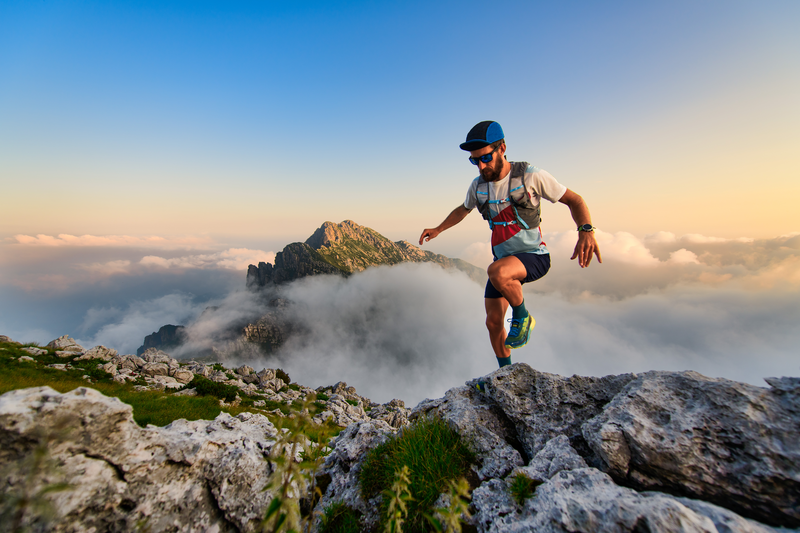
94% of researchers rate our articles as excellent or good
Learn more about the work of our research integrity team to safeguard the quality of each article we publish.
Find out more
MINI REVIEW article
Front. Chem. , 22 January 2020
Sec. Chemical Biology
Volume 7 - 2019 | https://doi.org/10.3389/fchem.2019.00931
This article is part of the Research Topic Probing the Ubiquitin Landscape View all 19 articles
The Ubiquitin CODE constitutes a unique post-translational modification language relying on the covalent attachment of Ubiquitin (Ub) to substrates, with Ub serving as the minimum entity to generate a message that is translated into different cellular pathways. The creation of this message is brought about by the dedicated action of writers, erasers, and readers of the Ubiquitin CODE. This CODE is greatly expanded through the generation of polyUb chains of different architectures on substrates thus regulating their fate. Through additional post-translational modification by Ub-like proteins (UbL), hybrid Ub/UbL chains, which either alter the originally encrypted message or encode a completely new one, are formed. Hybrid Ub/UbL chains are generated under both stress or physiological conditions and seem to confer improved specificity and affinity toward their cognate receptors. In such a manner, their formation must play a specific, yet still undefined role in cellular signaling and thus understanding the UbCODE message is crucial. Here, we discuss the evidence for the existence of hybrid Ub/UbL chains in addition to the current understanding of its biology. The modification of Ub by another UbL complicates the deciphering of the spatial and temporal order of events warranting the development of a hybrid chain toolbox. We discuss this unmet need and expand upon the creation of tailored tools adapted from our previously established toolkit for the Ubiquitin Proteasome System to specifically target these hybrid Ub/UbL chains.
Ubiquitin (Ub) is a 76 amino acid, highly conserved protein among eukaryotes post-translationally modifying proteins thereby dictating almost every fundamental cellular process. Malfunction of its action drives diverse pathologies such as cancer and neurological disorders like Parkinson's, Alzheimer's, and Huntington's disease (McNaught et al., 2001; Du and Mei, 2013; Ciechanover and Kwon, 2015). It exerts its action through the covalent attachment of its C-terminus to the target substrates by an orchestrated enzymatic cascade composed by three different enzyme families named E1, E2, E3 (writers of the code) (Figure 1A). This conjugation process, commonly referred to as ubiquitination, is initiated once the E1 activating enzyme catalyzes adenylation of the C-terminus of Ub at the expense of ATP thereby forming a high-energy E1-Ub thioester. Afterwards, the activated Ub is transferred by trans-thioesterification to the cysteine of the E2 conjugating enzyme which allows E3 ligase mediated Ub conjugation the substrate lysine residue through a stable peptide bond. Ub transfer to the substrate can be carried out by three different mechanisms depending on the nature of participating E3 ligase [RING, HECT, and RING-in-between-RING (RBR)] (Zheng and Shabek, 2017). Activated Ub can be transferred onto the catalytic cysteine of the HECT E3s via a transthioesterification reaction followed by conjugation to the lysine residue of the substrate. Alternatively, transfer of the E2-Ub thioester to the substrate lysine is accomplished by the contribution of a scaffolding RING E3 enzyme accommodating both the E2-Ub complex and the substrate. RBR E3s catalyze Ub conjugation by a concerted RING/HECT hybrid mechanism in which the RING1 domain recruits the E2-Ub complex, followed by thioester transfer of Ub to a cysteine in the RING2 domain (Spratt et al., 2014).
Figure 1. Complexity of the ubiquitin CODE (A) General overview ubiquitination process. (B) Ubiquitin chain types.
Different ubiquitination patterns can be observed depending on the constitution of the lysine residues of the substrate, giving rise to mono-ubiquitination or multi mono-ubiquitination, respectively. Additionally, this enzymatic process can be repeated by utilizing the ε-amine functionality of any of the seven internal lysine residues or the N-terminal amine of Ub. Thus, self-conjugation of Ub to any of these residues permits the formation of eight different homogenous polymeric Ub chains (M1, K6/11/27/29/33/48/63). Due to the different disposition adopted by each of these Ub linkages, a wide variety of cellular signaling (Akutsu et al., 2016) events can be modulated all exerting different biological outcomes. For instance, Lys-48 and Lys-63 linked poly-Ub, the best characterized polymeric chains are mainly involved in proteasome mediated protein degradation and cell signaling respectively, whereas the cellular responses of the remaining linkages, known as atypical chains, remains undefined (Kulathu and Komander, 2012). Furthermore, complexity can be augmented through permutation of linkages, either through modification of different internal lysines (branched chains) or by repetition of different linkages within the chain (heterologous/mixed chains) thereby endowing the UbCODE with an extraordinary versatility and specificity (Nakasone et al., 2013; Stolz and Dikic, 2018; Haakonsen and Rape, 2019) (Figure 1B).
To counterbalance ubiquitination and further sculpting the physiological effects or rescuing proteins destined for proteasomal degradation, dedicated proteases known as deubiquitinases (DUBs) not only remove mono-Ub from their substrates, but also alter Ub chain topology (editors of the code) (Leznicki and Kulathu, 2017). Alternatively, modulating the formation and processing of Ub chains can be achieved through their interaction with Ubiquitin Binding Domains (UBDs). These UBDs are endowed with a specific affinity toward Ub or Ub chains permitting the modulation of both chain elongation as well as governing the interaction of the Ub chains with the substrates (Dikic et al., 2009).
While Ubiquitin represents the minimum entity to express a code, the Ubiquitin CODE as coined by Komander and Rape, it is a highly complex, yet still elusive signaling system reliant on the interplay of its “writers,” “editors,” and “readers” (Komander and Rape, 2012). Nonetheless, intricacy arises when Ub, is further “PTMylated” by the classical modifications such as acetylation (Ohtake et al., 2015), phosphorylation (Herhaus and Dikic, 2015), or ribosylation (Vivelo et al., 2019), or even by some Ubiquitin-like proteins (UbL). Structurally and biochemically similar to Ub, UbLs are covalently attached to the lysine residues of their substrates through the sequential action of dedicated activating, conjugating, and ligating enzymes. Conjugation of UbLs to Ub and vice versa, results in hybrid chains, expanding the utility of the Ubiquitin CODE to enable an extensive crosstalk among the different UbL pathways and the UPS (Schmidt and Dikic, 2006; Schimmel et al., 2008; Geoffroy and Hay, 2009; Hjerpe et al., 2012a) (Figure 1B). However, the assembly, topology, architecture, as well as the encoded information of these Ub/UbLs hybrid chains remains cryptic warranting the development of suitable reagents to decipher this intricate CODE.
Given the breadth of this review, we will focus on evidence supporting the existence of these Hybrid Chains with ubiquitin-like modifiers mainly composed of Ub and the UbL proteins NEDD8, SUMO, and ISG15 as well as the future potential for this emerging field. Additionally, we will touch upon the crosstalk between the Ubiquitin and the Ubiquitin-like enzyme cascades that cooperate to form hybrid Ub/UbL chains.
SUMOylation, which is involved in a large plethora of fundamental cellular processes, is catalyzed through the interplay of specific enzymes and counteracted by the action of SUMO specific isopeptidases (Pichler et al., 2017). The SUMO family is composed by three different members known as SUMO-1, -2, and -3, which, subsequent to the exposure of their C-terminal di-glycine signature, are conjugated onto specific lysines embedded within a SUMO consensus motif (ψ-Lys-X-Glu, with ψ encoding a hydrophobic residue of their substrates) (Geiss-Friedlander and Melchior, 2007). While the most predominant isoforms SUMO-2 and SUMO-3 are virtually identical and mainly form K11-linked polymeric chains (Matic et al., 2008; Hendriks et al., 2014), SUMO-1 bears only a 50% sequence similarity and does not form polymeric chains give the absence of the necessary conserved lysine residue within the consensus motif (Saitoh and Hinchey, 2000). However, it has been shown that SUMO-1 can be linked to the end of a poly-SUMO-2/-3 chain, effectively terminating chain growth (Matic et al., 2008). Formation of SUMO-2/-3 chains is elicited upon cellular stressors such as heat shock (Saitoh and Hinchey, 2000) and their recognition is mediated by SUMO interactive motifs (SIMs)- specific regions interacting with SUMO and SUMO polymers (Song et al., 2004).
In addition to modification with SUMO itself, several proteomic studies have identified that Ubiquitination at various lysines in SUMO-1–3 can occur (Danielsen et al., 2011; Wagner et al., 2012; Hendriks et al., 2014; Hendriks and Vertegaal, 2016). Interestingly, while SUMO-1 cannot be SUMOylated, it is Ubiquitinated at six lysine residues most likely inducing a different response than Ubiquitinated SUMO-2/3 (Hendriks and Vertegaal, 2016). Thus, given the sheer number of Ubiquitination sites in SUMO a plethora of hybrid chains combinations are possible.
Intriguingly, proteomics revealed not only the vast number of modification possibilities on the different SUMO isoforms, but also allowed to identify whether the modification occurs on SUMO or on the Ubiquitin lysines (Hendriks et al., 2014, 2017), further increasing the complexity (Figure 2A). The hybrid chains predominantly occur upon specific stressors (Hendriks et al., 2014) (Figure 2A) and despite the advances in detection and elucidation of the branched architecture of SUMO-Ub hybrid chains, comprehending their cellular function is still in its infancy. Discerning their physiological roles is of utmost importance since the architecture of hybrid SUMO-Ub chains expands the potential for distinct signaling events by SUMO and Ub.
Figure 2. Mechanism for the formation of hybrid chains. (A) StubLs containing tandem of SIMS recognize polySUMO2/3 chains and poly-ubiquitinate in a linkage specific manner the PolySUMO chains targeting them for enhanced proteasomal degradation (Aillet et al., 2012) or initiates signaling for DDR events through the RAP80/BRCA1 complex (Guzzo et al., 2012). The insert highlights ubiquitinitated SUMO1-3 (Hendriks and Vertegaal, 2016) and SUMOylated Ubiquitin identified by proteomics so far (Hendriks et al., 2014). (B) Canonical and atypical pathways (Leidecker et al., 2012) and dual activity of HUWE1 under stress conditions which lead to formation of hybrid chains which protect the UPS by via the formation of aggregates that are no longer processed by the proteasome (Maghames et al., 2018). The insert displays identified Ub-Nedd8 linkages (Leidecker et al., 2012). (C) Although the mechanism for the formation of ISG15 hybrid chains is still outstanding, data supporting the existence of Ub-ISG15 is available. First, K29 gets ISGylated, followed by K48 as the second ISG15 site (Fan et al., 2015).
Hybrid chains can be recognized by a variety of receptors containing tandem SUMO-interacting motifs (SIMs) and UBDs. Moreover, to counterbalance or alter the effect of SUMOylation, subsequent ubiquitination of poly-SUMOylated proteins, catalyzed by SUMO-targeted Ubiquitin ligases (STUbls) can ensue. Upon recognition of the poly-SUMO signal through virtue of their SIMs, STUbls install a specific Ub-linkage onto the lysine of the SUMO-modification (Sriramachandran and Dohmen, 2014). Although, SUMO-Ub chains were primarily identified on proteins impending proteasomal degradation (Lallemand-Breitenbach et al., 2008; Tatham et al., 2008; Erker et al., 2013; McIntosh et al., 2018), roles in maintaining genome stability (Guzzo et al., 2012; Nie and Boddy, 2016) have been assigned more recently through the K63 poly-ubiquitination of poly-SUMO chains (Figure 2A). Here RNF4, a STUbl, mediates poly-ubiquitination of SUMOylated proteins, thereby evoking the recruitment of RAP80 and its subsequent interaction with BRCA1 complex, to promote genomic stability (Guzzo et al., 2012). Another example involves Arkadia which ubiquitinates SUMOylated xeroderma pigmentosum C (XPC), a pivotal player in nucleotide excision repair, driving XPC to UV-damaged DNA sites (Poulsen et al., 2013).
DUBs such as USP11 can trim or reverse ubiquitination on hybrid SUMO-Ub chains to modulate the associated cellular responses (Hendriks et al., 2015). Thus, the amalgamation of ubiquitination and SUMOylation resembles an efficacious strategy to confer both specificity and increased affinity to the target proteins (Aillet et al., 2012; Guzzo et al., 2012).
Akin to SUMOylation, Neddylation is accomplished by its own specific enzymes and is counterbalanced by a few dedicated proteases (Enchev et al., 2015). Given the similarities between Ubiquitin and Nedd8, it is unsurprising that both have the propensity to form hybrid chains. However, the formation of Ubiquitin-Nedd8 hybrid chains occurs predominantly in response to proteotoxic stress, perhaps as a mechanism to dampen cellular signaling in this context or to protect the UPS from proteotoxicity (Maghames et al., 2018; Santonico, 2019). Neddylation and the Nedd8 enzyme cascade have been demonstrated to be crucial to the development of neurological disorders (Dil Kuazi et al., 2003; Mori et al., 2005; Chen et al., 2012; Lu et al., 2013). Thus, given the protective role of Ubiquitin-Nedd8 hybrid chains against proteotoxic stress, these complex posttranslational modifications may play a pivotal role in the pathogenesis and progression of neurodegenerative diseases (Ross and Poirier, 2004; Gestwicki and Garza, 2012; Dantuma and Bott, 2014; Valastyan and Lindquist, 2014; Sweeney et al., 2017).
In an attempt to elucidate the architecture of the hybrid chains several hybrid linkages were determined by SILAC-based proteomics upon proteasomal inhibition (Leidecker et al., 2012) (Figure 2B). Neddylation occurs via the interplay of enzymes relying on its own specificity and is referred to as the “Canonical” pathway. However, under stress conditions such as proteasome inhibition, oxidative stress, or heat shock Neddylation is mediated “atypically” by the Ubiquitin activating enzyme UBE1 instead (Hjerpe et al., 2012a,b; Leidecker et al., 2012). This tight synchronization of the Ubiquitin and Nedd8 systems to fine-tune the cellular response during proteotoxic stress has been observed not only for UBE1, but also for also for the E3 ligase HUWE1, a crucial component of the Protein Quality Control (PQC) pathway (Xirodimas et al., 2008; Sung et al., 2016a,b), which targets ribosomal proteins (RPs) and protects the UPS from stress-induced toxicity by ribosomal protein aggregation (Maghames et al., 2018) (Figure 2B). Importantly, during the persistence of stress, the unconjugated Ub pool is rapidly depleted triggering Neddylation through the Ubiquitin pathway and targeting several substrates typically ubiquitinated (Leidecker et al., 2012) (Figure 2B). Akin to the sophisticated regulatory system provided by DUBs, research underscores that Nedd8-Ub hybrid chains seem to be modulated in a similar fashion by DUBs subsequent to cellular stress (Leidecker et al., 2012; Singh et al., 2012, 2014).
Firstly identified upon IFN treatment on Ehrlich ascites tumor cells (Farrell et al., 1979), ISG15 had initially not been identified as an Ubiquitin-like protein, until cross-reactivity toward Ub antibodies suggested the existence of UbL proteins (Haas et al., 1987). Unlike all other UbLs, ISG15 is composed of two Ub like domains tethered by a “hinge” polypeptide sequence. Analogous to Ub, ISG15 can be conjugated onto the target substrates through the orchestrated interplay of its E1, E2, and E3 enzymes through its exposed C-terminal glycine (Perng and Lenschow, 2018). Given its increased activation upon interferon stimulation, conjugation of ISG15 to protein substrates plays a crucial role in the antiviral response and thereby constituting a key contributor to innate immunity (Harty et al., 2009; Durfee et al., 2010; Perng and Lenschow, 2018).
In contrast to Ub, SUMO and NEDD8 (Jones et al., 2008), ISG15 has not been reported to generate polymeric chains and does not seem to have specific ISG15-interacting motifs. Although some studies have suggested an antagonistic relationship of Ub and ISG15 in certain contexts such as during tumorigenesis (Liu et al., 2003; Desai et al., 2006; Kim et al., 2006; Malakhova and Zhang, 2008; Wood et al., 2011), evidence of a crosstalk between ISG15 and Ub conjugation pathways still remains perplexing. Unexpectedly, a proteomic study revealed that ISG15 was conjugated to Ub (Giannakopoulos et al., 2005), and further investigation by Zhang et al. corroborated the formation of hybrid ISG15-Ub chains (Fan et al., 2015) (Figure 2C).
Little is known about the biological function of these hybrid Ub-ISG15 chains, but it has been established that they do not act as proteasomal degradation signals. Thus, ISG15 could potentially function as a chain termination moiety to rescue ubiquitylated proteins from degradation. However, given the fact that ISG15 is predominantly conjugated to Ub via K29, a plausible role of these hybrid chains could be modulation of K29-Ub mediated biological signaling (Kulathu and Komander, 2012). Moreover, this type of hybrid chains could trigger new signaling pathways exerting different biological outcomes, but the assignment of their biological role is a daunting task since no ISG15 interactive motifs have been identified and readers containing both UIM and ISG15 interacting motifs cannot be predicted.
Considering the impact of ubiquitination on regulating a vast array of fundamental biological processes, with dysregulation of the dedicated enzymes giving rise to pathologies such as cancer and neurodegenerative diseases, understanding its function merited the development and innovation of respective tools. Advances in synthetic strategies for generating ubiquitin, constituted a qualitative leap forward in the development of a plethora of ubiquitin assay reagents and numerous activity-based probes (ABPs) enabling study of enzymes involved in the complex system of ubiquitination (El Oualid et al., 2010; Ekkebus et al., 2013; Hameed et al., 2017).
The modification of Ub by another UbL complicates the deciphering of the spatial and temporal order of events, as well as the underlying biological role of this modification, underscoring the urgent need for new next generation ABPs and assay reagents. The lack of a robust methodology to chemically access some UbL proteins has hampered the study on the biological role that hybrid chains display as well as the identification of their readers, writers, erasers, and interpreters. Generating such complex hybrid chains is a challenging feat as the E2/E3 enzymes generating these linkages in vitro remain unknown. So far, only (semi)-synthetic strategies for obtaining ubiquitinated Rub1, the yeast NEDD8 homolog (Singh et al., 2014) and SUMO-2-K63diUb hybrid chains (Bondalapati et al., 2017) have been reported. Only in the last decade, efforts to devise synthetic strategies for UbL proteins such as Nedd8 (Mulder et al., 2014), SUMO (Dobrota et al., 2012; Wucherpfennig et al., 2014; Mulder et al., 2018) and Ufm1 (Ogunkoya et al., 2012; Witting et al., 2018) have been undertaken. More recently, ISG15 synthesis has been accomplished as a modular synthesis of both domains and its subsequent ligation (Xin et al., 2019). These developments in the chemical synthesis of UbL proteins in combination with the advancements made in polyUb probes (Mulder et al., 2014; Flierman et al., 2016; Paudel et al., 2019) open a new avenue to UbL and hybrid Ub/UbL reagents allowing research on their respective enzymatic cascades, but also enabling in depth studies on their crosstalk with ubiquitin.
Mass spectrometry (MS) has become an invaluable tool in the quest for understanding cell signaling and in particular to study the UPS (Heap et al., 2017). This type of proteomics relies on the isolation and enrichment of the target proteins through affinity-based approaches (Mattern et al., 2019) such as affimers, antibodies targeting the di-Glycine signature, anti/mini/nanobodies, endogenous tags, biotin, and molecular entities based in the repetition of UBDs and SIMs capturing poly-Ub and SUMO chains, respectively (TUBES and SUBES) (Hjerpe et al., 2009; Da Silva-Ferrada et al., 2013) with a high affinity. However, many of these approaches cannot be undertaken in the study toward hybrid Ub-UbL biology since they are not endowed with specific affinity toward these linkages or due to the shared homology under Ub and UbL proteins as exemplified by the shared GG remnant after enzymatic digestion. To overcome these pitfalls, an UbiSite antibody approach (Akimov et al., 2018) which relies on LysC digestion has recently been described to allow differentiation among Ub and UbL proteins. The translation of the existing affinity technologies toward hybrid chains and UbL proteins would facilitate the understanding of the crosstalk among the different Ub-UbL proteins. For example, an elegant combination of SIMs and UBDs, a mixed TUBE/SUBE approach, could potentially enrich for substrates endowed with hybrid chains generated by STUbLs. Unsurprisingly due to the high similarity of Nedd8 and Ub, all known binding domains with affinity for Nedd8 display cross-reactivity with Ub. Recently, the first specific binding domain for Nedd8 was reported (Castagnoli et al., 2019) and thus a similar approach as the TUBES/SUBES could potentially be designed, “NEBES.”
Furthermore, a proteomic approach called Ubi-clipping (Swatek et al., 2019) has shown the great percentage (10–20%) of which branched chains are present in polymeric forms of Ub. This method relies on an engineered version of an ISG15-specificenzyme that partially removes Ub from substrates and leave the characteristic diglycine signature on Ub while simultaneously allowing the identification of different branched architectures. The translation of such technology into the hybrid chains field would shed light on the different architectures that such chains exhibit. In addition to this innovation, the generation of specific antibodies toward the linkage of hybrid chains, in a similar fashion as the first Ub branched K11/K48 antibody (Yau et al., 2017) could be a feasible approach toward the generation a Hybrid Chain Tool Box.
Despite the recent advances made in developing innovative reagents on the Ubiquitin-field, there are still many conundrums to be resolved regarding the writers, editors and readers of this part of the Ub CODE. The origin of the identified Ub-SUMO linkages in which Ub is SUMOylated is still unclear, the possibility of a parallel mechanism such as the STUbL in which SUMO ligases target polyUb-chains and SUMOylate (UbTSLs) them might explain their existence. The enzymes catalyzing the formation of Ub-ISG15 hybrid chains are still unknown and efforts to identify them should be undertaken. Moreover, the formation of these hybrid chains confers an extra layer of complexity to the CODE that could be translated into terms of specificity and increased affinity that the “readers” display for them. Such readers must be endowed with “hybrid” recognition domains which could be screened by bioinformatic analysis as exemplified in the discovery of RAP80 (Nie and Boddy, 2016). It has been shown that hybrid chains are processed by the proteasome more efficiently compared with poly-Ub or poly-SUMO chains. This pronounced affinity could be derived from the improved recognition of either a proteasome subunit or of a shuttle protein containing the aforementioned “hybrid” recognition domains. For the Ub-Nedd8 and Ub-ISG15 hybrid chains, the field is less explored and hybrid chain recognition domains still need to be identified.
The fact that Ub and UbL proteins can generate this array of chains, conferring new architectures and topology to the chains and thereby triggering different signaling events, increases complexity of the already intricate Ubiquitin CODE. The current knowledge regarding hybrid-chain formation is based upon chain formation between Ub and UbL proteins. However, a recent report revealed that a small fraction of NEDD8 becomes modified by K0-SUMO (Hendriks et al., 2017). Although SUMOylation of NEDD8 is likely to be a very rare event, it does extend the knowledge regarding hybrid chain cross-talk and opens a new perspective to the intrinsic code (Hendriks et al., 2014). The creation of tailored tools specific toward these hybrid chains by adapting the methodology already applied for the study of the Ubiquitin Proteasome System will augment our knowledge about hybrid chains.
DP, KW, and MM wrote sections of the manuscript. All authors contributed to manuscript revision, read, and approved the submitted version.
This work was supported by the European Union's Horizon 2020 research and innovation programme under the Marie Skłodowska-Curie grant agreement No. 765445. MM was supported by an Off Road grant (ZonMw #451001024).
HO is shareholder of the reagent company UbiQ Bio BV.
The remaining authors declare that the research was conducted in the absence of any commercial or financial relationships that could be construed as a potential conflict of interest.
Aillet, F., Lopitz-Otsoa, F., Egaña, I., Hjerpe, R., Fraser, P., Hay, R. T., et al. (2012). Heterologous SUMO-2/3-ubiquitin chains optimize IκBα degradation and NF-κB activity. PLoS ONE 7:e51672. doi: 10.1371/journal.pone.0051672
Akimov, V., Barrio-Hernandez, I., Hansen, S. V. F., Hallenborg, P., Pedersen, A.-K., Bekker-Jensen, D. B., et al. (2018). UbiSite approach for comprehensive mapping of lysine and N-terminal ubiquitination sites. Nat. Struct. Mol. Biol. 25, 631–640. doi: 10.1038/s41594-018-0084-y
Akutsu, M., Dikic, I., and Bremm, A. (2016). Ubiquitin chain diversity at a glance. J. Cell Sci. 129, 875–880. doi: 10.1242/jcs.183954
Bondalapati, S., Eid, E., Mali, S. M., Wolberger, C., and Brik, A. (2017). Total chemical synthesis of SUMO-2-Lys63-linked diubiquitin hybrid chains assisted by removable solubilizing tags. Chem. Sci. 8, 4027–4034. doi: 10.1039/C7SC00488E
Castagnoli, L., Mandaliti, W., Nepravishta, R., Valentini, E., Mattioni, A., Procopio, R., et al. (2019). Selectivity of the CUBAN domain in the recognition of ubiquitin and NEDD8. FEBS J. 286, 653–677. doi: 10.1111/febs.14752
Chen, Y., Neve, R. L., and Liu, H. (2012). Neddylation dysfunction in Alzheimer's disease. J. Cell. Mol. Med. 16, 2583–2591. doi: 10.1111/j.1582-4934.2012.01604.x
Ciechanover, A., and Kwon, Y. T. (2015). Degradation of misfolded proteins in neurodegenerative diseases: therapeutic targets and strategies. Exp. Mol. Med. 47:e147. doi: 10.1038/emm.2014.117
Da Silva-Ferrada, E., Xolalpa, W., Lang, V., Aillet, F., Martin-Ruiz, I., de la Cruz-Herrera, C. F., et al. (2013). Analysis of SUMOylated proteins using SUMO-traps. Sci. Rep. 3:1690. doi: 10.1038/srep01690
Danielsen, J. M., Sylvestersen, K. B., Bekker-Jensen, S., Szklarczyk, D., Poulsen, J. W., Horn, H., et al. (2011). Mass spectrometric analysis of lysine ubiquitylation reveals promiscuity at site level. Mol. Cell. Proteomics 10:M110.003590. doi: 10.1074/mcp.M110.003590
Dantuma, N. P., and Bott, L. C. (2014). The ubiquitin-proteasome system in neurodegenerative diseases: precipitating factor, yet part of the solution. Front. Mol. Neurosci. 7:70. doi: 10.3389/fnmol.2014.00070
Desai, S. D., Haas, A. L., Wood, L. M., Tsai, Y. C., Pestka, S., Rubin, E. H., et al. (2006). Elevated expression of ISG15 in tumor cells interferes with the ubiquitin/26S proteasome pathway. Cancer Res. 66, 921–928. doi: 10.1158/0008-5472.CAN-05-1123
Dikic, I., Wakatsuki, S., and Walters, K. J. (2009). Ubiquitin-binding domains — from structures to functions. Nat. Rev. Mol. Cell Biol. 10:659. doi: 10.1038/nrm2767
Dil Kuazi, A., Kito, K., Abe, Y., Shin, R.-W., Kamitani, T., and Ueda, N. (2003). NEDD8 protein is involved in ubiquitinated inclusion bodies. J. Pathol. 199, 259–266. doi: 10.1002/path.1283
Dobrota, C., Fasci, D., Hadade, N. D., Roiban, G. D., Pop, C., Meier, V. M., et al. (2012). Glycine fluoromethylketones as SENP-specific activity based probes. Chembiochem 13, 80–84. doi: 10.1002/cbic.201100645
Du, W., and Mei, Q.-B. (2013). Ubiquitin-proteasome system, a new anti-tumor target. Acta Pharmacol. Sin. 34, 187–188. doi: 10.1038/aps.2012.192
Durfee, L. A., Lyon, N., Seo, K., and Huibregtse, J. M. (2010). The ISG15 conjugation system broadly targets newly synthesized proteins: implications for the antiviral function of ISG15. Mol. Cell 38, 722–732. doi: 10.1016/j.molcel.2010.05.002
Ekkebus, R., van Kasteren, S. I., Kulathu, Y., Scholten, A., Berlin, I., Geurink, P. P., et al. (2013). On terminal alkynes that can react with active-site cysteine nucleophiles in proteases. J. Am. Chem. Soc. 135, 2867–2870. doi: 10.1021/ja309802n
El Oualid, F., Merkx, R., Ekkebus, R., Hameed, D. S., Smit, J. J., de Jong, A., et al. (2010). Chemical synthesis of ubiquitin, ubiquitin-based probes, and diubiquitin. Angew. Chem. 49, 10149–10153. doi: 10.1002/anie.201005995
Enchev, R. I., Schulman, B. A., and Peter, M. (2015). Protein neddylation: beyond cullin-RING ligases. Nat. Rev. Mol. Cell Biol. 16, 30–44. doi: 10.1038/nrm3919
Erker, Y., Neyret-Kahn, H., Seeler, J. S., Dejean, A., Atfi, A., and Levy, L. (2013). Arkadia, a novel SUMO-targeted ubiquitin ligase involved in PML degradation. Mol. Cell. Biol. 33, 2163–2177. doi: 10.1128/MCB.01019-12
Fan, J.-B., Arimoto, K.-L., Motamedchaboki, K., Yan, M., Wolf, D. A., and Zhang, D.-E. (2015). Identification and characterization of a novel ISG15-ubiquitin mixed chain and its role in regulating protein homeostasis. Sci. Rep. 5:12704. doi: 10.1038/srep12704
Farrell, P. J., Broeze, R. J., and Lengyel, P. (1979). Accumulation of an mRNA and protein in interferon-treated Ehrlich ascites tumour cells. Nature 279, 523–525. doi: 10.1038/279523a0
Flierman, D., van Noort, G. J. V., Ekkebus, R., Geurink, P. P., Mevissen, T. E. T., Hospenthal, M. K., et al. (2016). Non-hydrolyzable diubiquitin probes reveal linkage-specific reactivity of deubiquitylating enzymes mediated by S2 pockets. Cell Chem. Biol. 23, 472–482. doi: 10.1016/j.chembiol.2016.03.009
Geiss-Friedlander, R., and Melchior, F. (2007). Concepts in sumoylation: a decade on. Nat. Rev. Mol. Cell Biol. 8, 947–956. doi: 10.1038/nrm2293
Geoffroy, M. C., and Hay, R. T. (2009). An additional role for SUMO in ubiquitin-mediated proteolysis. Nat. Rev. Mol. Cell Biol. 10, 564–568. doi: 10.1038/nrm2707
Gestwicki, J. E., and Garza, D. (2012). Protein quality control in neurodegenerative disease. Prog. Mol. Biol. Transl. Sci. 107, 327–353. doi: 10.1016/B978-0-12-385883-2.00003-5
Giannakopoulos, N. V., Luo, J. K., Papov, V., Zou, W., Lenschow, D. J., Jacobs, B. S., et al. (2005). Proteomic identification of proteins conjugated to ISG15 in mouse and human cells. Biochem. Biophys. Res. Commun. 336, 496–506. doi: 10.1016/j.bbrc.2005.08.132
Guzzo, C. M., Berndsen, C. E., Zhu, J., Gupta, V., Datta, A., Greenberg, R. A., et al. (2012). RNF4-dependent hybrid SUMO-ubiquitin chains are signals for RAP80 and thereby mediate the recruitment of BRCA1 to sites of DNA damage. Sci. Signal. 5:ra88. doi: 10.1126/scisignal.2003485
Haakonsen, D. L., and Rape, M. (2019). Branching out: improved signaling by heterotypic ubiquitin chains. Trends Cell Biol. 29, 704–716. doi: 10.1016/j.tcb.2019.06.003
Haas, A. L., Ahrens, P., Bright, P. M., and Ankel, H. (1987). Interferon induces a 15-kilodalton protein exhibiting marked homology to ubiquitin. J. Biol. Chem. 262, 11315–11323.
Hameed, D. S., Sapmaz, A., and Ovaa, H. (2017). How chemical synthesis of ubiquitin conjugates helps to understand ubiquitin signal transduction. Bioconjug. Chem. 28, 805–815. doi: 10.1021/acs.bioconjchem.6b00140
Harty, R. N., Pitha, P. M., and Okumura, A. (2009). Antiviral activity of innate immune protein ISG15. J. Innate Immun. 1, 397–404. doi: 10.1159/000226245
Heap, R. E., Gant, M. S., Lamoliatte, F., Peltier, J., and Trost, M. (2017). Mass spectrometry techniques for studying the ubiquitin system. Biochem. Soc. Trans. 45, 1137–1148. doi: 10.1042/BST20170091
Hendriks, I. A., D'Souza, R. C., Yang, B., Verlaan-de Vries, M., Mann, M., and Vertegaal, A. C. (2014). Uncovering global SUMOylation signaling networks in a site-specific manner. Nat. Struct. Mol. Biol. 21, 927–936. doi: 10.1038/nsmb.2890
Hendriks, I. A., Lyon, D., Young, C., Jensen, L. J., Vertegaal, A. C., and Nielsen, M. L. (2017). Site-specific mapping of the human SUMO proteome reveals co-modification with phosphorylation. Nat. Struct. Mol. Biol. 24, 325–336. doi: 10.1038/nsmb.3366
Hendriks, I. A., Schimmel, J., Eifler, K., Olsen, J. V., and Vertegaal, A. C. (2015). Ubiquitin-specific protease 11 (USP11). Deubiquitinates hybrid Small Ubiquitin-like Modifier (SUMO)-ubiquitin chains to counteract RING Finger Protein 4 (RNF4). J. Biol. Chem. 290, 15526–15537. doi: 10.1074/jbc.M114.618132
Hendriks, I. A., and Vertegaal, A. C. (2016). A comprehensive compilation of SUMO proteomics. Nat. Rev. Mol. Cell Biol. 17, 581–595. doi: 10.1038/nrm.2016.81
Herhaus, L., and Dikic, I. (2015). Expanding the ubiquitin code through post-translational modification. EMBO Rep. 16, 1071–1083. doi: 10.15252/embr.201540891
Hjerpe, R., Aillet, F., Lopitz-Otsoa, F., Lang, V., England, P., and Rodriguez, M. S. (2009). Efficient protection and isolation of ubiquitylated proteins using tandem ubiquitin-binding entities. EMBO Rep. 10, 1250–1258. doi: 10.1038/embor.2009.192
Hjerpe, R., Thomas, Y., Chen, J., Zemla, A., Curran, S., Shpiro, N., et al. (2012a). Changes in the ratio of free NEDD8 to ubiquitin triggers NEDDylation by ubiquitin enzymes. Biochem. J. 441, 927–936. doi: 10.1042/BJ20111671
Hjerpe, R., Thomas, Y., and Kurz, T. (2012b). NEDD8 overexpression results in neddylation of ubiquitin substrates by the ubiquitin pathway. J. Mol. Biol. 421, 27–29. doi: 10.1016/j.jmb.2012.05.013
Jones, J., Wu, K., Yang, Y., Guerrero, C., Nillegoda, N., Pan, Z. Q., et al. (2008). A targeted proteomic analysis of the ubiquitin-like modifier nedd8 and associated proteins. J. Proteome Res. 7, 1274–1287. doi: 10.1021/pr700749v
Kim, K. I., Yan, M., Malakhova, O., Luo, J. K., Shen, M. F., Zou, W., et al. (2006). Ube1L and protein ISGylation are not essential for alpha/beta interferon signaling. Mol. Cell. Biol. 26, 472–479. doi: 10.1128/MCB.26.2.472-479.2006
Komander, D., and Rape, M. (2012). The ubiquitin code. Annu. Rev. Biochem. 81, 203–229. doi: 10.1146/annurev-biochem-060310-170328
Kulathu, Y., and Komander, D. (2012). Atypical ubiquitylation - the unexplored world of polyubiquitin beyond Lys48 and Lys63 linkages. Nat. Rev. Mol. Cell Biol. 13, 508–523. doi: 10.1038/nrm3394
Lallemand-Breitenbach, V., Jeanne, M., Benhenda, S., Nasr, R., Lei, M., Peres, L., et al. (2008). Arsenic degrades PML or PML-RARalpha through a SUMO-triggered RNF4/ubiquitin-mediated pathway. Nat. Cell Biol. 10, 547–555. doi: 10.1038/ncb1717
Leidecker, O., Matic, I., Mahata, B., Pion, E., and Xirodimas, D. P. (2012). The ubiquitin E1 enzyme Ube1 mediates NEDD8 activation under diverse stress conditions. Cell Cycle 11, 1142–1150. doi: 10.4161/cc.11.6.19559
Leznicki, P., and Kulathu, Y. (2017). Mechanisms of regulation and diversification of deubiquitylating enzyme function. J. Cell Sci. 130, 1997–2006. doi: 10.1242/jcs.201855
Liu, M., Li, X. L., and Hassel, B. A. (2003). Proteasomes modulate conjugation to the ubiquitin-like protein, ISG15. J. Biol. Chem. 278, 1594–1602. doi: 10.1074/jbc.M208123200
Lu, B., Al-Ramahi, I., Valencia, A., Wang, Q., Berenshteyn, F., Yang, H., et al. (2013). Identification of NUB1 as a suppressor of mutant Huntingtin toxicity via enhanced protein clearance. Nat. Neurosci. 16:562. doi: 10.1038/nn.3367
Maghames, C. M., Lobato-Gil, S., Perrin, A., Trauchessec, H., Rodriguez, M. S., Urbach, S., et al. (2018). NEDDylation promotes nuclear protein aggregation and protects the Ubiquitin Proteasome System upon proteotoxic stress. Nat. Commun. 9:4376. doi: 10.1038/s41467-018-06365-0
Malakhova, O. A., and Zhang, D. E. (2008). ISG15 inhibits Nedd4 ubiquitin E3 activity and enhances the innate antiviral response. J. Biol. Chem. 283, 8783–8787. doi: 10.1074/jbc.C800030200
Matic, I., van Hagen, M., Schimmel, J., Macek, B., Ogg, S. C., Tatham, M. H., et al. (2008). In vivo Identification of human small ubiquitin-like modifier polymerization sites by high accuracy mass spectrometry and an in vitro to in vivo strategy. Moll Cell Proteomics 7, 132–144. doi: 10.1074/mcp.M700173-MCP200
Mattern, M., Sutherland, J., Kadimisetty, K., Barrio, R., and Rodriguez, M. S. (2019). Using ubiquitin binders to decipher the ubiquitin code. Trends Biochem. Sci. 44, 599–615. doi: 10.1016/j.tibs.2019.01.011
McIntosh, D. J., Walters, T. S., Arinze, I. J., and Davis, J. (2018). Arkadia (RING finger protein 111). Mediates sumoylation-dependent stabilization of Nrf2 through K48-linked ubiquitination. Cell. Physiol. Biochem. 46, 418–430. doi: 10.1159/000488475
McNaught, K. S. P., Olanow, C. W., Halliwell, B., Isacson, O., and Jenner, P. (2001). Failure of the ubiquitin–proteasome system in Parkinson's disease. Nat. Rev. Neurosci. 2, 589–594. doi: 10.1038/35086067
Mori, F., Nishie, M., Piao, Y.-S., Kito, K., Kamitani, T., Takahashi, H., et al. (2005). Accumulation of NEDD8 in neuronal and glial inclusions of neurodegenerative disorders. Neuropathol. Appl. Neurobiol. 31, 53–61. doi: 10.1111/j.1365-2990.2004.00603.x
Mulder, M. P. C., El Oualid, F., ter Beek, J., and Ovaa, H. (2014). A native chemical ligation handle that enables the synthesis of advanced activity-based probes: diubiquitin as a case study. Chembiochem 15, 946–949. doi: 10.1002/cbic.201402012
Mulder, M. P. C., Merkx, R., Witting, K. F., Hameed, D. S., El Atmioui, D., Lelieveld, L., et al. (2018). Total chemical synthesis of SUMO and SUMO-based probes for profiling the activity of SUMO-specific proteases. Angew. Chem. 57, 8958–8962. doi: 10.1002/anie.201803483
Nakasone, M. A., Livnat-Levanon, N., Glickman, M. H., Cohen, R. E., and Fushman, D. (2013). Mixed-linkage ubiquitin chains send mixed messages. Structure 21, 727–740. doi: 10.1016/j.str.2013.02.019
Nie, M., and Boddy, M. N. (2016). Cooperativity of the SUMO and ubiquitin pathways in genome stability. Biomolecules 6:14. doi: 10.3390/biom6010014
Ogunkoya, A. O., Pattabiraman, V. R., and Bode, J. W. (2012). Sequential alpha-ketoacid-hydroxylamine (KAHA). ligations: synthesis of C-terminal variants of the modifier protein UFM1. Angew. Chem. 51, 9693–9697. doi: 10.1002/anie.201204144
Ohtake, F., Saeki, Y., Sakamoto, K., Ohtake, K., Nishikawa, H., Tsuchiya, H., et al. (2015). Ubiquitin acetylation inhibits polyubiquitin chain elongation. EMBO Rep. 16, 192–201. doi: 10.15252/embr.201439152
Paudel, P., Zhang, Q., Leung, C., Greenberg, H. C., Guo, Y., Chern, Y.-H., et al. (2019). Crystal structure and activity-based labeling reveal the mechanisms for linkage-specific substrate recognition by deubiquitinase USP9X. Proc. Natl. Acad. Sci. U.S.A. 116, 7288–7297. doi: 10.1073/pnas.1815027116
Perng, Y. C., and Lenschow, D. J. (2018). ISG15 in antiviral immunity and beyond. Nat. Rev. Microbiol. 16, 423–439. doi: 10.1038/s41579-018-0020-5
Pichler, A., Fatouros, C., Lee, H., and Eisenhardt, N. (2017). SUMO conjugation - a mechanistic view. Biomol. Concepts 8, 13–36. doi: 10.1515/bmc-2016-0030
Poulsen, S. L., Hansen, R. K., Wagner, S. A., van Cuijk, L., van Belle, G. J., Streicher, W., et al. (2013). RNF111/Arkadia is a SUMO-targeted ubiquitin ligase that facilitates the DNA damage response. J. Cell Biol. 201, 797–807. doi: 10.1083/jcb.201212075
Ross, C. A., and Poirier, M. A. (2004). Protein aggregation and neurodegenerative disease. Nat. Med. 10, S10–S17. doi: 10.1038/nm1066
Saitoh, H., and Hinchey, J. (2000). Functional heterogeneity of small ubiquitin-related protein modifiers SUMO-1 versus SUMO-2/3. J. Biol. Chem. 275, 6252–6258. doi: 10.1074/jbc.275.9.6252
Santonico, E. (2019). “New insights into the mechanisms underlying NEDD8 structural and functional specificities,” in Ubiquitin Proteasome System Current Insights into Mechanism Cellular Regulation and Disease (IntechOpen). Available online at: https://www.intechopen.com/books/ubiquitin-proteasome-system-current-insights-into-mechanism-cellular-regulation-and-disease
Schimmel, J., Larsen, K. M., Matic, I., van Hagen, M., Cox, J., Mann, M., et al. (2008). The ubiquitin-proteasome system is a key component of the SUMO-2/3 cycle. Mol. Cell. Proteomics 7, 2107–2122. doi: 10.1074/mcp.M800025-MCP200
Schmidt, M. H. H., and Dikic, I. (2006). Ubiquitin and NEDD8: brothers in arms. Sci. STKE 2006:pe50. doi: 10.1126/stke.3622006pe50
Singh, R. K., Sundar, A., and Fushman, D. (2014). Nonenzymatic rubylation and ubiquitination of proteins for structural and functional studies. Angew. Chem. 53, 6120–6125. doi: 10.1002/anie.201402642
Singh, R. K., Zerath, S., Kleifeld, O., Scheffner, M., Glickman, M. H., and Fushman, D. (2012). Recognition and cleavage of related to ubiquitin 1 (Rub1). and Rub1-ubiquitin chains by components of the ubiquitin-proteasome system. Mol. Cell. Proteomics 11, 1595–1611. doi: 10.1074/mcp.M112.022467
Song, J., Durrin, L. K., Wilkinson, T. A., Krontiris, T. G., and Chen, Y. (2004). Identification of a SUMO-binding motif that recognizes SUMO-modified proteins. Proc. Natl. Acad. Sci. U.S.A. 101, 14373–14378. doi: 10.1073/pnas.0403498101
Spratt, D. E., Walden, H., and Shaw, G. S. (2014). RBR E3 ubiquitin ligases: new structures, new insights, new questions. Biochem. J. 458, 421–437. doi: 10.1042/BJ20140006
Sriramachandran, A. M., and Dohmen, R. J. (2014). SUMO-targeted ubiquitin ligases. Biochim. Biophys. Acta 1843, 75–85. doi: 10.1016/j.bbamcr.2013.08.022
Stolz, A., and Dikic, I. (2018). Heterotypic ubiquitin chains: seeing is believing. Trends Cell Biol. 28, 1–3. doi: 10.1016/j.tcb.2017.11.005
Sung, M. K., Porras-Yakushi, T. R., Reitsma, J. M., Huber, F. M., Sweredoski, M. J., Hoelz, A., et al. (2016a). A conserved quality-control pathway that mediates degradation of unassembled ribosomal proteins. eLife 5:e19105. doi: 10.7554/eLife.19105.026
Sung, M. K., Reitsma, J. M., Sweredoski, M. J., Hess, S., and Deshaies, R. J. (2016b). Ribosomal proteins produced in excess are degraded by the ubiquitin-proteasome system. Mol. Biol. Cell 27, 2642–2652. doi: 10.1091/mbc.e16-05-0290
Swatek, K. N., Usher, J. L., Kueck, A. F., Gladkova, C., Mevissen, T. E. T., Pruneda, J. N., et al. (2019). Insights into ubiquitin chain architecture using Ub-clipping. Nature 572, 533–537. doi: 10.1038/s41586-019-1482-y
Sweeney, P., Park, H., Baumann, M., Dunlop, J., Frydman, J., Kopito, R., et al. (2017). Protein misfolding in neurodegenerative diseases: implications and strategies. Transl. Neurodegener. 6:6. doi: 10.1186/s40035-017-0077-5
Tatham, M. H., Geoffroy, M. C., Shen, L., Plechanovova, A., Hattersley, N., Jaffray, E. G., et al. (2008). RNF4 is a poly-SUMO-specific E3 ubiquitin ligase required for arsenic-induced PML degradation. Nat. Cell Biol. 10, 538–546. doi: 10.1038/ncb1716
Valastyan, J. S., and Lindquist, S. (2014). Mechanisms of protein-folding diseases at a glance. Dis. Models Mech. 7, 9–14. doi: 10.1242/dmm.013474
Vivelo, C. A., Ayyappan, V., and Leung, A. K. L. (2019). Poly(ADP-ribose)-dependent ubiquitination and its clinical implications. Biochem. Pharmacol. 167, 3–12. doi: 10.1016/j.bcp.2019.05.006
Wagner, S. A., Beli, P., Weinert, B. T., Schölz, C., Kelstrup, C. D., Young, C., et al. (2012). Proteomic analyses reveal divergent ubiquitylation site patterns in murine tissues. Mol. Cell. Proteomics 11, 1578–1585. doi: 10.1074/mcp.M112.017905
Witting, K. F., van der Heden van Noort, G. J., Kofoed, C., Talavera Ormeno, C., El Atmioui, D., Mulder, M. P. C., et al. (2018). Generation of the UFM1 toolkit for profiling UFM1-specific proteases and ligases. Angew. Chem. 57, 14164–14168. doi: 10.1002/anie.201809232
Wood, L. M., Sankar, S., Reed, R. E., Haas, A. L., Liu, L. F., McKinnon, P., et al. (2011). A novel role for ATM in regulating proteasome-mediated protein degradation through suppression of the ISG15 conjugation pathway. PLoS ONE 6:e16422. doi: 10.1371/journal.pone.0016422
Wucherpfennig, T. G., Pattabiraman, V. R., Limberg, F. R., Ruiz-Rodriguez, J., and Bode, J. W. (2014). Traceless preparation of C-terminal alpha-ketoacids for chemical protein synthesis by alpha-ketoacid-hydroxylamine ligation: synthesis of SUMO2/3. Angew. Chem. 53, 12248–12252. doi: 10.1002/anie.201407014
Xin, B.-T., Gan, J., Fernandez, D. J., Knobeloch, K.-P., Geurink, P. P., and Ovaa, H. (2019). Total chemical synthesis of murine ISG15 and an activity-based probe with physiological binding properties. Org. Biomol. Chem. 17, 10148–10152. doi: 10.1039/C9OB02127B
Xirodimas, D. P., Sundqvist, A., Nakamura, A., Shen, L., Botting, C., and Hay, R. T. (2008). Ribosomal proteins are targets for the NEDD8 pathway. EMBO Rep. 9, 280–286. doi: 10.1038/embor.2008.10
Yau, R. G., Doerner, K., Castellanos, E. R., Haakonsen, D. L., Werner, A., Wang, N., et al. (2017). Assembly and function of heterotypic ubiquitin chains in cell-cycle and protein quality control. Cell 171, 918–933.e20. doi: 10.1016/j.cell.2017.09.040
Keywords: ubiquitin-like modifiers, hybrid chains, SUMO and ubiquitin signaling, NEDD8, ISG15, proteotoxic conditions, stress conditions, toolbox
Citation: Pérez Berrocal DA, Witting KF, Ovaa H and Mulder MPC (2020) Hybrid Chains: A Collaboration of Ubiquitin and Ubiquitin-Like Modifiers Introducing Cross-Functionality to the Ubiquitin Code. Front. Chem. 7:931. doi: 10.3389/fchem.2019.00931
Received: 22 October 2019; Accepted: 20 December 2019;
Published: 22 January 2020.
Edited by:
Laszlo Otvos, Olpe LLC, United StatesReviewed by:
Gary Kleiger, University of Nevada, Las Vegas, United StatesCopyright © 2020 Pérez Berrocal, Witting, Ovaa and Mulder. This is an open-access article distributed under the terms of the Creative Commons Attribution License (CC BY). The use, distribution or reproduction in other forums is permitted, provided the original author(s) and the copyright owner(s) are credited and that the original publication in this journal is cited, in accordance with accepted academic practice. No use, distribution or reproduction is permitted which does not comply with these terms.
*Correspondence: Monique P. C. Mulder, bS5wLmMubXVsZGVyQGx1bWMubmw=
Disclaimer: All claims expressed in this article are solely those of the authors and do not necessarily represent those of their affiliated organizations, or those of the publisher, the editors and the reviewers. Any product that may be evaluated in this article or claim that may be made by its manufacturer is not guaranteed or endorsed by the publisher.
Research integrity at Frontiers
Learn more about the work of our research integrity team to safeguard the quality of each article we publish.