- 1Laboratory of Organic and Metal-organic Nitrogen-Oxygen Systems, N. D. Zelinsky Institute of Organic Chemistry, Moscow, Russia
- 2Department of Innovational Materials and Technologies Chemistry, Plekhanov Russian University of Economics, Moscow, Russia
Reductive amination of carbonyl compounds with primary amines is a well-established synthetic methodology for the selective production of unsymmetrically substituted secondary and tertiary amines. From the industrial and green chemistry perspective, it is attractive to combine reductive amination with the synthesis of primary amines in a single one-pot catalytic process. In this regard, nitro compounds, which are readily available and inexpensive feedstocks, received much attention as convenient precursors to primary amines in such processes. Although the direct reductive coupling of nitro compounds with aldehydes/ketones to give secondary and tertiary amines has been known since the 1940's, due to the development of highly efficient and selective non-noble metal-based catalysts a breakthrough in this area was made in the last decade. In this short overview, recent progress in the methodology of the reductive amination with nitro compounds is summarized together with applications to the synthesis of bioactive amines and heterocycles. Remaining challenges in this field are also analyzed.
Introduction
Secondary amines are privileged compounds in the design of pharmaceutically relevant molecules, as well as important building blocks in the synthesis of agrochemicals, dyes, and functional materials. However, direct synthesis of secondary amines by double alkylation of ammonia is problematic because of its low selectivity and environmental issues. Preparation of unsymmetrically substituted secondary amines by selective monoalkylation of primary amines is even more challenging. The selectivity issue is efficiently solved by the reductive amination process, in which controlled alkylation of primary amines is performed by condensation with aldehyde/ketone to form an imine followed by the reduction of the C=N bond (usually by catalytic hydrogenation or hydride transfer) (Tarasevich and Kozlov, 1999; Afanasyev et al., 2019). From the viewpoint of green chemistry, it is beneficial to combine reductive amination with the synthesis of primary amines is a one-pot process. This strategy is efficiently realized by using nitroarenes and nitroalkanes as convenient sources of aromatic and aliphatic amines (Orlandi et al., 2018).
Nitro compounds are readily available feedstocks (Green and Johnson, 2000). Simple aliphatic nitro compounds are prepared by Konovalov nitration of alkanes on an industrial scale as well as by the radical nitration of C–H active compounds (Ono, 2001). Kormblum reaction (nucleophilic substitution of halide for the nitrite ion) and the addition of electrophiles to nitronate anions are widely used to prepare branched and functionalized nitro compounds of aliphatic series (Ono, 2001). Nitroarenes are easily accessed through the electrophilic nitration of C–H, C–B, C–M, and C–Hal bonds in arenes (Olah and Malhotra, 2001; Yan and Yang, 2013). Furthermore, both aliphatic and aromatic nitro compounds can be delivered from the oxidation of various nitrogen-containing derivatives, such as nitroso compounds, oximes, azides, etc. (Ono, 2001).
Conventional reductive amination protocols utilizing mild hydride-based reagents (Abdel-Magid et al., 1996) are not suitable for the reduction of the nitro group. For this reason, catalytic hydrogenation is used to transform NO2 fragment into the amino group and perform subsequent reductive amination under the same conditions in a tandem sequence. In this short overview, recent advances in the methodology and application of the catalytic reductive amination with nitro compounds are outlined.
Intermolecular Reductive Amination of Aldehydes and Ketones With Nitro Compounds: Recent Progress
Studies on the reductive amination of carbonyl derivatives with nitro compounds (reductive alkylation of nitro compounds) date back to the first half of twentieth century (Major, 1931) (Figure 1a). In 1940, Emerson and Mohrman reported on the coupling of aromatic nitro compounds with aldehydes under catalytic hydrogenation over Raney nickel catalyst (cat = Ra-Ni) (Emerson and Mohrman, 1940). Although the procedure was general for both aromatic and aliphatic aldehydes, the yields of secondary amines were moderate in many cases.
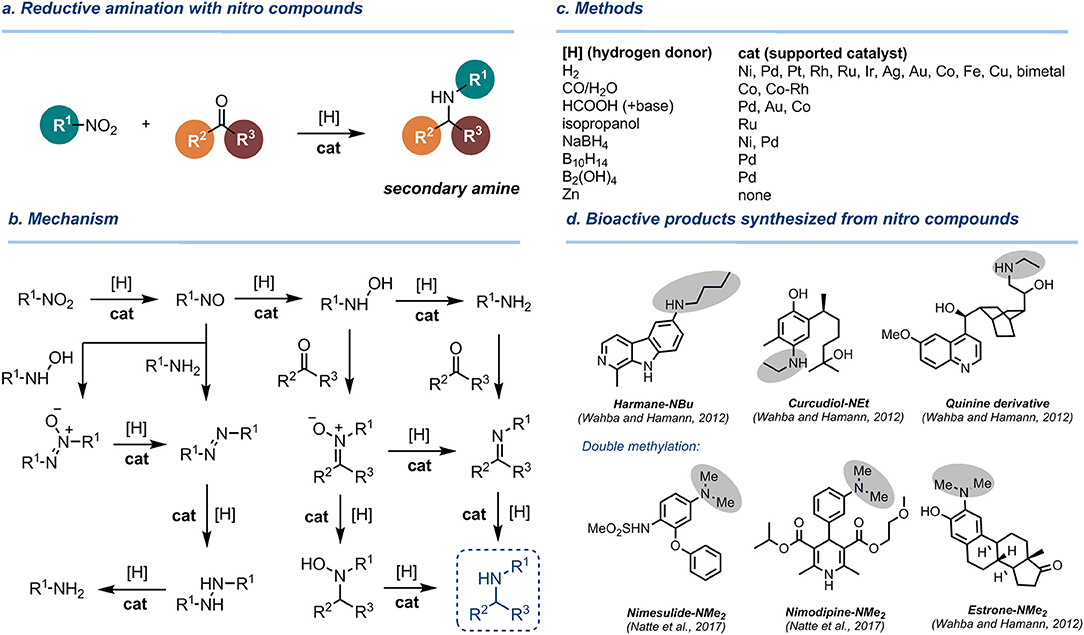
Figure 1. Reductive amination of aldehydes and ketones with nitro compounds. (a) Overall reaction scheme. (b) Mechanism. (c) Methods. (d) Bioactive products synthesized by reductive alkylation of nitro compounds.
The reasons for low selectivity in this and other catalytic protocols are underlay by the complexity of the mechanism of the nitro group reduction involving numerous reactive intermediates (nitroso derivatives, hydroxylamines, azocompounds, imines, oximes, etc., Figure 1b), which can be isolated as side-products (Benchekroun-Mounir et al., 1993; Maeda et al., 1999). These intermediates can react with carbonyl compounds or primary amines, leading to side processes and a decrease of yield of target secondary amines. Other selectivity issues arise from the hydrogenation of the aromatic ring (Cirujano et al., 2013) and the formation of tertiary amines by a double reductive amination (Emerson and Uraneck, 1941). In reactions with formaldehyde, double reductive methylation to give tertiary amines occurs very easily, while the controlled monomethylation is challenging (Natte et al., 2017).
The nature of catalyst, support, and additives is highly important to ensure full conversion of the aforementioned intermediates and for achieving selective synthesis of secondary amines from nitro compounds. The application of supported platinum catalysts was shown to be advantageous in terms of selectivity and yield of target secondary amines. The platinum-catalyzed process for reductive alkylation of nitroarenes developed by Bayer could be used for a semi-industrial production of N-alkyl-N-aryl amines (Maurer et al., 1998). Modification of platinum catalysts by various acidic additives increases the selectivity and yield of secondary amines by preventing degradation of amines through the formation of salts (Tarasevich and Kozlov, 1999).
In the last decade, numerous other catalytic systems were developed based on supported palladium (Sydnes et al., 2008; Sreedhar et al., 2009; Dell'anna et al., 2011; Wei et al., 2013; Zhou et al., 2015; Sharma et al., 2017), platinum (Hu et al., 2011; Cirujano et al., 2013), rhodium (Huang et al., 2015), iridium Pintado-Sierra et al., 2013; Sui et al., 2017), ruthenium (Del Pozo et al., 2012), silver (Artiukha et al., 2017), and gold (Yamane et al., 2009; Artiukha et al., 2015) nanoparticles. Heterobimetallic systems such as Pd-Ag (Li et al., 2013; Chen et al., 2015), Pd-Au (Cho et al., 2018; Yin et al., 2018), Ni-Pd (Nişanci et al., 2015), and Rh-Co (Park and Chung, 2015) were also shown to be efficient catalysts for the reductive alkylation of nitro compounds. With noble metal catalysts, reductive alkylation of nitro compounds is performed under mild conditions (r.t. to 100°C) and low hydrogen pressure (typically, 1–25 bar). Palladium and platinum-catalyzed processes are characterized by high TON (up to 3,800, average ca. 100÷200) and TOF (up to 1,900, average ca. 50÷100 h−1) values (Cirujano et al., 2013; Kalbasi and Mazaheri, 2016). Furthermore, these catalysts often show very good recyclability (Park and Chung, 2015; Cho et al., 2018). However, noble metal catalysts are not always selective for the reductive N-alkylation of nitroarenes with aromatic aldehydes. Benzyl alcohols and methylarenes resulting from the hydrogenolysis of aromatic aldehydes are usually found as the by-products with platinum metal-based catalysts (Huang et al., 2015). Another limitation is the inability to tolerate functional groups reducible under hydrogenation over platinum metal catalysts (C–I, C–Br, C–S, double and triple bonds, azido group, etc.). Few examples of palladium-catalyzed methods tolerating bromoarenes and cyano-group were reported (Bae et al., 2000; Kalbasi and Mazaheri, 2016; Sharma et al., 2017). With silver and gold catalysts, bromoarene and alkene fragments are retained in the resulting amine product (Zhang et al., 2016; Artiukha et al., 2017).
Considerable efforts have been made toward finding non-noble metal catalysts to replace noble metals in the reductive amination of carbonyl compounds with nitro compounds. Supported catalysts based on nickel (Fiore et al., 2019; Li et al., 2019), cobalt (Stemmler et al., 2014; Jagadeesh et al., 2017; Senthamarai et al., 2018), copper (Nuzhdin et al., 2017), and iron (Natte et al., 2017) nanoparticles were developed. An important advantage of non-noble metal catalyzed reactions is their tolerance to C–I and C–S bonds, which undergo hydrogenolysis with palladium/platinum catalysts (Stemmler et al., 2014; Natte et al., 2017; Pedrajas et al., 2017; Fiore et al., 2019). However, harsh conditions (110–170°C and 50–70 bar H2), long reaction times (10–40 h), and high catalyst loadings (average TON 25÷50) are usually required with non-noble metal catalysts. Recently, Beller and Llusar reported that a well-defined molybdenum sulfide cluster Mo3S4 exhibits exceptionally high catalytic activity allowing for the performance of reductive benzylation and alkylation of aromatic and aliphatic nitro compounds at 70°C and 20–50 bar H2 with >99% conversion and high yields (Pedrajas et al., 2017). Unfortunately, this catalyst showed poor recyclability.
Although most of the catalytic protocols for reductive amination with nitroarenes were designed for batch reactors, flow methods using supported gold and silver catalysts have been reported in recent years (Artiukha et al., 2015, 2017).
Numerous studies were performed to replace hydrogen for other reducing agents, in particular those which are easy-to-use in the lab (Figure 1c). As an example, Rhee developed reductive amination of aldehydes with nitroarenes using ammonium formate over Pd/C catalyst (Byun et al., 2007). However, this method was not applicable to aromatic aldehydes. Cao and co-workers developed reductive amination of aldehydes with nitroarenes using formic acid as a transfer hydrogenation reagent and gold catalyst supported on rutile titania (Au/TiO2-R) under very mild conditions (80°C, 1 bar) in water (Zhang et al., 2016). Li and co-workers accomplished reductive benzylation of nitrobenzene with formic acid over Pd on graphitic carbon nitride (g-C3N4) catalyst at 100°C, yet the substrate scope was not studied (Xu et al., 2018). Zhou and Zhang successfully performed reductive alkylation of nitro compounds with HCOOH over Co-Nx catalyst (Zhou and Zhang, 2017) or Co nanoparticles embedded in mesoporous nitrogen-doped carbon (Jiang et al., 2017; Zhou et al., 2017b). Very recently, Chen and co-workers used N,S-codoped carbon shell-enclosed ultrafine Co nanoparticles to catalyze the same reaction (Guo et al., 2019). These Co-catalyzed transfer hydrogenation protocols are characterized by a broad functional group tolerance, yet harsh conditions were required (up to 190°C) to achieve full conversion. As a result, formylation of the amino group takes place as a side process in transfer hydrogenation processes with HCOOH at elevated temperatures (Guo et al., 2019).
Jagadeesh developed an efficient catalytic system based on a nitrogen-doped, graphene-activated nanoscale Co3O4-based catalyst with HCOOH-Et3N as a hydrogen source (Senthamarai et al., 2018). Importantly, not only aromatic and aliphatic aldehydes, but also formaldehyde could be involved in the reductive coupling with nitro compounds under these conditions. Transfer hydrogenation with a CO/H2O system with supported Co-nanoparticles (Zhou et al., 2017a) and cobalt-rhodium heterobimetallic nanoparticle catalysts (Park and Chung, 2015) was also used with a similar efficacy and substrate scope. Several reports deal with the use of NaBH4 as a mild, inexpensive, and safe reducing agent in the presence of nickel (Kalbasi and Mazaheri, 2015; Fiore et al., 2019) or palladium nanoparticles (Kalbasi and Mazaheri, 2016). Decaborane (B10H14) (Bae et al., 2000) and diboronic acid (B2(OH)4) (Zhou Y. et al., 2017) in combination with Pd/C catalyst were also shown to be convenient systems for reductive alkylation of nitroarenes with aromatic and aliphatic aldehydes. Non-catalytic methods utilizing zinc as a reducing agent have also been reported (Zou et al., 2007; Jiang et al., 2009; Wahba and Hamann, 2012; Lin et al., 2016), yet their discussion is beyond the scope of this short review.
Reductive alkylation of nitro compounds was shown to be a useful methodology for the synthesis of pharmacologically active ingredients and bioactive molecules (Figure 1d). Hamann et al. successfully used this strategy to synthesize modified natural molecules (alkaloids, sesquiterpenes, and steroids), possessing secondary and tertiary amino groups (Wahba and Hamann, 2012). In a similar fashion, Beller and co-workers utilized iron-catalyzed reductive methylation of nitro compounds to perform the post-modification of pharmaceuticals and fluorescent molecules (Natte et al., 2017).
Reductive Alkylation of Nitro Compounds in the Synthesis of Heterocycles: Characteristic Examples
The application of polyfunctionalized substrates in the reductive alkylation of nitro compounds provides a versatile access to useful saturated and partially saturated heterocyclic products (characteristic examples are shown in Figure 2). Thus, reductive coupling of o-dinitrobenzene with benzaldehyde over Au/TiO2-R catalyst afforded 2-phenylbenzimidazole in 87% yield as reported by Cao and co-authors (Zhang et al., 2016) (Figure 2a). Reductive benzylation of nitroarenes with 2-formylbenzoic acid produced isoindolinones upon catalysis by Au-Pd/Fe3O4 (Cho et al., 2018) (Figure 2b). This cascade transformation involves the hydrogenolysis of the nitro group, reductive amination reaction with the aldehyde, and subsequent lactamization.
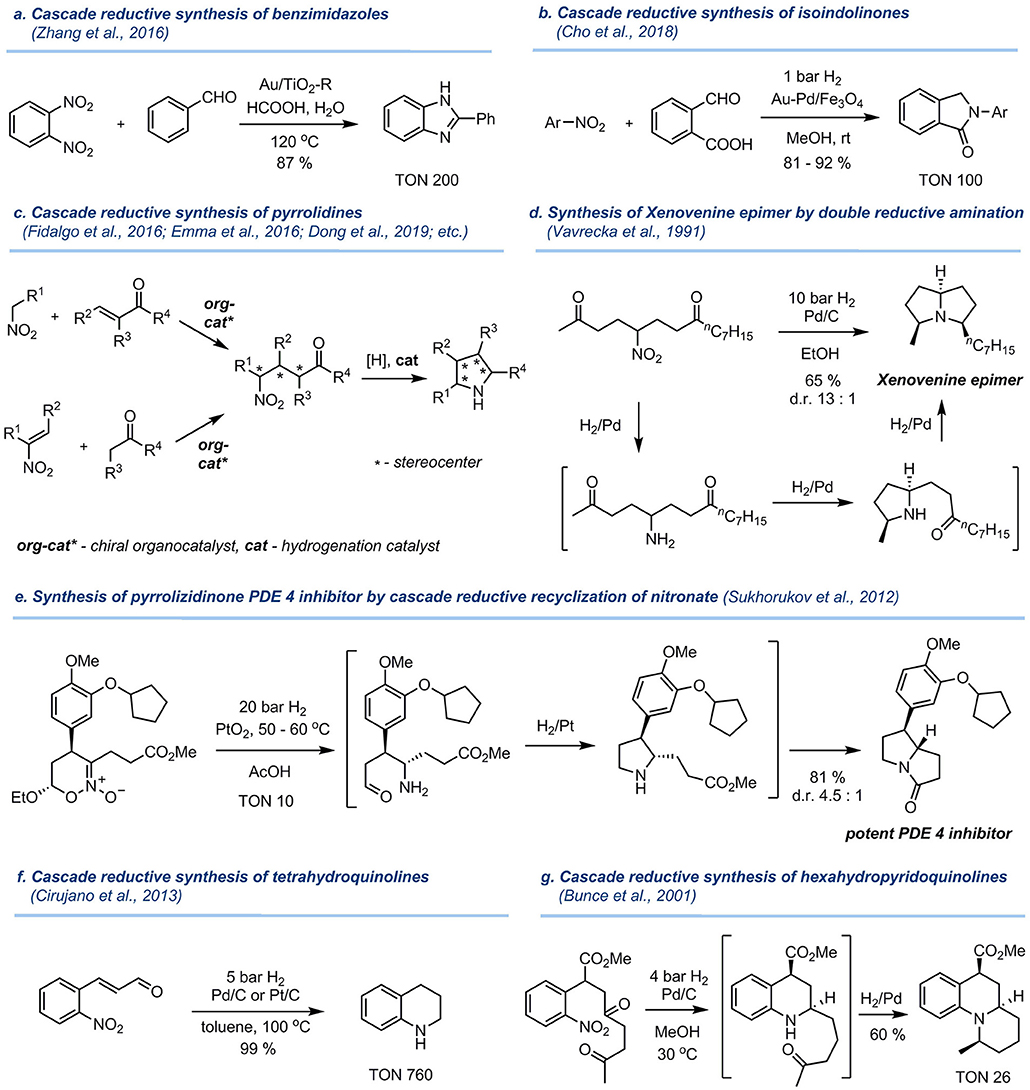
Figure 2. Examples of a cascade synthesis of bioactive heterocycles by reductive amination/cyclization involving nitro compounds. (a) Cascade reductive synthesis of benzimidazoles. (b) Cascade reductive synthesis of isoindolinones. (c) Cascade reductive synthesis of pyrrolidines. (d) Synthesis of Xenovenine epimer by double reductive amination. (e) Synthesis of pyrrolizidinone PDE 4 inhibitor by cascade reductive recyclization of nitronate. (f) Cascade reductive synthesis of tetrahydroquinolines. (g) Cascade reductive synthesis of hexahydropyridoquinolines.
Aliphatic γ-nitrocarbonyl compounds are of particular interest in this context, as their reduction results in the formation of polysubstituted pyrrolidines (Figure 2c), which are a highly important class of saturated heterocycles for drug development (Tasker et al., 1997; Jae et al., 2001; Dziki et al., 2006; Trost and Hisaindee, 2006; García-García et al., 2008; Ballini and Petrini, 2009; Dong et al., 2010, 2019; Xu et al., 2010; Cirujano et al., 2013; Corbett et al., 2014; Sasaki et al., 2014; Emma et al., 2016; Fidalgo et al., 2016). In most of these reactions, standard hydrogenation catalysts like Pd/C, Raney nickel and Adam's catalyst were used. The stereochemical outcome of the intramolecular reductive amination can be controlled by the nature of hydrogenation catalyst (Sukhorukov et al., 2012), albeit the diastereoselectivity is moderate in many cases (Zhu et al., 2010; Lu et al., 2012). The initial γ-nitrocarbonyl compounds are readily accessed by the Michael addition of nitroalkanes to α,β-unsaturated carbonyl compounds (Ballini et al., 2005) or by the addition of aldehydes/ketones to conjugated nitroalkenes (Barrett and Graboski, 1986; Berner et al., 2002) (Figure 2e). Importantly, by using asymmetric organocatalysis, γ-nitrocarbonyl compounds can be prepared in an enantioenriched form, thus allowing access to individual stereoisomers of final pyrrolidines (Sukhorukov et al., 2016; Alonso et al., 2017).
Introduction of a second carbonyl group allows the construction of saturated bicyclic systems containing two fused pyrrolidine units (Ballini and Petrini, 2009; O'Connell et al., 2012). For example, an epimer of alkaloid Xenovenine was synthesized in good yield and stereoselectivity by the catalytic hydrogenation of the γ-nitrodiketone over Pd/C (Vavrecka et al., 1991) (Figure 2d). In this process, two sequential reductive amination reactions lead to the formation of a fully unsymmetrically substituted tertiary amine motif. In another example shown in Figure 2e, the synthesis of a potent PDE4 inhibitor was accomplished by a catalytic cascade recyclization of a cyclic nitronate involving hydrogenolysis of NO2 group, intramolecular reductive amination forming a pyrrolidine ring, and subsequent lactamization (Sukhorukov et al., 2012).
Catalytic reduction of δ-nitrocarbonyl compounds results in the formation of a six-membered piperidine ring, which is a common motif in alkaloids and pharmaceuticals. Hydrogenation of 2-nitrocynnamaldehyde over Pt/C or Pd/C, shown in Figure 2f, afforded tetrahydroquinoline in quantitative yield at 100°C and 5 bar H2 (Cirujano et al., 2013). Bunce et al. (2001) developed the cascade synthesis of an angular-fused tricyclic amine by hydrogenation of the δ-nitrodicarbonyl compound over Pd-C catalyst (Figure 2g). Intriguingly, in this transformation two new stereogenic centers are formed and the target compound is obtained as a single stereoisomer in 60% yield.
Discussion and Outlook
Catalytic reductive amination of aldehydes and ketones with nitro compounds is a promising methodology for an efficient and green synthesis of secondary and tertiary amines. To date, numerous catalytic hydrogenation and transfer hydrogenation processes have been designed employing both noble and non-noble metal catalysts. Many of these methods are characterized with high levels of selectivity, broad substrate scope, and functional group tolerance, as well as relatively mild conditions. Reductive amination with nitro compounds was shown to be beneficial for application in the synthesis of various pharmacophore amines and saturated N-heterocycles both on laboratory and semi-industrial scales. Nevertheless, there are still many challenges to be addressed in this methodology. Some of these are listed below:
(1) Catalysts based on non-noble metals seem to be more efficient compared to noble metals in terms of selectivity and functional group tolerance. However, due to decreased activity and harsh conditions the use of non-noble metals catalysts in reductive alkylation of nitro compounds remains limited.
(2) The development of stereoselective methods (in particular, homogeneous catalytic systems) for reductive amination of prochiral ketones with nitro compounds is highly desirable for the application of this methodology in the synthesis of enantiopure active pharmaceutical ingredients.
(3) Selective synthesis of fully unsymmetrical tertiary amines by sequential reductive alkylation of nitro compounds is attractive, yet difficult to accomplish in an intramolecular variant.
(4) Further design of cascade cyclizations involving reductive amination with nitro compounds would greatly contribute to the synthesis of saturated N-heterocycles and polycyclic systems, which are highly relevant to drug development.
Thus, the development of new methods for catalytic reductive amination with nitro compounds can be anticipated in the near future.
Author Contributions
AS collected the references, summarized the material, and wrote the manuscript.
Funding
This work was supported by the Council on grants of the President of the Russian Federation (grant MD-1312.2020.3).
Conflict of Interest
The author declares that the research was conducted in the absence of any commercial or financial relationships that could be construed as a potential conflict of interest.
References
Abdel-Magid, A. F., Carson, K. G., Harris, B. D., Maryanoff, C. A., and Shah, R. D. (1996). Reductive amination of aldehydes and ketones with sodium triacetoxyborohydride. Studies on direct and indirect reductive amination procedures. J. Org. Chem. 61, 3849–3862. doi: 10.1021/jo960057x
Afanasyev, O. I., Kuchuk, E., Usanov, D. L., and Chusov, D. (2019). Reductive amination in the synthesis of pharmaceuticals. Chem. Rev. 119, 11857–11911. doi: 10.1021/acs.chemrev.9b00383
Alonso, D., Baeza, A., Chinchilla, R., Gómez, C., Guillena, G., Pastor, I., et al. (2017). Recent advances in asymmetric organocatalyzed conjugate additions to nitroalkenes. Molecules 22:895. doi: 10.3390/molecules22060895
Artiukha, E. A., Nuzhdin, A. L., Bukhtiyarova, G. A., and Bukhtiyarov, V. I. (2017). Flow synthesis of secondary amines over Ag/Al2O3 catalyst by one-pot reductive amination of aldehydes with nitroarenes. RSC Adv. 7, 45856–45861. doi: 10.1039/C7RA08986D
Artiukha, E. A., Nuzhdin, A. L., Bukhtiyarova, G. A., Zaytsev, S. Y., Plyusnin, P. E., Shubin, Y. V., et al. (2015). One-pot reductive amination of aldehydes with nitroarenes over an Au/Al2O3 catalyst in a continuous flow reactor. Catal. Sci. Technol. 5, 4741–4745. doi: 10.1039/C5CY00964B
Bae, J. W., Cho, Y. J., Lee, S. H., Yoon, C.-O. M., and Yoon, C. M. (2000). A one-pot synthesis of -alkylaminobenzenes from nitroaromatics: reduction followed by reductive amination using BH. Chem. Commun. 2000, 1857–1858. doi: 10.1039/b005194m
Ballini, R., Bosica, G., Fiorini, D., Palmieri, A., and Petrini, M. (2005). Conjugate additions of nitroalkanes to electron-poor alkenes: recent results. Chem. Rev. 105, 933–972. doi: 10.1021/cr040602r
Ballini, R., and Petrini, M. (2009). Nitroalkanes as key building blocks for the synthesis of heterocyclic derivatives. ARKIVOC 9, 195–223. doi: 10.3998/ark.5550190.0010.912
Barrett, A. G. M., and Graboski, G. G. (1986). Conjugated nitroalkenes: versatile intermediates in organic synthesis. Chem. Rev. 86, 751–762. doi: 10.1021/cr00075a002
Benchekroun-Mounir, N., Dugat, D., Gramain, J. C., and Husson, H. P. (1993). Stereocontrolled formation of octahydro-1H-pyrrolo[2,3-d]carbazoles by reductive cyclization: total synthesis of (±)-N-benzylaspidospermidine. J. Org. Chem. 58, 6457–6465. doi: 10.1021/jo00075a049
Berner, O. M., Tedeschi, L., and Enders, D. (2002). Asymmetric michael additions to nitroalkenes. Eur. J. Org. Chem. 2002, 1877–1894. doi: 10.1002/1099-0690(200206)2002:12<1877::AID-EJOC1877>3.0.CO;2-U
Bunce, R. A., Herron, D. M., Johnson, L. B., and Kotturi, S. V. (2001). Diastereoselective synthesis of substituted tetrahydroquinoline-4-carboxylic esters by a tandem reduction–reductive amination reaction. J. Org. Chem. 66, 2822–2827. doi: 10.1021/jo001761n
Byun, E., Hong, B., De Castro, K. A., Lim, M., and Rhee, H. (2007). One-pot reductive mono-N-alkylation of aniline and nitroarene derivatives using aldehydes. J. Org. Chem. 72, 9815–9817. doi: 10.1021/jo701503q
Chen, Y.-Z., Zhou, Y.-X., Wang, H., Lu, J., Uchida, T., Xu, Q., et al. (2015). Multifunctional PdAg@MIL-101 for one-pot cascade reactions: combination of host–guest cooperation and bimetallic synergy in catalysis. ACS Catal. 5, 2062–2069. doi: 10.1021/cs501953d
Cho, A., Byun, S., and Kim, B. M. (2018). AuPd–Fe3O4 nanoparticle catalysts for highly selective, one-pot cascade nitro-reduction and reductive amination. Adv. Synth. Catal. 360, 1253–1261. doi: 10.1002/adsc.201701462
Cirujano, F. G., Leyva-Pérez, A., Corma, A., and Llabrés i Xamena, F. X. (2013). MOFs as multifunctional catalysts: synthesis of secondary arylamines, quinolines, pyrroles, and arylpyrrolidines over bifunctional MIL-101. ChemCatChem 5, 538–549. doi: 10.1002/cctc.201200878
Corbett, M. T., Xu, Q., and Johnson, J. S. (2014). Trisubstituted 2-trifluoromethyl pyrrolidines via catalytic asymmetric michael addition/reductive cyclization. Org. Lett. 16, 2362–2365. doi: 10.1021/ol500679w
Del Pozo, C., Corma, A., Iglesias, M., and Sánchez, F. (2012). Multisite solid (NHC)NN-Ru-catalysts for cascade reactions: synthesis of secondary amines from nitro compounds. J. Catal. 291, 110–116. doi: 10.1016/j.jcat.2012.04.015
Dell'anna, M. M., Mastrorilli, P., Rizzuti, A., and Leonelli, C. (2011). One-pot synthesis of aniline derivatives from nitroarenes under mild conditions promoted by a recyclable polymer-supported palladium catalyst. Appl. Catal. A Gen. 401, 134–140. doi: 10.1016/j.apcata.2011.05.010
Dong, X., Yang, B., Hu, Y., He, Q., Wen, Q., Zhan, W., et al. (2019). Polyfluoro-Substituted Aromatic Heterocyclic Compound Derivative, Pharmaceutical Composition Containing Same, and Applications Thereof . Patent EP3575291 (A1) Paris: European Patent Office.
Dong, X.-Q., Teng, H.-L., Tong, M.-C., Huang, H., Tao, H.-Y., and Wang, C.-J. (2010). Organocatalytic asymmetric Michael addition of α-aryl cyclopentanones to nitroolefins for construction of adjacent quaternary and tertiary stereocenters. Chem. Commun. 46, 6840–6842. doi: 10.1039/c0cc01987a
Dziki, W., Lu, Z., Rasmussen, M. W., Wardrop, J., Zhane, G. G. Z., and Linton, C. L. (2006). Crystalline Form of a Drug. Patent US20060063825 Washington, DC: U.S. Patent and Trademark Office.
Emerson, W. S., and Mohrman, H. W. (1940). Secondary amines from nitro compounds. J. Am. Chem. Soc. 62, 69–70. doi: 10.1021/ja01858a015
Emerson, W. S., and Uraneck, C. A. (1941). Secondary and tertiary amines from nitro compounds. J. Am. Chem. Soc. 63, 749–751. doi: 10.1021/ja01848a029
Emma, M. G., Lombardo, M., Trombini, C., and Quintavalla, A. (2016). The organocatalytic α-fluorination of chiral γ-nitroaldehydes: the challenge of facing the construction of a quaternary fluorinated stereocenter. Eur. J. Org. Chem. 2016, 3223–3232. doi: 10.1002/ejoc.201600378
Fidalgo, J. D. V., Hu, C., Li, X., Lu, P., Mergo, W., Mutnick, D., et al. (2016). Quinolone Derivatives as Antibacterials. Patent WO2016020836 (A1). Geneva: World Intellectual Property Organization.
Fiore, A. M., Romanazzi, G., Dell'anna, M. M., Latronico, M., Leonelli, C., Mali, M., et al. (2019). Mild and efficient synthesis of secondary aromatic amines by one-pot stepwise reductive amination of arylaldehydes with nitroarenes promoted by reusable nickel nanoparticles. Mol. Catal. 476:110507. doi: 10.1016/j.mcat.2019.110507
García-García, P., Ladépêche, A., Halder, R., and List, B. (2008). Catalytic asymmetric Michael reactions of acetaldehyde. Angew. Chem. Int. Ed. 47, 4719–4721. doi: 10.1002/anie.200800847
Guo, H., Wang, B., Qiu, P., Gao, R., Sun, M., and Chen, L. (2019). N,S-codoped carbon shells embedded with ultrafine co NPs for reductive amination with formic acid. ACS Sustain. Chem. Eng. 7, 8876–8884. doi: 10.1021/acssuschemeng.9b00894
Hu, L., Cao, X., Ge, D., Hong, H., Guo, Z., Chen, L., et al. (2011). Ultrathin platinum nanowire catalysts for direct C-N coupling of carbonyls with aromatic nitro compounds under 1 bar of hydrogen. Chem. Eur. J. 17, 14283–14287. doi: 10.1002/chem.201100818
Huang, L., Wang, Z., Geng, L., Chen, R., Xing, W., Wang, Y., et al. (2015). Selective and recyclable rhodium nanocatalysts for the reductive N-alkylation of nitrobenzenes and amines with aldehydes. RSC Adv. 5, 56936–56941. doi: 10.1039/C5RA05243B
Jae, H.-S., Winn, M., Von Geldern, T. W., Sorensen, B. K., Chiou, W. J., Nguyen, B., et al. (2001). Pyrrolidine-3-carboxylic Acids as endothelin antagonists. 5. highly selective, potent, and orally active ETA antagonists. J. Med. Chem. 44, 3978–3984. doi: 10.1021/jm010237l
Jagadeesh, R. V., Murugesan, K., Alshammari, A. S., Neumann, H., Pohl, M.-M., Radnik, J., et al. (2017). MOF-derived cobalt nanoparticles catalyze a general synthesis of amines. Science 358:326. doi: 10.1126/science.aan6245
Jiang, L., Zhou, P., Zhang, Z., Jin, S., and Chi, Q. (2017). Synthesis of Secondary Amines from one-pot reductive amination with formic acid as the hydrogen donor over an acid-resistant cobalt catalyst. Indust. Eng. Chem. Res. 56, 12556–12565. doi: 10.1021/acs.iecr.7b03621
Jiang, X., Zhang, Y., Chan, A. S. C., and Wang, R. (2009). Highly Enantioselective synthesis of γ-nitro heteroaromatic ketones in a doubly stereocontrolled manner catalyzed by bifunctional thiourea catalysts based on dehydroabietic amine: a doubly stereocontrolled approach to pyrrolidine carboxylic acids. Org. Lett. 11, 153–156. doi: 10.1021/ol8025268
Kalbasi, R. J., and Mazaheri, O. (2015). Synthesis and characterization of hierarchical ZSM-5 zeolite containing Ni nanoparticles for one-pot reductive amination of aldehydes with nitroarenes. Catal. Commun. 69, 86–91. doi: 10.1016/j.catcom.2015.05.016
Kalbasi, R. J., and Mazaheri, O. (2016). Facile one-pot tandem reductive amination of aldehydes from nitroarenes over a hierarchical ZSM-5 zeolite containing palladium nanoparticles. New J. Chem. 40, 9627–9637. doi: 10.1039/C6NJ02262F
Li, J., Wang, B., Qin, Y., Tao, Q., and Chen, L. (2019). MOF-derived Ni@NC catalyst: synthesis, characterization, and application in one-pot hydrogenation and reductive amination. Catal. Sci. Technol. 9, 3726–3734. doi: 10.1039/C9CY00734B
Li, L., Niu, Z., Cai, S., Zhi, Y., Li, H., Rong, H., et al. (2013). A PdAg bimetallic nanocatalyst for selective reductive amination of nitroarenes. Chem. Commun. 49, 6843–6845. doi: 10.1039/c3cc00249g
Lin, C.-H., Hong, B.-C., and Lee, G.-H. (2016). Asymmetric synthesis of functionalized pyrrolizidines by an organocatalytic and pot-economy strategy. RSC Adv. 6, 8243–8247. doi: 10.1039/C5RA25103F
Lu, R.-J., Wei, W.-T., Wang, J.-J., Nie, S.-Z., Zhang, X.-J., and Yan, M. (2012). Organocatalytic conjugate addition of α-nitroacetates to β,γ-unsaturated α-keto esters and subsequent decarboxylation: synthesis of optically active δ-nitro-α-keto esters. Tetrahedron 68, 9397–9404. doi: 10.1016/j.tet.2012.09.014
Maeda, H., Selvakumar, N., and Kraus, G. A. (1999). An efficient synthesis of 4-aryl kainic acid analogs. Tetrahedron 55, 943–954. doi: 10.1016/S0040-4020(98)01101-6
Major, R. T. (1931). Catalytic reduction of mixtures of para-nitro- and nitrosophenols with aldehydes and ketones J. Am. Chem. Soc. 53, 1901–1908. doi: 10.1021/ja01356a042
Maurer, F., Rohe, L., and Knops, H.-J. (1998). Preparation of N-Alkyl-Arylamines. Patent US5817876A. Washington, DC: U.S. Patent and Trademark Office.
Natte, K., Neumann, H., Jagadeesh, R. V., and Beller, M. (2017). Convenient iron-catalyzed reductive aminations without hydrogen for selective synthesis of N-methylamines. Nat. Commun. 8:1344. doi: 10.1038/s41467-017-01428-0
Nişanci, B., Ganjehyan, K., Metin, Ö., Daştan, A., and Török, B. (2015). Graphene-supported NiPd alloy nanoparticles: A novel and highly efficient heterogeneous catalyst system for the reductive amination of aldehydes. J. Mol. Catal. A: Chem. 409, 191–197. doi: 10.1016/j.molcata.2015.08.022
Nuzhdin, A. L., Artiukha, E. A., Bukhtiyarova, G. A., Derevyannikova, E. A., and Bukhtiyarov, V. I. (2017). Synthesis of secondary amines by reductive amination of aldehydes with nitroarenes over supported copper catalysts in a flow reactor. Catal. Commun. 102, 108–113. doi: 10.1016/j.catcom.2017.09.001
O'Connell, K. M. G., Díaz-Gavilán, M., Galloway, W. R. J. D., and Spring, D. R. (2012). Two-directional synthesis as a tool for diversity-oriented synthesis: Synthesis of alkaloid scaffolds. Beilstein J. Org. Chem. 8, 850–860. doi: 10.3762/bjoc.8.95
Olah, G. A., and Malhotra, R. S. C. (2001). Narahg, Nitration: Methods and Mechanism. New York, NY: Wiley-VCH.
Orlandi, M., Brenna, D., Harms, R., Jost, S., and Benaglia, M. (2018). Recent developments in the reduction of aromatic and aliphatic nitro compounds to amines. Org. Proc. Res. Dev. 22, 430–445. doi: 10.1021/acs.oprd.6b00205
Park, J. W., and Chung, Y. K. (2015). Hydrogen-free cobalt-rhodium heterobimetallic nanoparticle-catalyzed reductive amination of aldehydes and ketones with amines and nitroarenes in the presence of carbon monoxide and water. ACS Catal. 5, 4846–4850. doi: 10.1021/acscatal.5b01198
Pedrajas, E., Sorribes, I., Junge, K., Beller, M., and Llusar, R. (2017). Selective reductive amination of aldehydes from nitro compounds catalyzed by molybdenum sulfide clusters. Green Chem. 19, 3764–3768. doi: 10.1039/C7GC01603D
Pintado-Sierra, M., Rasero-Almansa, A. M., Corma, A., Iglesias, M., and Sánchez, F. (2013). Bifunctional iridium-(2-aminoterephthalate)-Zr-MOF chemoselective catalyst for the synthesis of secondary amines by one-pot three-step cascade reaction. J. Catal. 299, 137–145. doi: 10.1016/j.jcat.2012.12.004
Sasaki, S., Suzuki, H., Ouchi, H., Asakawa, T., Inai, M., Sakai, R., et al. (2014). Practical synthesis of kainoids: a new chemical probe precursor and a fluorescent probe. Org. Lett. 16, 564–567. doi: 10.1021/ol403434e
Senthamarai, T., Murugesan, K., Natte, K., Kalevaru, N. V., Neumann, H., Kamer, P. C. J., et al. (2018). Expedient synthesis of N-methyl- and N-alkylamines by reductive amination using reusable cobalt oxide nanoparticles. ChemCatChem 10, 1235–1240. doi: 10.1002/cctc.201701617
Sharma, H., Bhardwaj, M., Kour, M., and Paul, S. (2017). Highly efficient magnetic Pd(0) nanoparticles stabilized by amine functionalized starch for organic transformations under mild conditions. Mol. Catal. 435, 58–68. doi: 10.1016/j.mcat.2017.03.019
Sreedhar, B., Reddy, P. S., and Devi, D. K. (2009). Direct one-pot reductive amination of aldehydes with nitroarenes in a domino fashion: catalysis by gum-acacia-stabilized palladium nanoparticles. J. Org. Chem. 74, 8806–8809. doi: 10.1021/jo901787t
Stemmler, T., Westerhaus, F. A., Surkus, A.-E., Pohl, M.-M., Junge, K., and Beller, M. (2014). General and selective reductive amination of carbonyl compounds using a core–shell structured Co3O4/NGr@C catalyst. Green Chem. 16, 4535–4540. doi: 10.1039/C4GC00536H
Sui, D., Mao, F., Fan, H., Qi, Z., and Huang, J. (2017). General reductive amination of aldehydes and ketones with amines and nitroaromatics under H2 by recyclable iridium catalysts. Chin. J. Chem. 35, 1371–1377. doi: 10.1002/cjoc.201600903
Sukhorukov, A. Y., Boyko, Y. D., Nelyubina, Y. V., Gerard, S., Ioffe, S. L., and Tartakovsky, V. A. (2012). Synthesis of PDE IVb inhibitors. 3. synthesis of (+)-, (–)-, and (±)-7-[3-(Cyclopentyloxy)-4-methoxyphenyl]hexahydro-3H-pyrrolizin-3-one via reductive domino transformations of 3-β-carbomethoxyethyl-substituted six-membered cyclic nitronates. J. Org. Chem. 77, 5465–5469. doi: 10.1021/jo300955n
Sukhorukov, A. Y., Sukhanova, A. A., and Zlotin, S. G. (2016). Stereoselective reactions of nitro compounds in the synthesis of natural compound analogs and active pharmaceutical ingredients. Tetrahedron 72, 6191–6281. doi: 10.1016/j.tet.2016.07.067
Sydnes, M. O., Kuse, M., and Isobe, M. (2008). Reductive monoalkylation of nitro aryls in one-pot. Tetrahedron 64, 6406–6414. doi: 10.1016/j.tet.2008.04.077
Tarasevich, V. A., and Kozlov, N. G. (1999). Reductive amination of oxygen-containing organic compounds. Russ. Chem. Rev. 68, 55–72. doi: 10.1070/RC1999v068n01ABEH000389
Tasker, A. S., Sorensen, B. K., Jae, H.-S., Winn, M., Von Geldern, T. W., Dixon, D. B., et al. (1997). Potent and selective non-benzodioxole-containing endothelin-a receptor antagonists. J. Med. Chem. 40, 322–330. doi: 10.1021/jm960077r
Trost, B. M., and Hisaindee, S. (2006). A heterodinuclear asymmetric catalyst for conjugate additions of α-hydroxyketones to β-substituted nitroalkenes. Org. Lett. 8, 6003–6005. doi: 10.1021/ol062485n
Vavrecka, M., Janowitz, A., and Hesse, M. (1991). Transformation of 4-nitroalkane-1,7-diones into pyrrolizidines. Tetrahedron Lett. 32, 5543–5546. doi: 10.1016/0040-4039(91)80079-L
Wahba, A. E., and Hamann, M. T. (2012). Reductive N-alkylation of nitroarenes: a green approach for the N-alkylation of natural products. J. Org. Chem. 77, 4578–4585. doi: 10.1021/jo300303d
Wei, S., Dong, Z., Ma, Z., Sun, J., and Ma, J. (2013). Palladium supported on magnetic nanoparticles as recoverable catalyst for one-pot reductive amination of aldehydes with nitroarenes under ambient conditions. Catal. Commun. 30, 40–44. doi: 10.1016/j.catcom.2012.10.024
Xu, X., Luo, J., Li, L., Zhang, D., Wang, Y., and Li, G. (2018). Unprecedented catalytic performance in amine syntheses via Pd/g-C3N4 catalyst-assisted transfer hydrogenation. Green Chemistry 20, 2038–2046. doi: 10.1039/C8GC00144H
Xu, Y., Matsunaga, S., and Shibasaki, M. (2010). syn-Selective Catalytic Asymmetric 1,4-addition of α-ketoanilides to nitroalkenes under dinuclear nickel catalysis. Org. Lett. 12, 3246–3249. doi: 10.1021/ol101185p
Yamane, Y., Liu, X., Hamasaki, A., Ishida, T., Haruta, M., Yokoyama, T., et al. (2009). One-pot synthesis of indoles and aniline derivatives from nitroarenes under hydrogenation condition with supported gold nanoparticles. Org. Lett. 11, 5162–5165. doi: 10.1021/ol902061j
Yan, G., and Yang, M. (2013). Recent advances in the synthesis of aromatic nitro compounds. Org. Biomol. Chem. 11, 2554–2566. doi: 10.1039/c3ob27354g
Yin, D., Li, C., Ren, H., Liu, J., and Liang, C. (2018). Gold-palladium-alloy-catalyst loaded UiO-66-NH2 for reductive amination with nitroarenes exhibiting high selectivity. Chem. Select 3, 5092–5097. doi: 10.1002/slct.201800740
Zhang, Q., Li, S.-S., Zhu, M.-M., Liu, Y.-M., He, H.-Y., and Cao, Y. (2016). Direct reductive amination of aldehydes with nitroarenes using bio-renewable formic acid as a hydrogen source. Green Chem. 18, 2507–2513. doi: 10.1039/C5GC02583D
Zhou, P., Yu, C., Jiang, L., Lv, K., and Zhang, Z. (2017a). One-pot reductive amination of carbonyl compounds with nitro compounds with CO/H2O as the hydrogen donor over non-noble cobalt catalyst. J. Catal. 352, 264–273. doi: 10.1016/j.jcat.2017.05.026
Zhou, P., and Zhang, Z. (2017). One-pot reductive amination of carbonyl compounds with nitro compounds by transfer hydrogenation over Co–Nx as catalyst. ChemSusChem 10, 1892–1897. doi: 10.1002/cssc.201700348
Zhou, P., Zhang, Z., Jiang, L., Yu, C., Lv, K., Sun, J., et al. (2017b). A versatile cobalt catalyst for the reductive amination of carbonyl compounds with nitro compounds by transfer hydrogenation. Appl. Catal. B Environ. 210, 522–532. doi: 10.1016/j.apcatb.2017.04.026
Zhou, X., Li, X., Jiao, L., Huo, H., and Li, R. (2015). Programmed Synthesis Palladium supported on Fe3O4@C: an efficient and heterogeneous recyclable catalyst for one-pot reductive amination of aldehydes with nitroarenes in aqueous reaction medium. Catal. Lett. 145, 1591–1599. doi: 10.1007/s10562-015-1552-1
Zhou, Y., Zhou, H., Liu, S., Pi, D., and Shen, G. (2017). Water as a hydrogen source in palladium-catalyzed reduction and reductive amination of nitroarenes mediated by diboronic acid. Tetrahedron 73, 3898–3904. doi: 10.1016/j.tet.2017.05.056
Zhu, S., Yu, S., Wang, Y., and Ma, D. (2010). Organocatalytic Michael Addition of aldehydes to protected 2-amino-1-nitroethenes: the practical syntheses of oseltamivir (tamiflu) and substituted 3-aminopyrrolidines. Angew. Chem. Int. Ed. 49, 4656–4660. doi: 10.1002/anie.201001644
Keywords: nitro compounds, reductive amination, secondary amines, heterogeneous catalysis, cyclizations, nitrogen heterocycles, pharmaceuticals
Citation: Sukhorukov AY (2020) Catalytic Reductive Amination of Aldehydes and Ketones With Nitro Compounds: New Light on an Old Reaction. Front. Chem. 8:215. doi: 10.3389/fchem.2020.00215
Received: 22 January 2020; Accepted: 09 March 2020;
Published: 15 April 2020.
Edited by:
Ming-Yu Ngai, Stony Brook University, United StatesReviewed by:
Michal Szostak, Rutgers, The State University of New Jersey, Newark, United StatesDiocletian Chusov, A. N. Nesmeyanov Institute of Organoelement Compounds (RAS), Russia
Copyright © 2020 Sukhorukov. This is an open-access article distributed under the terms of the Creative Commons Attribution License (CC BY). The use, distribution or reproduction in other forums is permitted, provided the original author(s) and the copyright owner(s) are credited and that the original publication in this journal is cited, in accordance with accepted academic practice. No use, distribution or reproduction is permitted which does not comply with these terms.
*Correspondence: Alexey Yu. Sukhorukov, sukhorukov@ioc.ac.ru