9, 160.5, 153.3, 147.8, 144.9, 133.6, 133.3, 130.9, 127.0, 125.5 (q, J = 114.2 Hz, CF3), 125.1, 119.2, 114.5, 108.6, 55.4, 50.1, 40.2 (q, J = 27.7 Hz, CH2CF3), 25.2, 22.6. 19F NMR (376 MHz, CDCl3; δ, ppm): −61.32 (s, 3F). IR (KBr, ν, cm−1): 2,205, 1,724, 1,605, 1,507, 1,457, 1,362, 1,257, 1,141, 1,070, 1,026, 832. HRMS (ESI, m/z): calcd for C22H18F3NO2Na [M + Na]+ 408.1182, found 408.1182.
3-[2-Amino-1-(4-chlorophenyl)ethyl]-6-methoxy-2-methyl-2-(2,2,2-trifluoroethyl)-2,3-dihydro-1H-inden-1-ol (4)
White oil, 11 mg, 51% yield. 1H NMR (400 MHz, CDCl3; δ, ppm): 8.39 (d, J = 8.4 Hz, 1H), 7.47–7.41 (m, 2H), 7.35–7.30 (m, 2H), 6.99 (s, 2H), 4.78 (s, 1H), 3.89 (s, 3H), 3.70–3.66 (m, 1H), 3.25–3.21 (m, 1H), 2.37–2.23 (m, 2H), 1.95–1.91 (m, 1H), 1.78 (s, 2H), 1.70–1.66 (m, 1H), 1.07 (s, 3H). 13C NMR (100 MHz, d6-DMSO; δ, ppm): 162.8, 160.4, 151.7, 134.3, 133.5, 130.7 (q, J = 154.3 Hz, CF3), 128.2, 126.1, 120.2, 115.7, 109.0, 102.2, 79.4, 67.5, 56.0, 53.7, 25.6, 24.2. 19F NMR (376 MHz, d6-DMSO; δ, ppm): −58.24 (s, 3F). HRMS (ESI, m/z): calcd for C21H23ClF3NNaO2 [M + Na]+ 436.8508, found 436.8517.
Data Availability Statement
The datasets generated for this study are available on request to the corresponding author.
Author Contributions
T-SZ, BJ, and P-JC designed the project. T-SZ performed the experiments. T-SZ, W-JH, S-JT, and P-JC analyzed the data. T-SZ, BJ, and GL wrote the manuscript.
Conflict of Interest
The authors declare that the research was conducted in the absence of any commercial or financial relationships that could be construed as a potential conflict of interest.
Acknowledgments
We are grateful for financial support from the Fundamental Research Funds for the Central Universities (JH180263).
Supplementary Material
The Supplementary Material for this article can be found online at: https://www.frontiersin.org/articles/10.3389/fchem.2020.00234/full#supplementary-material
References
Bergmann, E. D. (1956). Fulvenes and thermochromic ethylenes. Part 30. experiments in the 2,3-diarylindone series. J. Org. Chem. 21, 461–464. doi: 10.1021/jo01110a023
Chen, M., Weng, Y., Guo, M., Zhang, H., and Lei, A. (2008). Nickel-catalyzed reductive cyclization of unactivated 1,6-enynes in the presence of organozinc reagents. Angew. Chem. Int. Ed. 47, 2279–2282. doi: 10.1002/anie.200704452
Chen, S., Feng, D.-F., Li, D.-Y., and Liu, P.-N. (2018). Radical cyanotrifluoromethylation of isocyanides: step-economical access to CF3-containing nitriles, amines, and imines. Org. Lett. 20, 5418–5422. doi: 10.1021/acs.orglett.8b02328
Chen, Z.-M., Bai, W., Wang, S.-H., Yan, B.-M., Tu, Y.-Q., and Zhang, F.-M. (2013). Copper-catalyzed tandem trifluoromethylation/semipinacol rearrangement of allylic alcohols. Angew. Chem. Int. Ed. 52, 9781–9785. doi: 10.1002/anie.201304557
Chernyak, N., Gorelsky, S. I., and Gevorgyan, V. (2011). Palladium-catalyzed carbocyclization of alkynyl ketones proceeding through a carbopalladation pathway. Angew. Chem. Int. Ed. 50, 2342–2345. doi: 10.1002/anie.201006751
Chu, L., and Qing, F.-L. (2012). Copper-catalyzed oxidative trifluoromethylation of terminal alkenes using nucleophilic CF3SiMe3: efficient C(sp3)-CF3 bond formation. Org. Lett. 14, 2106–2109. doi: 10.1021/ol300639a
Dai, J.-R., Hallock, Y. F., Cardellina, J. H., and Boyd, M. R. (1998). HIV-inhibitory and cytotoxic oligostilbenes from the leaves of Hopea malibato. J. Nat. Prod. 61, 351–353. doi: 10.1021/np970519h
deSolms, S. J, Woltersdorf, O. W. Jr, Cragoe, E. J. Jr, Watson, L. S, and Fanelli, G. M. Jr. (1978). (Acylaryloxy)acetic acid diuretics. 2. (2-Alkyl-2-Aryl-1-Oxo-5-Indanyloxy)acetic acids. J. Med. Chem. 21, 437–443. doi: 10.1021/jm00203a006
Egami, H., Shimizu, R., and Sodeoka, M. (2012). Oxytrifluoromethylation of multiple bonds using copper catalyst under mild conditions. Tetrahedron Lett. 53, 5503–5506. doi: 10.1016/j.tetlet.2012.07.134
Egami, H., Shimizu, R., Usui, Y., and Sodeoka, M. (2013). Iron-catalyzed trifluoromethylation with concomitant C-C bond formation via 1,2-migration of an aryl group. Chem. Commun. 49, 7346–7348. doi: 10.1039/c3cc43936d
Frank, R. L., Eklund, H., Richter, J. W., Vanneman, C. R., and Wennerberg, A. N. (1944). Some 2,3-disubstituted indones. J. Am. Chem. Soc. 66, 1–4. doi: 10.1021/ja01229a001
Fu, W., Guo, W., Zou, G., and Xu, C. (2012). Selective trifluoromethylation and alkynylation of tetrahydroisoquinolines using visible light irradiation by Rose Bengal. J. Fluor. Chem. 140, 88–94. doi: 10.1016/j.jfluchem.2012.05.009
Ge, G.-C., Huang, X.-J., Ding, C.-H., Wan, S.-L., Dai, L.-X., and Hou, X.-L. (2014). A new strategy to construct a C-C-CF3 subunit via CuBr-catalyzed domino reaction of homopropargyl amines: an efficient synthesis of trifluoromethyl containing building blocks 4-trifluoromethyl-2,3-dihydro-pyrroliums. Chem. Commun. 50, 3048–3051. doi: 10.1039/c3cc49059a
Hagmann, W. K. (2008). The many roles for fluorine in medicinal chemistry. J. Med. Chem. 51, 4359–4369. doi: 10.1021/jm800219f
Hao, W.-J., Gao, Q., Jiang, B., Liu, F., Wang, S.-L., Tu, S.-J., et al. (2016). Base-promoted [4+1]/[3+1+1] bicyclization for accessing functionalized Indeno[1,2-c]furans. J. Org. Chem. 81, 11276–11281. doi: 10.1021/acs.joc.6b02249
He, G., Wu, C., Zhou, J., Yang, Q., Zhang, C., Zhou, Y., et al. (2018). A method for synthesis of 3-Hydroxy-1-indanones via Cu-catalyzed intramolecular annulation reactions. J. Org. Chem. 83, 13356–13362. doi: 10.1021/acs.joc.8b02149
He, Y.-T., Li, L.-H., Yang, Y.-F., Zhou, Z.-Z., Hua, H.-L., and Liu, X.-, Liang, Y.-M. (2014a). Copper-catalyzed intermolecular cyanotrifluoromethylation of alkenes. Org. Lett. 16, 270–273. doi: 10.1021/ol403263c
He, Y.-T., Li, L.-H., Zhou, Z.-Z., Hua, H.-L., Qiu, Y.-F., Liu, X.-Y., et al. (2014b). Copper-catalyzed three-component cyanotrifluoromethylation/azidotrifluoromethylation and carbocyclization of 1,6-enynes. Org. Lett. 16, 3896–3899. doi: 10.1021/ol501574f
He, Y.-T., Wang, Q., Zhao, J., Liu, X.-Y., Xu, P.-F., and Liang, Y.-M. (2015). The copper-catalyzed synthesis of β-trifluoromethylated acrylonitriles and trifluoromethyl-substituted 2H-azirines. Chem. Commun. 51, 13209–13212. doi: 10.1039/C5CC05066A
Huang, M.-H., Hao, W.-J., Li, G., Tu, S.-J., and Jiang, B. (2018). Recent advances in radical transformations of internal alkynes. Chem. Commun. 54, 10791–10811. doi: 10.1039/C8CC04618B
Iqbal, N., Jung, J., Park, S., and Cho, E. J. (2014). Controlled trifluoromethylation reactions of alkynes through visible-light photoredox catalysis. Angew. Chem. Int. Ed. 53, 539–542. doi: 10.1002/anie.201308735
Ito, T., Tanaka, T., Iinuma, M., Nakaya, K., Takahashi, Y., Sawa, R., et al. (2004). Three new resveratrol oligomers from the stem bark of Vatica pauciflora. J. Nat. Prod. 67, 932–937. doi: 10.1021/np030236r
Janson, P. G., Ghoneim, I., Ilchenko, N. O., and Szabó, K. J. (2012). Electrophilic trifluoromethylation by copper-catalyzed addition of CF3-transfer reagents to Alkenes and Alkynes. Org. Lett. 14, 2882–2885. doi: 10.1021/ol3011419
Ji, C.-L., Hao, W.-J., Zhang, J., Geng, F.-Z., Xu, T., Tu, S.-J., et al. (2019). Catalytic three-component synthesis of functionalized naphtho[2,1-b]oxecines via a double bond cleavage–rearrangement cascade. Org. Lett. 21, 6494–6498. doi: 10.1021/acs.orglett.9b02367
Kamigata, N., Fukushima, T., and Yoshida, M. (1990). Reactions of perfluoroalkanesulfonyl chlorides with aromatic compounds catalyzed by a Ruthenium(II) complex. Chem. Lett. 649–650. doi: 10.1246/cl.1990.649
Koelsch, C. F. (1932). Synthesis with triarylvinylmagnesium romides. triarylacrylic acids and the indones derived from them. J. Am. Chem. Soc. 54, 2487–2493. doi: 10.1021/ja01345a046
Kong, W., Fuentes, N., García-Domínguez, A., Merino, E., and Nevado, C. (2015). Stereoselective synthesis of highly functionalized indanes and dibenzocycloheptadienes through complex radical cascade reactions. Angew. Chem. Int. Ed. 54, 2487–2491. doi: 10.1002/anie.201409659
Kuninobu, Y., Nagase, M., and Kanai, M. (2015). Benzylic C(sp3)-H perfluoroalkylation of six-membered heteroaromatic compounds. Angew. Chem. Int. Ed. 54, 10263–10266. doi: 10.1002/anie.201505335
Li, Y., and Studer, A. (2012). Transition-metal-free trifluoromethylaminoxylation of alkenes. Angew. Chem. Int. Ed. 51, 8221–8224. doi: 10.1002/anie.201202623
Lin, J.-S., Dong, X.-Y., Li, T.-T., Jiang, N.-C., Tan, B., and Liu, X.-Y. (2016). A dual-catalytic strategy to direct asymmetric radical aminotrifluoromethylation of alkenes. J. Am. Chem. Soc. 138, 9357–9360. doi: 10.1021/jacs.6b04077
Liu, S., Chen, K., Hao, W.-J., Tu, X.-C., Tu, S.-J., and Jiang, B. (2019). Generation of 1,3-dimethylene-substituted isobenzofurans via Pd(II)-catalyzed selective oxo-cyclization/SO2 insertion cascade of β-Alkynyl ketones. J. Org. Chem. 84, 1964–1971. doi: 10.1021/acs.joc.8b02934
Liu, W., Huang, X., Cheng, M.-J., Nielsen, R. J., Goddard, III W. A., and Groves, J. T. (2012). Oxidative aliphatic C-H fluorination with fluoride ion catalyzed by a manganese porphyrin. Science. 337, 1322–1325. doi: 10.1126/science.1222327
Liu, X., Xiong, F., Huang, X., Xu, L., Li, P., and Wu, X. (2013). Copper-Catalyzed trifluoromethylation-initiated radical 1,2-Aryl Migration in α, α-diaryl allylic alcohols. Angew. Chem. Int. Ed. 52, 6962–6966. doi: 10.1002/anie.201302673
Liu, Y., Zhang, J.-L., Song, R.-J., Qian, P.-C., and Li, J.-H. (2014). Cascade Nitration/Cyclization of 1,7-Enynes with tBuONO and H2O: one-pot self-assembly of Pyrrolo[4,3,2-de]quinolinones. Angew. Chem. Int. Ed. 53, 9017–9020. doi: 10.1002/anie.201404192
Manning, C., McClory, M. R., and McCullough, J. J. (1981). Sigmatropic Rearrangements of 1,1-diarylindenes. Migratory aptitudes of aryl migration in the ground and electronically excited states. J. Org. Chem. 46, 919–930. doi: 10.1021/jo00318a018
Müller, K., Faeh, C., and Diederich, F. (2007). Fluorine in pharmaceuticals: looking beyond intuition. Science 317, 1881–1886. doi: 10.1126/science.1131943
Nagle, D. G., Zhou, Y.-D., Park, P. U., Paul, V. J., Rajbhandari, I., Duncan, C. J. G., et al. (2000). A new indanone from the marine cyanobacterium lyngbya majuscula that inhibits hypoxia-induced activation of the VEGF promoter in Hep3B cells. J. Nat. Prod. 63, 1431–1433. doi: 10.1021/np000216e
Nitta, T., Arai, T., Takamatsu, H., Inatomi, Y., Murata, H., Iinuma, M., et al. (2002). Antibacterial activity of extracts prepared from tropical and subtropical plants on methicillin-resistant Staphylococcus aureus. J. Health Sci. 48, 273–276. doi: 10.1248/jhs.48.273
Pair, E., Monteiro, N., Bouyssi, D., and Baudoin, O. (2013). Copper-catalyzed trifluoromethylation of N, N-dialkylhydrazones. Angew. Chem. Int. Ed. 52, 5346–5349. doi: 10.1002/anie.201300782
Pan, Y., Wang, S., Kee, C. W., Dubuisson, E., Yang, Y., Loh, K. P., et al. (2011). Graphene oxide and Rose Bengal: oxidative C–H functionalisation of tertiary amines using visible light. Green Chem. 13, 3341–3344. doi: 10.1039/c1gc15865a
Qin, X.-Y., He, L., Li, J., Hao, W.-J., Tu, S.-J., and Jiang, B. (2019). Regioselective synthesis of polycyclic sulfones via radical-induced three-component bicyclization cascades. Chem. Commun. 55, 3227–3230. doi: 10.1039/C9CC00324J
Shen, Z.-J., Shi, H.-N., Hao, W.-J., Tu, S.-J., and Jiang, B. (2018b). Visible-light photocatalytic bicyclization of β-alkynyl propenones for accessing diastereoenriched syn-fluoren-9-ones. Chem. Commun. 54, 11542–11545. doi: 10.1039/C8CC06086J
Shen, Z.-J., Wang, S.-C., Hao, W.-J., Yang, S.-Z., Tu, S.-J., and Jiang, B. (2019). Switching between copper-catalysis and photocatalysis for tunable halofluoroalkylation and hydrofluoroalkylation of 1,6-enynes toward 1-indenones. Adv. Synth. Catal. 361, 3837–3851. doi: 10.1002/adsc.201900559
Shen, Z.-J., Wu, Y.-N., He, C.-L., He, L., Hao, W.-J., Wang, A.-F., et al. (2018a). Stereoselective synthesis of sulfonated 1-indenones via radical-triggered multicomponent cyclization of β-alkynyl propenones. Chem. Commun. 54, 445–448. doi: 10.1039/C7CC08516H
Shi, H.-N., Huang, M.-H., Hao, W.-J., Tu, X.-C., Tu, S.-J., and Jiang, B. (2019a). Synthesis of diastereoenriched 1-indanones via double-Base cooperatively Promoted 1,4-Oxo-Migration/Cyclization of β-Alkynyl Ketones. J. Org. Chem. 84, 16027–16035. doi: 10.1021/acs.joc.9b02525
Shi, H.-N., Huang, M.-H., He, C.-L., Lu, H.-P., Hao, W.-J., Tu, X.-C., et al. (2019b). Completely stereoselective synthesis of sulfonated 1,3-dihydroisobenzofurans via radical multicomponent reactions. J. Org. Chem. 84, 13686–13695. doi: 10.1021/acs.joc.9b01918
Shimizu, R., Egami, H., Hamashima, Y., and Sodeoka, M. (2012). Copper-catalyzed trifluoromethylation of allylsilanes. Angew. Chem., Int. Ed. 51, 4577–4480. doi: 10.1002/anie.201201095
Shintani, R., Takatsu, K., and Hayashi, T. (2007). Rhodium-catalyzed asymmetric synthesis of 3,3-disubstituted 1-indanones. Angew. Chem. Int. Ed. 46, 3735–3737. doi: 10.1002/anie.200700226
Song, L., Tian, G., and Van der Eycken, E. V. (2019). Chemo- and regioselective catalyst-controlled carbocyclization of alkynyl ketones: rapid synthesis of 1-indanones and 1-naphthols. Chem. Eur. J. 25, 7645–7648. doi: 10.1002/chem.201901860
Studer, A. (2012). A “Renaissance” in radical trifluoromethylation. Angew. Chem. Int. Ed. 51, 8950–8958. doi: 10.1002/anie.201202624
Tomita, R., Koike, T., and Akita, M. (2015). Photoredox-catalyzed stereoselective conversion of alkynes into tetrasubstituted trifluoromethylated alkenes. Angew. Chem. Int. Ed. 54, 12923–12927. doi: 10.1002/anie.201505550
Umemoto, T. (1996). Electrophilic perfluoroalkylating agents. Chem. Rev. 96, 1757–1778. doi: 10.1021/cr941149u
Wang, F., Wang, D., Wan, X., Wu, L., Chen, P., and Liu, G. (2016). Enantioselective copper-catalyzed intermolecular cyanotrifluoromethylation of alkenes via radical process. J. Am. Chem. Soc. 138, 15547–15550. doi: 10.1021/jacs.6b10468
Wang, F., Zhu, N., Chen, P., Ye, J., and Liu, G. (2015). Copper-catalyzed trifluoromethylazidation of alkynes: efficient access to CF3-substituted azirines and aziridines. Angew. Chem. Int. Ed. 54, 9356–9360. doi: 10.1002/anie.201503412
Wang, N.-N., Hao, W.-J., Zhang, T.-S., Li, G., Wu, Y.-N., Tu, S.-J., et al. (2016a). Metal-free C(sp3)-H functionalization: oxidative carbo-oxygenation of α-diazo carbonyls via radical dediazotization. Chem. Commun. 52, 5144–5147. doi: 10.1039/C6CC00816J
Wang, N.-N., Huang, L.-R., Hao, W.-J., Zhang, T.-S., Li, G., Tu, S.-J., et al. (2016b). Synergistic rhodium/copper catalysis: synthesis of 1,3-enynes and N-Aryl enaminones. Org. Lett. 18, 1298–1301. doi: 10.1021/acs.orglett.6b00238
Wessig, P., and Teubner, J. (2006). Total synthesis of pterosines B and C via a photochemical key step. Synlett 2006, 1543–1546. doi: 10.1055/s-2006-944190
Wilger, D. J., Gesmundo, N. J., and Nicewicz, D. A. (2013). Catalytic hydrotrifluoromethylation of styrenes and unactivated aliphatic alkenesvia an organic photoredox system. Chem. Sci. 4, 3160–3165. doi: 10.1039/c3sc51209f
Wu, X., Chu, L., and Qing, F.-L. (2013). Silver-catalyzed hydrotrifluoromethylation of unactivated alkenes with CF3SiMe3. Angew. Chem. Int. Ed. 52, 2198–2202. doi: 10.1002/anie.201208971
Wu, Z., Wang, D., Liu, Y., Huan, L., and Zhu, C. (2017). Chemo- and regioselective distal heteroaryl ipso-Migration: a general protocol for heteroarylation of unactivated alkenes. J. Am. Chem. Soc. 139, 1388–1391. doi: 10.1021/jacs.6b11234
Xiao, H., Liu, Z., Shen, H., Zhang, B., Zhu, L., and Li, C. (2019). Copper-catalyzed late-stage benzylic C(sp3)-H trifluoromethylation. Chemistry 5, 940–949. doi: 10.1016/j.chempr.2019.02.006
Yang, X., Wu, T., Phipps, R. J., and Toste, F. D. (2015). Advances in catalytic enantioselective fluorination, Mono-, Di-, and trifluoromethylation, and trifluoromethylthiolation reactions. Chem. Rev. 115, 826–870. doi: 10.1021/cr500277b
Yasu, Y., Koike, T., and Akita, M. (2013). Visible-light-induced synthesis of a variety of trifluoromethylated alkenes from potassium vinyltrifluoroborates by photoredox catalysis. Chem. Commun. 49, 2037–2039. doi: 10.1039/c3cc39235j
Zhang, Z. Q., Meng, X. Y., Sheng, J., Lan, Q., and Wang, X. S. (2019). Enantioselective copper-catalyzed 1, 5-cyanotrifluoromethylation of vinylcyclopropanes. Organic Lett. 21, 8256–8260. doi: 10.1021/acs.orglett.9b03012
Zhu, R., and Buchwald, S. L. (2012). Copper-catalyzed oxytrifluoromethylation of unactivated alkenes. J. Am. Chem. Soc. 134, 12462–12465. doi: 10.1021/ja305840g
Zhu, R., and Buchwald, S. L. (2013). Enantioselective functionalization of radical intermediates in redox catalysis: copper-catalyzed asymmetric oxytrifluoromethylation of alkenes. Angew. Chem. Int. Ed. 52, 12655–12658. doi: 10.1002/anie.201307790
Zhu, X.-T., Lu, Q.-L., Wang, X., Zhang, T.-S., Hao, W.-J., Tu, S.-J., et al. (2018a). Substrate-controlled generation of 3-sulfonylated 1-indenones and 3-arylated (Z)-indenes via Cu-catalyzed radical cyclization cascades of o-Alkynylbenzonitriles. J. Org. Chem. 83, 9890–9901. doi: 10.1021/acs.joc.8b01343
Zhu, X.-T., Zhang, T.-S., Zhao, Q., Cai, P.-J., Hao, W.-J., Tu, S.-J., et al. (2018b). Sulfinate-salt-mediated radical relay cyclization of cyclic ethers with 2-alkynylbenzonitriles toward 3-alkylated 1-indenones. Chem. Asian J. 13, 1157–1164. doi: 10.1002/asia.201800211
Keywords: Cu(II) catalysis, annulation–difunctionalization, cyanotrifluoromethylation, 1, 6-enynes, 1-indanones
Citation: Zhang T-S, Hao W-J, Cai P-J, Li G, Tu S-J and Jiang B (2020) Copper-Catalyzed Annulation–Cyanotrifluoromethylation of 1,6-Enynes Toward 1-Indanones via a Radical Process. Front. Chem. 8:234. doi: 10.3389/fchem.2020.00234
Received: 13 January 2020; Accepted: 11 March 2020;
Published: 17 April 2020.
Edited by:
Nam-Jung Kim, Kyung Hee University, South KoreaReviewed by:
Albert Moyano, University of Barcelona, SpainAlexandre Pradal, UMR8232 Institut Parisien de Chimie Moléculaire, France
Norio Shibata, Nagoya Institute of Technology, Japan
Copyright © 2020 Zhang, Hao, Cai, Li, Tu and Jiang. This is an open-access article distributed under the terms of the Creative Commons Attribution License (CC BY). The use, distribution or reproduction in other forums is permitted, provided the original author(s) and the copyright owner(s) are credited and that the original publication in this journal is cited, in accordance with accepted academic practice. No use, distribution or reproduction is permitted which does not comply with these terms.
*Correspondence: Pei-Jun Cai, pjcai@cumt.edu.cn; Bo Jiang, jiangchem@jsnu.edu.cn