- 1College of Engineering and Technology, Southwest University, Chongqing, China
- 2Chongqing Electric Power Research Institute, State Grid Chongqing Electric Power Company, Chongqing, China
Oil-immersed power transformers are considered to be one of the most crucial and expensive devices used in power systems. Hence, high-performance gas sensors have been extensively explored and are widely used for detecting fault characteristic gases dissolved in transformer oil which can be used to evaluate the working state of transformers and thus ensure the reliable operation of power grids. Hitherto, as a typical n-type metal-oxide semiconductor, tungsten trioxide (WO3) has received considerable attention due to its unique structure. Also, the requirements for high quality gas detectors were given. Based on this, considerable efforts have been made to design and fabricate more prominent WO3 based sensors with higher responses and more outstanding properties. Lots of research has focused on the synthesis of WO3 nanomaterials with different effective and controllable strategies. Meanwhile, the various morphologies of currently synthesized nanostructures from 0-D to 3-D are discussed, along with their respective beneficial characteristics. Additionally, this paper focused on the gas sensing properties and mechanisms of the WO3 based sensors, especially for the detection of fault characteristic gases. In all, the detailed analysis has contributed some beneficial guidance to the exploration on the surface morphology and special hierarchical structure of WO3 for highly sensitive detection of fault characteristic gases in oil-immersed transformers.
Introduction
The safe and reliable operation of transformers is of vital importance for a stable and continuous power supply to the power grid (Lu et al., 2018; Zhang D. Z. et al., 2018; Zhang Q. Y. et al., 2018; Cui et al., 2019; Yang et al., 2019a,b). To date, the number of oil-immersed transformers accounts for more than 90% of the total number of power transformers, and the operating state of these power transformers will directly affect the condition of power systems (Zhou et al., 2016; Zhang X. X. et al., 2019). For a long-running transformer, partial overheating and partial discharge will lead to the decomposition of transformer oil into a variety of fault gases, namely hydrogen (H2), carbon monoxide (CO), carbon dioxide (CO2), methane (CH4), acetylene (C2H2), ethylene (C2H4), and ethane (C2H6) (Jin et al., 2017; Gao et al., 2019; Park et al., 2019; Wang J. X. et al., 2019). Hence, the detection of these fault characteristic gases has been extensively applied to diagnose early latent faults and evaluate the operation quality of oil-immersed transformers (Zhang et al., 2018a; Cui et al., 2019; Gui et al., 2019). In this respect, metal oxide semiconductor (MOS) gas sensors have attracted considerable attention due to their high-performance capability and wide range of applications for the detection of these fault characteristic gases in transformer oil (Zhou et al., 2013; Zhang Y. Z. et al., 2019).
Given this, various metal oxides have been investigated via different synthesis routes (Ge et al., 2017; Zhou et al., 2018a,b; Wei et al., 2019a). Of all the oxides, as a typical n-type metal-oxide semiconductor, WO3 has attracted a large amount interest due to its excellent physicochemical properties (Miao et al., 2015; Xu et al., 2019). To improve the performance of the gas sensors, sustainable efforts have been made to synthesize various nanostructures such as nanoparticles, nanorods, nanosheets, and nanoflowers (Wei et al., 2019b). Additionally, previous researchers have confirmed that these unique structures are closely related to its gas sensing properties (Yu et al., 2016). Therefore, the morphology controllable synthesis of different hierarchical WO3 nanostructures and the enhanced gas sensing performances thereof are of great importance to explore and discuss. In this review, we focus on the morphology controllable synthesis of hierarchical WO3 nanostructures including 0-dimensional (0-D), 1-dimensional (1-D), 2-dimensional (2-D), and 3-dimensional (3-D). In addition, the enhanced gas sensing performance and related mechanisms, especially the detection of the dissolved gases in transformer oil, have been introduced.
Synthesis, Sensor Fabrication and Measurement
Synthesis of WO3 Materials With Different Strategies
Up to now, various effective strategies have been proposed for preparing special surface morphologies and then fabricating WO3 based sensors with an enhanced gas sensing performance. Among these synthesis routes the template route, hydrothermal process, electrospinning method, and chemical deposition have all been widely used. Wang M. D. et al. (2019) synthesized three-dimensionally porous WO3 materials with different pore sizes via the template route, and they proposed a relationship between the pore size and the enhanced gas sensing performance. Gibot et al. (2011) reported the template synthesis of a highly specific surface area WO3 nanoparticle and discussed the surface properties, morphology and crystallographic structure in detail. Jin et al. (2019) developed different types of WO3 nanoparticles through a facile hydrothermal process and proposed the morphology controllable route of changing the proportion of the reagents. Cao and Chen (2017) used a facile CTAB (Hexadecyl trimethyl ammonium bromide)-assisted hydrothermal method to synthesize an urchin-like WO3 nanostructure, and a sensor based on this possessed an excellent gas sensing performance due to its special microstructure. Giancaterini et al. (2016) investigated the influence of thermal- and visible light-activation on the response of WO3 nanofibers via an electrospinning method. Jaroenapibal et al. (2018) presented the electrospinning synthesis of Ag-doped WO3 nanofibers and demonstrated an enhanced gas sensing mechanism.
Sensor Fabrication and Measurement
To investigate the gas sensing performances of the different morphologies of WO3 materials, the prepared samples are used to fabricate side-heated structures, the most common versions of which are known as planar and tubular configurations. As depicted in Figure 1A, both of the structures were composed of four parts: sensing materials, wires, electrodesm, and substrate. The sensing materials in the sensor structure are prepared by dissolving the obtained WO3 powders into a water-ethanol mixed solution. After forming a homogeneous slurry, the paste is coated onto an alumina ceramic substrate evenly to obtain a sensing film (Zhou et al., 2019a,b). The wires are used to connect the whole measuring circuit and the electrodes are used to measure the change in sensor resistance which directly reflects the performance of the fabricated sensor (Zhou et al., 2018a). The substrate is usually made of aluminum, which can provide reliable support for sensing materials (Zhou et al., 2018c,d).
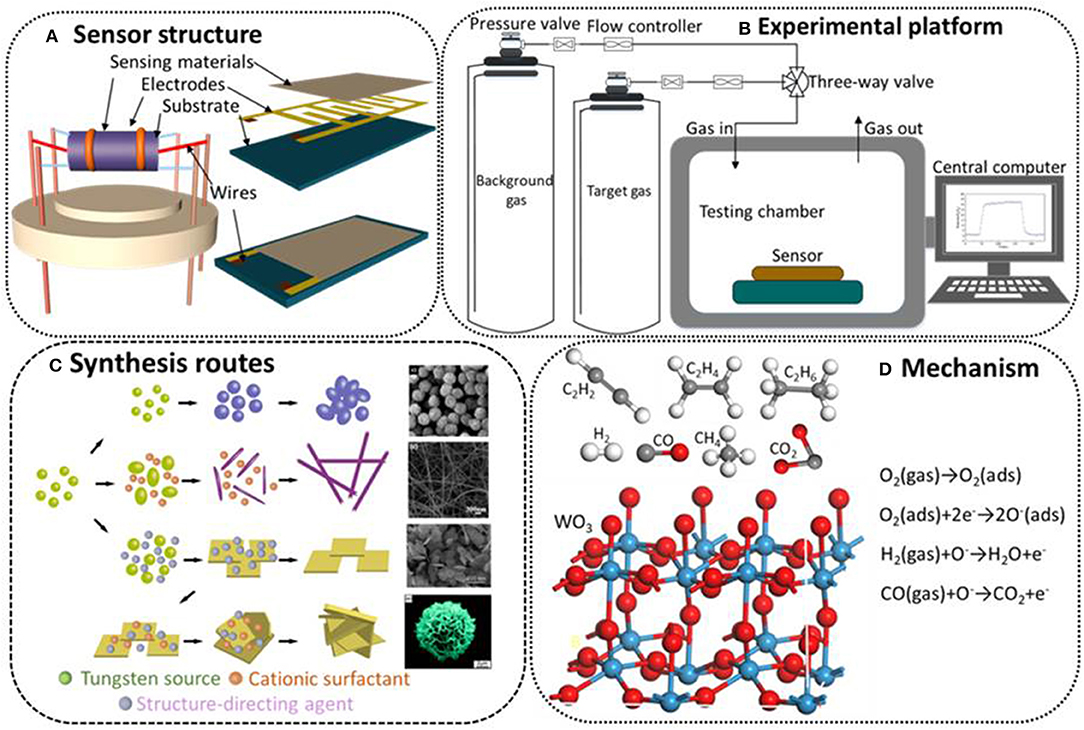
Figure 1. (A) Schematic diagram of sensor structures. (B) Schematic illustration of a gas sensing experimental platform. (C) Synthesis routes of different morphologies. Nanoparticles. Reprinted with permission from Kwon et al. Copyright (2010) American Chemical Society. Nanowires. Reprinted with permission from Wang et al. Copyright (2008) American Chemical Society. Nanosheets. Reprinted with permission from Zhang et al. Copyright (2015) American Chemical Society. Nanoflowers. Reprinted with permission from Liu et al. Copyright (2010) American Chemical Society. (D) Gas sensing mechanism.
The gas sensing properties of fabricated WO3 based sensors are investigated using a static intelligent gas sensing analysis platform. Figure 1B presents an example gas sensor experimental process. In this set up the background gas and target gas are alternately introduced into the gas chamber to measure the characteristic dynamic response and response-recovery rate of the prepared device. The flow controller is used to adjust the flux and speed of gases in order to control their concentrations. The fabricated sensors are installed in the testing chamber and the gas sensitivity data will be directly transmitted to the central computer for processing (Wei et al., 2019c).
Morphology Control From 0-D TO 3-D
In general, the change in sensor resistance caused by the redox reaction between oxygen molecules and test gas molecules is used to explain the basic operating principle of gas sensors. The surface morphology and special hierarchical microstructures have a crucial effect on the performance of gas sensors. In this respect, various morphologies from 0-D to 3-D with unique physical and chemical properties have been successfully synthesized and extensively explored via different effective strategies (Guo et al., 2015; Yao et al., 2015). Additionally, the controllable synthesis routes of WO3 nanostructures have been proposed to allow further investigation into how surface morphology affects gas sensing properties. As shown in Figure 1C, the four typical kinds of nanostructures, from 0-D to 3-D, can be controllably synthesized with different effective strategies. Given this, to further optimize the performance of WO3 based sensors for practical application, the exploration of surface morphology and special hierarchical structure is still a challenging but meaningful work.
0-Dimensional (0-D) WO3
As the lowest dimensional structure, 0-D WO3 has been investigated less as it is limited by its low specific surface area and insufficient porous structure. These disadvantages limit the diffusion and adsorption of target gas molecules during the sensing process, leading to unsatisfactory performances. Additionally, during the preparation of 0-D WO3 nanoparticles and the operation of the fabricated sensor, the coarsening and agglomeration of the nanoparticles might decrease the response of the device. However, various WO3 nanoparticles have been rationally designed and synthesized. Based on the defects mentioned above, WO3 nanoparticles with high dispersivity and ultra-small diameters might improve the performance of nanoparticle based sensors. In this respect, Li et al. (2019) synthesized highly dispersible WO3 nanoparticles with sizes ranging from 10 to 50 nm and they found the fabricated sensor exhibited an excellent gas sensing performance due to the highly effective surface area and sufficient oxygen vacancies.
1-Dimensional (1-D) WO3
1-D WO3 structures, for instance, nanorods, nanofibers, nanotubes, and nanowires, are considered to be beneficial nanostructures with improved special surface areas compared to. Also, the typical morphology has been applied to the detection the fault characteristic gases dissolved in transformer oil. Wisitsoorat et al. (2013) developed 1-D WO3 nanorods via a magnetron sputtering method, an H2 sensor based on which possessed prominent properties including a high response and fast response-recovery time. To further enhance the performance of 1-D WO3, the doping of metal ions and the introduction of surfactants have been confirmed to be effective strategies to improve the redox reaction and the orientation of special structures. Atomic platinum (Pt) is considered to be an effective doping element which can optimize the sensing properties and this strategy can be explained by the spillover effect of oxygen species and the enhancement of adsorption and desorption (Park et al., 2012).
2-Dimensional (2-D) WO3
Compared with low dimensional structures, 2-D structures possess a larger special surface area for the target gas molecules and therefore higher gas responses (Dral and ten Elshof, 2018). In comparison to the bulk 3-D structure, freestanding 2-D structures such as nanosheets, nanoplates, and thin films can provide better optimization routes including the modulation of the materials activity, surface polarization and rich oxygen vacancies. Additionally, the hierarchical microstructure assembled by rigid 2-D nanosheets possesses an open and well-defined structure which can promote the diffusion of target gas molecules (Nasir and Pumera, 2019). Especially in the field of the detection of fault characteristic gases in oil-immersed transformers, 2-D WO3 based sensors have been confirmed to be promising candidates with excellent gas sensing performances. Huang et al. (2020) synthesized Ru-loaded WO3 nanosheets via a facile impregnation method and they believed that the higher activity of surface lattice oxygens in WO3 nanosheets was activated by the introduction of Ru. Ou et al. (2012) fabricated H2 sensors based on WO3 nanoplates at different calcination temperatures and proved that the 2-D structure possesses a higher surface to volume ratio which clearly increased the number of surface interactive areas that could interact with H2 molecules.
3-Dimensional (3-D) WO3
Hierarchical 3-D structures are always assembled from diverse lower dimension fundamental blocks such nanoparticles, nanorods, and nanosheets. These various assembly routes make the hierarchical microstructures present different special morphologies, for instance, microspheres, microflowers, mesoporous structures, and other irregular structures. The well-defined structures always possess a larger special surface area and more unique microstructures, leading to better gas sensing performances including higher response times, more prominent selectivity, stability, and repeatability (Zhang et al., 2013). To detect fault characteristic gases, Zhang Y. X. et al. (2019) prepared a sea-urchin-like hexagonal WO3 structure created by the capping effect of potassium sulfate (which can prompt the anisotropic growth of WO3) and the H2 sensing performance was confirmed to benefit from the special hierarchical 3-D microstructure. Wei et al. (2017) synthesized hollow cauliflower-like WO3 by a facile hydrothermal process and found that the higher and faster response to CO might benefit from the hollow porous microstructure.
Gas Sensing Properties and Mechanism
To improve the performances of the detection of fault characteristic gases in oil-immersed transformers, WO3 based sensors with different hierarchical structures have been confirmed to be promising candidates for on-line monitoring of oil-immersed power transformers due to their excellent gas sensing properties. In this section, we summarize the related works based on the recently published investigations (Table 1) and propose a plausible gas sensing mechanism.
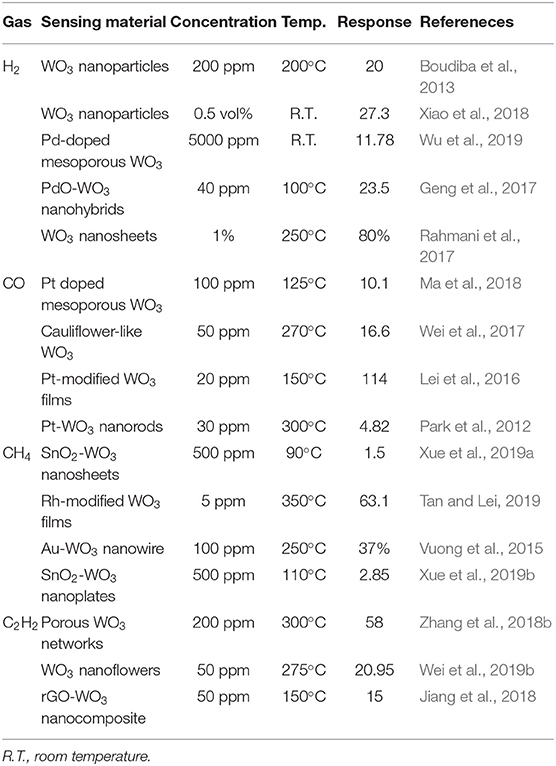
Table 1. Summary of recent researches on WO3 based sensors for sensing of fault characteristic gases dissolved in transformer oil.
The gas sensing mechanism of the WO3 based sensors can be demonstrated as the change in sensor resistance caused by the redox reaction between the oxygen species (mainly O−) and test gas molecules on the surface of synthesized materials, as shown in Figure 1D. For typical n-type WO3 based sensing materials, the oxygen molecules in the testing environment will be reduced and adsorbed on the surface of the materials by capturing the electrons from the conduction band, and the target gas molecules will react with the oxygen ions and release the electrons back to the conduction band. The involved reactions can be described as follows (H2 and CO gas are taken as examples):
Conclusion
In this mini review, we focus on the synthesis strategies, morphology control, sensing experimental procedures, and gas sensing performances of hierarchical WO3 structures from 0-D to 3-D. The gas sensing properties of various high-performance WO3 based sensors are summarized and discussed, especially in regards to the detection of fault characteristic gases dissolved in transformer oil. With an increasing requirement for high quality gas sensors with high responses, prominent selectivity, outstanding stability, and excellent repeatability, considerable efforts have been made to propose more effective synthesis routes, more beneficial morphology control and more accurate experiment processes. It can be foreseen that more and more hierarchical WO3 structures will be rationally designed and prepared due to their complicated microstructures with high special surface areas, broad internal contact area, and well-defined structures. These special hierarchical structures will provide more diffusion paths, reactive sites, and micro reaction spaces for target gas molecules adsorption, retention, and reaction. Although some achievements have been made by unremitting efforts, the further enhancement of the gas sensing properties of WO3 based sensors for practical applications is still a challenging but meaningful work. We hope that our work can contribute some beneficial guidance to the exploration of the surface morphology and special hierarchical structures of WO3. Additionally, much effort should be made to fabricate high-performance WO3 based sensors with predictably complicated hierarchical structures for detecting various gases, especially the fault characteristic gases dissolved in transformer oil.
Author Contributions
All authors listed have made a substantial, direct and intellectual contribution to the work, and approved it for publication.
Funding
This work has been supported in part by the National Natural Science Foundation of China (No. 51507144), Fundamental Research Funds for the Central Universities (No. XDJK2019B021), the Chongqing Science and Technology Commission (CSTC) (No. cstc2016jcyjA0400) and the project of China Scholarship Council (CSC).
Conflict of Interest
The authors declare that the research was conducted in the absence of any commercial or financial relationships that could be construed as a potential conflict of interest.
References
Boudiba, A., Roussel, P., Zhang, C., Olivier, M. G., Snyders, R., and Debliquy, M. (2013). Sensing mechanism of hydrogen sensors based on palladium-loaded tungsten oxide (Pd-WO3). Sensor. Actuator. B Chem. 187, 84–93. doi: 10.1016/j.snb.2012.09.063
Cao, S. X., and Chen, H. (2017). Nanorods assembled hierarchical urchin-like WO3 nanostructures: hydrothermal synthesis, characterization, and their gas sensing properties. J. Alloy Compo. 702, 644–648. doi: 10.1016/j.jallcom.2017.01.232
Cui, H., Zhang, X. X., Zhang, G. Z., and Tang, J. (2019). Pd-doped MoS2 monolayer: a promising candidate for DGA in transformer oil based on DFT method. Appl. Surf. Sci. 470, 1035–1042. doi: 10.1016/j.apsusc.2018.11.230
Dral, A. P., and ten Elshof, J. E. (2018). 2D metal oxide nanoflakes for sensing applications: review and perspective. Sensor Actuator B Chem. 272, 369–392. doi: 10.1016/j.snb.2018.05.157
Gao, X., Zhou, Q., Lu, Z. R., Xu, L. N., Zhang, Q. Y., and Zeng, W. (2019). Synthesis of Cr2O3 nanoparticle-coated SnO2 nanofibers and C2H2 sensing properties. Front. Mater. 6:163. doi: 10.3389/fmats.2019.00163
Ge, Q., Ma, S. Y., Xu, Y. B., Xu, X. L., Chen, H., Qiang, Z., et al. (2017). Preparation, characterization and gas sensing properties of Pr-doped ZnO/SnO2 nanoflowers. Mater. Lett. 191, 5–9. doi: 10.1016/j.matlet.2016.12.116
Geng, X., Luo, Y. F., Zheng, B. B., and Zhang, C. (2017). Photon assisted room-temperature hydrogen sensors using PdO loaded WO3 nanohybrids. Int. J. Hydrogen Energ. 42, 6425–6343. doi: 10.1016/j.ijhydene.2016.12.117
Giancaterini, L., Emamjomeh, S. M., De Marcellis, A., Palange, E., Resmini, A., Anselmi-Tamburini, U., et al. (2016). The influence of thermal and visible light activation modes on the NO2 response of WO3 nanofibers prepared by electrospinning. Sensor Actuator B Chem. 229, 387–395. doi: 10.1016/j.snb.2016.02.007
Gibot, P., Comet, M., Vidal, L., Moitrier, F., Lacroix, F., Suma, Y., et al. (2011). Synthesis of WO3 nanoparticles for superthermites by the template method from silica spheres. Solid State Sci. 13, 908–914. doi: 10.1016/j.solidstatesciences.2011.02.018
Gui, Y. G., Li, T., He, X., Ding, Z. Y., and Yang, P. G. (2019). Pt cluster modified h-BN for gas sensing and adsorption of dissolved gases in transformer oil: a Density Functional Theory Study. Nanomaterials 9:1746. doi: 10.3390/nano9121746
Guo, T., Yao, M. S., Lin, Y. H., and Nan, C. W. (2015). A comprehensive review on synthesis methods for transition-metal oxide nanostructures. CrystEngComm 17, 3551–3585. doi: 10.1039/C5CE00034C
Huang, D., Yuan, W. J., Fan, S. R., Tian, C., Hua, Z. Q., Tian, X. M., et al. (2020). Hydrogen sensing mechanism of Ru-loaded WO3 nanosheets. Sensor Actuator B Chem. 304:127339. doi: 10.1016/j.snb.2019.127339
Jaroenapibal, P., Boonma, P., Saksilaporn, N., Horprathum, M., Amornkitbamrung, V., and Triroj, N. (2018). Improved NO2 sensing performance of electrospun WO3 nanofibers with silver doping. Sensor Actuator B Chem. 255, 1831–1840. doi: 10.1016/j.snb.2017.08.199
Jiang, Z. K., Chen, W. G., Jin, L. F., Cui, F., Song, Z. H., and Zhu, C. Z. (2018). High performance acetylene sensor with heterostructure based on WO3 nanolamellae/reduced graphene oxide (rGO) nanosheets operating at low temperature. Nanomaterials 8. doi: 10.3390/nano8110909
Jin, L. F., Chen, W. G., Zhang, H., Xiao, G. W., Yu, C. T., and Zhou, Q. (2017). Characterization of reduced graphene oxide (rGO)-loaded SnO2 nanocomposite and applications in C2H2 gas detection. Appl. Sci. Basel 7:19. doi: 10.3390/app7010019
Jin, Z. W., Hu, P. F., Xu, W., Zhou, J., Guo, W. W., Chen, Y., et al. (2019). Hydrothermal synthesis and gas sensing properties of hybrid WO3 nano-materials using octadecylamine. J. Alloy. Compo. 785, 1047–1055. doi: 10.1016/j.jallcom.2019.01.248
Lei, T., Deng, Q., Zhang, S. P., Cai, S. Z., and Xie, C. S. (2016). Fast identification of CO by using single Pt-modified WO3 sensing film based on optical modulation. Sensor Actuator B Chem. 232, 506–513. doi: 10.1016/j.snb.2016.04.001
Li, T. T., Shen, Y. B., Zhao, S. K., Zhong, X. X., Zhang, W., Han, C., et al. (2019). Sub-ppm level NO2 sensing properties of polyethyleneimine-mediated WO3 nanoparticles synthesized by a one-pot hydrothermal method. J. Alloy. Compo. 783, 103–112. doi: 10.1016/j.jallcom.2018.12.287
Lu, Z. R., Zhou, Q., Xu, L. N., Gui, Y. G., Zhao, Z. Y., Tang, C., et al. (2018). Synthesis and characterization of highly sensitive hydrogen (H2) sensing device based on Ag doped SnO2 nanospheres. Materials 11:492. doi: 10.3390/ma11040492
Ma, J. H., Ren, Y., Zhou, X. R., Liu, L. L., Zhu, Y. H., Cheng, X. W., et al. (2018). Pt Nanoparticles sensitized ordered mesoporous WO3 semiconductor: gas sensing performance and mechanism study. Adv. Funct. Mater. 28:1705268. doi: 10.1002/adfm.201705268
Miao, B., Zeng, W., Mu, Y. J., Yu, W. J., Hussain, S., Xu, S. B., et al. (2015). Controlled synthesis of monodisperse WO3∙H2O square nanoplates and their gas sensing properties. Appl. Surf. Sci. 349, 380–386. doi: 10.1016/j.apsusc.2015.04.226
Nasir, M. Z. M., and Pumera, M. (2019). Emerging mono-elemental 2D nanomaterials for electrochemical sensing applications: from borophene to bismuthene. Trac Trend. Anal. Chem. 121:115696. doi: 10.1016/j.trac.2019.115696
Ou, J. Z., Yaacob, M. H., Campbell, J. L., Breedon, M., Kalantar-zadeh, K., and Wlodarski, W. (2012). H2 sensing performance of optical fiber coated with nano-platelet WO3 film. Sensor Actuator B Chem. 166, 1–6. doi: 10.1016/j.snb.2011.01.033
Park, M. S., Lee, J. H., Park, Y., Yoo, R., Park, S., Jung, H., et al. (2019). Doping effects of ZnO quantum dots on the sensitive and selective detection of acetylene for dissolved-gas analysis applications of transformer oil. Sensor Actuator B Chem. 299:126992. doi: 10.1016/j.snb.2019.126992
Park, S., Kim, H., Jin, C., Choi, S. W., Kim, S. S., and Lee, C. (2012). Enhanced CO gas sensing properties of Pt-functionalized WO3 nanorods. Thermochim. Acta 542, 69–73. doi: 10.1016/j.tca.2011.12.002
Rahmani, M. B., Yaacob, M. H., and Sabri, Y. M. (2017). Hydrogen sensors based on 2D WO3 nanosheets prepared by anodization. Sensor Actuator B Chem. 251, 57–64. doi: 10.1016/j.snb.2017.05.029
Tan, Y., and Lei, Y. (2019). Atomic layer deposition of Rh nanoparticles on WO3 thin film for CH4 gas sensing with enhanced detection characteristics. Ceram. Inter. 46, 9936–9942. doi: 10.1016/j.ceramint.2019.12.094
Vuong, N. M., Kim, D., and Kim, H. (2015). Porous Au-embedded WO3 nanowire structure for efficient detection of CH4 and H2S. Sci. Rep. 5:11040. doi: 10.1038/srep11040
Wang, J. X., Zhou, Q., Lu, Z. R., Wei, Z. J., and Zeng, W. (2019). A Gas sensing performances and mechanism at atomic level of Au-MoS2 microspheres. Appl. Surf. Sci. 490, 124–136. doi: 10.1016/j.apsusc.2019.06.075
Wang, M. D., Li, Y. Y., Yao, B. H., Zhai, K. H., Li, Z. J., and Yao, H. C. (2019). Synthesis of three-dimensionally ordered macro/mesoporous C-doped WO3 materials: effect of template sizes on gas sensing properties. Sensor Actuator B Chem. 288, 656–666. doi: 10.1016/j.snb.2019.03.051
Wei, S. H., Han, L. X., Wang, M. Y., Zhang, H. H., Du, W. M., and Zhou, M. H. (2017). Hollow cauliflower-like WO3 nano structures: Hydrothermal synthesis and their CO sensing properties. Mater. Lett. 186, 259–262. doi: 10.1016/j.matlet.2016.10.016
Wei, Z. J., Zhou, Q., Lu, Z. R., Xu, L. N., Gui, Y. G., and Tang, C. (2019b). Morphology controllable synthesis of hierarchical WO3 nanostructures and C2H2 sensing properties. Phys. E 109, 253–260. doi: 10.1016/j.physe.2019.01.006
Wei, Z. J., Zhou, Q., Wang, J. X., Gui, Y. G., and Zeng, W. (2019a). A novel porous NiO nanosheet and its H2 sensing performance. Mater. Lett. 245, 166–169. doi: 10.1016/j.matlet.2019.03.013
Wei, Z. J., Zhou, Q., Wang, J. X., Lu, Z. R., Xu, L. N., and Zeng, W. (2019c). Hydrothermal synthesis of SnO2 nanoneedle-anchored NiO microsphere and its gas sensing performances. Nanomaterials 9:1015. doi: 10.3390/nano9071015
Wisitsoorat, A., Ahmad, M. Z., Yaacob, M. H., Horpratum, M., Phakaratkul, D., Lomas, T., et al. (2013). Optical H2 sensing properties of vertically aligned Pd/ WO3 nanorods thin films deposited via glancing angle rf magnetron sputtering. Sensor Actuator B Chem. 182, 795–801. doi: 10.1016/j.snb.2013.03.091
Wu, C. H., Zhu, Z., Huang, S. Y., and Wu, R. J. (2019). Preparation of palladium-doped mesoporous WO3 for hydrogen gas sensors. J. Alloy Compo. 776, 965–973. doi: 10.1016/j.jallcom.2018.10.372
Xiao, S. H., Liu, B., Zhou, R., Liu, Z. W., Li, Q. H., and Wang, T. H. (2018). Room-temperature H2 sensing interfered by CO based on interfacial effects in palladium-tungsten oxide nanoparticles. Sensor Actuator B Chem. 254, 966–972. doi: 10.1016/j.snb.2017.07.169
Xu, Y., Zeng, W., and Li, Y. Q. (2019). A novel seawave-like hierarchical WO3 nanocomposite and its ammonia gas properties. Mater. Lett. 248, 86–88. doi: 10.1016/j.matlet.2019.04.010
Xue, D. P., Wang, J. J., Wang, Y., Sun, G., Cao, J. L., Bala, H., et al. (2019a). Enhanced methane sensing properties of WO3 nanosheets with dominant exposed (200) facet via loading of SnO2 nanoparticles. Nanomaterials 9:351. doi: 10.3390/nano9030351
Xue, D. P., Wang, Y., Cao, J. L., Sun, G., and Zhang, Z. Y. (2019b). Improving methane gas sensing performance of flower-like SnO2 decorated by WO3 nanoplates. Talanta 199, 603–611. doi: 10.1016/j.talanta.2019.03.014
Yang, Z., Zhou, Q., Wu, X. D., and Zhao, Z. Y. (2019b). A novel measuring method of interfacial tension of transformer oil combined PSO optimized SVM and multi frequency ultrasonic technology. IEEE Access 7, 182624–182631. doi: 10.1109/ACCESS.2019.2954899
Yang, Z., Zhou, Q., Wu, X. D., Zhao, Z. Y., Tang, C., and Chen, W. G. (2019a). Detection of water content in transformer oil using multi frequency ultrasonic with PCA-GA-BPNN. Energies 12:1379. doi: 10.3390/en12071379
Yao, M. S., Li, Q. H., Hou, G. L., Lu, C., Cheng, B. L., Wu, K. C., et al. (2015). Dopant-controlled morphology evolution of WO3 polyhedra synthesized by RF thermal plasma and their sensing properties. ACS Appl. Mater. Inter. 7, 2856–2866. doi: 10.1021/am5081277
Yu, Y. C., Zeng, W., Xu, M. X., and Peng, X. H. (2016). Hydrothermal synthesis of WO3∙H2O with different nanostructures from 0D to 3D and their gas sensing properties. Phys. E 79, 127–132. doi: 10.1016/j.physe.2015.12.023
Zhang, D. Z., Jiang, C. X., and Wu, J. F. (2018). Layer-by-layer assembled In2O3 nanocubes/flower-like MoS2 nanofilm for room temperature formaldehyde sensing. Sensor Actuator B Chem. 273, 176–184. doi: 10.1016/j.snb.2018.06.044
Zhang, H., Chen, W. G., Jin, L. F., and Cui, F. (2018b). Hierarchically porous WO3 microstructures with networks for acetylene sensing application. Mater. Lett. 214, 198–201. doi: 10.1016/j.matlet.2017.11.122
Zhang, H., Chen, W. G., Li, Y. Q., Jin, L. F., Cui, F., and Song, Z. H. (2018a). 3D Flower-like NiO hierarchical structures assembled with size-controllable 1D blocking units: gas sensing performances towards acetylene. Front. Chem. 6:472. doi: 10.3389/fchem.2018.00472
Zhang, H. B., Yao, M. S., Bai, L. Y., Xiang, W. C., Jin, H. C., Li, J. L., et al. (2013). Synthesis of uniform octahedral tungsten trioxide by RF induction thermal plasma and its application in gas sensing. CrystEngComm 15, 1432–1438. doi: 10.1039/c2ce26514a
Zhang, Q. Y., Zhou, Q., Lu, Z. R., Wei, Z. J., Xu, L. N., and Gui, Y. G. (2018). Recent advances of SnO2-based sensors for detecting fault characteristic gases extracted from power transformer oil. Front. Chem. 6:364. doi: 10.3389/fchem.2018.00364
Zhang, X. X., Fang, R. X., Chen, D. C., and Zhang, G. Z. (2019). Using Pd-doped gamma-graphyne to detect dissolved gases in transformer oil: a density functional theory investigation. Nanomaterials 9:1490. doi: 10.3390/nano9101490
Zhang, Y. Z., Zeng, W., and Li, Y. Q. (2019). NO2 and H2 sensing properties for urchin-like hexagonal WO3 based on experimental and first-principle investigations. Ceram. Inter. 45, 6043–6050. doi: 10.1016/j.ceramint.2018.12.075
Zhang, Y. X., Sun, X., Tan, S., Liu, T., and Cui, H. (2019). Adsorption characteristic of Rh-doped MoSe2 monolayer towards H2 and C2H2 for DGA in transformer oil based on DFT method. Appl. Surf. Sci. 487, 930–937. doi: 10.1016/j.apsusc.2019.05.108
Zhou, Q., Chen, W. G., Xu, L. N., Kumar, R., Gui, Y. G., Zhao, Z. Y., et al. (2018c). Highly sensitive carbon monoxide (CO) gas sensors based on Ni and Zn doped SnO2 nanomaterials. Ceram. Inter. 44, 4392–4399. doi: 10.1016/j.ceramint.2017.12.038
Zhou, Q., Chen, W. G., Xu, L. N., and Peng, S. D. (2013). Hydrothermal synthesis of various hierarchical ZnO nanostructures and their methane sensing properties. Sensors 13, 6171–6182. doi: 10.3390/s130506171
Zhou, Q., Hong, C. X., Yao, Y., Hussain, S., Xu, L. N., Zhang, Q. Y., et al. (2018d). Hierarchically MoS2 nanospheres assembled from nanosheets for superior CO gas-sensing properties. Mater. Res. Bull. 101, 132–139. doi: 10.1016/j.materresbull.2018.01.030
Zhou, Q., Lu, Z. R., Wei, Z. J., Xu, L. N., Gui, Y. G., and Chen, W. G. (2019b). Hydrothermal synthesis of hierarchical ultrathin NiO nanoflakes for high-performance CH4 sensing. Front. Chem. 6:194. doi: 10.3389/fchem.2018.00194
Zhou, Q., Tang, C., Zhu, S. P., Chen, W. G., and Li, J. (2016). Synthesis, characterisation and sensing properties of Sm2O3 doped SnO2 nanorods to C2H2 gas extracted from power transformer oil. Mater. Technol. 31, 364–370. doi: 10.1179/1753555715Y.0000000069
Zhou, Q., Umar, A., Sodki, E., Amine, A., Xu, L. N., Gui, Y. G., et al. (2018a). Fabrication and characterization of highly sensitive and selective sensors based on porous NiO nanodisks. Sensor Actuator B Chem. 259, 604–615. doi: 10.1016/j.snb.2017.12.050
Zhou, Q., Xu, L. N., Umar, A., Chen, W. G., and Kumar, R. (2018b). Pt nanoparticles decorated SnO2 nanoneedles for efficient CO gas sensing applications. Sensor Actuator B Chem. 256, 656–664. doi: 10.1016/j.snb.2017.09.206
Keywords: WO3, gas sensors, hierarchical structure, oil-immersed transformer, fault characteristic gas, mechanism
Citation: Wei Z, Xu L, Peng S and Zhou Q (2020) Application of WO3 Hierarchical Structures for the Detection of Dissolved Gases in Transformer Oil: A Mini Review. Front. Chem. 8:188. doi: 10.3389/fchem.2020.00188
Received: 11 January 2020; Accepted: 28 February 2020;
Published: 07 April 2020.
Edited by:
Mingshui Yao, Kyoto University, JapanReviewed by:
Yanqiong Li, Chongqing University of Arts and Sciences, ChinaRuixian Luo, Beijing University of Chemical Technology, China
Copyright © 2020 Wei, Xu, Peng and Zhou. This is an open-access article distributed under the terms of the Creative Commons Attribution License (CC BY). The use, distribution or reproduction in other forums is permitted, provided the original author(s) and the copyright owner(s) are credited and that the original publication in this journal is cited, in accordance with accepted academic practice. No use, distribution or reproduction is permitted which does not comply with these terms.
*Correspondence: Lingna Xu, lingnaxu@swu.edu.cn; Qu Zhou, zhouqu@swu.edu.cn