- Department of Chemistry, Indian Institute of Technology Gandhinagar, Gandhinagar, India
Difluoroboron-dipyrromethenes (BODIPYs) are highly popular fluorescent dyes with applications as NIR probes for bioimaging, fluorescent tags/sensors and as photosensitizers in cancer therapy and organic photovoltaics. This review concentrates on the synthesis and spectral properties of BODIPY dyes conjugated with carbazole heterocycle. The carbazole is an electron rich tricyclic compound and due to its excellent electronic properties, it is extensively used in the production of electroluminescent materials and polymers. This review highlights the recent progress made on the series of BODIPY derivatives containing carbazole ring at alpha, beta, and meso-positions of the BODIPY skeleton. Carbazole based hybrid BODIPYs, carbazole linked aza-BODIPYs and carbazole-fused BODIPYs are also discussed.
Introduction
Certain organic or inorganic molecules can act as fluorophores; and they can re-emit the light upon irradiation with the light source. The fluorescent organic dyes have been extensively used in the wide range of applications such as: biomolecular labels (Celli et al., 2010; Kowada and Kikuchi, 2015), chemosensors (Wu et al., 2015), energy transfer cassettes (Fan et al., 2013), organic light emitting diodes (Zampetti et al., 2017), dye-sensitized solar cells (Klfout et al., 2017), etc. Among the highly fluorescent organic molecules reported in the literature, the dyes based on 4,4-difluoro-4-bora-3α,4α-diaza-s-indacene (difluoroboron dipyrromethene, abbreviated as BODIPY, Figure 1); show possibly the highest potential and have become enormously popular in recent times. Although, Treibs and Kreuzer first reported these molecules in 1968 (Treibs and Kreuzer, 1968); the field was not developed much till 1980. In the 1980s, researchers reported potential use of these dyes for biological labeling (Vedamalai et al., 2018). After that, there was a clear rise in the number of reports on BODIPY dyes; making them hugely popular among chemists and biologists to develop BODIPY based fluorescent sensors (Vedamalai et al., 2016, 2018), bioimaging agents (Kesavan et al., 2019), and photosensitizers for PDT (Kamkaew et al., 2013; Zheng et al., 2018).
By mid of the 1990s BODIPY's potential applications in the area of biological sciences (Vedamalai and Gupta, 2018) and materials sciences were fully recognized and research reports in this area tremendously increased (Ulrich et al., 2008). These molecules showed remarkable properties like sharp absorption and emission, large molar absorption coefficients, high fluorescence quantum yields and high photo-stability. Thus, this group of fluorescent dyes meet the criteria for a good fluorophore; they exhibit enormous synthetic variations and versatile applications (Loudet and Burgess, 2007; Ziessel et al., 2007; Kolemen and Akkaya, 2018). Understanding the photophysical properties of these systems (Lu et al., 2014) is of principal importance, not only because of the intrinsic potential applications but, also in the design of new dyes with specific properties. The main synthetic advantage of BODIPY dye is that, the unique structure of dye skeleton provides eight positions which can be easily functionalized to fine-tune their electronic properties (Lakshmi et al., 2015, 2016). There are excellent reviews available on the BODIPYs based on the different applications, such as: fluorescent NIR probes (Yuan et al., 2013), sensitizers for PDT (Kamkaew et al., 2013), organic materials for photovoltaics (Bessette and Hanan, 2014), D-A type systems with focus on energy/electron transfer (Loudet and Burgess, 2007), fluorescent sensors (Boens et al., 2012; Ni and Wu, 2014), BODIPY based multi-chromophore cassettes (Ziessel et al., 2007), etc. This review presents the structural diversity of the carbazole-BODIPY conjugates, with the emphasis on the effect of the substitution of carbazole heterocycle on the optical properties of the BODIPYs.
Carbazole is a well-known heterocyclic aromatic system. The aromatic nature of carbazole makes it chemically and thermally stable; and the ring can be easily functionalized at different positions. Carbazole and its derivatives are electron rich compounds and they exhibit good absorption and emission properties (Li et al., 2004; Barberis and Mikroyannidis, 2006; Mudadu et al., 2008). Also, due to their excellent photoluminescence and hole-transport property; these systems are used for various applications in photovoltaic systems and OLEDs. They are also employed as photosensitizers (Promarak et al., 2008; Wang et al., 2008; Tang et al., 2010) in DSSCs. Carbazole derivatives are also known for their anti-microbial, anti-tumor properties and as bioimaging agents (Głuszyńska, 2015).
In recent years, the reports on carbazole substituted BODIPYs and porphyrinoids (Das and Gupta, 2019) have significantly increased. It is observed that, the presence of electron rich carbazole moiety can alter the absorption and emission properties of BODIPYs; which depend on the position and kind of linkage through which carbazole is attached on the BODIPY skeleton. In this review, we present the overview of synthetic strategies used to prepared various kinds of carbazole substituted BODIPYs; also, the change in the electronic properties due to substitution and their applications are discussed.
Beta-Substituted BODIPYs
Main advantage of BODPY core is that, the three available positions (α, β, and meso) are prone to derivetisation (Figure 1). But the feasibility of substitution is highly depended on the other functional groups, already present on the BODIPY core. The substitution of electron rich groups at the beta-positions is expected to enhancethe electronic communication between the BODIPY core and the substituents.
In 2009, Zhang et al. reported the synthesis of BODIPY 3 (Scheme 1); the key precursor 2 was coupled with 9-ethyl-3-(prop-1-ynyl)-9H-carbazole via Pd-catalyzed Sonogashira reaction. The ethynyl linkages present in this molecule helped to show efficient ICT process. The absorption and emission maxima of BODIPY 3 were very much red shifted (Scheme 1) as compared to the parent meso-tetraphenyl BODIPY, which reflected the effect of carbazole ring linked via rigid ethyne linker to the boron-dipyrrin core. The linear D-π-D type structure resulted in the extended conjugation along with efficient ICT process, which made this molecule to exhibit two-photon absorption properties (Zhang et al., 2009). As a result, this compound showed a sharp emission peak around 670 nm with reasonable quantum yield. This emission was attributed to two-photon emission fluorescence (TPEF). Since this emission wavelength fall in human body's therapeutic window (650–800 nm), this molecule has potential in bioimaging applications.
The BODIPYs 6 and 7 linked to carbazole via thiophene spacer were used for organic photovoltaic (OPV) applications (Lin et al., 2012). The target BODIPYs 6 and 7 were synthesized by Stille and/or Sonogashira coupling reactions between precursors BODIPY 4 and 5 with the appropriate stannyl functionalized carbazole derivative (Scheme 2). The studies showed that, insertion of an alkyne moiety renders flexibility between the donor carbazole and acceptor BODIPY core, which is beneficial for light harvesting. The large Stokes shift displayed by BODIPYs 6 and 7 suggests that, the excited state of the molecules have a more planar conformation, which is not favorable for solar cell applications. Both the molecules 6 and 7 showed reasonable light to current conversion efficiency of 1.8 and 2.6%, respectively. Zhao and co-workers reported BODIPYs 9 and 10 where, one beta-position is substituted with carbazole ring and the other beta-position is linked to C60 (Scheme 3) (Yang et al., 2012) or rhenium metal complex (Chart 1). In BODIPYs 9 and 10, the electron rich carbazole derivative is attached through rigid ethynyl bond by Pd(0) catalyzed Sonogashira coupling. The carbazole derivative linked to the BODIPY unit, is acting as a light-harvesting antenna system. In compound 10, intramolecular energy transfer was observed from the BODIPY based singlet excited state to the singlet excited state localized on the C60 unit.
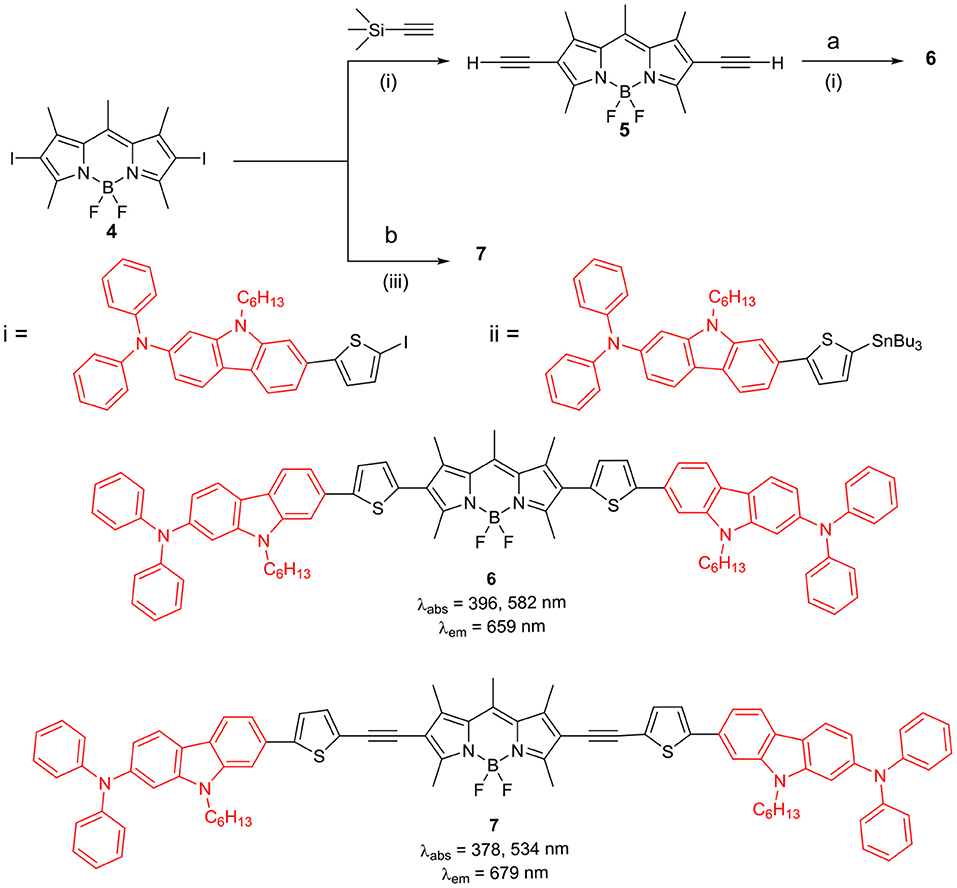
Scheme 2. BODIPYs linked to carbazole derivatives with thiophene spacer. (i) Pd(PPh3)2Cl2, CuI, THF, Et3N, TBAF, THF; (ii) Pd(PPh3)2Cl2, CuI, THF, diisopropylamine; (iii) Pd(PPh3)2Cl2, PPh3, DMF.
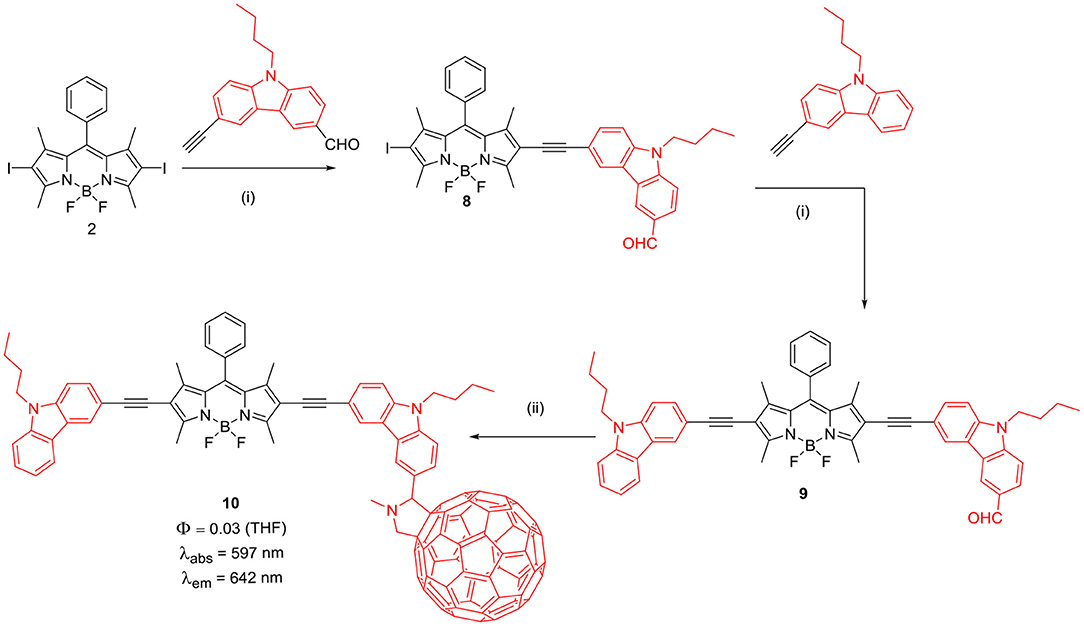
Scheme 3. Synthesis of BODIPY linked via ethyne linkage to carbazole and C60. (i) PdCl2(PPh3)2, PPh3, CuI, NEt3, THF, argon atmosphere, 60°C, 6 h; (ii) sarcosine, C60, toluene, reflux.
The intrinsic intersystem crossing resulted in the triplet-excited state of the C60 in the absence of a heavy atom. The dyad 10 showed TTA up-conversion with quantum yield up to 2.9% (Yang et al., 2012). Complex 11 showed much weaker TTA up-conversion, which can be assigned to the weak absorption of 11 at the excitation wavelength and less efficient ISC (Yi et al., 2013).
Direct substitution at the beta-positions of BODIPYs (Scheme 4) through Suzuki coupling between boronic ester derivative of carbazole and the 2,6-dibromo substituted BODIPY 12 is another method to incorporate carbazole ring on the BODIPY core. The pronounced effect of substitution of carbazole rings on the beta-position of the BODIPY, reflected in the increased absorption efficiency from 300 to 900 nm; thus molecule 13 acted as a panchromatic dye. This molecule showed an excellent red-shift in its emission and had high thermal stability. Photovoltaic performance studies showed that, by further engineering the molecular structure and optimization of the morphology; this type of dyes can become potential candidates for the efficient organic solar cell materials (Liao et al., 2016). Wanwong et al. reported the application of beta-carbazole substituted BODIPY dyad and triad (Scheme 5; 15 and 16) as field effect transistors (FET). Though these derivatives provided moderate performance, modification of this structure may help to develop better dye with better performance (Wanwong et al., 2018).
Electron rich carbazole ring acts as very good electron donor and its derivatives exhibit photorefractive and hole-trasnport properties; thus they are polupar constituents of electroluminescent materials. Mao et al. reported application of beta-substituted BODIPYs, having D-A-π-A system (Scheme 6) for DSSC applications. Incorporation of the extra acceptor in between the donor moiety and π-conjugating unit decreases the HOMO-LUMO energy gap, and as a result, these BODIPYs can show an efficient photoinduced electron transfer from the donor to the BODIPY acceptor unit; which is linked to the anchor group at the opposite end (Mao et al., 2017).
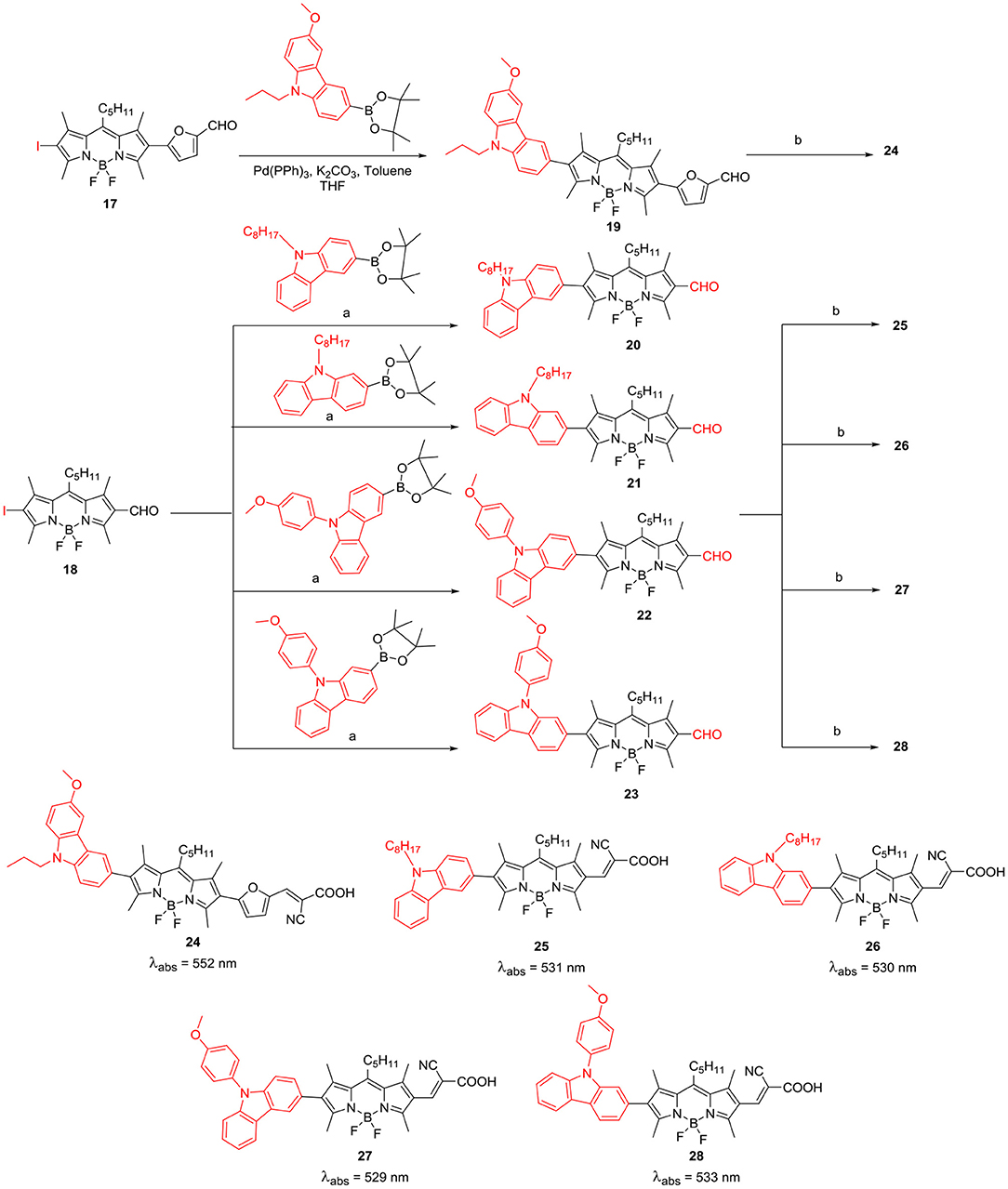
Scheme 6. beta-carbazole substituted BODIPYs for DSSC application; a: Pd(PPh3)4, K2CO3, toluene/THF; b: piperidine, CNCH2COOH, DCM/ACN.
BODIPY 24 with cyanoacetic acid anchoring group was used as a photosensitizer for DSSC; this linear system showed reasonable PCE efficiency of 3.1%. A series of beta-substituted BODIPYs 25–28 having D-π-A system were constructed for DSSC application (Scheme 6). The N-alkyl carbazole ring served as donor and BODIPY core linked to cyanoacetic acid was the acceptor unit (Liao et al., 2017b). The DSSC analysis revealed that photosensitizers 26 and 28, having 2-carbazolyl substituent at the beta-position showed better Jsc than BODIPYs 25 and 27 where carbazole is substituted through 3-position and it was reflected in their overall efficiency (Liao et al., 2017b). BODIPY derivatives having heavy atoms such as: bromo, iodo groups on the dipyrrin core, have been used as triplet sensitizers. Such metal-free triplet sensitizers can be effectively used for singlet-oxygen generation, PDT agents and triplet-triplet annihilation upconversion (TTA-UC). Wei et al. (2017) have prepared BODIPY based organic photosensitizers connected to C60 and N-butylcarbazole at the β-positions 29 and 30 (Chart 2). As per the report, C60 has high ISC (inter system crossing) efficiency but weak absoprtion in the visible region of the solar spectrum. Thus, linking of C60 to the carbazole substiuted BODIPY can be useful to effectually populate the triplet excited state of the C60, which in turn can transfer the energy to perylene acceptor. The calculated TTA-UC quantum yield was 4.9 for the carbazole-BODIPY—C60 triad 30 shown in Chart 2 (Wei et al., 2017).
Alpha-Substituted BODIPYs
There are two alpha positions on the BODIPY skeleton which are available for derivatization after various reactions such as: nucleophilic or electrophilic substitution, cross-coupling reactions, and Knoevenagel condensation (Wood and Thompson, 2007), etc.
Ooyama et al. recently reported a new strategy to develop effective BODIPY based sensitisers possessing a good light harvesting efficiency (LHE) in the range of visible light to NIR region. They developed (D)2-π-A type BODIPYs 33 and 34 (Scheme 7) having pyridyl and cyanoacrylic acid groups, respectively. The electron-withdrawing anchoring group (pyridyl/cyanoacetic acid) helps to bind the BODIPY on the titanium dioxide layer for photovoltaic application. The presence of strong electron-donating units of 9-butyl-N,N-phenyl-7-(thiophen-2-yl)-9H-carbazol-2-amine at alpha-positions of the BODIPY core, helped to obtain strong and broad absorption band ranging from 600 to 850 nm. Also, these molecules showed high LHE in the range of visible light to NIR region. Though these molecules showed good photophysical properties; these BODIPY dyes showed low photovoltaic performance in DSSC studies, which was attributed to the low lying LUMO levels (Ooyama et al., 2017).
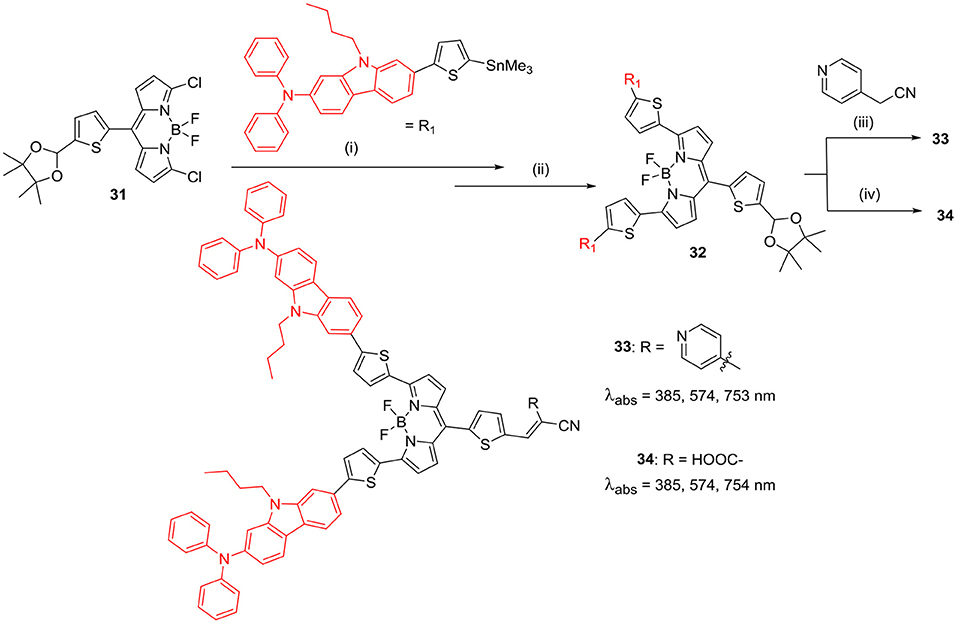
Scheme 7. Synthesis of 3,5-carbazole substituted BODIPY for DSSC. (i) Pd(PPh3)4, toluene; (ii) 2M HCl, THF; (iii) NEt3, CH2Cl2; (iv) Cyanoacetic acid, piperidine, CHCl3/CH3CN
Knoevenagel condensation is another method to introduce carbazole unit on the BODIPY core. Zhang et al. reported mono-, di- and tetra-styryl carbazole substituted BODIPYs (35, 38, and 39). The Knoevenagel condensation between methyl-2-(2-formyl-9H-crbazole-9-yl)acetate with BODIPY 35 (Scheme 8) produced target styryl BODIPYs 36, 37, and 38 in good yields. The extended π-conjugation converted the simple BODIPYs to NIR dyes with strong absorption maxima in between the 600 and 727 nm range. Their corresponding emission maxima were also considerably red shifted; which reflected the increased conjugation between the carbazole units and the BODIPY core. The BODIPY 39 with four styryl-carbazole unit showed the highest PCE of 2.7% (Zhang et al., 2015).
The synthesis of di-styryl BODIPY based D-A and D-π-A systems (40–44) was reported, where thiophene served as π-linker between the N-alkylcarbazole unit and the BODIPY core (Chart 3) (Brzeczek et al., 2016; Kurowska et al., 2018). The target BODIPYs were synthesized through multistep synthetic procedure; firstly the precursor 1,3,5,7-tetramethyl-8-mesityl BODIPY was prepared by the conventional synthetic protocol. Microwave assisted Knoevenagel condensation of the precursor BODIPY with appropriate carbazole derivative afforded target BODIPYs 40–44 (Chart 3). The carbazole substituion at alpha-positions of the BODIPY core has prominent effect on the electronic properties of the dyes; the target compounds 40–44 showed markdly red shifted absorption (736–740 nm) and emission maxima (775–780 nm). Particularly, the BODIPY–carbazole conjugates with single thiophene linker showed highly red-shifted absorption and emission spectra. On increasing the number of thiophene linkers, the effect of carbazole donor on the BODIPY acceptor was diminished; the quenched emission was attributed to the stronger push-pull effect for systems with elongated conjugation framework.
Followed by this work, Cheema et al. studied the application of 3,5-di-styryl BODIPYs having N-alkyl carbazole units (45–46) for DSSC application (Chart 3). Carboxylic acid group on the meso-phenyl ring of the BODIPY acted as anchor group (Cheema et al., 2016). It was revealed that, the alkyl substitution did not change the position of absorption and emission maxima; but the intensity of these bands was altered. With the increases in the length of N-alkyl chain, the intensity of the lower energy absorption band decreased. DSSC performance for the dyes was much less than expected, which was attributed to the aggregation related losses (Cheema et al., 2016).
Alpha-styryl substituted BODIPY derivatives have excellent photophysical properties; they exhibit strong absorption and fluorescence in near infra-red (NIR) region. Such α-styryl substituted BODIPY derivatives have tremendous potential as bioimaging agents; particularly cell organelle targeting becomes facile as their absorption and emission falls in the biological window. The α-styryl BODIPYs 48–50 (Scheme 9) having one or two N-ethynyl-carbazole groups were prepared by Zhang et al. (2013). Extended conjugation of the BODIPY core with the carbazole ring resulted in strong absorption with high extinction coefficients between 620 and 703 nm and red emission in the range of 650–730 nm. These BODIPYs showed high fluorescence quantum yields and decent two-photon absorption properties; also, the NIR probe 50 demonstrated significant localization in the mitochondria of MCF-7 cells, due to the presence of triphenylphosphonium group (Zhang et al., 2013).
An interesting system of subphthalocyanine-BODIPY scaffold containing one or two N-ethyl-carbaole moieties was reported by Eçik et al. (2017). Synthesis of these molecules followed multistep synthetic procedure, Knoevenagel condensation of the precursor BODIPY (51) with 9-ethyl-9H-carbazole-2-carbadehyde (Scheme 10) afforded 52 and 53. Click reaction of ethyne functionalized subphthalocyanine with BODIPYs 52 and 53, resulted in the formation of the desired target molecules 54 and 55 (Scheme 10). These systems (54 and 55) showed efficient energy transfer from subphthalocyanine unit to the BODIPY unit via fluorescence resonance energy transfer (FRET). Authors suggested that, these kinds of systems can be developed into a BODIPY-based multi-chromophore systems and this will help to reveal their energy transfer potential in efficient light-harvesting systems (Eçik et al., 2017).
Another method to introduce carbazole ring on the BODIPY core is through N-linkage (Scheme 11; 57 and 58). Zhang et al. showed that, 4-(9H-carbazol-9-yl)benzaldehyde can be linked to BODIPY 56 via Knoevenagel condensation, followed by the deprotection of 57 to produce 58. The presence of N-phenyl carbazolyl groups on the BODPY 58 can help to reduce the aggregation of dye to some extent; hence 58 exhibited enhanced photon to electron conversion efficiency of 4.4%. Since the electron rich carbazole ring has excellent hole-transport properties; it is widely used in optoelectronics; most of the scientists are interested in substituting carbazole to BODIPY core to enhance their photovoltaic applications (Zhang et al., 2016). A new synthetic approach for the modification of alpha-positions of the BODIPY with carbazole was reported by Satoh et al. (2018). As shown in the Scheme 12, to obtain 60, alpha-positions of the BODIPY core can be substituted by nucleophilic aromatic reaction (SNAr) of carbazole with compound 59 in THF (Satoh et al., 2018). Absorption and emission spectra exhibited bathochromic shifts as compared to the parent BODIPY; these molecule promises development of new BODIPY based fluorophores through this synthetic methodology (Satoh et al., 2018).
Alpha-styryl BODIPY having one N-alkylcarbazole substituent was prepared by Mani et al. (2017); Knoevenagel condensation of the precursor compound 61 with 7-bromo-9-butyl-9H-carbazole-2-carbaldehyde (Scheme 13) afforded 62. The BODIPY 62 exhibited huge red shifts of 92 nm in the absorption and 118 nm in the emission w.r.t. the starting BODIPY 61 (Mani et al., 2017). Chang et al. reported donor-acceptor systems of alpha-styryl BODIPYs 63 and 64 (Chart 4) having N-alkylcarbazole and/or cyanuric chloride as linker group (Su et al., 2014b). BODIPYs 63 and 64 exhibited red shifted absorption and fluorescence around 617 nm and considerable pseudo Stokes shift (~120 nm) due to intramolecular DRET (dark resonance energy transfer) in such systems (Su et al., 2014a). Significant pseudo Stokes shifts, red shifted fluorescence, and biocompatibility of cyanuric chloride linker group in 64 suggest its potential application in bioimaging of live cells (Su et al., 2014a).
Han et al. reported the alpha-styryl BODIPY 65 (Chart 5) having two carbazole rings with N-PEGylated chains for the deep tissue imaging and PDT application (Huang et al., 2016). The BODIPY 65 showed NIR absorption at 661 and emission at 755 nm with fluorescence quantum yield of 4%. The calculated singlet oxygen quantum efficiency 67% for compound 65 was considerably high and its water soluble nanoparticles were prepared by mixing it with the biodegradable polymer PLA-PEG-FA (comprising of poly-lactic acid, poly-ethyleneglycol, and folate). The biocompatible nanoparticles showed broad absorption and emission in the NIR region (650–800 nm) with 58% singlet oxygen quantum yield upon excitation by NIR light ~670–800 nm; also they can be used for deep tissue imaging and for the treatment of tumors. The organic nanoparticles displayed green emission upon light irradiation in HeLA cells ~670–800 nm and negligible cytotoxicity; making them suitable candidates for PDT studies (Huang et al., 2016). The alpha-distyryl BODIPYs 66–68 (Chart 6) having two carbazolylethynyl groups and two beta-bromo groups was used as photosensitizers for PDT (Zhou et al., 2018). Compound 67 exhibited strong absorption bands around 513 and 708 nm corresponding to the carbazolyl group and boron-dipyrrin core. For the BODIPY 67 containing glibenclamide analogous moiety, the fluorescene band appeared at 753 with 45 nm Stokes shift; also it demonstrated effective localization in endoplasmic reticulum (ER) of HeLa (human cervical cancer) and HepG2 (human hepatocarcinoma) cells. The compound 67 was able to generate singlet oxygen upon excitation at 610 nm; ER stress was the main reason of cell death as per the report (Zhou et al., 2018).
Meso-Substituted BODIPYs
Apart from beta- and alpha- positions of the BODIPY skeleton, the meso-position (C-8) can also be substituted with carbazole to prepare variety of dyes with improved absorption and emission properties. Carbazole-based D–π-A type BODIPYs 69–71 were synthesized and studied for DSSC application (Chart 7) (Ooyama et al., 2013a). In BODIPY 69, two 4-(thiophene-2-yl)pyridine units on the alpha-positions served as electron-withdrawing anchor groups and the meso-position was substituted with an electron donating 9-butyl-N,N-phenyl-7-(thiophen-2-yl)-9H-carbazol-2-amine group. The introduction of thiophene linkers between the donor and BODIPY core, extended the π conjugation of the entire system; which was indicated by the noticeable bathochromic shifts in absorption and emission of 69 (673, 696 nm, respectively). In BODIPYs 70 and 71 (Chart 7), the meso-substituent: 9-butyl-N,N-phenyl-7-(thiophen-2-yl)-9H-carbazol-2-amine acted as donor unit, which was linked to BODIPY core with the phenyl linker. The presence of small methyl/ethyl groups on the alpha-position of the BODIPY core in 70 and 71 does not cause much shifts in their absorption and emission maxima (Ooyama et al., 2013b).
The BODIPY 69 showed significant red shift in its absorption and emission; however, it showed moderate performance in DSSC studies. Authors attributed the low performance mainly to the following reasons: (1) Formation of strong π-stacked aggregates of BODIPY on the TiO2 surface; (2) The lower LUMO level and the radiation less relaxation of the photoexcited dye which leads to a reduction in the electron-injection yield; (3) faster charge recombination between the injected electrons and ions, leading to a decrease in the Voc value (Ooyama et al., 2013a). Aggregation between BODIPY cores can be prevented by using co-adsorption of chenodeoxycholic acid (CDCA). One of the main reasons for radiation less relaxation is the free rotation of the aryl substituents at alpha- and meso-positions of the BODIPY skeleton. This rotation can be reduced by methyl substitution on BODIPY core (Ooyama et al., 2013b). Also, dyes 70 and 71 (Chart 7) showed solid-state red fluorescence and green metallic luster properties in both crystalline and amorphous states (Ooyama et al., 2014).
Substitution of carbazole unit on the meso-position of the BODIPY core through direct linkage is another method to incorporate carbazole unit on BODIPY skeleton. Gupta et al. reported synthesis and photophysical properties of meso-substituted carbazole-BODIPY dyad 72 (Scheme 14). This dyad exhibited energy transfer efficiency from donor carbazole unit to the acceptor BODIPY core. As shown in the Scheme 14, dyad was synthesized from the dipyrromethane having meso-carbazolyl unit, followed by complexation with BF3.OEt2 to obtain the desired product. BODIPY 72 was further used to make BODIPY derivatives 73–75 (Scheme 14). It was found that the meso-carbazoyl group altered the electronic properties of the four BODIPYs which was reflected in the higher extinction coefficient, red-shifted emission maxima, increased quantum yields and large Stokes shifts. Fluorescence studies indicated an efficient energy transfer from meso-carbazolyl moiety to the boron-dipyrrin core in all the compounds. Due to increased conjugation with the electron donor meso-carbazole group, anodic shifts were observed in the redox potentials of all four BODIPYs 72–75 (Kesavan and Gupta, 2014). The synthesis and photovoltaic application of meso-carbazolyl substituted BODIPY based photosensitizers 77 and 80 (Scheme 15) was reported. The photosensitizers were synthesized in four steps from the precursor BODIPYs 72 and 78 as shown in the Scheme 15. The BODIPY 80 exhibited higher photovoltaic performance than the photosensitizer 77 in DSSC studies (Kesavan et al., 2017).
Sekar et al. reported BODIPYs 81–83 having 9-ethyl-9H-carbazole or 9-phenyl-9H-carbazole group at the meso-position; the alpha- and beta-positions were substituted with the alkyl groups (Chart 8). The direct substitution of carbazole ring on the BODIPY skeleton resulted in the enhanced photostability, good lasing ability and singlet oxygen generation property of the dyes (Thorat et al., 2015; Telore et al., 2016). Misra et al. (2014) reported meso-ethynyl linked carbazole-BODIPYs 84–86 (Chart 9); efficient intramolecular charge transfer from carbazole unit to the BODIPY unit was observed (Dhokale et al., 2015). Compounds 84–86 showed high open circuit voltage and thus exhibited good application in bulk heterojunction organic solar cells (Jadhav et al., 2015).
Farfan and Correon-Castro groups (Corona-Sánchez et al., 2016) reported DFT studies of the thin films of meso-substituted BODIPY 87 having N-ethyl-carabzole ring (Chart 10). The thin films of BODIPY 87 were prepared by the vapor deposition on indium tin oxide; packing morphology of the films was simulated through computational methods and their semi-conductor behavior was predicted. Such kind of DFT study can be helpful when such BODIPY dyes are used in the electronic devices for OPV applications (Corona-Sánchez et al., 2016). Another interesting report by Xing et al., used BODIPYs 88 and 89 to make liposomes encapsulated fluorescent nanoparticles (Lv et al., 2017). The nanoparticles of 88 and 89 showed decent absorption in HEPES buffer at 504 nm; also their emission was centered on 525 nm with about 21 nm Stokes shift. These hydrophilic fluorescent nanoparticles were also tested for live cell imaging on HeLa cells, and the results indicated primary localization in the lysosomes (Lv et al., 2017).
Carbazole ring can also be linked to the meso-position of BODIPY core via N-linkage (Chart 11); Nguyen et al. (2014) revealed the OLED application of BODIPY 90, having 9-phenyl-9H-carbazole group at the meso-position. BODIPY 90 exhibited green emission with low turn-on voltage in OLED performance, maximum brightness, current efficiency and power efficiency. Report by Li et al. revealed the AIEE ability of BODIPY 91 (Chart 11), which showed enhanced emission in the aggregated form. BODIPY 91 showed weak emission in THF; and the nano aggregates of 91 were prepared in THF water mixture by precipitation method. The noticeable increment in fluorescence intensity of 91 was observed with the gradual increase in the water fraction. In 90% water/THF mixture molecule 91 showed the strongest emission intensity. Similar aggregation study was carried out by preparing carbazole-BODIPY 91 loaded silica nanoparticles, and these nanoparticles demonstrated a stable uniform morphology and strong fluorescence. This AIEE effect was successfully applied for cell imaging and found that BODIPY 91 showed good cellular uptake in MCF-7 cells (Li and Qian, 2016). The recent report by Reddy et al. showed that meso-substitution of BODIPY with carbazole ring can be achieved through N-linkage; such BODIPYs 92–94 (Chart 12) exhibited photoinduced energy transfer (PEnT). They studied the effect of linker length on PEnT using varying lengths of bridges (phenyl, biphenyl and diphenylethyne) on the BODIPYs 92–94 (Chart 12). Selective excitation of these molecules at carbazole unit resulted in a very efficient energy transfer process (Reddy et al., 2018).
Recently, Thayumanavan et al. prepared donor-acceptor type BODIPYs 95–97 (Chart 13) and studied photo-induced electron transfer process for these dyes (Strahan et al., 2019). The electron rich carbazole donor group was attached at either beta- or meso-position of the BODIPYs to access intramolecular charge transfer (ICT) in the dyes 95–97 (Chart 13). The ICT was more facile in the BODIPY 95 as compared to the 96 and 97 in polar solvents; also the carbazole substation at beta-position of the BODIPY skeleton shifted the absorption of 95 toward red region with higher molar extinction coefficient than the meso- substituted BODIPYs 96 and 97 (Strahan et al., 2019). Qian's group had reported meso-N-ethylcarbazole substituted BODIPYs 98–100 (Scheme 16); the molecule 101 containing nitro-substituted benzoxadiazole (NBD) moieties, was used as fluorescent probe for biothiol detection and live cell imaging (Xia and Qian, 2018). The probe 100 also demonstrated visible color change from blue to green upon addition of biothiols in the solution; thus it can be used to develop sensor-kit for biothiols in future. In addition, 100 was successfully applied to detect Cys, Hcy, and GSH in living cells (Xia and Qian, 2018).
Carbazole Bridged BODIPY Dimers
Zong et al. reported carbazole bridged BODIPY dimers 101–103 with extended π conjugation (Chart 14) by introducing linker moieties in between carbazole and BODIPY units. The linkers varied from phenyl, thiophene to furan rings; all these conjugates possess good thermal stability. From the photophysical and electrochemical analysis, it was revealed that thiophene and furan linked carbazole-BODIPY dimers (101, 102) are potential candidates for p-type semiconductor materials in organic solar cells (Zong et al., 2017). Report by Liao et al. showed that presence of alkynyl group as bridging unit (Chart 13; 104) shifted the absorption and emission toward red region. BODIPY 104 exhibited average photovoltaic performance of 3.1% by a hole mobility mechanism (Liao et al., 2017a). Also, compound 105 (Chart 14) showed decent cytotoxic activity against HT29 cell lines (Sengul et al., 2015). In another report Gupta et al. synthesized N-butylcarbazole bridged BODIPY dimer 106 (Chart 14); the compound showed bathochromic shift in the emission band with good Stokes shift of 83 nm (Kesavan et al., 2015).
Meso-carbazole substituted BODIPY 107 and N-alkyl-carbazole bridged BODIPY dimer 108 (Chart 15) were prepared and their biological activities were tested in the human colon cancer cell lines (Sengul et al., 2015). The BODIPYs 107 and 108 exhibited strong absorption around 503 nm and fluorescence at 512 nm; cytotoxicity assays in HT29 cancer cells revealed that, 108 is more toxic than 107 (Chart 15). The observed IC50 values for 107 and 108 were 21.7 ng/mL and 8.3 ng/mL, respectively (Sengul et al., 2015).
BODIPY based nanocar containing p-carborane wheels and central N-butylcarbazole moiety was prepared by Godoy et al. (2010). The BODIPY nanocar 109 (Chart 16), was highly emissive in nature making it ideal candidate for single molecule fluorescence spectroscopy. Such nanocars are reported to move by an average speed of 4 nm/s on the glass surface under ambient conditions due to the presence of p-carborane wheels (Godoy et al., 2010).
Miscellanious Systems
In 2013, Ma et al. reported NIR emissive D–π-A polymers 112, where aza-BODIPY moiety acted as acceptor and electron rich carbazole group served as donor. The synthesis of beta-diiodinated derivative of aza-BODIPY 111 was prepared by treating 110 with N-iodosuccinimide (Scheme 17). The polymerization of BODIPY 111 was accomplished by a palladium-catalyzed Sonogashira coupling reaction with 3,6-diethynyl 9-octyl-9H-carbazole moiety (Scheme 17). The good advantage of such polymer systems is that, these molecules exhibit near-infrared fluorescence around 750 nm and also show tunable band gap in the range of 0.96–1.14 eV. These photophysical and electrochemical properties promises the application of this polymer 112 in device based applications (Ma et al., 2013).
Another interesting report by Patra et al. described the synthesis of porous organic polymer 113 (Chart 17) consisting of N-alkyl bridged carbazole and BODIPY units (Bandyopadhyay et al., 2018); the macromolecule was able to generate substantial singlet oxygen in solution. The porous soluble polymer 113 exhibited red shifted absorption and emission in the range of 530–610 nm; and used as fluorescent probe for superoxide anion (Bandyopadhyay et al., 2018). The π-conjugated polymers having BODIPY backbone are used as photosensitizers in organic photovoltaics (OPV); and strong absorption in the red or NIR region is desired for high performance of the devices. Combination of BODIPY unit with good electron rich donor moiety in the polymer chain can yield the copolymer with strong absorption profile in the visible-NIR region of the solar spectrum. The optical band gap can be reduced in copolymers by linking donor and acceptor units; Thayumanavan et al. have synthesized π-conjugated BODIPY copolymers 114 (Chart 18) having N-alkylcarbazole/dithienopyrrole/bithiophene/fluorene as donor moieties (Popere et al., 2012). The rationally designed copolymers exhibited lower band gap and broad absorption spectra encompassing the entire visible region; thus, making them good panchromatic dyes for OPV applications (Popere et al., 2012). Such copolymers containing π-conjugated donor-acceptor units also show interesting charge transfer and/or energy transfer properties with enhanced absorption and fluorescence in the visible to red region.
Another π-conjugated copolymer 116 incorporating indolo[3,2,-b]-carbazole and BODIPY units (Chart 18) was prepared from 115, by Khetubol et al. (2015). Copolymer 116 showed broad and red shifted absorption and emission spectra along with the energy transfer from donor indolo[3,2,-b]-carbazole to the acceptor BODIPY unit. The electronic properties of organic π-conjugated polymers can be fine-tuned by introducing electron donor and accepter moieties in the main chain; the resultant macromolecules are popular in OPV, solar cells, and OLEDs, etc. due to light weight and flexible structures. Ma et al. (2014) had reported D-π-A type chiral copolymer 117 by joining BODIPY and N-alkylcarbazole via ethyne linkages (Chart 18). Chiral polymer 117 displayed red shifted fluorescence (624–650 nm) and small band gap of about 159–196 eV (Ma et al., 2014). Organic conjugated copolymers are popular for their applications in OLEDs and solar cells due to low cost and light weight; and their electronic properties can be fine-tuned by altering the donor and acceptor units in the polymer backbone. Such BODIPY based macromolecules can be synthesized by replacing the electron donor moieties with different aromatic heterocycles like fluorene, phenothiazine, bithiphene, and carbazole derivatives to enhance their photophysical and electrochemical properties for various applications (Ma et al., 2014).
Aza-BODIPYs are class of BODIPYs which are obtained by substitution of the meso-carbon (C-8) atom by nitrogen-atom (Balsukuri et al., 2018). This alteration shifts the absorption and emission maxima of the resultant aza-BODIPY toward NIR region (600–900 nm). Aza-BODIPYs (Balsukuri et al., 2016a) are excellent candidates for the deep tissue imaging as NIR fluorescent probes and as photosensitizers for PDT and DSSC applications. In 2016, Gupta et al. have reported synthesis and optical studies of donor-acceptor type NIR aza-BODIPYs 118–121 (Chart 19), having N-phenylcarbazole or N-butylcarbazole at the 1,7-positions of the BODIPY core (Balsukuri et al., 2016a). These molecules showed significantly red shifted (~100 nm) absorption and emission relative to the parent tetraphenylaza–BODIPY (Balsukuri et al., 2016b). Also, Fluorescence studies of these molecules suggested effective energy transfer (up to 93%) from donor groups to the aza–BODIPY core. This strategy validated that, simple substitution with energy-donor groups on aza–BODIPYs can induce large red shifts in their electronic spectra, and this approach can be applied to make novel NIR dyes. In 2017, Gawale's group reported synthesis of N-ethylcarbazole linked aza-BODIPYs 122–125 (Chart 19), and studied their efficiency to produce triplet excited state and singlet oxygen generation (Gawale et al., 2017). The presence of iodo groups at the beta-positions of the aza-BODIPY helped in the enhancement of intersystem crossing efficiency in these molecules. Aza-BODIPYs 122–125 (Chart 19) were able to have sufficiently long triplet excited state and showed 70% singlet oxygen generation efficiency. Also, authors reported application of these molecules in deep tissue photo-acoustic imaging and up to 2 cm deep photoacoustic imaging was successfully demonstrated by using 122 as contrast agent; making it a potential candidate for theranostic application.
Triplet photosensitizers based on porphyrins and transition metal complexes are widely used in photo-catalysis of organic transformations and PDT. However, triplet sensitizers based on BODIPYs have iodo- or bromo- substituents to induce efficient ISC in such molecules. Typically, triplet sensitizers show strong absorption in the visible region, corresponding to the chromophores present in the molecule; though, panchromatic absorption of such molecules is highly desirable for various applications. Zhao and co-workers have combined the BODIPY and aza-BODIPY units to prepare a triad 126 (Chart 20) with interesting photophysical properties (Guo et al., 2014). The triad 126 displayed wide absorption in the visible-red region; along with the intramolecular energy transfer from donor units (BODIPY) and acceptor unit (aza-BODIPY). The resonance energy transfer (RET) in the triad 126 (Chart 20) was helpful to populate triplet excited state upon visible light excitation; which was further used to generate singlet oxygen with 58% quantum yield (Guo et al., 2014). Liu et al. (2013) carried out theoretical calculations for better understanding of the electronic structures and linear absorption of the series of aza-BODIPYs. DFT studies were also performed to investigate the two-photon absorption (TPA) properties of the aza-BODIPYs having various substituents viz. thiophene, phenyl, N-alkylcarbazole, fluorine, and pyrene, etc. Among the series of molecules investigated, the aza-BODIPY 127 (Chart 21) with elongated π-conjugated system was predicted to show lower HOMO-LUMO energy gap. Another interesting report by Huang's group discussed the synthesis of color tunable NIR aza-BODIPY 128 (Chart 21) and its application for sensing mercury ions (Liu et al., 2014). The introduction of aromatic carbazole ring induced red-shifts in the absorption and emission spectra; aza-BODIPY 128 (Chart 21) exhibited strong absorption peaks at 720 and 736 nm along with intense fluorescence at 848 nm. The two thienyl groups in the aza-BODIPY 128 served as binding pocket for mercury ion and its fluorescence was quenched after Hg+ binding making it a “turn-off” type fluorescent probe (Liu et al., 2014).
Ziessel et al. prepared BODIPYs 129–131 (Chart 22) substituted with triazatruxene (TAT) moiety at alpha-styryl or meso-phenyl positions of the BODIPY skeleton (Bura et al., 2011). TAT is a star shaped molecule consisting of three fused carbazole rings with flat aromatic structure; its derivatives have shown good two-photon absorption (TPA) properties and high hole mobility. The TAT substituted BODIPYs 129–131 exhibited large absorption coefficients and strong emission around 655–675 nm. The interesting electrochemical properties of molecules 129–131 (Chart 22) were examined, these blue dyes exhibited photovoltaic efficiencies in the range of 0.08–0.9% in bulk heterojunction solar cells (Bura et al., 2011).
Carbazole is an electron rich aromatic heterocycle and its derivatives are known for their good electronic and hole transport properties; therefore, carbazole conjugated systems have found application in DSSCs and OLEDs. Carbazole based dendrimers can be linked to other chromophores to enhance the absorption and emission properties of such dyes; D-π-A (Donor-π-Acceptor) type BODIPY core dendrimers 132–134 (Chart 23) were synthesized by Babu et al. (2018). The carbazole based dendrimers having BODIPY at the center, displayed rise in their absorption coefficients and red shifted emission upon moving from G0 to G2 generations (Chart 24). Compound 134 (G3 dendrimer) showed 2.7% light to energy conversion as sensitizer in DSSC, which was higher than the G0 and G1 dendrimers. Such carbazole based dendrimers are attractive alternatives for solar light harvesting materials, as compared to small organic molecules (Babu et al., 2018).
Typically, BODIPY dyes have short lifetimes for singlet excited states and small Stokes shifts, which restrict their application in solar light harvesting systems. The linking of BODIPY chromophore to another metal complex can overcome such limitation; Chart 25 shows interesting dual emission systems 135 and 136 comprising of carbazole substituted BODIPY unit and Ru(II) polypyridyl unit (Swavey et al., 2019). Compound 135 acted as precursor for the BODIPY and Ru(II)polypyridyl conjugate 136; the major absorption band was significantly red shifted in the later (Chart 25). Also, the luminescence of the BODIPY unit in 136 was quenched due to the presence of Ru(II) polypyridyl unit; which could be attributed to the increased ISC and other non-radiative decay processes in such conjugates. Compound 136 generated significant singlet oxygen in acetonitrile solution, upon irradiation with long wavelength light; indicating its potential use in PDT (Swavey et al., 2019).
Sekar et al. have reported coumarin-carbazole conjugates and their BF2 complexes 137 and 138 (Chart 26); such D-π-A systems displayed intramolcular charge-transfer process from donor carbazole ring to the coumarin acceptor unit (Rajeshirke et al., 2018). For conjugates 137 and 138, the emission maxima were observed at 592 and 627 nm, respectively. The strong fluorescence of the BF2 complexed coumarin unit in the red region was attributed to the attachment of carbazole donor group; these dyes can be potentially useful for biological applications (Rajeshirke et al., 2018). The BF2 complexes of carbazole-benzimidazole conjugates 139 and 140 (Chart 26) were synthesized by Dutta et al. (2017). Both the compounds 139 and 140 displayed relatively large Stokes shifts (34–51 nm) as compared to the typical BODIPY dyes; such molecular scaffolds can be used to develop fluorescence probes in future.
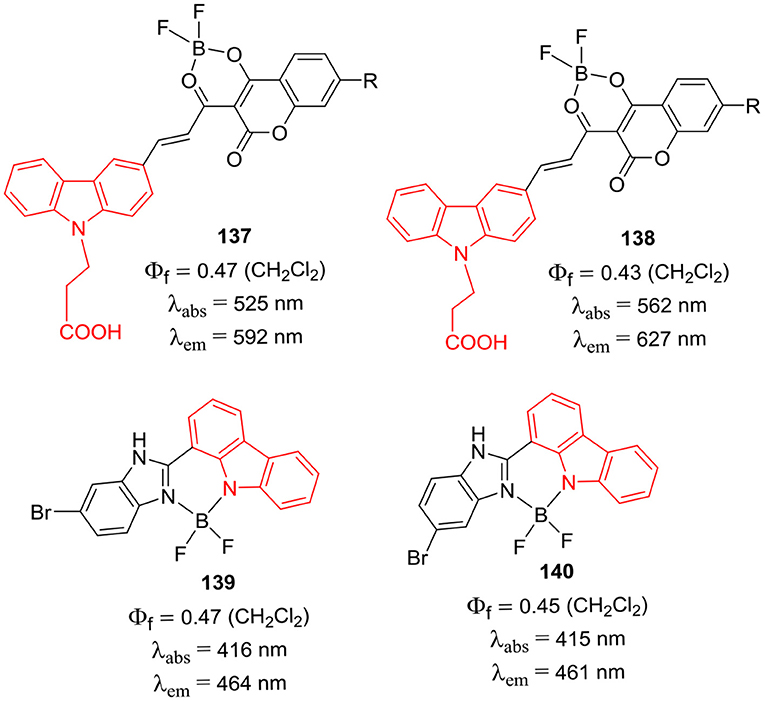
Chart 26. BF2 complexes of coumarin-carbazole conjugates (above); BF2 complexes of carbazole-benzimidazole conjugates (below).
Zhu et al. (2015) have prepared very interesting BF2 complexes 141 and 142 (Chart 27); where the aza-dipyrromethene skeleton was replaced by the aza-boron-diquinomethene. The aza-boron-diquinomethene scaffold was substituted with N-carbazolyl and/or 3,6-di-tert-butyl-N-carbazolyl moieties (Chart 27); the photoluminescence spectra of 141 and 142 showed green-yellow emission due to intramolecular charge transfer. The fluorescence quantum yields were reasonably high between 0.73 and 0.78; such BF2 complexes may be suitable for developing pH-sensors and bioimaging probes in future (Zhu et al., 2015). Ema et al. have reported a series of exciting BF2 complexes based on carbazole scaffold (Maeda et al., 2016); where carbazole ring was substituted with thiazole, benzothiazole, imidazole, indolone, and benzimidazole (Chart 28). The carbazole-based BF2 complexes 143–148 (Chart 28) displayed strong absorption (382–663 nm range) and fluorescence (427–796 nm range) in dichloromethane solution; the emission quantum yield were in the range of 0.074–0.547, except for molecule 145. The molecules 143–148 (Chart 28) exhibited large Stokes shifts of about 76–130 nm in the solutions.
These dyes also showed color tunable solid state emission with emission maxima between 424 and 542 nm range; with the quantum yields around 0.13–0.21. The solid state emission maxima were slightly red shifted relative to those in solution, which was attributed to the J-type packing in the solid state (Maeda et al., 2016). Same group had prepared BF2 complexes using organometallic approach, where carbazole was incorporated into the BODIPY framework (Maeda et al., 2015). The substitution of electron withdrawing or electron-donating groups on the carbazole skeleton altered the absorption and emission properties of 149 and 150 (Chart 29). The derivatives of 149 and 150 (Chart 29) showed absorption in the 292–493 nm range and fluorescence maxima between 508 and 650 nm. Also, the derivatives of 149 and 150 showed negligible fluorescence quantum yield with large Stokes shift of 46–142 nm (Maeda et al., 2015).
Ema et al. have also reported BF2 complexes of carbazole-benzobisthiazole 151 and biscarbazole-benzobisthiazole 152 (Chart 29), these dyes displayed solid state emission in red region (Maeda et al., 2017). The compound 151 exhibited absorption and fluorescence at 474 and 541 nm, respectively; with 40 nm Stokes shift. The derivatives of dimer 152 showed absorption maxima between 516 and 523 nm range and red shifted emission in the range of 547–573 nm, with 31–47 nm Stokes shifts. Interestingly, 151 and the derivatives of 152 (Chart 29) exhibited solid state fluorescence around 564–639 nm, such dyes may have potential application in organic photovoltaics due to strong fluorescence in red-NIR region. Recently, same group has synthesized BF2 complexes of carbazole-benzoxazole/carbazole-benzothiazole hybrids 153 and 154; which were further reacted with binapthyl derivative in the Al-mediated reaction to produce 155 and 156 (Chart 30, Maeda et al., 2019). These chiral dyes 155–160 showed circularly polarize luminescence in solution and in solid state. The major absorption band was centered around 438–468 nm and fluorescence maxima were in between 496 and 538 nm; the emission quantum yields were in between 0.22 and 0.44 with considerable Stokes shifts of around 72–75 nm. For compounds 155–160 (Chart 30), the solid state emission bands appeared between 524 and 581 nm; which were red shifted as compared to those in solution. Such chiral BF2 complexes of carbazole-benzoxoazole/carbazole-benzothiazole hybrids have potential applications as circularly polarized luminescence materials in the chiral fields (Maeda et al., 2017).
Summary
Carbazole-containing BODIPYs, carbazole-fused BODIPYs have become quite popular in the recent past; owing to their applications in live cell imaging, light harvesting systems, photovoltaics, and electroluminescent materials. The excellent hole-transport, photorefractive properties, and fluorescent nature of carbazole ring was exploited to design the BODIPY-carbazole conjugates with improved electronic and photovoltaic properties for DSSC and OLED applications. Various synthetic strategies were employed to substitute the three available positions (alpha, beta and meso) of the BODIPY skeleton; this resulted in the spurt of reports on carbazole substituted BODIPYs. The substitution of electron rich carbazole ring and its derivative on the BODIPY skeleton affected the spectral properties of the parent dye; which reflected in the red shifted absorption and emission maxima of the carbazole-BODIPY conjugates. Typically, direct linkage of carbazole ring on the alpha- and meso-positions of the BODIPY skeleton caused decent to large Stoke's shifts with fluorescence in the NIR region. Overall, the optical, photophysical, photoluminescent properties of the BODIPY dye can be fine- tuned for the desired application by substituting the carbazole derivatives on the BODIPY core; this knowledge can lead to the development of better more efficient BODIPY dyes in the near future.
Author Contributions
All authors listed have made a substantial, direct and intellectual contribution to the work, and approved it for publication.
Conflict of Interest
The authors declare that the research was conducted in the absence of any commercial or financial relationships that could be construed as a potential conflict of interest.
Abbreviations
AIEE, aggregation induced enhanced emission; BODIPY, difluoroboron dipyrromethene; CDCA, chenodeoxycholic acid; DSSC, dye sensitized solar cell; FET, field effect transistor; FRET, fluorescence resonance energy transfer; ICT, inrtamolecular charge transfer; LHE, light harvesting efficiency; OLED, oraganic light emitting diode; OPV, organic photovoltaic; PET, photoinduced energy transfer; TPEF, two-photon emission fluorescence; TTA, triplet–triplet annihilation.
References
Babu, J., Ganesan, S., Karuppusamy, M., and Rajakumar, P. (2018). Synthesis, photophysical, electrochemical properties, DFT studies and DSSC performance of BODIPY cored triazole bridged 3,6-ditertiary butyl carbazole decorated dendrimers. Chem. Sel. 3, 9222–9231. doi: 10.1002/slct.201801794
Balsukuri, N., Boruah, N. J., Kesavan, P. E., and Gupta, I. (2018). Near infra-red dyes based on pyrene aza-BODIPYs. New J. Chem. 42, 5875–5888. doi: 10.1039/C7NJ03408C
Balsukuri, N., Lone, M. Y., Jha, P. C., Mori, S., and Gupta, I. (2016a). Synthesis, structure, and optical studies of donor-acceptor-type near-infrared (NIR) aza-boron-dipyrromethene (BODIPY) dyes. Chem. Asian J. 11, 1572–1587. doi: 10.1002/asia.201600167
Balsukuri, N., Mori, S., and Gupta, I. (2016b). Donor acceptor type ferrocene substituted aza-BODIPYs: synthesis, optical and electrochemical studies. J. Porphy. Phthaloc. 20, 719–729. doi: 10.1142/S1088424616500693
Bandyopadhyay, S., Kundu, S., Giri, A., and Patra, A. (2018). A smart photosensitizer based on a red emitting solution processable porous polymer: generation of reactive oxygen species. Chem. Com. 54, 9123–9126. doi: 10.1039/C8CC04328K
Barberis, V. P., and Mikroyannidis, J. A. (2006). Novel blue luminescent twin molecules containing fluorene, carbazole or phenothiazine units. Synth. Met. 156, 1408–1414. doi: 10.1016/j.synthmet.2006.11.007
Bessette, A., and Hanan, G. S. (2014). Design, synthesis and photophysical studies of dipyrromethene-based materials: insights into their applications in organic photovoltaic devices. Chem. Soc. Rev. 43, 3342–3405. doi: 10.1039/C3CS60411J
Boens, N., Leen, V., and Dehaen, W. (2012). Fluorescent indicators based on BODIPY. Chem. Soc. Rev. 41, 1130–1172. doi: 10.1039/C1CS15132K
Brzeczek, A., Piwowar, K., Domagala, W., Mikolajczyk, M. M., Walczak, K., and Wagner, P. (2016). Systematic elongation of thienyl linkers and their effect on optical and electrochemical properties in carbazole-BODIPY donor-acceptor systems. RSC Adv. 6, 36500–36509. doi: 10.1039/C6RA04984B
Bura, T., Leclerc, N., Fall, S., Lévêque, P., Heiser, T., and Ziessel, R. (2011). Absorption tuning of monosubstituted triazatruxenes for bulk heterojunction solar cells. Org. Lett. 13, 6030–6033. doi: 10.1021/ol202443g
Celli, J. P., Spring, B. Q., Rizvi, I., Evans, C. L., Samkoe, K. S., Verma, S., et al. (2010). Imaging and photodynamic therapy: mechanisms, monitoring, and optimization. Chem. Rev. 110, 2795–2838. doi: 10.1021/cr900300p
Cheema, H., Younts, R., Gautam, B., Gundogdu, K., and El-Shafei, A. (2016). Design and synthesis of BODIPY sensitizers with long alkyl chains tethered to N-carbazole and their application for dye sensitized solar cells. Mater. Chem. Phys. 184, 57–63. doi: 10.1016/j.matchemphys.2016.09.024
Corona-Sánchez, R., Arcos-Ramos, R., Maldonado-Domínguez, M., Amelines-Sarria, O., Jerezano-Domínguez, A., García-Ortega, H., et al. (2016). Structural and morphological studies of meso-ethylcarbazole F-BODIPY thin films. Monatsh. Fur Chem. 147, 1915–1923. doi: 10.1007/s00706-016-1760-4
Das, S., and Gupta, I. (2019). Synthetic aspects of carbazole containing porphyrins and porphyrinoids. J. Porphy. Phthaloc. 23, 367–409. doi: 10.1142/S1088424619300052
Dhokale, B., Jadhav, T., Mobin, S. M., and Misra, R. (2015). Meso enyne substituted BODIPYs: synthesis, structure and properties. Dalton Trans. 44, 15803–15812. doi: 10.1039/C5DT00565E
Dutta, R., Firmansyah, D., Yoo, J., Kumar, R., Mulugeta, E., Jo, H., et al. (2017). BF2-complexes of carbazole–benzimidazole conjugates: synthesis, structures, and spectroscopic properties. Bull. Korean Chem. Soc. 38, 1163–1168. doi: 10.1002/bkcs.11240
Eçik, E. T., Özcan, E., Kandemir, H., Sengul, I. F., and Çoşut, B. (2017). Light harvesting systems composed of carbazole based subphthalocyanine-BODIPY enhanced with intramolecular fluorescence resonance energy transfer (FRET). Dyes Pigmen. 136, 441–449. doi: 10.1016/j.dyepig.2016.09.003
Fan, J., Hu, M., Zhan, P., and Peng, X. (2013). Energy transfer cassettes based on organic fluorophores: construction and applications in ratiometric sensing. Chem. Soc. Rev. 42, 29–43. doi: 10.1039/C2CS35273G
Gawale, Y., Adarsh, N., Kalva, S. K., Joseph, J., Pramanik, M., Ramaiah, D., et al. (2017). Carbazole-linked near-infrared aza-BODIPY dyes as triplet sensitizers and photoacoustic contrast agents for deep-tissue imaging. Chem. Eur. J. 23, 6570–6578. doi: 10.1002/chem.201605702
Głuszyńska, A. (2015). Biological potential of carbazole derivatives. Eur. J. Med. Chem. 94, 405–426. doi: 10.1016/j.ejmech.2015.02.059
Godoy, J., Vives, G., and Tour, J. M. (2010). Synthesis of highly fluorescent BODIPY-based nanocars. Org. Lett. 12, 1464–1467. doi: 10.1021/ol100108r
Guo, S., Ma, L., Zhao, J., Küçüköz, B., Karatay, A., Hayvali, M., et al. (2014). BODIPY triads triplet photosensitizers enhanced with intramolecular resonance energy transfer (RET): broadband visible light absorption and application in photooxidation. Chem. Sci. 5, 489–500. doi: 10.1039/C3SC52323C
Huang, L., Li, Z., Zhao, Y., Zhang, Y., Wu, S., Zhao, J., et al. (2016). Ultralow-power near infrared lamp light operable targeted organic nanoparticle photodynamic therapy. J. Am. Chem. Soc. 138, 14586–14591. doi: 10.1021/jacs.6b05390
Jadhav, T., Misra, R., Biswas, S., and Sharma, G. D. (2015). Bulk heterojunction organic solar cells based on carbazole-BODIPY conjugate small molecules as donors with high open circuit voltage. Phys. Chem. Chem. Phys. 17, 26580–26588. doi: 10.1039/C5CP04807A
Kamkaew, A., Lim, S. H., Lee, H. B., Kiew, L. V., Chung, L. Y., and Burgess, K. (2013). BODIPY dyes in photodynamic therapy. Chem. Soc. Rev. 42, 77–88. doi: 10.1039/C2CS35216H
Kesavan, P. E., Behera, R. N., Mori, S., and Gupta, I. (2017). Carbazole substituted BODIPYs: synthesis, computational, electrochemical and DSSC studies. J. Fluoresc. 27, 2131–2144. doi: 10.1007/s10895-017-2152-9
Kesavan, P. E., Das, S., Lone, M. Y., Jha, P. C., Mori, S., and Gupta, I. (2015). Bridged bis-BODIPYs: their synthesis, structures and properties. Dalton Trans. 44, 17209–17221. doi: 10.1039/C5DT01925G
Kesavan, P. E., and Gupta, I. (2014). Carbazole substituted boron dipyrromethenes. Dalton Trans. 43, 12405–12413. doi: 10.1039/C4DT01160K
Kesavan, P. E., Pandey, V., Raza, M. K., Mori, S., and Gupta, I. (2019). Water soluble thioglycosylated BODIPYs for mitochondria targeted cytotoxicity. Bioorg. Chem. 91:103139. doi: 10.1016/j.bioorg.2019.103139
Khetubol, A., Van Snick, S., Clark, M. L., Fron, E., Coutiño-González, E., Cloet, A., et al. (2015). Improved spectral coverage and fluorescence quenching in donor-acceptor systems involving indolo[3-2-b]carbazole and boron-dipyrromethene or diketopyrrolopyrrole. Photochem. Photobiol. 91, 637–653. doi: 10.1111/php.12437
Klfout, H., Stewart, A., Elkhalifa, M., and He, H. (2017). BODIPYs for dye-sensitized solar cells. ACS Appl. Mater. Interfaces 9, 39873–39889. doi: 10.1021/acsami.7b07688
Kolemen, S., and Akkaya, E. U. (2018). Reaction-based BODIPY probes for selective bio-imaging. Coord. Chem. Rev. 354, 121–134. doi: 10.1016/j.ccr.2017.06.021
Kowada, T., and Kikuchi, K. (2015). BODIPY-based probes for the fluorescence imaging of biomolecules in living cells. Chem. Soc. Rev. 44, 4953–4972. doi: 10.1039/C5CS00030K
Kurowska, A., Brzeczek-Szafran, A., Zassowski, P., Lapkowski, M., Domagala, W., Wagner, P., et al. (2018). Mono and di-substituted BODIPY with electron donating carbazole, thiophene, and 3,4-ethylenedioxythiophene units. Electrochim. Acta 271, 685–698. doi: 10.1016/j.electacta.2018.03.044
Lakshmi, V., Rajeswara Rao, M., and Ravikanth, M. (2015). Halogenated boron-dipyrromethenes: synthesis, properties and applications. Org. Biomol. Chem. 13, 2501–2517. doi: 10.1039/C4OB02293A
Lakshmi, V., Sharma, R., and Ravikanth, M. (2016). Functionalized boron-dipyrromethenes and their applications. Rep. Org. Chem. 6, 1–24. doi: 10.2147/ROC.S60504
Li, Q., and Qian, Y. (2016). Aggregation-induced emission enhancement and cell imaging of a novel (carbazol-N-yl)triphenylamine–BODIPY. New J. Chem. 40, 7095–7101. doi: 10.1039/C6NJ01495J
Li, Y., Ding, J., Day, M., Tao, Y., Lu, J., and D'iorio, M. (2004). Synthesis and properties of random and alternating fluorene/carbazole copolymers for use in blue light-emitting devices. Chem. Mater. 16, 2165–2173. doi: 10.1021/cm030069g
Liao, J., Xu, Y., Zhao, H., Zong, Q., and Fang, Y. (2017a). Novel A-D-A type small molecules with β -alkynylated BODIPY flanks for bulk heterojunction solar cells. Org. Electron. 49, 321–333. doi: 10.1016/j.orgel.2017.06.054
Liao, J., Zhao, H., Xu, Y., Cai, Z., Peng, Z., Zhang, W., et al. (2016). Novel D–A–D type dyes based on BODIPY platform for solution processed organic solar cells. Dyes Pigm. 128, 31–140. doi: 10.1016/j.dyepig.2016.01.020
Liao, J., Zhao, H., Xu, Y., Zhou, W., Peng, F., Wang, Y., et al. (2017b). Novel BODIPY dyes with electron donor variety for dye-sensitized solar cells. RSC Adv. 7, 33975–33985. doi: 10.1039/C7RA04402J
Lin, H. Y., Huang, W. C., Chen, Y. C., Chou, H. H., Hsu, C. Y., Lin, J. T., et al. (2012). BODIPY dyes with β-conjugation and their applications for high-efficiency inverted small molecule solar cells. Chem. Commun. 48, 8913–8915. doi: 10.1039/c2cc34286c
Liu, S., Shi, Z., Xu, W., Yang, H., Xi, N., Liu, X., et al. (2014). A class of wavelength-tunable near-infrared aza-BODIPY dyes and their application for sensing mercury ion. Dyes Pigmen. 103, 145–153. doi: 10.1016/j.dyepig.2013.12.004
Liu, X., Zhang, J., Li, K., Sun, X., Wu, Z., Ren, A., et al. (2013). New insights into two-photon absorption properties of functionalized aza-BODIPY dyes at telecommunication wavelengths: a theoretical study. Phy. Chem. Chem. Phys. 15, 4666–4676. doi: 10.1039/c3cp44435j
Loudet, A., and Burgess, K. (2007). BODIPY dyes and their derivatives: syntheses and spectroscopic properties. Chem. Rev. 107, 4891–4932. doi: 10.1021/cr078381n
Lu, H., Mack, J., Yang, Y., and Shen, Z. (2014). Structural modification strategies for the rational design of red/NIR region BODIPYs. Chem. Soc. Rev. 43, 4778–4823. doi: 10.1039/C4CS00030G
Lv, H. J., Zhang, X. T., Wang, S., and Xing, G. W. (2017). Assembly of BODIPY-carbazole dyes with liposomes to fabricate fluorescent nanoparticles for lysosomal bioimaging in living cells. Analyst 142, 603–607. doi: 10.1039/C6AN02705A
Ma, X., Azeem, E. A., Liu, X., Cheng, Y., and Zhu, C. (2014). Synthesis and tunable chiroptical properties of chiral BODIPY-based D-π-A conjugated polymers. J. Mater. Chem. C 2, 1076–1084. doi: 10.1039/C3TC32029D
Ma, X., Mao, X., Zhang, S., Huang, X., Cheng, Y., and Zhu, C. (2013). Aza-BODIPY-based D–π-A conjugated polymers with tunable band gap: synthesis and near-infrared emission. Polym. Chem. 4, 520–527. doi: 10.1039/C2PY20677C
Maeda, C., Nagahata, K., Takaishi, K., and Ema, T. (2019). Synthesis of chiral carbazole-based BODIPYs showing circularly polarized luminescence. Chem. Com. 55, 3136–3139. doi: 10.1039/C9CC00894B
Maeda, C., Todaka, T., and Ema, T. (2015). Carbazole-based boron dipyrromethenes (BODIPYs): facile synthesis, structures, and fine-tunable optical properties. Org. Lett. 17, 3090–3093. doi: 10.1021/acs.orglett.5b01363
Maeda, C., Todaka, T., Ueda, T., and Ema, T. (2016). Color-tunable solid-state fluorescence emission from carbazole-based BODIPYs. Chem. Eur. J. 22, 7508–7513. doi: 10.1002/chem.201505150
Maeda, C., Todaka, T., Ueda, T., and Ema, T. (2017). Synthesis of carbazole-based BODIPY dimers showing red fluorescence in the solid state. Org. Biomol. Chem. 15, 9283–9287. doi: 10.1039/C7OB02419C
Mani, V., Krishnakumar, V. G., Gupta, S., Mori, S., and Gupta, I. (2017). Synthesis and characterization of styryl-BODIPY derivatives for monitoring in vitro Tau aggregation. Sens. Actuators B Chem. 244, 673–683. doi: 10.1016/j.snb.2016.12.104
Mao, M., Li, Q. S., Zhang, X. L., Wu, G. H., Dai, C. G., and Ding, Y. (2017). Effects of donors of bodipy dyes on the performance of dye-sensitized solar cells. Dyes Pigm. 141, 148–160. doi: 10.1016/j.dyepig.2017.02.017
Misra, R., Jadhav, T., Dhokale, B., Gautam, P., Sharma, R., Maragani, R., et al. (2014). Carbazole-BODIPY conjugates: design, synthesis, structure and properties. Dalton Trans. 43:13076. doi: 10.1039/C4DT00983E
Mudadu, M. S., Singh, A. N., and Thummel, R. P. (2008). Preparation and study of 1,8-Di(pyrid-2′-yl)carbazoles. J. Org. Chem. 73, 6513–6520. doi: 10.1021/jo801132w
Nguyen, Q. P. B., Hwang, H. M., Song, M. -S., Song, H. J., Kim, G. H., Kwon, J. H., et al. (2014). Synthesis and electroluminescent properties of OLED green dopants based on BODIPY derivatives. Bull. Korean Chem. Soc. 35, 1247–1250. doi: 10.5012/bkcs.2014.35.4.1247
Ni, Y., and Wu, J. (2014). Far-red and near infrared BODIPY dyes: synthesis and applications for fluorescent pH probes and bio-imaging. Org. Biomol. Chem. 12, 3774–3791. doi: 10.1039/c3ob42554a
Ooyama, Y., Hagiwara, Y., Mizumo, T., Harima, Y., and Ohshita, J. (2013a). Photovoltaic performance of dye-sensitized solar cells based on D–π-A type BODIPY dye with two pyridyl groups. New J. Chem. 37, 2479–2485. doi: 10.1039/c3nj00456b
Ooyama, Y., Hagiwara, Y., Mizumo, T., Harima, Y., and Ohshita, J. (2013b). Synthesis of diphenylamino-carbazole substituted BODIPY dyes and their photovoltaic performance in dye-sensitized solar cells. RSC Adv. 3, 18099–18106. doi: 10.1039/c3ra43577f
Ooyama, Y., Hagiwara, Y., Oda, Y., Fukuoka, H., and Ohshita, J. (2014). BODIPY dye possessing solid-state red fluorescence and green metallic luster properties in both crystalline and amorphous states. RSC Adv. 4, 1163–1167. doi: 10.1039/C3RA45785K
Ooyama, Y., Kanda, M., EnoKi, T., Adachi, Y., and Ohshita, J. (2017). Synthesis, optical and electrochemical properties, and photovoltaic performance of a panchromatic and near-infrared (D)2–π-A type BODIPY dye with pyridyl group or cyanoacrylic acid. RSC Adv. 7, 13072–13081. doi: 10.1039/C7RA00799J
Popere, B. C., Pelle, A. M. D., Poe, A., Balaji, G., and Thayumanavan, S. (2012). Predictably tuning the frontier molecular orbital energy levels of panchromatic low band gap BODIPY-based conjugated polymers. Chem. Sci. 3, 3093–3102. doi: 10.1039/c2sc20731a
Promarak, V., Ichikawa, M., Sudyoadsuk, T., Saengsuwan, S., Jungsuttiwong, S., and Keawin, T. (2008). Thermally and electrochemically stable amorphous hole-transporting materials based on carbazole dendrimers for electroluminescent devices. Thin Solid Films 516, 2881–2888. doi: 10.1016/j.tsf.2007.05.062
Rajeshirke, M., Tathe, A. B., and Sekar, N. (2018). Viscosity sensitive fluorescent coumarin-carbazole chalcones and their BF 2 complexes containing carboxylic acid – synthesis and solvatochromism. J. Mol. Liq. 264, 358–366. doi: 10.1016/j.molliq.2018.05.074
Reddy, G., Duvva, N., Seetharaman, S., DSouza, F., and Lingamallu, G. (2018). Photoinduced energy transfer in carbazole-BODIPY dyads. Phys. Chem. Chem. Phy. 20, 27418–27428. doi: 10.1039/C8CP05509B
Satoh, T., Fujii, K., Kimura, Y., and Matano, Y. (2018). Synthesis of 3,5-disubstituted BODIPYs bearing N-containing five-membered heteroaryl groups via nucleophilic C–N bond formation. J. Org. Chem. 83, 5274–5281. doi: 10.1021/acs.joc.8b00087
Sengul, I. F., Okutan, E., Kandemir, H., Astarci, E., and Çoşut, B. (2015). Carbazole substituted BODIPY dyes: synthesis, photophysical properties and antitumor activity. Dyes Pigm. 123, 32–38. doi: 10.1016/j.dyepig.2015.07.025
Strahan, J., Popere, B. C., Khomein, P., Pointer, C. A., Martin, S. M., Oldacre, A. N., et al. (2019). Modulating absorption and charge transfer in bodipy-carbazole donor-acceptor dyads through molecular design. Dalton Trans. 48, 8488–8501. doi: 10.1039/C9DT00094A
Su, D., Oh, J., Lee, S. C., Lim, J. M., Sahu, S., Yu, X., et al. (2014a). Dark to light! a new strategy for large stokes shift dyes: coupling of a dark donor with tunable high quantum yield acceptors. Chem. Sci. 5, 4812–4818. doi: 10.1039/C4SC01821D
Su, D., Teoh, C. L., Sahu, S., Das, R. K., and Chang, Y. T. (2014b). Live cells imaging using a turn-on FRET-based BODIPY probe for biothiols. Biomaterials 35, 6078–6085. doi: 10.1016/j.biomaterials.2014.04.035
Swavey, S., Wertz, A., and Erb, J. (2019). Bichromophoric properties of ruthenium(II) polypyridyl complexes bridged by boron dipyrromethenes: synthesis, electrochemical, spectroscopic, computational evaluation, and plasmid DNA photoreactions. Chem. Eur. J. 2019, 3690–3698. doi: 10.1002/ejic.201900527
Tang, J., Hua, J., Wu, W., Li, J., Jin, Z., Long, Y., et al. (2010). New starburst sensitizer with carbazole antennas for efficient and stable dye-sensitized solar cells. Energy. Environ. Sci. 3, 1736–1745. doi: 10.1039/c0ee00008f
Telore, R. D., Jadhav, A. G., and Sekar, N. (2016). NLOphoric and solid state emissive BODIPY dyes containing N -phenylcarbazole core at meso position – synthesis, photophysical properties of and DFT studies. J. Lumin. 179, 420–428. doi: 10.1016/j.jlumin.2016.07.035
Thorat, K. G., Kamble, P., Ray, A. K., and Sekar, N. (2015). Novel pyrromethene dyes with N-ethyl carbazole at the meso position: a comprehensive photophysical, lasing, photostability and TD-DFT study. Phys. Chem. Chem. Phys. 17, 17221–17236. doi: 10.1039/C5CP01741F
Treibs, A., and Kreuzer, F. H. (1968). Difluorboryl-komplexe von Di-und tripyrrylmethenen. Justus Liebigs Ann. Chem. 718, 208–223. doi: 10.1002/jlac.19687180119
Ulrich, G., Ziessel, R., and Harriman, A. (2008). The chemistry of fluorescent bodipy dyes: versatility unsurpassed. Angew. Chem. Int. Ed. 47, 1184–1201. doi: 10.1002/anie.200702070
Vedamalai, M., and Gupta, I. (2018). Design and synthesis of the BODIPY–BSA complex for biological applications. Luminescence 33, 10–14. doi: 10.1002/bio.3365
Vedamalai, M., Kedaria, D., Vasita, R., and Gupta, I. (2018). Oxidation of phenothiazine based fluorescent probe for hypochlorite and its application to live cell imaging. Sens. Actu. B Chem. 263, 137–142. doi: 10.1016/j.snb.2018.02.071
Vedamalai, M., Kedaria, D., Vasita, R., Mori, S., and Gupta, I. (2016). Design and synthesis of BODIPY-clickate based Hg2+ sensors: The effect of triazole binding mode with Hg2+ on signal transduction. Dalton Trans. 45, 2700–2708. doi: 10.1039/C5DT04042F
Wang, Z.-S., Koumura, N., Cui, Y., Takahashi, M., Sekiguchi, H., Mori, A., et al. (2008). Hexylthiophene-functionalized carbazole dyes for efficient molecular photovoltaics: tuning of solar-cell performance by structural modification. Chem. Mater. 20, 3993–4003. doi: 10.1021/cm8003276
Wanwong, S., Khomein, P., and Thayumanavan, S. (2018). BODIPY dyads and triads: synthesis, optical, electrochemical and transistor properties. Chem. Cent. J. 12:60. doi: 10.1186/s13065-018-0430-5
Wei, Y., Zhou, M., Zhou, Q., Zhou, X., Liu, S., Zhang, S., et al. (2017). Triplet-triplet annihilation upconversion kinetics of C 60 -Bodipy dyads as organic triplet photosensitizers. Phy. Chem. Chem. Phy. 19, 22049–22060. doi: 10.1039/C7CP03840B
Wood, T. E., and Thompson, A. (2007). Advances in the chemistry of dipyrrins and their complexes. Chem. Rev. 107, 1831–1861. doi: 10.1021/cr050052c
Wu, J., Kwon, B., Liu, W., Anslyn, E. V., Wang, P., and Kim, J. S. (2015). Chromogenic/fluorogenic ensemble chemosensing systems. Chem. Rev. 115, 7893–7943. doi: 10.1021/cr500553d
Xia, X., and Qian, Y. (2018). NIR two-photon fluorescent probe for biothiol detection and imaging of living cells in vivo. Analyst 143, 5218–5224. doi: 10.1039/C8AN01605D
Yang, P., Wu, W., Zhao, J., Huang, D., and Yi, X. (2012). Using C60-bodipy dyads that show strong absorption of visible light and long-lived triplet excited states as organic triplet photosensitizers for triplet–triplet annihilation upconversion. J. Mater. Chem. 22, 20273–20283. doi: 10.1039/c2jm34353c
Yi, X., Zhao, J., Sun, J., Guo, S., and Zhang, H. (2013). Visible light-absorbing rhenium(i) tricarbonyl complexes as triplet photosensitizers in photooxidation and triplet–triplet annihilation upconversion. Dalton Trans. 42, 2062–2074. doi: 10.1039/C2DT32420B
Yuan, L., Lin, W., Zheng, K., He, L., and Huang, W. (2013). Far-red to near infrared analyte-responsive fluorescent probes based on organic fluorophore platforms for fluorescence imaging. Chem. Soc. Rev. 42, 622–661. doi: 10.1039/C2CS35313J
Zampetti, A., Minotto, A., Squeo, B. M., Gregoriou, V. G., Allard, S., and Scherf, U. (2017). Highly efficient solid-state near-infrared organic light-emitting diodes incorporating ADA dyes based on α, β-unsubstituted “BODIPY” moieties. Sci. Rep. 7:1611. doi: 10.1038/s41598-017-01785-2
Zhang, D., Wang, Y., Xiao, Y., Qian, S., and Qian, X. (2009). Long-wavelength boradiazaindacene derivatives with two-photon absorption activity and strong emission: versatile candidates for biological imaging applications. Tetrahedron 65, 8099–8103. doi: 10.1016/j.tet.2009.08.002
Zhang, J., Lu, F., Qi, S., Zhao, Y., Wang, K., Zhang, B., et al. (2016). Influence of various electron-donating triarylamine groups in BODIPY sensitizers on the performance of dye-sensitized solar cells. Dyes Pigm. 128, 296–303. doi: 10.1016/j.dyepig.2016.02.008
Zhang, X., Xiao, Y., Qi, J., Qu, J., Kim, B., Yue, X., et al. (2013). Long-wavelength, photostable, two-photon excitable BODIPY fluorophores readily modifiable for molecular probes. J. Org. Chem. 78, 9153–9160. doi: 10.1021/jo401379g
Zhang, X., Zhang, Y., Chen, L., and Xiao, Y. (2015). Star-shaped carbazole-based BODIPY derivatives with improved hole transportation and near-infrared absorption for small-molecule organic solar cells with high open-circuit voltages. RSC Adv. 5, 32283–32289. doi: 10.1039/C5RA02414E
Zheng, Z., Zhang, T., Liu, H., Chen, Y., Kwok, R. T. K., Ma, C., et al. (2018). Bright near-infrared aggregation-induced emission luminogens with strong two-photon absorption, excellent organelle specificity, and efficient photodynamic therapy potential. ACS Nano 12, 8145–8159. doi: 10.1021/acsnano.8b03138
Zhou, Y., Cheung, Y. K., Ma, C., Zhao, S., Gao, D., Lo, P. C., et al. (2018). Endoplasmic reticulum-localized two-photon-absorbing boron dipyrromethenes as advanced photosensitizers for photodynamic therapy. J. Med. Chem. 61, 3952–3961. doi: 10.1021/acs.jmedchem.7b01907
Zhu, X., Huang, H., Liu, R., Jin, X., Li, Y., Wang, D., et al. (2015). Aza-boron-diquinomethene complexes bearing N-aryl chromophores: synthesis, crystal structures, tunable photophysics, the protonation effect and their application as pH sensors. J. Mater. Com. C 3, 3774–3782. doi: 10.1039/C4TC02955K
Ziessel, R., Ulrich, G., and Harriman, A. (2007). The chemistry of bodipy: a new El Dorado for fluorescence tools. New J.Chem. 31, 496–501. doi: 10.1039/b617972j
Keywords: BODIPY, carbazole, fluorescence, absorption, dyes
Citation: Gupta I and Kesavan PE (2019) Carbazole Substituted BODIPYs. Front. Chem. 7:841. doi: 10.3389/fchem.2019.00841
Received: 02 September 2019; Accepted: 19 November 2019;
Published: 10 December 2019.
Edited by:
Zhen Shen, Nanjing University, ChinaCopyright © 2019 Gupta and Kesavan. This is an open-access article distributed under the terms of the Creative Commons Attribution License (CC BY). The use, distribution or reproduction in other forums is permitted, provided the original author(s) and the copyright owner(s) are credited and that the original publication in this journal is cited, in accordance with accepted academic practice. No use, distribution or reproduction is permitted which does not comply with these terms.
*Correspondence: Iti Gupta, iti@iitgn.ac.in