- State Key Laboratory of Quality Research in Chinese Medicine, Institute of Chinese Medical Sciences, University of Macau, Macau, China
Anabasine (ANA), a major piperidine alkaloid originally isolated from wild tobacco trees (Nicotiana glauca), has been known to induce serious developmental toxicities such as skeletal deformities in livestock and humans. In this study, we thoroughly investigated the supramolecular nano-encapsulations of ANA by an artificial nanocontainer, cucurbit[7] uril (CB[7]), and examined the influences of the nano-encapsulation on ANA's inherent developmental toxicities on a zebrafish model. We have shown that CB[7] formed 1:1 host-guest inclusion complexes with ANA via a relatively high binding strength [Ka of (7.45 ± 0.31) × 104 M−1] in an aqueous solution, via UV-vis and 1H nuclear magnetic resonance spectroscopic titrations, as well as isothermal titration calorimetry titration. As a consequence, CB[7] significantly attenuated the developmental toxicity of ANA on zebrafish in vivo. In contrast, for a comparative purpose, β-CD didn't exert any influence on the toxicity of ANA due to its weak binding with ANA, which was not even measurable via either spectroscopic methods or ITC titration. This is the first head-to-head comparison of this pair of nanocontainers, CB[7] and β-CD, on their potential roles in influencing the toxicity of guest molecules and the results suggested that CB[7] could become a more promising functional excipient for reducing the inherent toxicities of active pharmaceutical ingredients, particularly alkaloids that may form relatively strong host-guest binding species with the host.
Introduction
Anabasine (ANA) (Figure 1A), a major piperidine alkaloid originally isolated from wild tobacco trees (Nicotiana glauca), has been known to induce serious developmental toxicities, particularly skeletal deformities in livestock (such as goats and swine) and humans (Keeler et al., 1984; Panter et al., 1990; Green et al., 2013a,b). When human beings were presented with poison symptoms after accidental injection of tobacco plant (N. glauca), ANA was identified as a crucial substance causing clinical toxicity (Manoguerra and Freeman, 1982; Mellick et al., 1999; Sims et al., 1999; Mizrachi et al., 2000). ANA is a potential nicotinic acetylcholine receptor (nAChR) agonist, existing in tobacco smoke in a trace amount as it is mainly located from the stalks of cultivated tobacco (Jacob et al., 1999; Daly, 2005; Green et al., 2013b). Thus, ANA could be applied as an indicator of smoking (Jacob et al., 1999; Daly, 2005; Green et al., 2013b).
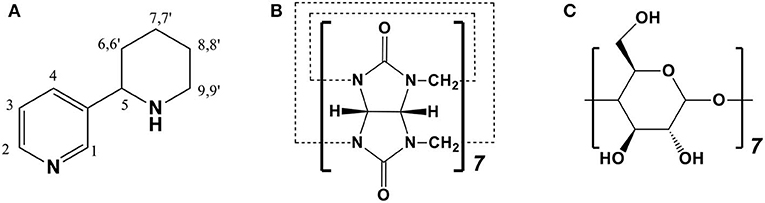
Figure 1. Chemical structures of (A) anabasine (ANA), (B) cucurbit[7]uril (CB[7]), and (C) beta-cyclodextrins (β-CD).
Cucurbit[n]uril (CB[n], n = 5–8, 10 in particular) is pumpkin-like nano-sized molecule comprised of n glycoluril connected by n pairs of methylene bridges (Lagona et al., 2005; Assaf and Nau, 2015). Compared to the well-known cyclodextrins (CDs) family, CB[n] family is relatively new group of macrocycles, comparable in many ways to CDs (Shetty et al., 2015). In the CB[n] family, CB[7] (Figure 1B) has caught the most attentions from researchers and has displayed a broad range of potential applications due to its promising biocompatibility, appropriate cavity size, superior molecular recognition ability and moderate solubility in water (Uzunova et al., 2010; Oun et al., 2014; Chen et al., 2015b; Kuok et al., 2017; Yin and Wang, 2018; Zhang et al., 2018). Although CB[7] and β-CD (Figure 1C) have rather similar internal cavity volume (279 Å3 vs. 263 Å3), CB[7] has generally higher binding affinities (Ka = 104-1017 M−1) with a variety of guest species than those of β-CD (Ka = 102-105 M−1).13 It has been reported that CB[7] may become an effective pharmaceutical excipient with several benefits (Chen et al., 2015a; Li et al., 2016; Yang et al., 2016, 2017; Huang et al., 2018a; Zhang et al., 2019). For example, it was demonstrated that CB[7] had the promising potential to serve as a taste-masking nanocontainer (Yang et al., 2017). Moreover, Zhang et al. also demonstrated that CB[7] may act as an oral antidote for paraquat poisoning (Zhang et al., 2019).
On the other hand, zebrafish (Danio rerio) is a common organism model being used in various biomedical researches. This teleost has high fecundity (200–300 eggs are laid by one adult female fish every week) and rapid ex utero development. The early development stages of zebrafish have been well-characterized. As zebrafish's embryo is transparent, the morphologies and internal organs could be well-observed by microscopy without sacrifice (Kimmerl et al., 1995; McGrath and Li, 2008). Thus, zebrafish is an ideal animal model for screening chemical compounds for toxicities. In the present study, we selected natural toxic compound, ANA, as the guest toxic molecule, examined its nano-encapsulation by the synthetic nanocontainer, CB[7], and investigated the possible role of macrocyclic nano-encapsulation in influencing the toxicity of ANA on zebrafish.
Materials and Methods
Ethics Statement
The animal experiments and associated experimental protocols were inspected and approved by the Animal Ethics Committee, University of Macau.
Reagents and Instrumentations
Anabasine (ANA) was purchased from Sigma Aldrich (USA), and β-CD was purchased from J&K (Shanghai, China). These chemicals were used without any further purification. CB[7] was prepared based on a published method by Day et al. (2001). Thermo LTQ Orbitrap XL coupled with an ESI/APcl multiprobe was employed to acquire ESI-MS data. Six hundred megahertz NMR (Bruker) spectrometer was employed to collect NMR spectra. A Confocal Imaging System (Olympus DSU) was used to record morphology of zebrafish.
Maintenance of Zebrafish
Zebrafish were originally procured from the ZFIN (Oregon). Zebrafish were kept and maintained at the Zebrafish Aquarium in the Institute of Chinese Medical Sciences, University of Macau. Zebrafish were maintained, bred and selected according to the Zebrafish Handbook, as was previously described (Chen et al., 2015b).
1H NMR Spectroscopy
Solution of ANA (50 μM) was prepared for 1H NMR characterization in D2O (pD = 7). An deuterated solution of ANA was mixed with different amount of CB[7], 0.6, 1.2, and 2.1 equiv. that of ANA, respectively, without changing the concentration of ANA. These solutions were characterized via 1H NMR spectroscopy (NS = 128 times).
UV-vis Spectroscopy
Two respective aqueous solutions (pH = 7) of ANA (50 μM) and CB[7] (50 μM) were prepared for Job's plot experiment. The samples were prepared by mixing ANA and CB[7] solutions of different volumes to make [CB[7]]/[[ANA] + CB[7]] from 0 to 1.0 at intervals of 0.1. During UV-vis spectroscopic titrations, an aqueous solution of ANA (50 μM) and an aqueous solution of 50 μM ANA and 250 μM CB[7] were prepared (pH = 7). Mixing of the two solutions with different volumes to have [CB[7]]/[ANA] varying from 0 to 5.0 at intervals of 0.5 was conducted and followed by UV-vis absorbance measurements. Same method was employed to prepare β-CD solution for UV-vis spectroscopic titrations with ANA.
ESI-MS Experiment
Aqueous solutions (pH = 7) containing the ANA (50 μM) and 5 equiv. CB[7] (250 μM), and neutral aqueous solution containing ANA (50 μM) and β-CD (1 mM) were prepared. They were subsequently filtered for ESI-MS analysis.
Isothermal Titration Calorimetry (ITC) Experiment
Aqueous solutions of ANA (200 μM) and β-CD (4 mM), ANA (100 μM) and CB[7] (1.5 mM) were prepared, respectively. The four solutions were subsequently degassed by centrifuge (13,000 rpm, 10 min) prior to use for titration. 0.2 mL ANA (200 μM) solution was placed in the sample cell, while 0.04 mL β-CD (4 mM) was placed to syringe for injection. Similarly, ANA (0.2 mL, 100 μM) was the sample cell, and 0.04 mL CB[7] was placed to syringe for injection. The ITC titrations were performed by titrating 19 aliquots (2 μL per drop) of β-CD or CB[7] solutions at room temperature, and the heat evolutions were recorded.
Developmental Toxicity Evaluations of ANA in Zebrafish
Wild type zebrafish embryo was employed as the in vivo model to assess the toxicity of ANA in the absence and in the presence of macrocyclic containers. Zebrafish embryos were known to take up chemical species mainly via skin (McGrath and Li, 2008), thus fish were simply incubated in media containing these compounds. The viable hatching rate, survival and morphology of zebrafish embryo were recorded. Zebrafish embryo of 24 hpf were randomly selected and distributed into a 6-well microplate (25–30 embryos/well). To evaluate the developmental toxicity of ANA alone in zebrafish, embryos were incubated in ANA solutions of 4 different concentrations (50, 100, 200, and 300 μM) without CB[7] or β-CD. Of important note, CB[7] was previously investigated to show excellent biocompatibility toward zebrafish embryos in these concentration range (Chen et al., 2015b). Additional experimental groups of embryos were, respectively, incubated in ANA solutions of these 4 different concentrations (50, 100, 200, and 300 μM) with 300 μM CB[7] and 1 mM β-CD (to ensure large excess of β-CD), respectively, for 2 days. At the end of incubation, the number of hatched larvae from each experimental group was recorded and the hatchability rate was analyzed. During the assessment of survival rate, zebrafish embryos (24 hpf) were dechorionated, and the dechorionated embryos were randomly selected and distributed into a 6-well microplate (25–30 embryos/well) and treated with ANA of different concentrations (50, 100, 200, and 300 μM) with or without 300 μM CB[7] or 1 mM β-CD, respectively, for 48 h. Survival rate was recorded by the end of the experiment. In addition, the morphology of zebrafish was measured via an Olympus Confocal Imaging System for further visual assessment.
Results and Discussions
Encapsulations of ANA by CB[7] and β-CD
The supramolecular nano-encapsulation of ANA by CB[7] was investigated via 1H NMR and UV-Vis spectroscopic titrations and ITC titration. As shown in Figure 2, all the protons of ANA exhibited an upfield shift after adding escalating concentration of CB[7], suggesting a full encapsulation of majority of ANA into the nano-cavity of CB[7], as the protons were shielded by the non-poplar cavity. Seilkhanov et al. studied the binding behavior between ANA and β-CD by NMR spectroscopy. Based on their results, ANA forms 1:1 host-guest binding pairs with β-CD with the piperidine fragment being included inside the cavity of CD (Seikhanov et al., 2016).
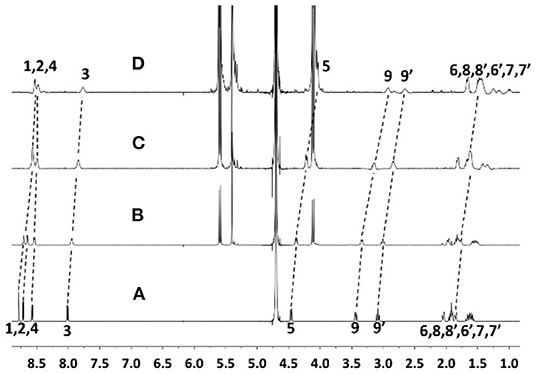
Figure 2. 1H NMR spectra of ANA (50 μM) (A) without and with (B) 0.6, (C) 1.2, and (D) 2.1 equiv. CB[7] in D2O (pD = 7) at room temperature.
UV-vis spectroscopic study was employed to assess the complexation ratio and binding affinity (Ka) between ANA and CB[7] (Figure 3). The Job's plot of ANA@CB[7] complex (Figure 3A) reached a maximum at 260 nm when [[CB[7]]/[CB[7]] + [ANA]] was 0.5, indicating a 1:1 complexation stoichiometry between ANA and CB[7]. UV-vis spectroscopic titration (Figure 3B) of ANA [0.05 mM) with increasing amounts of CB[7] (from 0 to 0.25 mM)] showed a moderate hypochromic shift. The non-linear least-squares fit was used for calculating the binding constant, affording a Ka of (7.45 ± 0.31) × 104 M−1 for ANA@CB[7] pair.
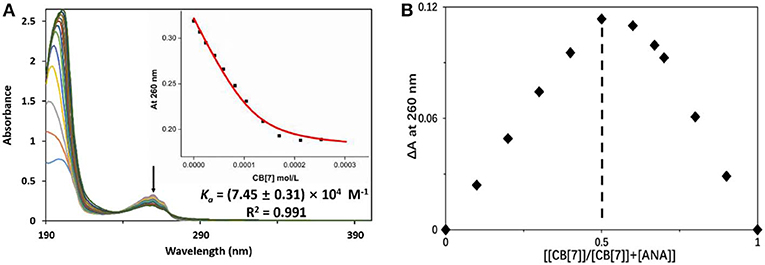
Figure 3. (A) UV-vis measurements of ANA (50 μM) in the presence of increasing concentration of CB[7] (up to 250 μM) in aqueous solution (pH = 7). Inset: the fitting curve of the titration data at 260 nm; (B) Job's plot of ANA@CB[7] system (with [ANA]+[CB[7]] = 50 μM) based on a continuous variation titration in aqueous solutions (pH = 7).
ESI-MS analysis was conducted to further confirm the complexation ratio between ANA and CB[7]. As shown in Figure 4, a doubly-charged peak at m/z = 663.24 and a singly-charged peak at m/z = 1325.46 were observed, corresponding to the [ANA+CB[7] + 2H]2+ complex (calculated m/z = 663.23) and [ANA+CB[7]+H]1+ complex (calculated m/z = 1325.46), respectively, confirming the 1:1 complex ratio between ANA and CB[7]. For comparative purpose, ESI-MS analysis of the mixture of ANA and β-CD did not generate any peaks attributed to host-guest complexations, implying that β-CD and ANA binds too weakly in aqueous solutions.
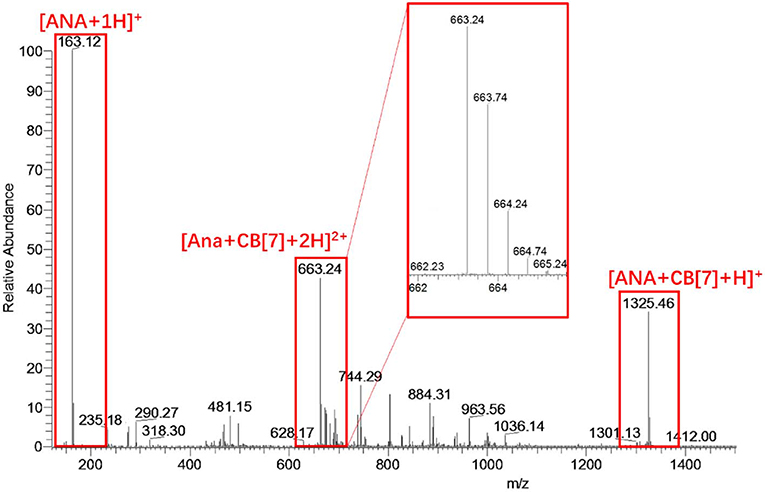
Figure 4. ESI-MS result of an aqueous solution of ANA and CB[7]. The singly-charged peak at m/z = 1325.46 and the doubly-charged peak at m/z = 663.24 suggested a likely 1:1 complexation ratio of ANA@CB[7].
ITC titration was conducted to further verify the relative strong binding between CB[7] and ANA. The result generated Ka = 1.40 × 105 M−1 shown in Figure 5A, consistent with UV-Vis titration results (Figure 3B). For a comparative purpose, ITC analysis of the mixture of ANA and β-CD was also conducted, however no meaningful thermal evolvement was observed (Figure 5B), suggesting the binding between β-CD and ANA was too weak in aqueous solutions. Collectively, all these results supported that CB[7] formed a relatively stable 1:1 supramolecular complex with ANA with a complexation affinity Ka of (7.45 ± 0.31) × 104 M−1, whereas the binding between β-CD and ANA was much weaker.
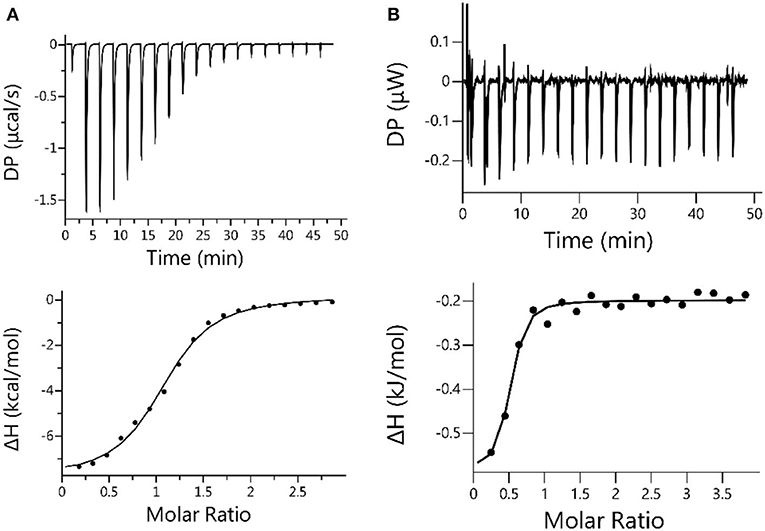
Figure 5. (A) Microcalorimetric measurements of ANA when titrated with CB[7] in water at room temperature. Top: Evolved thermogram during the titration. Bottom: Plot of ΔH against the molar ratio between CB[7] and ANA during the titration, with the best fit by using the “one set of binding sites” binding mode; (B) Microcalorimetric measurements of ANA when titrated with β-CD in water at room temperature. Top: Evolved thermogram of during the titration. Bottom: Plot of ΔH against the molar ratio between β-CD and ANA, with the best fit by using the “one set of binding sites” binding mode.
The Impact of Supramolecular Nano-Encapsulation on ANA's Inherent Developmental Toxicities in Zebrafish
Zebrafish embryos were incubated in different concentrations of ANA with or without CB[7] or β-CD. As was observed in Figure 6A, the hatching rate of the fish was decreased with increasing concentrations of ANA, confirming a dose-dependent developmental toxicity of ANA in zebrafish. Embryos incubated with the highest concentration (300 μM) of ANA led to <50% hatching rate. Very interestingly, the hatching rate of embryonic fish incubated with ANA@CB[7] complex exhibited almost the same rate as that of the control group (medium only), suggesting that CB[7] could dramatically alleviate the embryonic developmental toxicity of ANA in fish embryos. Of note, CB[7] alone did not exhibit any signs of toxicity in this study. In contrast, the hatching rate of embryos treated with ANA in the presence of a high concentration of β-CD decreased dramatically with increasing amount of ANA, and the viable hatching rate turned to zero with 300 μM ANA with 1 mM β-CD, which is dramatically lower than that in the free ANA treated group, suggesting that β-CD even somewhat worsened the toxicity of ANA. Similarly, the survival rate of zebrafish treated with free ANA exhibited a dose-dependent manner. In contrast, the survival rate of embryos incubated with ANA@CB[7] complex was maintained the same during 48 h incubation in spite of the increasing doses of ANA. While β-CD showed little effect on the toxicity of ANA in the survival study (Figure 6B).
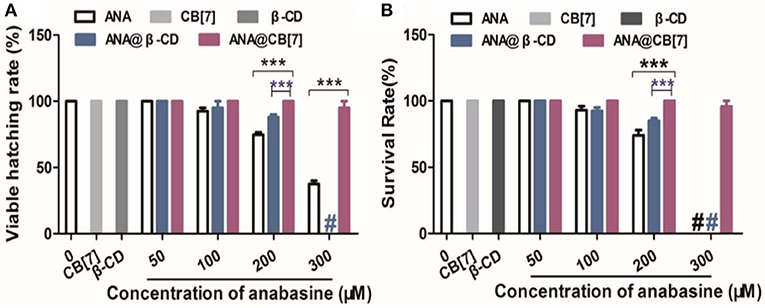
Figure 6. (A) The hatching percentage, and (B) survival rate of zebrafish embryos treated with ANA w and w/o CB[7] (300 μM) or β-CD (1 mM), respectively, for 2 days. Data are presented as mean ± S.E.M. (n = 25–30). *** denotes P < 0.001. # denotes that all zebrafish embryos/larva were dead.
In addition, the developmental morphologies of the fish were further examined. As shown in Figure 7, embryo larvae treated with ANA@CB[7] complex displayed normal morphology as well as usual developmental progress, comparable to those in the blank group. In a dramatic contrast, zebrafish larvae treated with free ANA and the mixture of ANA and β-CD both showed obvious developmental malformation, including the malformation of notochord, such as lordosis or cyphosis, yolk deformity, and abnormal head shape. Moreover, with the highest concentration (300 μM) of ANA with and without β-CD, 100% larva were dead. Taken together, CB[7] exhibited significant inhibition effect on the developmental toxicity of ANA whereas β-CD exhibited negligible or even negative effects.
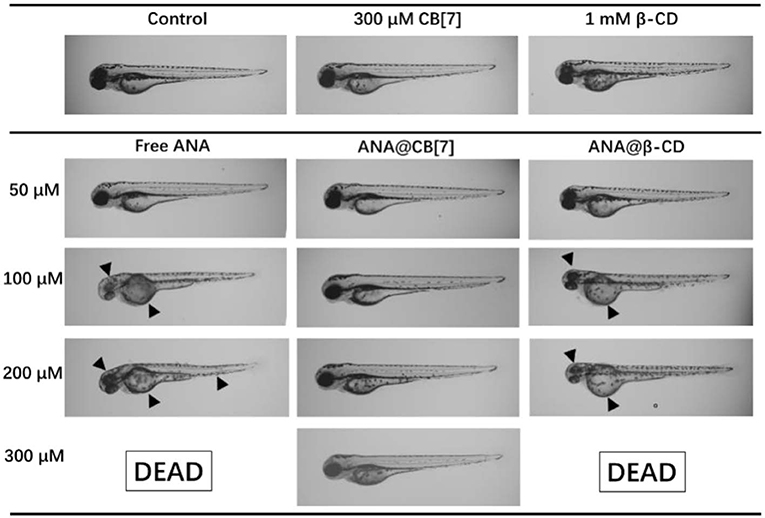
Figure 7. Morphology of representative zebrafish larvae (72 hpf) upon treatment with ANA w and w/o CB[7]/β-CD, respectively, for 48 h. Black arrowheads point to the abnormal morphologies.
Indeed, several works have previously demonstrated that the CB[n]s may alleviate toxicity of guest or included species. For instance, CB[8] played a critical role on formulating a safe, photo-responsive herbicide formulation (Gao et al., 2018). CB[7] was previously reported to inhibit the non-specific teratogenic toxicity of a couple of representative pesticides on a zebrafish model, through a facile supramolecular encapsulation (Yang et al., 2018). In addition, CB[7] was studied for its encapsulation of trazodone (TZ), an anti-depression drug approved by the FDA, but can cause liver damage due to its toxicity (Huang et al., 2018b). CB[7] formed binary host-guest complex with TZ in a relatively strong complexation affinity, and in vitro and in vivo studies indicated that the hepatotoxicity from TZ were alleviated by CB[7] encapsulation Our present ANA@CB[7] study, as a representative case for guest alkaloid, further supported that CB[7] could become a functional excipient to potentially alleviate undesired toxicities of selected drug or bioactive molecules. And this investigation is the first one to compare the influences of two macrocyclic molecules, CB[7] and β-CD, on the toxicity of a guest species, which may provide new insights on drug's formulations.
Conclusions
In summary, CB[7] was shown to form a 1:1 stable host-guest complex with a natural alkaloid—ANA, with a relatively high binding affinity. The supramolecular encapsulation exhibited promising inhibitory effects on the developmental toxicity of ANA in zebrafish. In addition, for comparative purpose, β-CD was also investigated in this study, however exhibited negligible effects on the toxicity of ANA. Although β-CD has served as a pharmaceutical excipient for decades, our present study demonstrated that CB[7] might become an important, complementary excipient to β-CD, particularly for reducing undesired toxicity of active compounds such as alkaloids.
Data Availability Statement
The datasets generated for this study are available on request to the corresponding author.
Ethics Statement
The animal study was reviewed and approved by the Animal Ethics Committee University of Macau.
Author Contributions
The project was conceptually designed by YG, YH, and YW. The majority of the experiments were performed by YG and XY, assisted by ZW and ZZ. Data analysis and interpretation were carried out by YG, XY, and YH. The manuscript was prepared by YG, XY, YH, and YW. All authors discussed the results and implications and commented on the manuscript.
Funding
This work was financially supported by the Macao Science and Technology Development Fund (FDCT 071/2017/A2 and SKL-QRCM-2020-2022), and the Research Fund of University of Macau (CPG2020-00016-ICMS).
Conflict of Interest
The authors declare that the research was conducted in the absence of any commercial or financial relationships that could be construed as a potential conflict of interest.
Acknowledgments
We are also grateful to Prof. Ruibing Wang for technical guidance and helpful discussions.
References
Assaf, K. I., and Nau, W. M. (2015). Cucurbiturils: from synthesis to high-affinity binding and catalysis. Chem. Soc. Rev. 44, 394–418. doi: 10.1039/C4CS00273C
Chen, H., Chan, J. Y. W., Li, S., Liu, J. J., Wyman, I. W., Lee, S. M. Y., et al. (2015a). In vivo reversal of general anesthesia by cucurbit[7]uril with zebrafish models. RSC Adv. 5, 63745–63752. doi: 10.1039/C5RA09406B
Chen, H., Chan, J. Y. W., Yang, X., Wyman, I. W., Bardelang, D., Macartney, D. H., et al. (2015b). Developmental and organ-specific toxicity of cucurbit[7]uril: in vivo study on zebrafish models. RSC Adv. 5, 30067–30074. doi: 10.1039/C5RA04335B
Daly, J. W. (2005). Nictotinic agonists, antagonists, and modulators from natural sources. Cell. Mol. Neurobiol. 25, 513–552. doi: 10.1007/s10571-005-3968-4
Day, A., Arnold, A. P., Blanch, R. J., and Snushal, B. (2001). Controlling factors in the synthesis of cucurbituril and its homologues. J. Org. Chem. 66, 8094–8100. doi: 10.1021/jo015897c
Gao, C., Huang, Q., Lan, Q., Feng, Y., Tang, F., Hoi, M. P. M., et al. (2018). A user-friendly herbicide derived from photo-responsive supramolecular vesicles. Nat. Commun. 9:2967. doi: 10.1038/s41467-018-05437-5
Green, B. T., Lee, S. T., Welch, K. D., and Panter, K. E. (2013a). Plant alkaloids that cause developmental defects through the disruption of cholinergic neurotransmission. Birth Defects Res. C 99, 235–246. doi: 10.1002/bdrc.21049
Green, B. T., Welch, K. D., Panter, K. E., and Lee, S. T. (2013b). Plant toxins that affect nicotinic acetylcholine receptors: a review. Chem. Res. Toxicol. 26, 1129–1138. doi: 10.1021/tx400166f
Huang, Q., Kuok, K. I., Zhang, X., Yue, X., Lee, S. M., Zhang, J., et al. (2018a). Inhibition of drug-induced seizure development in both zebrafish and mouse models by a synthetic nanoreceptor. Nanoscale 10, 10333–10336. doi: 10.1039/C8NR02041H
Huang, Q., Li, S., Yin, H., Wang, C., Lee, S. M. Y., and Wang, R. (2018b). Alleviating the hepatotoxicity of trazodone via supramolecular encapsulation. Food Chem. Toxicol. 112, 421–426. doi: 10.1016/j.fct.2017.12.016
Jacob, P., Yu, L., Shulgin, A. T., and Benowitz, N. L. (1999). Minor tobacco alkaloids as biomarkers for tobacco use: comparison of users of cigarettes, smokeless tobacco, cigars, and pipes. Am. J. Public Health 89, 731–736. doi: 10.2105/AJPH.89.5.731
Keeler, R. F., Crowe, M. W., and Lambert, E. A. (1984). Teratogenicity in swine of the tobacco alkaloid anabasine isolated from Nicotiana glauca. Teratology 30, 61–69. doi: 10.1002/tera.1420300109
Kimmerl, C. B., Ballard, W. W., Kimmel, S. R., Ullmann, B., and Schilling, T. F. (1995). Stages of embryonic development of the zebrafish. Dev. Dyn. 203, 253–310. doi: 10.1002/aja.1002030302
Kuok, K. L., Li, S., Wyman, I. W., and Wang, R. (2017). Cucurbit[7]uril: an emerging candidate for pharmaceutical excipients. Ann. N. Y. Acad. Sci. 1398, 108–119. doi: 10.1111/nyas.13376
Lagona, J., Mukhopadhyay, P., Chakrabarti, S., and Isaacs, L. (2005). The cucurbit[n]uril family. Angew. Chem. Int. Ed. Engl. 44, 4844–4870. doi: 10.1002/anie.200460675
Li, S., Chan, J. Y. W., Li, Y., Bardelang, D., Zheng, J., Yew, W. W., et al. (2016). Complexation of clofazimine by macrocyclic cucurbit[7]uril reduced its cardiotoxicity without affecting the antimycobacterial efficacy. Org. Biomol. Chem. 14, 7563–7569. doi: 10.1039/C6OB01060A
Manoguerra, A. S., and Freeman, D. (1982). Acute poisoning from the ingestion of Nicotiana glauca. J. Toxicol. Clin. Toxicol. 19, 861–864. doi: 10.3109/15563658208992520
McGrath, P., and Li, C. Q. (2008). Zebrafish: a predictive model for assessing drug-induced toxicity. Drug Discov. Today 13, 394–401. doi: 10.1016/j.drudis.2008.03.002
Mellick, L. B., Makowski, T., Mellick, G. A., and Borger, R. (1999). Neuromuscular blockade after ingestion of tree tobacco (Nicotiana glauca). Ann. Emerg. Med. 34, 101–104. doi: 10.1016/S0196-0644(99)70280-5
Mizrachi, N., Levy, S., and Goren, Z. (2000). Fatal poisoning from Nicotiana glauca leaves: identification of anabasine by gas-chromatography/mass spectrometry. J. Forensic Sci. 45, 736–741. doi: 10.1520/JFS14761J
Oun, R., Floriano, R. S., Isaacs, L., Rowan, E. G., and Wheate, N. J. (2014). The ex vivo neurotoxic, myotoxic and cardiotoxic activity of cucurbituril-based macrocyclic drug delivery vehicles. Toxicol. Res. 3, 447–455. doi: 10.1039/C4TX00082J
Panter, K. E., Bunch, R. F., Keeler, R. F., Sisson, D. V., and Callan, R. J. (1990). Multiple congenital contractures (mcc) and cleft palate induced in goats by ingestion of piperidine alkaloid-containing plants: reduction in fetal movement as the probable cause. J. Toxicol. Clin. Toxicol. 28, 69–83. doi: 10.3109/15563659008993477
Seikhanov, T. M., Nurkenov, O. A., Isaeva, A. Z., Nazarenko, L. A., and Seilkhanov, O. T. (2016). Preparation and NMR spectroscopic studies of the supramolecular inclusion complex of anabasine and β-cyclodextrin. Chem. Nat. Comp. 52, 1066–1068. doi: 10.1007/s10600-016-1862-6
Shetty, D., Khedkar, J. K., Park, K. M., and Kim, K. (2015). Can we beat the biotin–avidin pair?: cucurbit[7]uril-based ultrahigh affinity host–guest complexes and their applications. Chem. Soc. Rev. 44, 8747–8761. doi: 10.1039/C5CS00631G
Sims, D., James, R., and Christensen, T. (1999). Another death due to ingestion of Nicotiana glauca. J. Forensic Sci. 44, 447–449. doi: 10.1520/JFS14484J
Uzunova, V. D., Cullinane, C., Brix, K., Nau, W. M., and Day, A. I. (2010). Toxicity of cucurbit[7]uril and cucurbit[8]uril: an exploratory in vitro and in vivo study. Org. Biomol. Chem. 8, 2037–2042. doi: 10.1039/b925555a
Yang, X., Li, S., Zhang, Q. W., Zheng, Y., Bardelang, D., Wang, L. H., et al. (2017). Concealing the taste of the Guinness World's most bitter substance by using a synthetic nanocontainer. Nanoscale 9, 10606–10609. doi: 10.1039/C7NR03608F
Yang, X., Li, S. K., Lee, M. Y., Wang, L. H., and Wang, R. W. (2018). Constraining the teratogenicity of pesticide pollution by a synthetic nanoreceptor. Chem. Asian J. 13, 41–45. doi: 10.1002/asia.201701527
Yang, X., Wang, Z., Niu, Y., Chen, X., Lee, S. M. Y., and Wang, R. (2016). Influence of supramolecular encapsulation of camptothecin by cucurbit[7]uril: reduced toxicity and preserved anti-cancer activity. Med. Chem. Commun. 7, 1392–1397 doi: 10.1039/C6MD00239K
Yin, H., and Wang, R. (2018). Applications of cucurbit[n]urils (n=7 or 8) in pharmaceutical sciences and complexation of biomolecules. Isr. J. Chem. 58, 188–198. doi: 10.1002/ijch.201700092
Zhang, X., Xu, X., Li, S., Li, L., Zhang, J., and Wang, R. (2019). A synthetic receptor as a specific antidote for paraquat poisoning. Theranostics. 9, 633–645. doi: 10.7150/thno.31485
Keywords: supermolecular, anabasine, cucurbit[7]uril, toxicity, zebrafish
Citation: Gao Y, Yang X, Wang Z, Zhong Z, Hu Y and Wang Y (2020) Supramolecular Nano-Encapsulation of Anabasine Reduced Its Developmental Toxicity in Zebrafish. Front. Chem. 8:134. doi: 10.3389/fchem.2020.00134
Received: 09 January 2020; Accepted: 13 February 2020;
Published: 28 February 2020.
Edited by:
Fang Liu, Guangzhou University of Chinese Medicine, ChinaReviewed by:
Simin Liu, Wuhan University of Science and Technology, ChinaJunzhe Lou, Harvard University, United States
Copyright © 2020 Gao, Yang, Wang, Zhong, Hu and Wang. This is an open-access article distributed under the terms of the Creative Commons Attribution License (CC BY). The use, distribution or reproduction in other forums is permitted, provided the original author(s) and the copyright owner(s) are credited and that the original publication in this journal is cited, in accordance with accepted academic practice. No use, distribution or reproduction is permitted which does not comply with these terms.
*Correspondence: Yuanjia Hu, yuanjiahu@um.edu.mo; Yitao Wang, ytwang@um.edu.mo
†These authors have contributed equally to this work