- 1Department of Chemistry, Nanjing Xiaozhuang University, Nanjing, China
- 2State Key Laboratory of Advanced Technology for Materials Synthesis and Processing, Wuhan University of Technology, Wuhan, China
Low-cost and highly effective catalysts are crucial to the electrocatalytic hydrogen evolution reaction (HER). Among non-noble catalysts, molybdenum carbides are promising candidates because of their high reserves, stability, low cost, and structural diversity. In this work, we report a simple method to fabricate a hollow porous Mo2C@C nanoball through a hydrothermal preparation process of molybdenum precursors at high temperatures. Specifically, we have combined interfacial polymerization and the chelation effect to synthesize the Mo-polydopamine (Mo-PDA) precursor. As a result, Mo2C@C-3 only requires an ultralow Tafel slope (~55 mV dec−1) and low overpotential (η50 ≈ 167 mV) in a 0.5 M H2SO4 solution with long-term cycling stability. Besides, it also exhibits outstanding activity and stability under extensive HER testing in alkaline media. This study is promising for the development of advanced molybdenum carbide electrocatalysts toward electrochemical applications.
Introduction
Hydrogen has been considered as a renewable, clean, and green energy source alternative to carbon-based fossil fuels for satisfying the ever-growing energy demand of the future (Huang C. et al., 2019; Tao et al., 2019; Takahashi et al., 2020). The hydrogen evolution reaction (HER) process is regarded as a best available strategy for producing high-purity hydrogen from abundant water (Huang C. et al., 2019; Jia et al., 2020). Platinum (Pt) and its alloys are commonly considered as benchmark catalysts for the HER, but the high cost largely impedes its commercial applications (Alinezhad et al., 2019; Park et al., 2019; Jia et al., 2020). Recently, earth-abundant catalysts, especially 3d transition metal compounds, have been reported (Li et al., 2017, 2018; Huang C. et al., 2019; Zhu et al., 2020. Among them, Molybdenum carbides (MoxC) are widely used catalysts that possess a similar electronic state of having noble Pt at the Fermi level, of which noble Pt is an ideal candidate as an inexpensive metal catalyst for the HER process (Huang C. et al., 2019; Zhu et al., 2019). However, until now, most of the MoxC catalysts are synthesized by the high carburization temperature method, which accelerates the agglomeration and sintering of MoxC nanomaterials, inhibiting the further improvement of electrocatalytic activity (Huang H. W. et al., 2019). Therefore, to obtain satisfactory electrocatalysis activity, developing an efficient strategy to construct advanced MoxC with a highly specific surface area and a variety of active sites remains a great challenge.
Nanocarbon hybridization has demonstrated impressive HER activities because it can effectively modify the electronic structure of catalysts, but their electrochemical accessibility is greatly depressed (Tang and Zhang, 2017; Wang Y. Q. et al., 2019; Zhang et al., 2020). Most importantly, during high-temperature thermal treatments, MoxC particles derived from those small precursors are likely to grow into larger particles, leading to poor catalytic activity. Thus, the design of a porous architecture can provide abundant active sites, resulting in significantly improved electrochemical performances (Park et al., 2018). Although substantial progress has been made, the controllable synthesis of hollow porous MoxC@C nanomaterials with high activity is still highly challenging.
Herein, we initiated an in situ strategy to synthesize a hollow porous Mo2C@C nanoball anchored on carbon substrates starting with a Mo-polydopamine (Scheme 1), and then investigated their trend in electrocatalytic activity for HER at different pH values. Consequently, the hollow porous Mo2C@C nanoball electrode exhibited remarkable electrocatalytic activity for HER in both acidic and alkaline conditions, which are superior to those of some recently reported Mo2C-based electrocatalysts and close to that of commercial 20% Pt/C. A detailed investigation revealed that the incorporation of carbon significantly reduces the agglomeration and induces strong electronic interaction between Mo2C and C, improving the electrical conductivity and resulting in an enhanced HER performance.
Results
As illustrated in Scheme 1, highly dispersed hollow porous Mo2C nanoballs anchored on carbon are obtained by a hydrothermal process of molybdenum precursors at high temperatures. Firstly, Mo reacts with dopamine hydrochloride, and different amounts of dopamine hydrochloride precursors are first mixed with an aqueous suspension of polydopamine (PDA)-coated Mo under vigorous stirring in the presence of ammonia, resulting in the precipitation of hollow porous materials. They are denoted as Mo-PD-X, where X refers to the mass of 3-hydroxytyramine in reactions. Meanwhile, the mass ratio of 1:2 for Mo: dopamine hydrochloride is the optimal condition. Then, the resulted precipitation of Mo-PD-X precursors is heated to 800°C in Ar and kept for 5 h to form Mo2C@C catalysts.
First of all, the morphology of the Mo-polymelamine precursor with varied 3-hydroxytyramine hydrochloride concentration (100, 200, and 300 mg) were observed by scanning electron microscope (SEM) and transmission electron microscope (TEM), and the corresponding pyrolyzed products anchored on carbon were obtained. Figures 1A–F presents representative SEM and TEM images of Mo-PD-X at different magnifications, which exhibit a similar structure to those of the hollow porous nanoball precursors. Further TEM observation (Figures 1E,F) reveals that the Mo-PD-3 sphere possesses an ultrathin shell composed of nanosheets about several nanometers in size, among which numerous mesopores are generated. Moreover, the outer layer is dendritic, which would endow abundant low-coordinated sites on the branch surface and electron transfer pathway (Zhong et al., 2018; Chen et al., 2019; Zhang et al., 2019). In addition, the thickness of the outer layer is controllable by varying the dopamine hydrochloride precursor amount.
The as-prepared hollow porous Mo-PD-X nanoballs were further annealed in Ar atmosphere at 800°C for 5 h to obtain hollow porous Mo2C@C nanoballs. In detail, the PDA inside the precursor was gradually decomposed and released from the PD-X cover. In the meantime, Mo reacted with PD-X, and then the Mo-based shell formed to self-support a hollow structure. The panoramic SEM and TEM images (Figures 2A,B) showed that the overall spherical morphology was well-preserved. Compared to the Mo-PD-X hollow nanoballs, the size of the hollow porous Mo2C@C nanoball shrank to ca. 500 nm. Furthermore, Figure 2C presents a high magnification TEM (HRTEM) image of Mo2C dendritic outer layers, and their lattice spacing (≈0.23 nm) was matched with (002) crystallographic planes of hexagonal Mo2C. Meanwhile, the carbon layer and porous channels of Mo2C/C can be clearly observed, indicating the formation of the charge-transfer pathway during the HER process. The carbon layer also can stabilize the hollow porous Mo2C cores to avoid deactivation and structure collapse during cyclic tests (Chen et al., 2016; Mir and Pandey, 2018; Zhu et al., 2018).
X-ray diffraction (XRD) (Figure 3A) and X-ray photoelectron spectroscopy (XPS) (Figure 3B) were also performed, and this certified a Mo2C/C nanostructure. From Figure 3A for Mo2C@C, besides the diffraction peaks from C, the other characteristic peaks are shown at 34.5, 38.0, 39.6, 52.3, 61.9, 69.8, 75.0, and 76.0°, which can only be ascribed to the hexagonal β -Mo2C phase (JCPDF#35-0781) (Mir and Pandey, 2018). Moreover, according to previous work, the value (ID/IG =) of Mo2C@C is 1.07, implying that more unordered carbon should increase with rich defects in Mo2C@C, which promotes the activity (Wang Y. H. et al., 2019). Considering the TEM, XRD, XPS, and SEM results together, we can conclude that the porous Mo2C nanoballs were formed in the graphitic carbon.
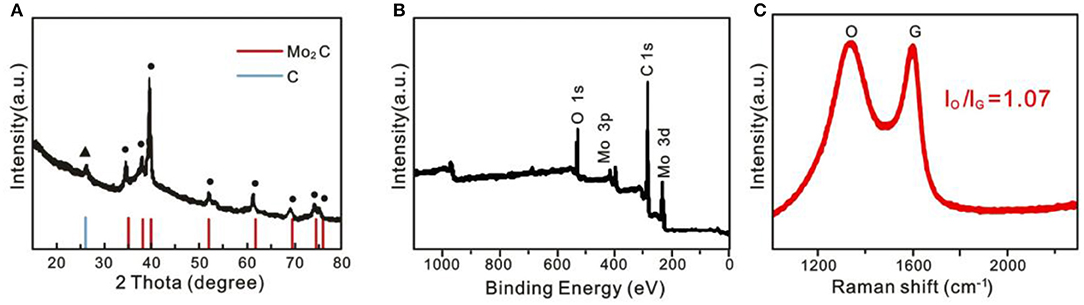
Figure 3. (A) XRD pattern, (B) XPS survey spectra, and (C) Raman spectra of hollow porous Mo2C@C nanoballs.
The electrocatalytic activity in the HER was evaluated by linear sweep voltammetry (LSV) in N2-saturated 0.5 M H2SO4 with a three-electrode electrochemical system (Chen et al., 2019; Liu et al., 2019a). For comparison, the HER activities of commercial Mo2C, commercial 20% Pt/C, Mo2C@C-1 (Mo-PD-1), and Mo2C@C-3 (Mo-PD-3) were also investigated, as shown in Figure 4A. Remarkably, the overpotential (η) for Mo2C@C-3 was small (only ≈ 129 mV) for achieving 10 mA cm−2, much lower than that for Mo2C@C-1 (271 mV) and commercial Mo2C (436 mV) catalysts. More significantly, the catalytic overpotential of Mo2C@C is lower than most non-noble metals based HER catalysts reported so far (Table S1). Meanwhile, the reaction kinetics and the rate-determining step for HER were further investigated by Tafel plots (Figure 4B and Table S1). Herein, the Tafel analysis of the Pt/C exhibited the lowest Tafel slope of 31 mV dec−1, suggesting that the Tafel reaction was the rate-limiting step (Xiang et al., 2018; Zheng et al., 2018). By comparison, the Tafel slope for Mo2C@C-3 (55 mV dec−1) was smaller than that for commercial Mo2C (125 mV dec−1) and Mo2C@C-1 (72 mV dec−1) catalysts, implying a faster HER rate. Additionally, the Mo2C@C-3 catalyst showed negligible change after 3,000 CV cycles (Figure 4C), reflecting superior durability in an acidic solution. The corresponding time-dependent potential curve (Figure 4D) further confirmed such stability. Clearly, the hollow porous Mo2C@C nanoball was a high performance HER catalyst in acidic solutions.
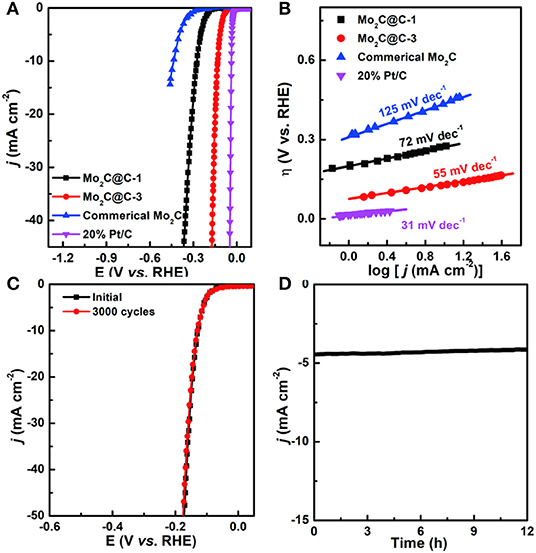
Figure 4. (A) LSV curves and (B) Tafel plots of Mo2C@C-1, Mo2C@C-3, 20% Pt/C, and commercial Mo2C in 0.5 M H2SO4 solution. (C) Cycling stability of Mo2C@C-3 before and after 3,000 cycles and (D) Galvanostatic data of the Mo2C@C-3 for 12 h in 0.5 M H2SO4 solution.
To broaden the application of catalysts, the HER performance of the samples prepared above in 1.0 M KOH aqueous solutions were also then examined by electrochemical measurements (Zheng et al., 2018; Liu et al., 2019b). Figure 5A shows the polarization curves of different samples after iR correction. Interestingly, Mo2C@C-3 also exhibits high activity under alkaline conditions with an overpotential of 115 mV, achieving a current density of 10 mA cm−2, which is much smaller than those of Mo2C@C-1 (191 mV) and commercial Mo2C (347 mV). Furthermore, the HER mechanism over these catalysts was studied by corresponding Tafel plots. As shown in Figure 5B, Mo2C@C-3 exhibits a low Tafel slope of 61 mV dec−1, indicating that the HER process occurs via the Volmer–Heyrovsky mechanism, and the electrochemical desorption (Heyrovsky step) is the rate-determining step (Zheng et al., 2018). Such superior activity is significantly better than or at least comparable with those reported for MoxC-based electrocatalysts (Table S2). Besides, the Mo2C@C-3 electrode also presented excellent durability in alkaline media (Figures 5C,D).
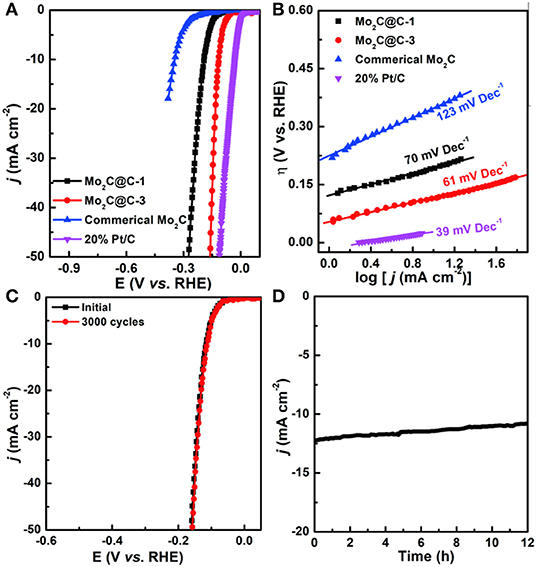
Figure 5. (A) LSV curves and Tafel plots of Mo2C@C-1, Mo2C@C-3, Pt/C, and commercial Mo2C in a 1.0 M KOH solution. (B) Tafel plots of Mo2C@C-1, Mo2C@C-3, 20% Pt/C, and commercial Mo2C in 1.0 M KOH solution. (C) LSV curves of Mo2C@C-3 before and after 3,000 CV cycles in a 1.0 M KOH solution. (D) Time-dependent current density curves of Mo2C@C-3 for 12 h.
Discussion
Significantly, the superior HER performance of hollow porous Mo2C@C nanoballs is highly correlated to the following aspects: (1) The hierarchical porous structure not only endows a high specific surface area and mitigates aggregation during carbonization process, but also facilitates efficient mass transfer of reactants and products, enhancing the HER performance (Park et al., 2018; Chen et al., 2019). (2) The presence of C not only prevents the aggregation of Mo-based compounds, but also accelerates the rate of charge transfer during HER (Chen et al., 2016; Zhu et al., 2018; Wang Y. H. et al., 2019; Wang Y. Q. et al., 2019).
In summary, hollow porous Mo2C nanoballs nested on carbon were fabricated by a in situ carbonization. The Mo2C@C-3 material was identified as low-cost and highly effective electrocatalysts for HER. It only needed overpotentials of 129 mV and 115 mV to drive a current density of 10 mA cm−2 in 0.5 M H2SO4 and 1 M KOH, respectively, and also exhibited robust catalytic stability for at least 12 h. This remarkable performance can be attributed to its unique hollow porous structure with carbon matrix. Undoubtedly, such a high-performance catalyst has promising potential to be commercialized in the future.
Data Availability Statement
All datasets generated for this study are included in the article/Supplementary Material.
Author Contributions
The original manuscript, figures, tables, and the Supplementary Materials were prepared by SLiu. The experimental data were prepared by XM. RC provided the original idea, helpful discussions, and the contribution in the manuscript revision. SM and CC conceived the idea. XM, RC, SLin, and YZ carried out the experiments. SLiu, XM, and RC analyzed the data.
Funding
This work was supported by the NSFC (21501096) and Natural Science Foundation of Jiangsu (BK20150086), Foundation of the Jiangsu Education Committee (15KJB150020), the Six Talent Peaks Project in Jiangsu Province (JY-087), Innovation Project of Jiangsu Province, Excellent Science and Technology Innovation Group of Jiangsu Province and Foundation of the Nanjing Xiaozhuang University (2019NXY20).
Conflict of Interest
The authors declare that the research was conducted in the absence of any commercial or financial relationships that could be construed as a potential conflict of interest.
Supplementary Material
The Supplementary Material for this article can be found online at: https://www.frontiersin.org/articles/10.3389/fchem.2020.00170/full#supplementary-material
References
Alinezhad, A., Gloag, L., Benedetti, T. M., Cheong, S., Webster, R. F., Roelsgaard, M., et al. (2019). Direct growth of highly strained Pt islands on branched Ni nanoparticles for improved hydrogen evolution reaction activity. J. Am. Chem. Soc. 141, 16202–16207. doi: 10.1021/jacs.9b07659
Chen, R. L., He, H. L., Pu, Z. H., Amiinu, I. S., Chen, L., Wang, Z., et al. (2019). Shrunken hollow Mo-N/Mo-C nanosphere structure for efficient hydrogen evolution in a broad pH range. Electrochim. Acta 298, 799–805. doi: 10.1016/j.electacta.2018.12.128
Chen, Y. Y., Zhang, Y., Jiang, W. J., Zhang, X., Dai, Z., Wan, L. J., et al. (2016). Pomegranate-like N,P-doped Mo2C@C nanospheres as highly active electrocatalysts for alkaline hydrogen evolution. ACS Nano 10, 8851–8860. doi: 10.1021/acsnano.6b04725
Huang, C., Miao, X. W., Pi, C. R., Gao, B., Zhang, X. M., Qin, P., et al. (2019). Mo2C/VC heterojunction embedded in graphitic carbon network: an advanced electrocatalyst for hydrogen evolution. Nano Energy 60, 520–526. doi: 10.1016/j.nanoen.2019.03.088
Huang, H. W., Yu, C., Huang, H. L., Guo, W., Zhang, M. D., Han, X. T., et al. (2019). Microwave-assisted ultrafast synthesis of molybdenum carbide nanoparticles grown on carbon matrix for efficient hydrogen evolution reaction. Small Methods 3:1900259. doi: 10.1002/smtd.201900259
Jia, W., Zhang, J., Lu, Z., Wang, S., and Feng, S. (2020). Pt decorated POMOF-derived constructions for efficient electrocatalytic hydrogen evolution. Nanoscale 12, 3902–3906. doi: 10.1039/C9NR08947K
Li, T. F., Luo, G., Liu, K. H., Li, X., Sun, D. M., Xu, L., et al. (2018). Encapsulation of Ni3Fe nanoparticles in N-doped carbon nanotube-grafted carbon nanofibers as high-efficiency hydrogen evolution electrocatalysts. Adv. Funct. Mater. 28:1805828. doi: 10.1002/adfm.201805828
Li, Y., Wang, Y., Pattengale, B., Yin, J., An, L., Cheng, F., et al. (2017). High-index faceted CuFeS2 nanosheets with enhanced behavior for boosting hydrogen evolution reaction. Nanoscale 9, 9230–9237. doi: 10.1039/C7NR03182C
Liu, S. L., Chen, C., Zhang, Y. F., Zheng, Q. H., Zhang, S. D., Mu, X. Q., et al. (2019a). Vacancy-coordinated hydrogen evolution reaction for MoO3−x anchored atomically dispersed MoRu Pairs. J. Mater. Chem. A 7, 14466–14472. doi: 10.1039/C9TA03719E
Liu, S. L., Mu, X. Q., Li, W. Q., Lv, M., Chen, B. Y., Chen, C. Y., et al. (2019b). Cation vacancy-modulated PtPdRuTe five-fold twinned nanomaterial for catalyzing hydrogen evolution reaction. Nano Energy 61, 346–351. doi: 10.1016/j.nanoen.2019.04.086
Mir, R. A., and Pandey, O. P. (2018). Influence of graphitic/amorphous coated carbon on HER activity of low temperature synthesized β-Mo2C@C nanocomposites. Chem. Eng. J. 348, 1037–1048. doi: 10.1016/j.cej.2018.05.041
Park, J., Kwon, T., Kim, J., Jin, H., Kim, H. Y., Kim, B., et al. (2018). Hollow nanoparticles as emerging electrocatalysts for renewable energy conversion reactions. Chem. Soc. Rev. 47, 8173–8202. doi: 10.1039/C8CS00336J
Park, J., Lee, S., Kim, H. E., Cho, A., Kim, S., Ye, Y. J., et al. (2019). Investigation of the support effect in atomically dispersed Pt on WO3−−x for utilization of Pt in the hydrogen evolution reaction. Angew. Chem. Int. Ed. 58, 16038–16042. doi: 10.1002/anie.201908122
Takahashi, Y., Kobayashi, Y., Wang, Z. Q., Ito, Y., Ota, M., Ida, H., et al. (2020). High-resolution electrochemical mapping of the hydrogen evolution reaction on transition-metal dichalcogenide nanosheets. Angew. Chem. Int. Ed. 59, 3601–3608. doi: 10.1002/anie.201912863
Tang, C., and Zhang, Q. (2017). Nanocarbon for oxygen reduction electrocatalysis: dopants, edges, and defects. Adv. Mater. 29:1604103. doi: 10.1002/adma.201604103
Tao, L., Qiao, M., Jin, R., Li, Y., Xiao, Z., Wang, Y., et al. (2019). Bridging surface charge and catalytic activity of defective carbon electrocatalyst. Angew. Chem. Int. Ed. 58, 1019–1024. doi: 10.1002/anie.201810207
Wang, Y. H., Han, X. J., Xu, P., Liu, D. W., Cui, L. R., Zhao, H. H., et al. (2019). Synthesis of pomegranate-like Mo2C@C nanospheres for highly efficient microwave absorption. Chem. Eng. J. 372, 312–320. doi: 10.1016/j.cej.2019.04.153
Wang, Y. Q., Xie, Y., Zhao, L., Sui, X. L., Gu, D. M., and Wang, Z. B. (2019). Hierarchical heterostructured Mo2C/Mo3Co3C bouquet-like nanowire arrays: an effcient electrocatalyst for hydrogen evolution reaction. ACS Sustain. Chem. Eng. 7, 7294–7303. doi: 10.1021/acssuschemeng.9b00358
Xiang, Z. P., Deng, H. Q., Peljo, P., Fu, Z. Y., Wang, S. L., Mandler, D., et al. (2018). Electrochemical dynamics of a single platinum nanoparticle collision event for the hydrogen evolution reaction. Angew. Chem. Int. Ed. 57, 3464–3468. doi: 10.1002/anie.201712454
Zhang, J., Jiang, Y., Shi, S., Li, H., Chen, J., Kuang, Q., et al. (2019). Hollow porous rhodium nanoballs. Chem. Commun. 55, 4989–4992. doi: 10.1039/C9CC01572H
Zhang, L. H., Shi, Y., Wang, Y., and Shiju, N. R. (2020). Nanocarbon catalysts: recent understanding regarding the active sites. Adv. Sci. 7:2070028. doi: 10.1002/advs.202070028
Zheng, Y., Jiao, Y., Vasileff, A., and Qiao, S. Z. (2018). The Hydrogen evolution reaction in alkaline solution: from theory, single crystal models, to practical electrocatalysts. Angew. Chem. Int. Ed. 57, 7568–7579. doi: 10.1002/anie.201710556
Zhong, H. X., Zhang, Y., and Zhang, X. B. (2018). Superior oxygen reduction electrocatalyst: hollow porous spinel microsphere. Chem 4, 196–198. doi: 10.1016/j.chempr.2018.01.015
Zhu, J., Hu, L., Zhao, P., Lee, L. Y. S., and Wong, K. Y. (2020). Recent advances in electrocatalytic hydrogen evolution using nanoparticles. Chem. Rev. 120, 851–918. doi: 10.1021/acs.chemrev.9b00248
Zhu, J., Yao, Y., Chen, Z., Zhang, A., Zhou, M., Guo, J., et al. (2018). Controllable synthesis of ordered mesoporous Mo2C@graphitic carbon core-shell nanowire arrays for efficient electrocatalytic hydrogen evolution. ACS Appl. Mater. Interfaces. 10, 18761–18770. doi: 10.1021/acsami.8b04528
Keywords: molybdenum carbide, mo-polydopamine, porous nanostructures, electrocatalyst, hydrogen evolution reaction
Citation: Liu S, Mu X, Cheng R, Lin S, Zhu Y, Chen C and Mu S (2020) In situ Engineering of Hollow Porous Mo2C@C Nanoballs Derived From Giant Mo-Polydopamine Clusters as Highly Efficient Electrocatalysts for Hydrogen Evolution. Front. Chem. 8:170. doi: 10.3389/fchem.2020.00170
Received: 30 January 2020; Accepted: 25 February 2020;
Published: 07 April 2020.
Edited by:
Quanbing Liu, Guangdong University of Technology, ChinaReviewed by:
Bao Yu Xia, Huazhong University of Science and Technology, ChinaMingwang Shao, Soochow University, China
Luhua Jiang, Qingdao University of Science and Technology, China
Yafei Li, Nanjing Normal University, China
Copyright © 2020 Liu, Mu, Cheng, Lin, Zhu, Chen and Mu. This is an open-access article distributed under the terms of the Creative Commons Attribution License (CC BY). The use, distribution or reproduction in other forums is permitted, provided the original author(s) and the copyright owner(s) are credited and that the original publication in this journal is cited, in accordance with accepted academic practice. No use, distribution or reproduction is permitted which does not comply with these terms.
*Correspondence: Changyun Chen, Y2hlbmNoYW5neXVuNTUwJiN4MDAwNDA7Z21haWwuY29t; Shichun Mu, bXNjJiN4MDAwNDA7d2h1dC5lZHUuY24=
†These authors have contributed equally to this work