- 1Engineering Research Center of None-food Biomass Efficient Pyrolysis and Utilization Technology of Guangdong Higher Education Institutes, Dongguan University of Technology, Dongguan, China
- 2Key Laboratory of Distributed Energy Systems of Guangdong Province, School of Chemical Engineering and Energy Technology, Dongguan University of Technology, Dongguan, China
- 3Guangzhou Higher Education Mega Center, School of Chemistry and Chemical Engineering, Guangzhou University, Guangzhou, China
In this paper, ferric nitrate was used to oxidize benzyl alcohol in a mild condition and demonstrated its better performance compared to HNO3. In the reaction, the conversion rate and product selectivity could be both as high as 95% in N2 atmosphere, while the benzaldehyde yield also reached 85% in air. Similar to Fe(NO3)3·9H2O, the other metallic nitrates such as Al(NO3)3·9H2O and Cu(NO3)2·3H2O could also oxidize the benzyl alcohol with high activity. The applicability of Fe(NO3)3·9H2O for other benzylic alcohol was also investigated, and the reaction condition was optimized at the same time. The results showed the Fe(NO3)3·9H2O would be more conducive in oxidizing benzyl alcohol under the anaerobic condition. The experiments in N2 or O2 atmospheres were conducted separately to study the catalytic mechanism of Fe(NO3)3. The results showed the co-existence of Fe3+ and will generate high activity, while either was with negligible oxidation property. The cyclic transformation of Fe3+ and Fe2+ provided the catalytic action to the benzyl alcohol oxidation. The role of was also an oxidant, by providing HNO2 in anaerobic condition, while would be regenerated from NO in aerobic condition. O2 did not oxidize the benzyl alcohol conversion directly, while it could still be beneficial to the procedure by eliminating the unwelcome NO and simultaneously reinforcing the circulation of Fe2+ and Fe3+, which therefore forms a green cyclic oxidation. Hence, the benzyl alcohol oxidation was suggested in an air atmosphere for efficiency and the need of green synthesis.
Introduction
Benzaldehyde (BzH) is one of the most important chemicals among the aromatic aldehyde family. It is used as the raw material for a large number of products, including perfume, beverage, pharmaceutical intermediates, and so on (Jachuck et al., 2006; Ragupathi et al., 2015; Ndolomingo and Meijboom, 2017; Zhu et al., 2017). Traditionally, BzH was synthesized by hydrolysis of benzal chloride or vapor/liquid-phase oxidation of toluene. In the former method, the chlorinated by-products and corresponding toxic acidic would be generated, which brought troubles to the industrial application (Mal et al., 2018; Lu et al., 2019), while the vapor/liquid oxidation of toluene was also limited because of the harsh reaction conditions and low selectivity (Miao et al., 2016). Recently, BzH production with benzyl alcohol oxidation was widely adopted in industry, based on its advantages of easy-control condition and high yield (Lv et al., 2018; Thao et al., 2018). In this method, potassium permanganate (KMnO4) (Mahmood et al., 1999) and dichromate (K2Cr2O7) (Thottathil et al., 1986) with a strong oxidizing property are chosen as oxidants. While those oxidants were not perfect industrial reagents, leading to a series of environmental issues and high cost.
Nitric acid (HNO3), as a rather inexpensive and high-performance oxidant, is commonly used in industry (Joshi et al., 2005; Aellig et al., 2012). For example, the niacin (vitamin B3) is synthesized from substituted pyridines oxidized by HNO3 (Yu et al., 2011). HNO3 is also regarded as the initiator in benzyl alcohol oxidation in the presence of O2 (Miao et al., 2011; Luo et al., 2012, 2014). In this approach, HNO3 initiates the oxidation of alcohols by decomposing NO2, which further formed HNO2 with H2O. HNO2 subsequently attacks substrate and generates the products by a series of reactions with releasing NOx. Finally, the HNO3 is regenerated by the NOx oxidation by O2. However, the disadvantages of HNO3 should not be ignored totally, due to the risks of pollution and corrosion. Green oxidants such as hydrogen peroxide (H2O2) (Cánepa et al., 2017) and O2 (Yu et al., 2011; Cao et al., 2013, 2015; Zhu et al., 2017; Chen et al., 2018a,b; Yuan et al., 2018) have been attracting extensive attention for many years. It is noted that O2 or H2O2 itself has almost no activity and its oxidative performance needs to be activated by other materials.
Besides oxidants, the catalytic systems, including homogeneous and heterogeneous, have been developed. In the past decade, the heterogeneous precious metals catalysts, like Au (Zhan et al., 2012; Albadi et al., 2014), Pt (Liu et al., 2017), Ru (Ganesamoorthy et al., 2013), and Pd (Villa et al., 2010), were employed for selective oxidation of benzyl alcohols to BzH, based on their excellent performances. However, the high cost and limited resource of noble metals hindered their practical application. Moreover, the catalytic activity of heterogeneous catalysts would be lower than their homogeneous counterparts after several recycles (Parmeggiani and Camilla, 2012). Hence, the metal-based homogeneous catalysts, including Ru (Shimizu et al., 2005), V (Hanson et al., 2008), Cu (Hansen et al., 2013; Jia et al., 2014), and Fe (Jiang et al., 2016; Li et al., 2016; Miao et al., 2016; Hu et al., 2018), continued to gain great interests.
Among those homogenous catalysts, non-toxic, abundant, and bio-friendly, iron-based metals have widely aroused attentions (Martin and Suárez, 2002; Wang et al., 2005; Zhang et al., 2013; Hu et al., 2016, 2018). Zhang and co-workers reported a series of Fe catalysts, such as FeBr3, Fe2O3, and Fe2(SO4)3, with performance catalyzing benzylamine to imine in air atmosphere (Zhang et al., 2013). Wang et al. (2005) found an effective FeCl3/TEMPO/NaNO2 catalyst for benzyl alcohol oxidation, whose yield of benzaldehyde was as high as 99.0%. In this catalytic system, NaNO2 activated the catalytic reaction by releasing NO2, which oxidized Fe2+-TEMPOH to Fe3+-TEMPO. Consequently, Fe3+-TEMPO oxidized the benzyl alcohol to be benzaldehyde (Wang et al., 2005). Zhang and co-workers introduced Fe into imidazolium and successfully prepared a nice iron-based catalyst [Imim-TEMPO][FeCl4]/NaNO2 (Miao et al., 2011), showing a good activity for the selective oxidation of aromatic alcohols under 5% NaNO2. The catalytic mechanism of [Imim-TEMPO][FeCl4]/NaNO2 was similar to that of FeCl3/TEMPO/NaNO2, and the aromatic alcohol was oxidized in the process of redox between Fe3+ and NO2. Martin and co-workers (Martin and Suárez, 2002) discovered an efficient system by combining Fe(NO3)3 and FeBr3 for selective oxidation of benzylic alcohol to corresponding aldehyde. Though the Fe-based catalysts had good activity, problems are obvious. For example, the introduction of Br or co-catalyst (TEMPO) has increased the cost as well as environmental concerns, resulting in weaker competitive when industrial applications.
The reported Fe-based catalytic systems usually contain . In fact, releases active NO2 and initiate the alcohol oxidation by several redox reactions. Inspired by this, we are intrigued whether the combination of metal iron (Mn+) and / can construct an efficient catalytic system for the alcohol oxidation. Studies from Jachuck et al. (2006) and Dressen et al. (2009) verified that Fe(NO3)3 could successfully oxidize benzyl alcohol to benzaldehyde under microwave irradiation. They deemed Fe3+ in the oxidation of benzyl alcohol as catalyst, because overall Fe3+ remained in its original oxidative state. However, this study was limited in microwave irradiation and lacked the universality for actual application. Besides, the oxidation performances of other system composed of metal iron (Cu, Al, Mg, Co, Ni) and / were unclear, and the role of Mn+ had not been reported.
Hence, we studied the performance of Fe(NO3)3·9H2O by continuous heating in O2 and N2 (He) atmosphere. Interestingly, the results were different from the phenomenon in microwave irradiation. The catalytic performance of Fe(NO3)3·9H2O in N2 was significantly improved compared to that in O2. Further, the oxidation performance of other nitrates was studied. To expand the applicability of the combination of Mn+ and , we conducted systematic research using Fe(NO3)39H2O as an example. The reaction conditions such as temperature and solvent were optimized. The optimized conditions were applied to the oxidation of a variety of alcohols. Finally, the catalytic mechanism was put forward.
Experimental
Materials
Benzyl alcohol, nitrates with crystalline waters, and other reagents were analytical grade. Gas chromatography (GC) analysis was performed on SHIMAZDU GC-2014 equipped with a HP-5 column (30 m × 0.32 mm × 0.25 um) and a flame ionization detector.
The Oxidation of Benzyl Alcohol
Typically, 3 mmol of benzyl alcohol, 3 mmol of naphthalene as an internal standard, and 15 mL of 1,4-dioxane as solvent were added to a 25 mL three-necked flask. Then the three-necked flask provided with a reflux condenser was evacuated using an aspirator and followed by the attachment of a nitrogen balloon. Subsequently, the solution was heated to the desired temperature. When the temperature of solution reached the set point, 2 mmol of ferric nitrate (Fe(NO3)3·9H2O) was added into it. Samples were taken at appropriate intervals through a silicon septum using a hypodermic needle and were filtered with a membrane filter (PVDF) with 13 mm × 0.22 μm pore prior to GC analysis.
The Product Analysis
The analysis of benzyl alcohol and oxidation products was carried out on Aglient 7980 series with a HP-5 column and a flame ionization detector. The condition of GC for the HP-5 capillary column (30 m, DF = 0.25 mm, 0.25 mm i.d.), and temperature program was carried out (initial temperature = 100°C, 3 min; final temperature = 250°C, heating rate = 10°C min−1, temperature of injector = 280°C, temperature of detector = 280°C). The quantitative results of products were based on the internal standard method, using naphthalene as an internal standard. The typical analytic procedure was as following: (1) 0.4 ml sample was taken from reaction solutions and was then filtered with a membrane filter (PVDF) with 13 mm × 0.22 μm; (2) then, the sample would be diluted 10-fold before GC analysis; (3) when the GC temperature reached the set points, the diluted solution was injected into this equipment to analyze products according to the different retention times and response peak area. The typical results of GC measurements and internal standard working curve were shown in Figure S1. The results reported as conversion and selectivity are expressed in mol%, based on the total benzyl alcohol intake. The calculation of the conversion and selectivity was as follows:
Results and Discussion
The Oxidation of Benzyl Alcohol by Ferric Nitrate
Figures 1A,B showed the oxidation results of benzyl alcohol to benzaldehyde in the presence of ferric nitrate and nitric acid. In N2 condition, the conversion of benzyl alcohol catalyzed by ferric nitrate was 94.9% after 6 h. To ensure reproducibility, the experimental error-based three parallel experiments was shown in Figure S2 and the carbon balance during experiments was also evaluated (Table S1). The results showed that performance of Fe(NO3)3 had a good testing repeatability and was highly efficient. Interestingly, the benzyl alcohol conversion catalyzed by Fe(NO3)3 could be as high as 96.84% with 94.5% selectivity when replacing N2 with He. Compared with HNO3, ferric nitrate exhibited an excellent activity in the benzyl alcohol oxidation. The conversion of benzyl alcohol catalyzed by ferric nitrate was 46.2%—higher than that by nitric acid. Similarly, under aerobic conditions (O2), the conversion of benzyl alcohol catalyzed by ferric nitrate was about 13% higher than that by nitric acid with similar selectivity. The results indicated the oxidation performance of ferric nitrate was markedly better than that of nitric acid, no matter under anaerobic or aerobic conditions.
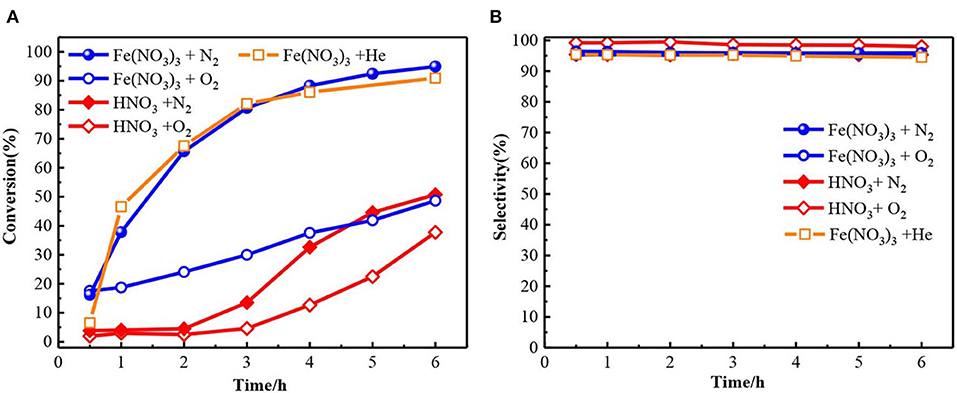
Figure 1. The conversion (A) and selectivity (B) for benzyl alcohol oxidation to benzaldehyde with Fe(NO3)3·9H2O and HNO3. Fe(NO3)3 denotes Fe(NO3)3·9H2O unless otherwise noted.
Compared with other typical catalysts, the catalytic activity of Fe(NO3)3 was also outstanding. The conversion of benzyl alcohol catalyzed by Fe(NO3)3 with any oxidant (96.84%) was close to that by CNT-HNO3 (Luo et al., 2012) (96.2%), as shown in Table 1 (Entry 2). Besides, the performance of Fe(NO3)3 surpassed the typical metal-based catalyst, such as Au/γ-Al2O3 (73.4%) (Ndolomingo and Meijboom, 2017), Fe/MCM41 (55%) (Cánepa et al., 2017), or Fe-N-C (78.0%) (Xie et al., 2017). Moreover, Fe(NO3)3 also exhibited comparable activity with transition-metal oxides, such as Co3O4/RGO-N (93.9%) (Nie et al., 2013), MnOx (72.7%) (Jing et al., 2007). The excellent catalytic activity of Fe(NO3)3 may be attributed to the combined action of Fe3+ and . On the one hand, could produce NO2, and then NO2 with H2O was converted to HNO2, which had been proved as a pivotal role in benzyl alcohol oxidation (Aellig et al., 2012; Luo et al., 2012). On the other hand, the valence change of Fe could catalyze benzyl alcohol oxidation according to the literatures (Miao et al., 2016; Hu et al., 2018). Coincidentally, the transformation between Fe3+ and Fe2+ was demonstrated by the K3[Fe(CN)6] solution. As shown in Figure S3, the Prussian blue precipitate appeared in the experimental process due to the existence of Fe2+. Therefore, Fe(NO3)3 exhibited the excellent oxidation activity via Fe3+ initiating a series of electron and proton transfer. It was noted that the anaerobic condition was beneficial to improve the oxidation performance of ferric nitric. The reason is discussed in detail in mechanism Part 3.4.
The Performance of Other Metallic Nitrates
Subsequently, the catalytic performance of other nitrates was also studied. The benzyl alcohol oxidation catalyzed by other nitrates including was investigated, as shown in Figure 2. The result proves other nitrates are also capable for converting the benzyl alcohol to benzaldehyde. In Al(NO3)3 system, the conversion of benzyl alcohol and the selectivity to benzaldehyde was 88.1% and 80% after 6 h, lower than those in Fe(NO3)3 system. Similarly, Cu(NO3)2 could also oxidize the benzyl alcohol with the conversion of 82.3%. While the selectivity to benzadehyde was only 70%, and the other 30% was benzoic acid from the excessive oxidation. The catalytic performance of Co(NO3)2 significantly decreased. While Mg(NO3)2 and Zn(NO3)2 had almost no catalytic activity. The order of activity of different nitrates is Fe(NO3)3 > Al(NO3)3 > Cu(NO3)2 > Co(NO3)2 > Mg(NO3)2 ≈ Zn(NO3)2. Yuvaraj et al. (2003) tested the decomposition temperature of these nitrates by TG/DAT. And they found Fe(NO3)3 and Al(NO3)3 had the lowest the decomposition temperature (130°C), followed by Cu(NO3)2 (227°C) and Co(NO3)2 (247°C), and Zn(NO3)2 (367°C) was the highest. Their decomposition products were metal oxide, NO2 and O2. NO2 and H2O together would convert into HNO2 which could attack the benzyl alcohol, finally producing benzaldehyde. Hence, the nitrates with lower decomposition temperature could easily produce NO2 and oxidize more benzyl alcohol. As expected, the rank of conversion of benzyl alcohol in different nitrate system was related to the decomposition temperature of these nitrates.
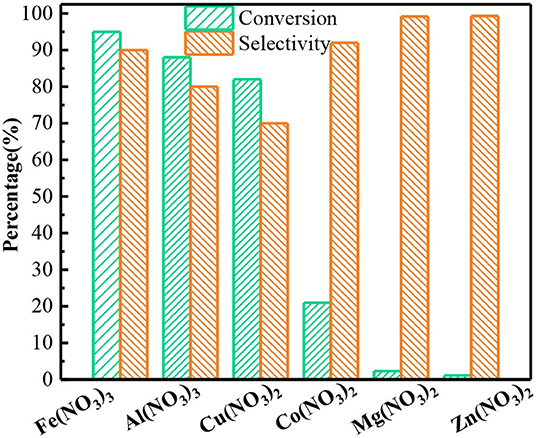
Figure 2. The oxidation of benzyl alcohol to benzaldehyde by different nitrates. Reaction conditions: benzyl alcohol (3 mmol), 1,4-dioxane (15 mL), Fe(NO3)3 and Al(NO3)3 (2 mmol), or other nitrates (3 mmol), 80°C, 6 h ball N2.
Effect of Reaction Conditions on Oxidation Performance of Fe(NO3)3
Fe(NO3)3 is an excellent catalyst among several nitrates; the effect of reaction condition and solvent on the performance of Fe(NO3)3 was investigated. As the results shown in Figure 3A, the higher temperature would markedly enhance the yields of benzaldehyde. Since high temperature would increase the risk of the over oxidation from benzaldehyde to benzoic acid, the optimum temperature was 80°C. Similarly, 2 mmol Fe(NO3)3 could selectively oxidize the benzyl alcohol to benzaldehyde with 91.5% yields shown in Figure 3B. Besides, the solvent had an important effect on the activity of Fe(NO3)3. The results in Figure 3C show the strong polar solvent could bring a mutual solution containing the aqueous (HNO2) and organic phase (benzyl alcohol), which would be more favorable to form of benzyl nitrite, resulting in high conversion and selectivity.
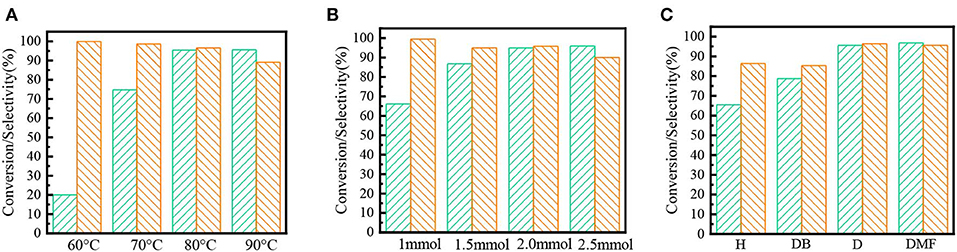
Figure 3. The effect of different factors on the oxidation activity of Fe(NO3)3. Green square: conversion; Orange square: selectivity; (A) temperature; (B) dosage of Fe(NO3)3; (C) solvent polarity: n-hexane (H), dimethylbenzene (DB); 1,4-dioxane (D); N,N-dimethylformamide (DMF).
The Applicability of Fe(NO3)3 Catalyst
Subsequently, to demonstrate the general applicability of Fe(NO3)3, selective oxidation of substituted benzyl alcohols with different functional groups was investigated. The results presented in Table 2 showed all these primary benzylic alcohols could be converted to corresponding aldehydes. The oxidation results were somewhat related to the substituent groups on the phenyl ring. The alcohols with electron withdrawing groups (–NO2, –Cl) gained lower yields of products than those with electron donating groups (–MeO, –OH). The effect rule of substituent groups in Fe(NO3)3 system is consistent with that in HNO3 system (Joshi et al., 2005). The formation of benzyl nitrite, a vital intermediate product, can be regarded as the electrophilic substitution reaction of benzyl alcohol. Thus, the electron-donating substituents would enhance the yield of product by increasing the electron density on the benzyl ring and vice versa. The results proved that the electron density on the aromatic ring played a critical role in the oxidation of benzylic alcohol.
Reaction Mechanism
Combined with the experimental results and literature reports, the following catalytic mechanism hypothesis was preliminarily put. Both Fe3+ and may have an important effect on the oxidation process. Fe3+ provides catalytic function by the electron transferring, while the NOx produced from the experimental process may have certain oxidation performance. To verify above possible speculations and analyze the catalytic mechanism, a series of experiments were designed, and the results were shown as follows.
To illustrate the role of Fe3+ and , Zn(NO3)2 or FeCl3 was individually added to the reaction mixtures. The result showed benzyl alcohol conversion in both systems was very low (Figure 4), indicating that only Fe3+ or had poor oxidation activity. While in Zn(NO3)2 + FeCl3 system, the conversion of benzyl alcohol was as high as 95.8% with 93.6% selectivity, which was similar to that in Fe(NO3)3 system with the same amount of Fe3+ and . The results proved the Fe3+ and together could oxidize the benzyl alcohol. To illuminate how Fe3+ and together catalyze the benzyl alcohol, introducing urea as a known HNO2 scavenger into Fe(NO3)3 system. The results in Figure 4 showed the catalytic activity of Fe(NO3)3 was almost prevented. This phenomenon proved that the oxidation process of Fe(NO3)3 was mainly achieved by HNO2 attacking benzyl alcohol. The intermediate benzyl nitrite detected by GC-MS in our experiments further verified the reaction between benzyl alcohol and HNO2. Besides, when butylated hydroxytoluene (BHT) as a radical trapping agent was added into the Fe(NO3)3 system, the conversion still kept a high level of 86.3%, proving that the benzyl alcohol oxidation catalyzed by Fe(NO3)3 is not a radical-involved reaction. Interestingly, the conversion would increase obviously, and the selectivity also kept at a high value (95%) when Fe3+ instead of H+ with the same mole of as shown in Figure 4. Furthermore, the addition of Fe3+ into HNO3 system also significantly enhanced the conversion of benzyl alcohol compared with the HNO3 system. From these results, we speculated Fe3+ had a special catalytic function in the process of benzyl alcohol oxidation.
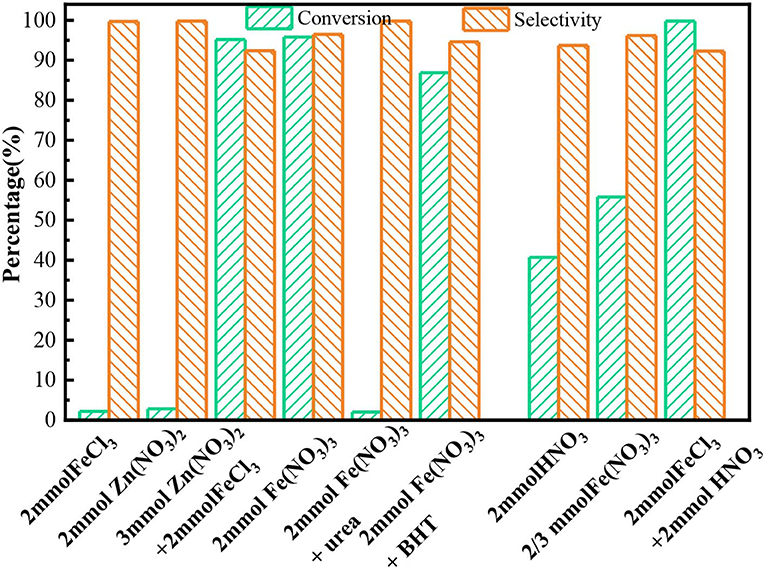
Figure 4. The conversion and selectivity for the oxidation of benzyl alcohol in different system. Benzyl alcohol (3 mmol), 1,4-dioxane (15 mL), 80°C, 6 h, ball N2.
A series of experiments were conducted to further illuminate the catalysis of Fe3+. As the results shown in Figure 5A, the reaction rate increased as the amount of Fe3+ increased in FeCl3-HNO3 system. Meanwhile, the results shown in Figure 5B proved that the variation of selectivity was little. To quantify the effect of Fe3+, the turnover frequency (TOF) was calculated based on Fe content at the conversion lower than 25.0%, as shown in Figure 5C. The results proved the Fe3+ had a remarkable effect on the oxidation reaction. The TOF of Fe3+ in the FeCl3-HNO3 system further enlarged compared to that in the Fe(NO3)3 system. As shown in Figure 5D, the TOF of Fe3+ in the FeCl3-HNO3 system was almost unchanged (8.1 h−1), even if the amount of Fe3+ increased from 0.5 to 1 mmol.
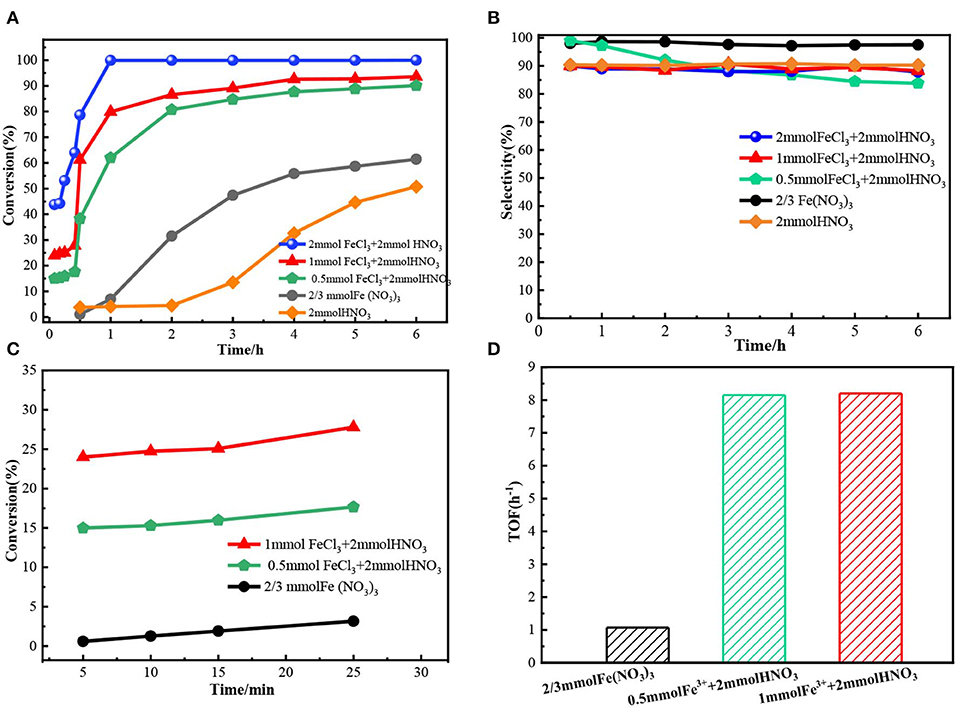
Figure 5. The oxidation results of benzyl alcohol in different catalyst systems. The TOF was defined as and were calculated at 15 min, for which the conversion was lower than 25.0%. (A) The benzyl alcohol conversion in 6 h. (B) The selectivity of benzaldehyde. (C) The benzyl alcohol conversion in 30 min. (D) TOF.
Furthermore, the change of Fe in benzyl alcohol oxidation was analyzed by experiments. The transformation between Fe3+ and Fe2+ was detected in situ by 1 mol/L KMnO4 solution in the oxidation process. For comparison, the reaction solvent (1,4-dioxane) was added to the KMnO4 solution, the solution was black-purple as shown in Figure 6. The color of KMnO4 solution changed from black-purple to yellow when KMnO4 solution was added into reaction solution at 2 h. This phenomenon showed the KMnO4 was reduced by Fe2+, and the Fe2+ existed in the reaction process. However, the KMnO4 solution changed from yellow to brown when it was added into the reaction solution at the reaction time of 6 h. The phenomenon illustrated that there were less Fe2+ in the reaction solution. Because the oxidation reaction of benzyl alcohol almost completed, most of Fe3+ did not convert anymore and Fe2+ would further oxidized to Fe3+ by the O2 from the decomposition of Fe(NO3)3. So, the cyclic conversion of Fe3+ and Fe2+ really occurred in benzyl alcohol oxidation. According to the reported results, the valence transformation of metal iron such as MnIII/MnII (Yang et al., 2014; Fei et al., 2017; Gurrala et al., 2018), CoIII/CoII (Zhou et al., 2015; Cordoba et al., 2017; Li et al., 2017), CrIII/ CrII (Thao et al., 2018) could catalyze the oxidation of alcohols. Especially, the transformation of Fe3+/Fe2+ possessed high catalytic activity by initiating a series of electron transfer (Hu et al., 2016, 2018; Miao et al., 2016). Combined with above results, Fe3+ could be regard as a catalyst and the electron transfer between of Fe3+ and Fe2+ would catalyze the oxidation process.
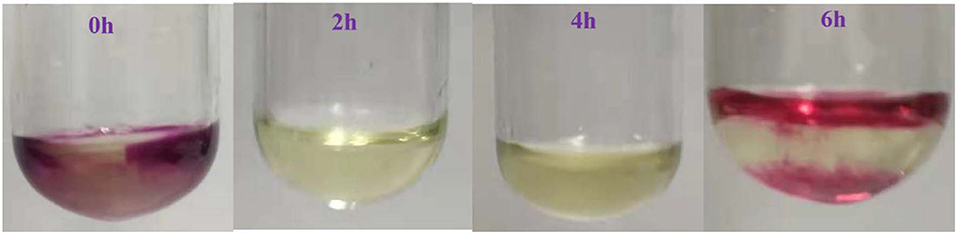
Figure 6. The phenomena in the experimental process at different moments after adding 1 mol/L KMnO4 to the reaction solution.
Finally, the function of NOx was investigated by a series of auxiliary experiments. As we know, nitric oxide (NO) would be immediately oxidized to nitrogen dioxide (NO2) by O2 (Wang et al., 2005; Shen et al., 2015, 2019; Miao et al., 2016; Hu et al., 2018; Dong et al., 2019; Zhao et al., 2019). O2 was flowed into the reaction system in the experimental process to detect the NO. Brown fumes immediately occurred (Figure S4), proving the presence of NO in the inert atmosphere. Subsequently, NO was prepared by the reaction between Cu and 35 wt % nitric acid (3Cu + 8HNO3 → 3Cu(NO3)2 + 2NO + 4H2O). The oxidation of benzyl alcohol by NO was conducted and the detailed experimental process was shown in Figure S5. The conversion of benzyl alcohol by NO was only 1% at 6 h with 99% selectivity, as shown in Figure 7. The results indicated NO itself could not oxidize benzyl alcohol. Hence, the NO2 from the decomposition of Fe(NO3)3 played the key role in oxidation process by forming HNO2.
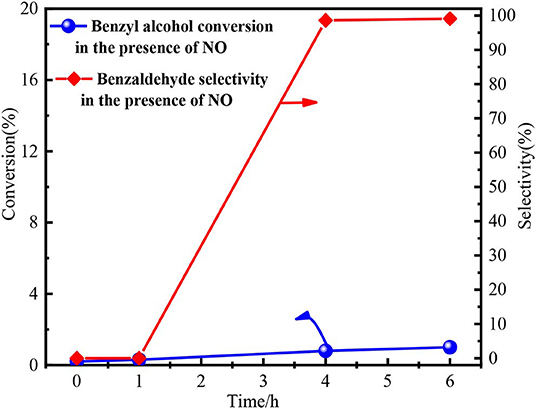
Figure 7. The performance of NO in benzyl alcohol oxidation process. Reaction condition: 3 mmol benzyl alcohol, 15.0 ml 1,4-dioxane, 80°C.
According to the above investigation, the mechanism of the benzyl alcohol oxidation in N2 condition was put forward and shown in Scheme 1. At the suitable reaction temperature, the Fe(NO3)3 decomposes into Fe2O3, NO2, and O2 (Yuvaraj et al., 2003) by Equation 1. Subsequently, the HNO2 and HNO3 can be formed by the reaction between NO2 and H2O, see Equation 2. Then, the oxidation reaction successfully gets into the propagation stage and HNO2 attacks the benzyl alcohol (PhCH2OH) and gives benzyl nitrite (PhCH2ONO). Benzyl nitrite decomposes into benzaldehyde (PhCHO) and HNO at experimental temperature by Equation 4. In the termination stage, as shown in Equation 6, Fe(NO3)2 can be formed by the reaction between the HNO2 and Fe2O3 with consuming O2. At the same time, Fe(NO3)2 is oxidized to Fe(NO3)3 by O2. Consequently, from Reaction 1 to Reaction 7, can be considered as the real oxidant, and the Fe ion acts a catalyst through the transformation between Fe2+ and Fe3+.
From the reaction mechanism, when the system was filled with O2, the decomposition of Fe(NO3)3 would be hindered, leading to low conversion compared with anaerobic condition (N2 or He). Interestingly, the O2 was double-edged gas since it could oxidize the Fe2+ to Fe3+ and eliminate NO Equation 7. In the presence of adequate oxygen, the Fe2+ was completely oxidized into Fe3+, and oxidative state of Fe remained the same before and after the reaction. The conversion of benzyl alcohol in Fe(NO3)3-O2 system was relatively low (Figure 8A), but it was still higher than that in HNO3 system. Moreover, in Fe(NO3)3 system, the conversion of benzyl alcohol could reach 82% when O2 replaced N2 after the reaction continuing 2 h (Figure 8A). As expected, the conversion of benzyl alcohol in air (N2 + O2) condition was close to that in N2 condition (Figure 8B). In the aerobic conditions, would be regenerated and the Fe2+ was completely oxidized to Fe3+, indicating O2 was the actual oxidation. Though the O2 would be not good for the high conversion, it could build a green cyclic oxidation process via removing NO and regenerating Fe(NO3)3. Hence, the benzyl alcohol conversion would be relatively high in air atmosphere, which also meet the need of green synthesis due to removing the NO.
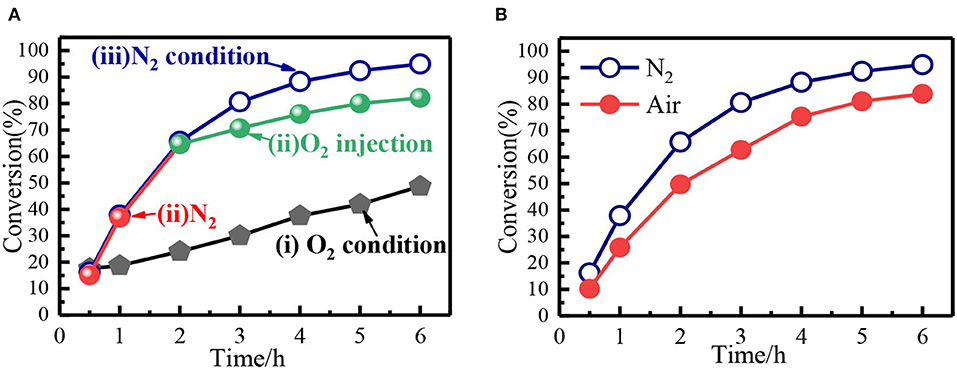
Figure 8. (A) The benzyl alcohol conversion under different conditions: (i) O2; (ii) N2 (1-2 h) and O2 (2–6 h); (iii) N2. (B) Comparison of the benzyl alcohol conversion in N2 and air.
Conclusion
As a bio-friendly and economical material, ferric nitrate showed an outstanding oxidation performance for benzyl alcohol oxidation. The conversion of benzyl alcohol in ferric nitrate system reached 95%, which was 46% higher than that in nitric acid system under N2 atmosphere. Other metallic nitrates that could release NO2 at reaction temperature also had high properties for benzyl alcohol oxidation. Moreover, ferric nitrate is of excellent applicability for other primary benzylic alcohols oxidation under optimized condition. The mechanism study indicated ferric nitrate was as initiator in the reaction. In the procedure, it would decompose into Fe2O3 and NO2 which immediately became HNO2, attacking benzyl alcohol, and forming the benzaldehyde afterward. While in anaerobic atmosphere, was the oxidant by providing HNO2 and the transformation cycle between Fe3+ and Fe2+ generates a catalytic effectiveness. Hence, the balance of high conversion and green synthesis requirement would be obtained for the benzyl alcohol oxidation in the air atmosphere.
Data Availability Statement
The datasets generated for this study are available on request to the corresponding author.
Author Contributions
SX, GZ, and FP designed experiments. JW, PH, CL, HL, YW, and ZW carried out experiments. SX, GZ, XF, and FP analyzed experimental results, analyzed data, and wrote the manuscript.
Funding
This work was supported by Engineering Research Center of None-food Biomass Efficient Pyrolysis and Utilization Technology of Guangdong Higher Education Institutes (2016GCZX009), National Natural Science Foundation of China (No. 21905044), Natural Science Foundation of Guangdong Province (No. 2018A030310004), and Research Start-up Funds of Dongguan University of Technology (GC300501-077).
Conflict of Interest
The authors declare that the research was conducted in the absence of any commercial or financial relationships that could be construed as a potential conflict of interest.
Supplementary Material
The Supplementary Material for this article can be found online at: https://www.frontiersin.org/articles/10.3389/fchem.2020.00151/full#supplementary-material
References
Aellig, C., Ulrich, N., and Hermans, I. (2012). Acid-catalyzed decomposition of the benzyl nitrite intermediate in HNO3-mediated aerobic oxidation of benzyl alcohol. ChemCatChem 4, 525–529. doi: 10.1002/cctc.201100439
Albadi, J., Alihoseinzadeh, A., and Razeghi, A. (2014). Novel metal oxide nanocomposite of Au/CuO–ZnO for recyclable catalytic aerobic oxidation of alcohols in water. Catal. Commun. 49, 1–5. doi: 10.1016/j.catcom.2014.01.030
Cánepa, A. L., Elías, V. R., Vaschetti, V. M., Sabre, E. V., Eimer, G. A., Casuscelli, S. G., et al. (2017). Selective oxidation of benzyl alcohol through eco-friendly processes using mesoporous V-MCM-41, Fe-MCM-41 and Co-MCM-41 materials. Appl. Catal. A Gen. 545, 72–78. doi: 10.1016/j.apcata.2017.07.039
Cao, Y., Li, Y., Yu, H., Peng, F., and Wang, H. (2015). Aerobic oxidation of α-pinene catalyzed by carbon nanotubes. Catal. Sci. Technol. 5, 3935–3944. doi: 10.1039/C5CY00136F
Cao, Y., Luo, X., Yu, H., Peng, F., Wang, H., Ning, G., et al. (2013). sp 2-and sp 3-hybridized carbon materials as catalysts for aerobic oxidation of cyclohexane. Catal. Sci. Technol. 3, 2654–2660. doi: 10.1039/c3cy00256j
Chen, C. T., Nguyen, C. V., Wang, Z. Y., Bando, Y., Yamauchi, Y., Bazziz, M. T. S., et al. (2018a). Hydrogen peroxide assisted selective oxidation of 5-hydroxymethylfurfural in water under mild conditions. ChemCatChem 10, 361–365. doi: 10.1002/cctc.201701302
Chen, H., Shen, J., Chen, K., Qin, Y., Lu, X., Ouyang, P., et al. (2018b). Atomic layer deposition of Pt nanoparticles on low surface area zirconium oxide for the efficient base-free oxidation of 5-hydroxymethylfurfural to 2,5-furandicarboxylic acid. Appl. Catal. A Gen. 555, 98–107. doi: 10.1016/j.apcata.2018.01.023
Choudhary, V. R., Dhar, A., Jana, P., Jha, R., and Uphade, B. S. (2005). A green process for chlorine-free benzaldehyde from the solvent-free oxidation of benzyl alcohol with molecular oxygen over a supported nano-size gold catalyst. Green Chem. 7, 768–770. doi: 10.1039/b509003b
Cordoba, M., Miranda, C., Lederhos, C., Coloma-Pascual, F., Ardila, A., Fuentes, G., et al. (2017). Catalytic performance of Co3O4 on different activated carbon supports in the benzyl alcohol oxidation. Catalysts 7:384. doi: 10.3390/catal7120384
Dong, G., Zhao, L., Wu, X., Zhu, M., and Wang, F. (2019). Photocatalysis removing of NO based on modified carbon nitride: the effect of celestite mineral particles. Appl. Catal. B Environ. 245, 459–468. doi: 10.1016/j.apcatb.2019.01.013
Dressen, M. H. C. L., Stumpel, J. E., van de Kruijs, B. H. P., Meuldijk, J., Vekemans, J. A. J. M., and Hulshof, L. A. (2009). The mechanism of the oxidation of benzyl alcohol by iron(III)nitrate: conventional versus microwave heating. Green Chem. 11, 60–64. doi: 10.1039/B813030B
Fei, J., Sun, L., Zhou, C., Ling, H., Yan, F., Zhong, X., et al. (2017). Tuning the synthesis of manganese oxides nanoparticles for efficient oxidation of benzyl alcohol. Nanoscale Res. Lett. 12:23. doi: 10.1186/s11671-016-1777-y
Ganesamoorthy, S., Tamizh, M. M., Shanmugasundaram, K., and Karvembu, R. (2013). Immobilization of Ru (III) complex on silica: a heterogenized catalyst for selective oxidation of alcohols in water at room temperature. Tetrahedron Lett. 54, 7035–7039. doi: 10.1016/j.tetlet.2013.10.070
Gurrala, L., Nagpure, A. S., Gurav, H. R., and Chilukuri, S. (2018). Spinel-type mixed oxides for stable and selective partial oxidation of benzyl alcohol. Chemistryselect 3, 3751–3761. doi: 10.1002/slct.201800321
Hansen, T. S., Sádaba, I., García-Suárez, E. J., and Riisager, A. (2013). Cu catalyzed oxidation of 5-hydroxymethylfurfural to 2,5-diformylfuran and 2,5-furandicarboxylic acid under benign reaction conditions. Appl. Catal. A Gen. 456, 44–50. doi: 10.1016/j.apcata.2013.01.042
Hanson, S. K., Baker, R. T., Gordon, J. C., Scott, B. L., Sutton, A. D., and Thorn, D. L. (2008). Aerobic oxidation of pinacol by vanadium (V) dipicolinate complexes: evidence for reduction to vanadium (III). J. Am. Chem. Soc. 131, 428–429. doi: 10.1021/ja807522n
Hu, Y., Chen, L., and Li, B. (2016). Iron nitrate/TEMPO-catalyzed aerobic oxidative synthesis of quinazolinones from alcohols and 2-aminobenzamides with air as the oxidant. RSC Adv. 6, 65196–65204. doi: 10.1039/C6RA12164K
Hu, Y., Chen, L., and Li, B. (2018). Fe(NO 3) 3 /2,3-dichloro-5,6-dicyano-1,4-benzoquinone (DDQ): an efficient catalyst system for selective oxidation of alcohols under aerobic conditions. Catal. Commun. 103, 42–46. doi: 10.1016/j.catcom.2017.09.019
Jachuck, R. J. J., Selvaraj, D. K., and Varma, R. S. (2006). Process intensification: oxidation of benzyl alcohol using a continuous isothermal reactor under microwave irradiation. Green Chem. 8, 29–33. doi: 10.1039/B512732G
Ji, H., Wang, T., Zhang, M., She, Y., and Wang, L. (2005). Simple fabrication of nano-sized NiO2 powder and its application to oxidation reactions. Appl. Catal. A Gen. 282, 25–30. doi: 10.1016/j.apcata.2004.11.043
Jia, X., Ma, J., Wang, M., Du, Z., Lu, F., Wang, F., et al. (2014). Promoted role of Cu(NO3)2 on aerobic oxidation of 5-hydroxymethylfurfural to 2,5-diformylfuran over VOSO4. Appl. Catal. A Gen. 482, 231–236. doi: 10.1016/j.apcata.2014.05.031
Jiang, X., Zhang, J., and Ma, S. (2016). Iron catalysis for room-temperature aerobic oxidation of alcohols to carboxylic acids. J. Am. Chem. Soc. 138, 8344–8347. doi: 10.1021/jacs.6b03948
Jing, H., Keqiang, S., Daiping, H., and Boqing, X. (2007). Amorphous manganese oxide for catalytic aerobic oxidation of benzyl alcohol. Chin. J. Catal. 28, 1025–1027. doi: 10.1016/S1872-2067(08)60001-7
Joshi, S. R., Kataria, K. L., Sawant, S. B., and Joshi, J. B. (2005). Kinetics of oxidation of benzyl alcohol with dilute nitric acid. Indust. Eng. Chem. Res. 44, 325–333. doi: 10.1021/ie0303911
Li, M., Wu, S., Yang, X., Hu, J., Peng, L., Bai, L., et al. (2017). Highly efficient single atom cobalt catalyst for selective oxidation of alcohols. Appl. Catal. A Gen. 543, 61–66. doi: 10.1016/j.apcata.2017.06.018
Li, M., Xu, F., Li, H., and Wang, Y. (2016). Nitrogen-doped porous carbon materials: promising catalysts or catalyst supports for heterogeneous hydrogenation and oxidation. Catal. Sci. Technol. 6, 3670–3693. doi: 10.1039/C6CY00544F
Liu, J., Zou, S., Lu, L., Zhao, H., Xiao, L., and Fan, J. (2017). Room temperature selective oxidation of benzyl alcohol under base-free aqueous conditions on Pt/TiO2. Catal. Commun. 99, 6–9. doi: 10.1016/j.catcom.2017.05.015
Long, J., Xie, X., Xu, J., Gu, Q., Chen, L., and Wang, X. (2012). Nitrogen-doped graphene nanosheets as metal-free catalysts for aerobic selective oxidation of benzylic alcohols. ACS Catal. 2, 622–631. doi: 10.1021/cs3000396
Lu, C., Hu, J. M., Meng, Y. N., Zhou, A. D., Zhang, F., and Zhang, Z. B. (2019). The synergistic effect of benzyl benzoate on the selective oxidation of toluene to benzaldehyde. Chem. Eng. Res. Des. 141, 181–186. doi: 10.1016/j.cherd.2018.10.019
Luo, J., Peng, F., Yu, H., and Wang, H. (2012). Selective liquid phase oxidation of benzyl alcohol catalyzed by carbon nanotubes. Chem. Eng. J. 204–206, 98–106. doi: 10.1016/j.cej.2012.07.098
Luo, J., Yu, H., Wang, H., Wang, H., and Peng, F. (2014). Aerobic oxidation of benzyl alcohol to benzaldehyde catalyzed by carbon nanotubes without any promoter. Chem. Eng. J. 240, 434–442. doi: 10.1016/j.cej.2013.11.093
Lv, L. B., Yang, S. Z., Ke, W. Y., Wang, H. H., Zhang, B., Zhang, P., et al. (2018). Mono-atomic Fe centers in nitrogen/carbon monolayers for liquid-phase selective oxidation reaction. ChemCatChem 10, 3539–3545. doi: 10.1002/cctc.201800707
Mahmood, A., Robinson, G. E., and Powell, L. (1999). An improved oxidation of an alcohol using aqueous permanganate and phase-transfer catalyst. Organ. Proc. Res. Dev. 3, 363–364. doi: 10.1021/op990021h
Mal, D. D., Khilari, S., and Pradhan, D. (2018). Efficient and selective oxidation of toluene to benzaldehyde on manganese tungstate nanobars: a noble metal-free approach. Green Chem. 20, 2279–2289. doi: 10.1039/C8GC00123E
Martin, S. E., and Suárez, D. F. (2002). Catalytic aerobic oxidation of alcohols by Fe(NO3)3–FeBr3. Tetrahedron Lett. 43, 4475–4479. doi: 10.1016/S0040-4039(02)00829-8
Miao, C., Zhao, H., Zhao, Q., Xia, C., and Sun, W. (2016). NHPI and ferric nitrate: a mild and selective system for aerobic oxidation of benzylic methylenes. Catal. Sci. Technol. 6, 1378–1383. doi: 10.1039/C5CY01245G
Miao, C. X., Wang, J.-Q., Yu, B., Cheng, W.-G., Sun, J., Chanfreau, S., et al. (2011). Synthesis of bimagnetic ionic liquid and application for selective aerobic oxidation of aromatic alcohols under mild conditions. Chem. Commun. 47, 2697–2699. doi: 10.1039/c0cc04644b
Ndolomingo, M. J., and Meijboom, R. (2017). Selective liquid phase oxidation of benzyl alcohol to benzaldehyde by tert-butyl hydroperoxide over gamma-Al2O3 supported copper and gold nanoparticles. Appl. Surf. Sci. 398, 19–32. doi: 10.1016/j.apsusc.2016.12.020
Nie, R., Shi, J., Du, W., Ning, W., Hou, Z., and Xiao, F. S. (2013). A sandwich N-doped graphene/Co3 O4 hybrid: an efficient catalyst for selective oxidation of olefins and alcohols. J. Mater. Chem. A 1, 9037–9045. doi: 10.1039/c3ta11672g
Öztürk, Ö. F., Zümreoglu-Karan, B., and Karabulut, S. (2008). Solvent-free oxidation of benzyl alcohol over chromium orthoborate. Catal. Commun. 9, 1644–1648. doi: 10.1016/j.catcom.2008.01.016
Parmeggiani, C., and Camilla, F. (2012). Transition metal based catalysts in the aerobic oxidation of alcohols. Green Chem. 14, 547–564. doi: 10.1039/c2gc16344f
Ragupathi, C., Judith Vijaya, J., Narayanan, S., Jesudoss, S. K., and John Kennedy, L. (2015). Highly selective oxidation of benzyl alcohol to benzaldehyde with hydrogen peroxide by cobalt aluminate catalysis: a comparison of conventional and microwave methods. Ceram Int. 41, 2069–2080. doi: 10.1016/j.ceramint.2014.10.002
Shen, X., Dong, G., Wang, L., Ye, L., and Sun, J. (2019). Enhancing photocatalytic activity of NO removal through an in situ control of oxygen vacancies in growth of TiO2. Adv. Mater. Interf. 6:1901032. doi: 10.1002/admi.201901032
Shen, Z., Chen, M., Fang, T., Li, M., Mo, W., Hu, B., et al. (2015). Transformation of ethers into aldehydes or ketones: a catalytic aerobic deprotection/oxidation pathway. Tetrahedron Lett. 56, 2768–2772. doi: 10.1016/j.tetlet.2015.04.033
Shimizu, H., Onitsuka, S., Egami, H., and Katsuki, T. (2005). Ruthenium(salen)-catalyzed aerobic oxidative desymmetrization of meso-diols and its kinetics. J. Am. Chem. Soc. 127, 5396–5413. doi: 10.1021/ja047608i
Tamizhdurai, P., Sakthinathan, S., Chen, S. M., Shanthi, K., Sivasanker, S., and Sangeetha, P. (2017). Environmentally friendly synthesis of CeO2 nanoparticles for the catalytic oxidation of benzyl alcohol to benzaldehyde and selective detection of nitrite. Sci. Rep. 7:46372. doi: 10.1038/srep46372
Thao, N. T., Nhu, N. T., and Lin, K. S. (2018). Liquid phase oxidation of benzyl alcohol to benzaldehyde over sepiolite loaded chromium oxide catalysts. J. Taiwan Inst. Chem. Eng. 83, 10–22. doi: 10.1016/j.jtice.2017.11.034
Thottathil, J. K., Moniot, J. L., Mueller, R. H., Wong, M. K., and Kissick, T. P. (1986). Conversion of L-pyroglutamic acid to 4-alkyl-substituted L-prolines. The synthesis of trans-4-cyclohexyl-L-proline. J. Organ. Chem. 51, 3140–3143. doi: 10.1021/jo00366a011
Villa, A., Wang, D., Dimitratos, N., Su, D., Trevisan, V., and Prati, L. (2010). Pd on carbon nanotubes for liquid phase alcohol oxidation. Catal Today 150, 8–15. doi: 10.1016/j.cattod.2009.06.009
Wang, N., Liu, R., Chen, J., and Liang, X. (2005). NaNO2-activated, iron-TEMPO catalyst system for aerobic alcohol oxidation under mild conditions. Chem. Commun. 42, 5322–5324. doi: 10.1039/b509167e
Xie, J., Yin, K., Serov, A., Artyushkova, K., Pham, H. N., Sang, X., et al. (2017). Selective aerobic oxidation of alcohols over atomically-dispersed non-precious metal catalysts. ChemSusChem 10, 359–362. doi: 10.1002/cssc.201601364
Yang, M., Ling, Q., Yang, H., Li, C., and Zhang, A. (2014). Enhanced catalytic activity of K-birnessite MnO2 confined in carbon nanotubes for selective oxidation of benzyl alcohol. Catal. Commun. 46, 238–241. doi: 10.1016/j.catcom.2013.12.031
Yang, X., Wu, S., Hu, J., Fu, X., Peng, L., Kan, Q., et al. (2016). Highly efficient N-doped magnetic cobalt-graphene composite for selective oxidation of benzyl alcohol. Catal. Commun. 87, 90–93. doi: 10.1016/j.catcom.2016.09.015
Yu, H., Peng, F., Tan, J., Hu, X., Wang, H., Yang, J., et al. (2011). Selective catalysis of the aerobic oxidation of cyclohexane in the liquid phase by carbon nanotubes. Angew. Chem. Int. Ed. 50, 3978–3982. doi: 10.1002/anie.201007932
Yuan, Z., Liu, B., Zhou, P., Zhang, Z., and Chi, Q. (2018). Aerobic oxidation of biomass-derived 5-hydroxymethylfurfural to 2, 5-diformylfuran with cesium-doped manganese dioxide. Catal. Sci. Technol. 8, 4430–4439. doi: 10.1039/C8CY01246F
Yuvaraj, S., Fan-Yuan, L., Tsong-Huei, C., and Chuin-Tih, Y. (2003). Thermal decomposition of metal nitrates in air and hydrogen environments. J. Phys. Chem. B 107, 1044–1047. doi: 10.1021/jp026961c
Zhan, G., Huang, J., Du, M., Sun, D., Abdul-Rauf, I., Lin, W., et al. (2012). Liquid phase oxidation of benzyl alcohol to benzaldehyde with novel uncalcined bioreduction au catalysts: high activity and durability. Chem. Eng. J. 187, 232–238. doi: 10.1016/j.cej.2012.01.051
Zhang, E., Tian, H., Xu, S., Yu, X., and Xu, Q. (2013). Iron-catalyzed direct synthesis of imines from amines or alcohols and amines via aerobic oxidative reactions under air. Organ. Lett. 15, 2704–2707. doi: 10.1021/ol4010118
Zhao, L., Dong, G., Zhang, L., Lu, Y., and Huang, Y. (2019). Photocatalytic nitrogen oxide removal activity improved step-by-step through serial multistep Cu modifications. ACS Appl. Mater. Interfaces 11, 10042–10051. doi: 10.1021/acsami.9b00111
Zhou, W., Liu, J., Pan, J., Sun, F. A., He, M., and Chen, Q. (2015). Effect of Mg2+ on the catalytic activities of CoMgAl hydrotalcites in the selective oxidation of benzyl alcohol to benzaldehyde. Catal. Commun. 69, 1–4. doi: 10.1016/j.catcom.2015.05.012
Keywords: selective oxidation, benzyl alcohol oxidation, green oxidation, ferric nitrate, catalytic mechanism
Citation: Xu S, Wu J, Huang P, Lao C, Lai H, Wang Y, Wang Z, Zhong G, Fu X and Peng F (2020) Selective Catalytic Oxidation of Benzyl Alcohol to Benzaldehyde by Nitrates. Front. Chem. 8:151. doi: 10.3389/fchem.2020.00151
Received: 07 January 2020; Accepted: 17 February 2020;
Published: 20 March 2020.
Edited by:
Hadi Nur, University of Technology Malaysia, MalaysiaReviewed by:
Giovanni Palmisano, Khalifa University, United Arab EmiratesDong Guohui, Shaanxi University of Science and Technology, China
Copyright © 2020 Xu, Wu, Huang, Lao, Lai, Wang, Wang, Zhong, Fu and Peng. This is an open-access article distributed under the terms of the Creative Commons Attribution License (CC BY). The use, distribution or reproduction in other forums is permitted, provided the original author(s) and the copyright owner(s) are credited and that the original publication in this journal is cited, in accordance with accepted academic practice. No use, distribution or reproduction is permitted which does not comply with these terms.
*Correspondence: Guoyu Zhong, emhvbmdneUBkZ3V0LmVkdS5jbg==; Feng Peng, ZnBlbmdAZ3podS5lZHUuY24=