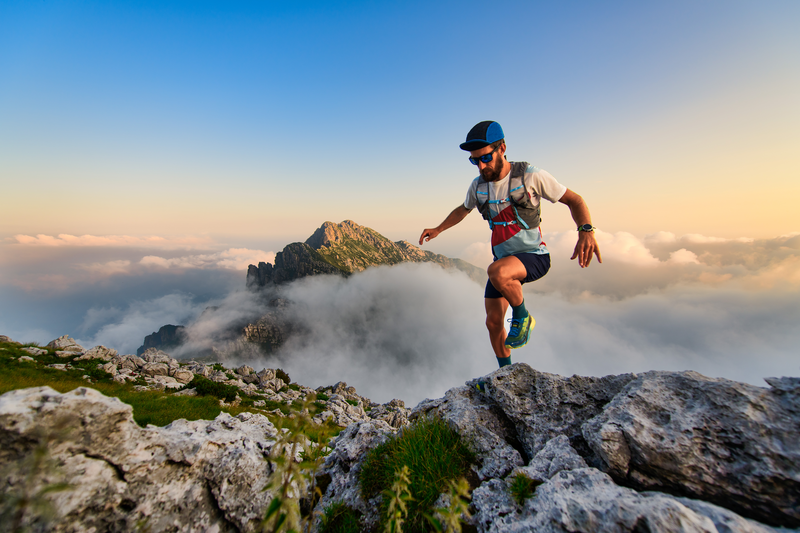
94% of researchers rate our articles as excellent or good
Learn more about the work of our research integrity team to safeguard the quality of each article we publish.
Find out more
MINI REVIEW article
Front. Chem. , 10 December 2019
Sec. Nanoscience
Volume 7 - 2019 | https://doi.org/10.3389/fchem.2019.00839
This article is part of the Research Topic Low-Dimension Sensing Nanomaterials View all 21 articles
Gas sensor, as one of the most important devices to detect noxious gases, provides a vital way to monitor the concentration and environmental information of gas in order to guarantee the safety of production. Therefore, researches on high sensitivity, high selectivity, and high stability have become hot issues. Since the discovery of the nanomaterial, it has been increasingly applied to the gas sensor for its distinguishing surface performances. However, 0-D and 1-D nanomaterials, with limited electronic confinement effect and surface effect, cannot reach the requirement for the production of gas sensors. This paper gives an introduction about the current researching progress and development trend of 2-D nanomaterials, analyzes the common forms of 2-D nanoscale structure, and summarizes the mechanism of gas sensing. Then, widely concerned factors including morphological properties and crystalline structure of 2-D nanomaterial, impact of doped metal on the sensibility of gas sensors, impact of symmetry, and working temperature on the selectivity of gas sensors have been demonstrated in detail. In all, the detailed analysis above has pointed out a way for the development of new 2-D nanomaterial and enhancing the sensibility of gas sensors.
Nanotechnology, a newly developed technology based on quantum mechanics, molecular biology, material science, microelectronics, and computer technology, is a scientific way to synthesize new materials on nanoscales. Prof. Tanggulachi firstly defined this newly emerged subject as nanotechnology in 1974 and clarified that the research on the characteristics and applications of nanomaterial should be restricted to the scale of 0.1–100 nm (Zhu et al., 2010; Huang et al., 2014). Nanomaterial has dramatic advantages over traditional material. Those advantages include distinguishing surface effect (Zhang, 2011) and quantum size effect (Xu et al., 2019). Factors like tiny particles, large surface areas, and high surface energy will enhance the performances of nanomaterial tremendously (Wang, 2013).
Gas sensor (You et al., 2012) converts the components and concentrations of various gases into standard electrical signals by using specific physical and chemical effects. It has been widely used in the detection of noxious and harmful gases and natural gas leakage. It has been improved greatly since the 1970s, with nanomaterials changed from single metallic oxide to combined metal oxide. Large progress has also been made on the sensing performances like sensitivity, accuracy, and stability when detecting specific variety of gas (Gonullu et al., 2012; Trung et al., 2012; Xu et al., 2012).
In recent years, the development of electronic devices is more about integration, miniaturization, and even microminiaturization. Nanomaterial plays an increasingly important role in the improvement of gas sensors. Based on the gas-sensing properties of 2-D nanomaterials such as response speed, selectivity, and stability, this paper gives a review of the factors that influence the performance of 2-D nanomaterial gas sensors and proposes the future development trend of the improvement of these sensors' parameters.
Since the successful extraction of graphene (a 2-D nanoscale graphite with single atomic layer) by the Geim Group (Geim and Novoselov, 2007; Geim, 2009; Perreault et al., 2015; Varghese et al., 2015) from Manchester University, UK, in 2004, more and more researchers have been attracted to the study of 2-D nanomaterial. According to numerous researches, nanomaterials are sorted into four categories by their number of nanoscale dimension. Each nanomaterial has different gas sensitivities due to electronics confinement effect, surface effect, etc. Generally speaking, three dimensions of 0-D nanomaterial are all in the scale of nanometers. Those 0-D nanomaterials include nanoscale particles, metallic cluster, etc. 1-D nanomaterial has two dimensions in nanoscale, with the other one of non-nanoscale size, such as the organic chain structures of nanoscale tube (Ma et al., 2014), nanoscale line (Tao et al., 2011), nanoscale band (Sun et al., 2003), nanoscale rod (Zhang et al., 2003), etc. 2-D nanomaterial only has one dimension of nanoscale, such as nanoscale membrane, 2-D single layer structure (Li et al., 2009), and nanoscale sheets (Liu et al., 2016). 3-D nanomaterial, such as nanoscale flowers (Wang and Rogach, 2014), etc. can be sorted into organic and inorganic nanomaterials according to its components.
In 2000, Kong et al. proved that a 1-D carbon nanoscale tube (CNTs) can detect the existence of NH3 and NO2 at low concentration under room temperature. CNTs have high absorption efficiency with rich adsorption structures and adsorption points, and they also have great value when it comes to application (Kong et al., 2000). In 2009, based on 2-D nanomaterial, Zhang et al. developed the SnO2 hollow microsphere, which was used for NO2 sensor. The results showed that SnO2 hollow sphere sensors can respond to NO2 at ppm level under 160°C and distinguishing selectivity (Zhang et al., 2009). In 2013, Sharm et al. produced a WO3 cluster/tin oxide heterostructure that can detect NO2 with low concentration of 10 ppm under 100°C (Sharma et al., 2013). In 2014, Rumyantsev et al. synthesized 2-D thin-film transistor MoS2 as well as figured out the CV graph of it. Comparing this MoS2 with ethanol, hexanenitrile, toluene, chloroform, and methanol on a time–current graph, a conclusion can be drawn that this material had better selectivity to alcohols (Shur et al., 2014). By using MoS2 layers of different thicknesses, photodetection of gas could be achieved (Wen et al., 2018). In 2016, Pang et al. used CNTs doped with nanoscale SnO2 particles to produce formic acid gas sensors, with their sensitivity reaching 13.49 (Pang et al., 2016). Also, this material lowered the working temperature by 120°C and shortened the response time by 4 s. Sun et al. constructed a graphene-like single-layered nanoscale structure (shown in Figure 1B). Though this material was a non-magnetic conductor, it had metallic characteristics. It could be a new research material in the nanotechnology field (Sun et al., 2016). In 2017, Tao et al. used ultrasonic spray pyrolysis with electrostatic enhancement to produce 2-DMWCNTs/SnO2 nanocomposite. When the deposition temperature was 300°C with MWCNTs' doping amount reaching 10 mg/ml, the performances of gas-sensitive material have improved greatly (Tao, 2017). In 2017, Yuan obtained 2-D PS/WO3 hollow nanoscale gas sensor with thick membrane by spin coating WO3 hollow nanoscale structure on the surface of PS. The experiment showed that this material had high sensitivity and distinguishing response characteristics when facing ppb level of NO2 (Yuan, 2017). In 2018, in order to cope with the problems that pure phase α − MoO3 had (excessively high working temperature, low stability, and long response time), Yu synthesized 2-DTiO2/α − MoO3 nanosheet, α − MoO3 − PANI p − n heterojuction, Au/α − MoO3 composite nanosheet, etc., which improved its sensitivity and stability greatly and shortened the response time (Yu, 2018). Shen et al. applied chemical vapor deposition (CVD) to synthesize ZnO nanofilm on the glass substrate, with the material having the best sensitivity to ethanol at room temperature (shown in Figure 1A) (Shen et al., 2018). Wang et al. composed Zn-Sn-O superlattice nanoscale particle (shown in Figure 1C), which had good selectivity and extremely high sensitivity to H2S (Wang, 2018). In 2019, Yu et al. made a new SnO2 nanoscale sheet structure (shown in Figure 1D), with the sensitivity of 12.14 at its best working temperature of 175°C and concentration of 100 ppm. This nanomaterial showed a tremendous improvement of sensitivity to gas like ethanol and formaldehyde (Yue and Yu, 2019). Kou's group systematically demonstrated the electronic, structure, and transport characters of monolayer BP with the adsorption of several typical gas molecules, CO, NH3, CO, NO2, and NO (Liu and Zhou, 2019). In 2018, Qiu et al. used ultraviolet rays and ozone method to in-situ synthesize oxide graphene membrane. The gas sensor of high performance can be made by combining oxide graphene membrane and two-terminal electrical devices. This sensor has higher sensitivity to NH3, and better selectivity to NH3 compared with acetone and absolute ethanol (Qiu et al., 2018). In 2019, Yang's study for dissertation proved this conclusion (Yang, 2019). Guo developed graphene/polyaniline material and analyzed its gas-sensing performances to multiple gases including NH3, CO, NO, H2, etc. The results showed that this material is characterized by higher selectivity to NH3 because of higher sensitivity and stronger adsorption to it (Guo, 2018).
Figure 1. Electron microscopic images of partial 2-D nanomaterials. (A) ZnO nanoscale membrane (adapted from Shen et al., 2018 with permission from Shen). (B) 2-D B3N2 single-layer structure (adapted from Sun et al., 2016 with permission from Sun). (C) InMO3(ZnO)m superlattice (adapted from Wang, 2018 with permission from Wang). (D) New SnO2 nanoscale sheets (adapted from Yue and Yu, 2019 with permission from Yue).
The detecting principle of gas sensor is that gas molecules are adsorbed on the surface of the substrate nanomaterial. Then, charge transfer occurs between the gas molecule and the substrate material, which changes the resistivity of the substrate nanomaterial. By testing the resistivity of the substrate nanomaterial, characteristics of gas such as properties and concentration can be known. When it comes to substrate nanomaterial, process of choosing from 0-D quantum dot, 1-D quantum wire, to 2-D quantum surface is experienced. A large number of studies have shown that 2-D nanomaterial has larger surface compared with 0-D and 1-D nanomaterials. Special membranous or lamellar structure has stronger capability to absorb gas molecules. Meanwhile the gas sensitivity can be improved by metal doping to pure nanomaterial (Dai and Yuan, 2010; Beheshtian et al., 2012a,b; Zhang et al., 2012; Rastegar et al., 2013; Ahmad et al., 2019; Choi et al., 2019; Kim et al., 2019). Take graphene for instance. Pure graphene absorbs common gas molecules physically, which has a large limitation on gas sensitivity (Feng et al., 2012; Meng et al., 2013; Abideen et al., 2018; Mirzaei et al., 2019; Mourya et al., 2019). The performance of graphene can be changed by metal doping. Arsenene 2-D semiconductor structure (Fleurence et al., 2012), antimony-vinyl folded honeycomb 2-D structure, and telluriene structure can be produced by imitating the graphene's structure (Liu, 2017). In conclusion, 2-D nanomaterial has higher sensitivity and selectivity when compared with other materials on gas-sensing mechanism.
Gas-sensitive materials have many properties, such as sensitivity, selectivity, stability, response time, etc., which are directly related to the surface characteristics of material. Surface characteristics are decided by the particle size of material. When the scale of the material reaches nano size, its surface area and surface activity will increase. Nanomaterials can be designed into different shapes, which will greatly increase the capability of absorbing specific gases. Therefore, gas sensors made by those nanomaterials will have their performances enhanced dramatically. Take SnO2 as an example; the porous hollow rod SnO2 composed of 1-D nanoscale rod provides more paths for gaseous diffusion, due to massive petal shape nanosheets and pores. The conversion of sphere-like structures into petal-like ones and successful synthesis of 1-D nanoscale rod and cones when composing flower-like SnO2 improves the gas sensitivity and shortens the response time. In order to avoid the performance degradation caused by accumulation of nanostructure, the porous flower-like SnO2 structure composed by 2-D nanosheets not only enlarges the surface area of the structure but also increases the internal hole channels as well, which will promote gas diffusion. Finally, flake-like layered SnO2 structure composed by numerous thin nanosheets marks further improvement on the material's surface activity and shows excellent gas selectivity and sensitivity in the test (shown in Table 1).
Table 1. Relationship between microstructure, preparation, and gas sensitivity in 2-D nanomaterial (WO3, ZnO).
Improving the response sensitivity of nanomaterial gas sensor is crucial in practical engineering applications. Response sensitivity can be improved by (1) changing the surface of the 2-D nanomaterial (generally metallic oxide) to enhance the sensibility of the reaction 1-D nanofiber formed by nanoparticles will provide more route for the electron to move rapidly and bigger specific surface area to improve its sensibility; Cho et al. produced hemispherical NiO nanomaterial, which had a sensitivity of 1.5 to ethanol vapor (Cho et al., 2011); Song et al. produced nanotube NiO nanomaterial, with a sensitivity of 3 to ethanol vapor; the particle size and touching area are also the important factors on the sensitivity (Song et al., 2011), (2) changing the morphology of the nanomaterial Szilagyi et al. sintered ammonium tungstate compounds to produce hexagonal phase h-WO3, which was sensitive to H2S and monoclinic γ − WO3, CH4, H2, and CO (Szilagyi et al., 2010); Gao et al. used hydrothermal method to synthesize triclinic δ − WO3 square nanosheet, which had higher gas response sensitivity to cyclohexene (Gao et al., 2013), and (3) doping of transition metal to improve the sensitivity of nanomaterial Wang et al. doped Cr to produce ferroelectric monoclinic ε − WO3, which had better selectivity to acetone (Wang et al., 2008); Kim et al. prepared NiO doped with Fe3+ to make a gas sensor with the response value of 100 ppm ethanol improved from 5.5 to 172.5, which showed tremendous improvement of nanomaterial sensitivity by metallic doping (Kim, 2012).
Something interesting will be found when we combine special microstructure of nanomaterial with specific structure of different gases. This interesting finding will be the nanomaterial's specific selectivity to gases in macroscopic. Selectivity of 2-D nanomaterial is an important factor to measure the effect of materials. The lower symmetry of structure indicates the better selectivity of gas sensors. Take WO3 nanocrystal with exocentric structure as an example. The symmetry of triclinic WO3 crystal is lower than that of monoclinic and hexagonal WO3 crystals. So, the triclinic WO3 crystal has higher sensitivity and selectivity on acetone molecules with larger dipole moments (Bai, 2014). The influence of working temperature on the selectivity of materials is also an important factor to consider. Triclinic WO3 nanomaterial has better selectivity and sensitivity to acetone at higher temperature and better selectivity to NO2 at lower temperature (Zhao et al., 2013).
Throughout the development of nanotechnology, gas sensor based on nanomaterial is always an extremely important research field. It has deep and wide influence on life and production. However, 2-D nanomaterial research is still full of problems in the aspects ranging from imperfect sensitive materials and immature preparation technology to disability on scaled production. Gas sensor materials will be developed from single metallic oxide to composite oxide. Morphology of nanomaterial can be changed to improve the sensing performances; particle size can be reduced to improve the surface activity of the material; new structure can be designed to absorb more specific gases; selectivity of the sensors can be improved by reducing the asymmetry of 2-D nanomaterial structure and improving its working temperature.
2-D nanomaterial plays an increasingly important role in the further improvement of the gas sensor's performance. In recent years, the development trend of various electronic components tends to be more integrated, miniaturized, and even microminiaturized. Gas sensor will also consume less power, be multi-functional, and have higher performance. The material of gas sensor will be changed from simple gas-sensitive materials to complex composite materials. The structure of gas sensor will be changed from monolayer to multilayer and from simple morphology to special morphology. Also, it will be widely used in chemical production, gas transportation, and other toxic and harmful gas detection. In 2019, Tian et al. prepared ternary complex of graphene/WO3 nanorod/polythiophene (3D-r GO/WO3/PTh), and studied its gas sensitivity to H2S. The study results showed that under low temperature of 75°C, this material has fast response and distinguishing selectivity to H2S (Tian et al., 2019). Therefore, it can be found that the study of multiple element compound is a very popular research issue for the development of new gas sensor materials with better performance.
In this paper, gas-sensing properties of the 2-D nanomaterial are reviewed. Firstly, the classification of nanomaterials on the number of dimensions is briefly introduced, and the latest research progress and development trend of 2-D nanomaterial are summarized. Secondly, the gas-sensing mechanism of 2-D nanomaterial is summarized by comparing the characteristics of existing 2-D nanomaterials. The effects of particle size and morphology property of 2-D nanomaterial on the performance of gas sensors are discussed. Then, the enhancement related to morphology property, phase structure, and metal doping of 2-D nanomaterial on the sensitivity of gas sensors is analyzed. Also, the effect of symmetry structure of 2-D nanomaterial on the selectivity of gas sensors is concluded. Finally, the development trend of 2-D nanomaterials for gas sensors is proposed, and references for the next development of 2-D nanomaterial are provided.
XM and LG conceived and designed the experiments. ZH and GT performed the experiments. XM and JA analyzed the data. XM and QH wrote the manuscript with input from all authors. All authors read and approved the manuscript.
This work was supported by the international science and technology cooperation and exchange research project of Sichuan province (18GJHZ0195), downhole Intelligent Measurement and Control Science and technology innovation team of Southwest Petroleum University (2018CXTD04), and the National Natural Science Foundation (51974273 and 51874255).
The authors declare that the research was conducted in the absence of any commercial or financial relationships that could be construed as a potential conflict of interest.
Abideen, Z., Kim, J.-H., Mirzaei, A., Kim, W. H., and Kim, S. S. (2018). Sensing behavior to ppm-level gases and synergistic sensing mechanism in metal-functionalized rGO-loaded ZnO nanofibers. Sens. Actuat. B Chem. 255, 1884–1896. doi: 10.1016/j.snb.2017.08.210
Ahmad, R., Majhi, S. M., Zhang, X., Swager, T. M., and Salama, K. N. (2019). Recent progress and perspectives of gas sensors based on vertically oriented ZnO nanomaterials. Adv. Colloid Interface Sci. 270, 1–27. doi: 10.1016/j.cis.2019.05.006
Alenezi, M. R., Alshammari, A. S., and Jayawardena, K. I. (2013). Role of the exposed polar facets in the performance of thermally and UV activated ZnO nanostructured gas sensors. J. Phys. Chem. C 117, 17850–17858. doi: 10.1021/jp4061895
Bai, X. (2014). Microscopic morphology, phase and surface structure control and gas sensing properties of WO3 nanocrystals (master's thesis). Tianjin University, Tianjin, China.
Beheshtian, J., Peyghan, A. A., and Bagheri, Z. (2012a). Selective function of Al12N12 nano-cage towards NO and COmolecules. Comput. Mater. Sci. 62, 71–74. doi: 10.1016/j.commatsci.2012.05.041
Beheshtian, J., Peyghan, A. A., Bagheri, Z., and Kamfiroozi, M. (2012b). Interaction of small molecules (NO, H2, N2, and CH4) with BNnanocluster surface. Struct. Chem. 23, 1567–1572. doi: 10.1007/s11224-012-9970-9
Chen, X., Liu, J., and Jing, X. (2013). Self-assembly of ZnO nanosheets into flower-like architectures and their gas sensing properties. Mater. Lett. 112, 23–25. doi: 10.1016/j.matlet.2013.08.118
Cho, N. G., Hwang, I.-S., Kim, H.-G., Lee, J. H., and Kim, I. D. (2011). Gas sensing properties of p-type hollow Ni O hemispheres prepared by polymeric colloidal templating method. Sens. Actuat. B Chem. 155, 366–371. doi: 10.1016/j.snb.2010.12.031
Choi, M. S., Bang, J. H., Mirzaei, A., Na, H. G., Jin, C., Oum, W., et al. (2019). Exploration of the use of p-TeO2-branch/n-SnO2 core nanowires nanocomposites for gas sensing. Appl. Surf. Sci. 484, 1102–1110. doi: 10.1016/j.apsusc.2019.04.122
Choi, S. J., Lee, I., Jang, B. H., Youn, D. Y., Ryu, W. H., Park, C. O., et al. (2012). Selective diagnosis of diabetes using Pt-functionalized WO3 hemitube networks as a sensing layer of acetone in exhaled breath. Anal. Chem. 85, 1792–1796. doi: 10.1021/ac303148a
Dai, J., and Yuan, J. (2010). Adsorption of molecular oxygen on doped graphene: atomic, electronic, and magnetic properties. Phys. Rev. B 81:165414. doi: 10.1103/PhysRevB.81.165414
Feng, B., Ding, Z., Meng, S., Yao, Y., He, X., Cheng, P., et al. (2012). Evidence of silicene in honeycomb structures of silicon on Ag (111). Nano Lett. 12, 3507–3511. doi: 10.1021/nl301047g
Fleurence, A., Friedlein, R., Ozaki, T., Kawai, H., Wang, Y., and Yamada-Takamura, Y. (2012). Experimental evidence for epitaxial silicene on diboride thin films. Phys. Rev. Lett. 108:245501. doi: 10.1103/PhysRevLett.108.245501
Gao, X., Su, X., Yang, C., Xiao, F., Wang, J., Cao, X., et al. (2013). Hydrothermal synthesis of WO3 nanoplates as highly sensitive cyclohexene sensor and high-efficiency MB photocatalyst. Sens. Actuat. B Chem. 181, 537–543. doi: 10.1016/j.snb.2013.02.031
Geim, A. K. (2009). Graphene: status and prospects. Science 324, 1530–1534. doi: 10.1126/science.1158877
Geim, A. K., and Novoselov, K. S. (2007). The rise of graphene. Nat. Mater. 6, 183–191. doi: 10.1038/nmat1849
Gonullu, Y., Rodriguez, G. C. M., Saruhan, B., and Ürgen, M. (2012). Improvement of gas sensing performance of TiO2 towards NO2 by nano~tubular structuring. Sens. Actuat. B Chem. 169, 151–160. doi: 10.1016/j.snb.2012.04.050
Guo, Z. (2018). Research on gas sensitivity of graphene/polyaniline composite material based on multiscale analysis[D]. University of Wenzhou.
Huang, J., Xu, X., and Gu, C. (2011). Large-scale synthesis of hydrated tungsten oxide 3D architectures by a simple chemical solution route and their gas-sensing properties. J. Mater. Chem. 21, 13283–13289. doi: 10.1039/c1jm11292a
Huang, X., Tan, C. L., Yin, Z. Y., and Zhang, H. (2014). 25th anniversary article: hybrid nanostructures based on two-dimensional nanomaterials. Adv. Mater. 26, 2185–2204. doi: 10.1002/adma.201304964
Kim, J.-H., Mirzaei, A., Kim, H. W., and Kim, S. S. (2019). Combination of Pd loading and electron beam irradiation for superior hydrogen sensing of electrospun ZnO nanofibers. Sens. Actuat. B Chem. 284, 628–637. doi: 10.1016/j.snb.2018.12.120
Kim, K.-M. (2012). Highly sensitive C2H5OH sensors using Fe-doped NiO hollow spheres. Sens. Actuat. B Chem. 171–172, 1029–1037. doi: 10.1016/j.snb.2012.06.029
Kong, J., Franklin, N. R., Zhou, C., Chapline, M. G., Peng, S., Cho, K., et al. (2000). Nanotube molecular wires as chemical sensors. Science 287, 622–625. doi: 10.1126/science.287.5453.622
Li, Y., Guo, Y., Tan, R., Cui, P., Li, Y., and Song, W. (2009). Synthesis of SnO2 nano-sheets by a template-free hydrothermal method. Mater. Lett. 63, 2085–2088. doi: 10.1016/j.matlet.2009.06.060
Liu, B., and Zhou, K. (2019). Recent progress on graphene-analogous 2D nanomaterials: properties, modeling and applications. Prog. Mater. Sci. 100, 99–169. doi: 10.1016/j.pmatsci.2018.09.004
Liu, C. (2017). Gas sensing properties based on 2-D nanomaterials (Master's thesis). Nanjing University of Posts and Telecommunications, Nanjing, China.
Liu, J. X., Cao, H., Jiang, B., Xue, Y., and Fu, L. (2016). Application of emerging 2-D materials in flexible energy storage and conversion. Sci. China Mater. 59, 459–474. doi: 10.1007/s40843-016-5055-5
Ma, R., Hu, J., Cai, Z., and Ju, H. (2014). Highly selective enrichment of phosphopeptides with high-index facets exposed octahedral tin dioxide nanoparticles for mass spectrometric analysis. Talanta 119, 452–457. doi: 10.1016/j.talanta.2013.11.049
Meng, L., Wang, Y., Zhang, L., Du, S., Wu, R., Li, L., et al. (2013). Buckled silicene formation on Ir (111). Nano Lett. 13, 685–690. doi: 10.1021/nl304347w
Mirzaei, A., Yousefi, H. R., Falsafic, F., Bonyani, M., Lee, J.-H., Kim, J.-H., et al. (2019). An overview on how Pd on resistive-based nanomaterial gas sensors can enhance response toward hydrogen gas. Int. J. Hydrogen Energy 44, 20552–20571. doi: 10.1016/j.ijhydene.2019.05.180
Mourya, S., Kumar, A., Jaiswal, J., Malik, G., Kumar, B., Chandra, R., et al. (2019). Development of Pd-Pt functionalized high performance H2 gas sensor based on silicon carbide coated porous silicon for extreme environment applications. Sens. Actuat. B Chem. 283, 373–383. doi: 10.1016/j.snb.2018.12.042
Pang, J. H., Tan, Q. L., and Liu, W. Y. (2016). Preparation of CNT-SnO2 composites and study on formaldehyde gas sensitivity. Sci. Technol. Eng. 16, 53–57. doi: 10.3969/j.issn.1671-1815.2016.01.009
Perreault, F., de Faria, A. F., and Elimelech, M. (2015). Environmental applications of graphene-based nanomaterials. Chem. Soc. Rev. 44, 5861–5896. doi: 10.1039/C5CS00021A
Qin, Y., Hu, M., and Zhang, J. (2010). Microstructure characterization and NO2-sensing properties of tungsten oxide nanostructures. Sens. Actuat. B Chem. 150, 339–345. doi: 10.1016/j.snb.2010.06.063
Qiu, H. F., Zhao, D., Teng, J. Q., Jia, T. H., Lei, S. C., Wang, C., et al. (2018). A construction method and gas sensitive characteristics of gaphene oxide sensors. J. Xi'an Jiaotong University 52, 95–101. doi: 10.7652/xjtuxb201810013
Rastegar, S. F., Peyghan, A. A., Ghenaatian, H. R., and Hadipour, N. L. (2013). NO2 detection by nanosized Al N sheet in the presence of NH3: DFT studies. Appl. Surf. Sci. 274, 217–220. doi: 10.1016/j.apsusc.2013.03.019
Sharma, A., Tomar, M., and Gupta, V. (2013). WO3 nanoclusters-SnO2 film gas sensor heterostructure with enhanced response for NO2. Sens. Actuat. B Chem. 176, 675–684. doi: 10.1016/j.snb.2012.09.094
Shen, Z. D., Hu, J. J., Li, Z. Z., Zhao, W. L., Qu, J., and Fan, H. (2018). Study on the gas sensitivity of ZnO Nano-film materials to ethanol at room temperature. J. Hebei Inst. Architect. Eng. 36, 130–134. doi: 10.3969/j.issn.1008-4185.2018.04.028
Shur, M. S., Rumyantsev, S. L., Jiang, C., Samnakay, R., Renteria, J., and Balandin, A. A. (2014). Selective gas sensing with MoS2 thin film transistors. Sensors 2014, 55–57. doi: 10.1109/ICSENS.2014.6984931
Song, X., Gao, L., and Mathur, S. (2011). Synthesis, characterization, and gas sensing properties of porous nickel oxide nanotubes. J. Phys. Chem. C. 115, 21730–21735. doi: 10.1021/jp208093s
Su, X., Li, Y., and Jian, J. (2010). In situ etching WO3 nanoplates: hydrothermal synthesis, photoluminescence and gas sensor properties. Mater. Res. Bull. 45, 1960–1963. doi: 10.1016/j.materresbull.2010.08.011
Sun, N. N., Liu, Y., Li, Y., Ji, Q. C., Wang, L., and Zhang, Y. M. (2016). Study on electronic properties and stability of 2-D B3N2 monolayer structure. J. Hebei Tech. Coll. 4, 25–29. doi: 10.16046/j.cnki.issn1008-3782.2016.04.007
Sun, S. H., Meng, G. W., Zhang, G. X., Gao, T., Geng, B. Y., Zhang, L. D., et al. (2003). Raman scattering study of rutile SnO2 nanobelts synthesized by thermal evaporation of Sn powders. Chem. Phys. Lett. 376, 103–107. doi: 10.1016/S0009-2614(03)00965-5
Szilagyi, I. M., Saukko, S., and Mizsei, J. (2010). Gas sensing selectivity of hexagonal and monoclinic WO3 to H2S. Solid State Sci. 12, 1857–1860. doi: 10.1016/j.solidstatesciences.2010.01.019
Tao, Q. (2017). Fabrication and characterization of carbon nanotube/SnO2 flexible gas sensor (Master's thesis). Tianjin University of Technology, Tianjin, China.
Tao, T., Chen, Q., Hu, H., and Chen, Y. (2011). Tree-like SnO2 nanowires and optical properties. Mater. Chem. Phys. 126, 128–132. doi: 10.1016/j.matchemphys.2010.11.052
Tian, J. F., Yi, Z. G., and Han, G. L. (2019). Preparation of three-dimensional of Graphene/WO3 nanorod/polythiophene Composites and their gas sensing properties at low temperature. Sci. Technol. Eng. 19, 94–98.
Trung, D. D., Toan, N. V., Tong, P. V., Duy, N. V., Hoa, N. D., and Van Hieu, N. (2012). Synthesis of single~crystal SnO2 nanowires for NOx gas sensors application. Ceram. Int. 38, 6557–6563. doi: 10.1016/j.ceramint.2012.05.039
Varghese, S. S., Varghese, S. H., Swaminathan, S., Singh, K. K., and Mittal, V. (2015). Two-dimensional materials for sensing: graphene and beyond. Electronics 4, 651–687. doi: 10.3390/electronics4030651
Wang, H., and Rogach, A. L. (2014). Hierarchical SnO2 nanostructures: recent advances in design, synthesis, and applications. Chem. Mater. 26, 123–133. doi: 10.1021/cm4018248
Wang, J. (2013). Synthesis, characterization and regulation of nanoparticles in ion implanted transparent insulating materials (Master's thesis). University of Tianjin, Tianjin, China.
Wang, L., Teleki, A., and Pratsinis, S. (2008). Ferroelectric WO3 nanoparticles for acetone selective detection. Chem. Mater. 20, 4794–4796. doi: 10.1021/cm800761e
Wang, Q. G. (2018). Preparation and gas sensitivity of new Zn-Sn-O superlattice nanoparticles (Master's thesis). Southwest Jiaotong University, Chengdu, China.
Wang, X., Liu, W., Liu, J., Wang, F., Kong, J., Qiu, S., et al. (2012). Synthesis of nestlike ZnO hierarchically porous structures and analysis of their gas sensing properties. ACS Appl. Mater. Interfaces 4, 817–825. doi: 10.1021/am201476b
Wen, W., Song, Y., Yan, X., Zhu, C., Du, D., Wang, S., et al. (2018). Recent advances in emerging 2D nanomaterials for biosensing and bioimaging applications. Mater. Today 21, 164–177. doi: 10.1016/j.mattod.2017.09.001
Xu, T., Wang, S., Li, L., and Liu, X. (2019). Dual templated synthesis of tri-modal porous SrTiO3/TiO2@ carbon composites with enhanced photocatalytic activity. Appl. Catal. A 575, 132–141. doi: 10.1016/j.apcata.2019.02.017
Xu, X. M., Wang, D. M., Wang, W., Sun, P., Ma, J., Liang, X., et al. (2012). Porous hierarchical In2O3 nanostructures: Hydrothermal preparation and gas sensing properties. Sens. Actuat. B Chem. 171, 1066–1072. doi: 10.1016/j.snb.2012.06.035
Yang, J. Z. (2019). Preparation of graphene oxide gas sensitive material and its application in the detection of harmful and toxic gases[D]. Southwest University of Science and Technology, Mianyang, China.
You, L., He, X., Wang, D., Sun, P., Sun, Y. F., Liang, X. S., et al. (2012). Ultrasensitive and low operating temperature NO2 gas sensor using nanosheets assembled hierarchical WO3 hollow microspheres. Sens. Actuat. Chem. B. 173, 426–432. doi: 10.1016/j.snb.2012.07.029
Yu, H. Q. (2018). Direct fabrication and properties of 2-D alpha-MoO3 nano-material gas sensors (Master's thesis). Jinan University, Guangzhou, China.
Yuan, L. (2017). Research on porous silicon-based nano-tungsten oxide multi-level structure material gas sensor (Master's thesis). Tianjin University, Tianjin, China.
Yue, Y., and Yu, H. (2019). Study on gas sensitivity of new SnO2 nanosheets. Enterprise Sci. Technol. Dev. 6, 90–91. doi: 10.3969/j.issn.1674-0688.2019.06.040
Zhang, D., Sun, L., Yin, J., and Yan, C. (2003). Low-temperature fabrication of highly crystalline SnO2 nanorods. Adv. Mater. 15, 1022–1025. doi: 10.1002/adma.200304899
Zhang, J., Wang, S., Wang, Y., Wang, Y., Zhu, B., Xia, H., et al. (2009). NO2 sensing performance of SnO2 hollow-sphere sensor. Sens. Actuat. B Chem. 135, 610–617. doi: 10.1016/j.snb.2008.09.026
Zhang, T., Xue, Q., Shan, M., Jiao, Z., Zhou, X., Ling, C., et al. (2012). Adsorption and catalytic activation of O2 molecule on the surface of Au-doped graphene under an external electric field. J. Phys. Chem. C 116, 19918–19924. doi: 10.1021/jp3073359
Zhang, X. Z. (2011). Research progress of metal oxide semiconductor nanometer gas sensitive materials. Sci. Technol. Western China 10, 5–6. doi: 10.3969/j.issn.1671-6396.2011.32.003
Zhao, L., Tian, F. H., Wang, X., Zhao, W., Fu, A., Shen, Y., et al. (2013). Mechanism of CO adsorption on hexagonal WO3 (001) surface for gas sensing: a DFT study. Comput. Mater. Sci. 79, 691–697. doi: 10.1016/j.commatsci.2013.07.046
Keywords: 2-D nanomaterial, gas sensor, performance improvement, current application, development trend
Citation: Ge L, Mu X, Tian G, Huang Q, Ahmed J and Hu Z (2019) Current Applications of Gas Sensor Based on 2-D Nanomaterial: A Mini Review. Front. Chem. 7:839. doi: 10.3389/fchem.2019.00839
Received: 25 August 2019; Accepted: 18 November 2019;
Published: 10 December 2019.
Edited by:
Wen Zeng, Chongqing University, ChinaReviewed by:
Lihui Wang, China Conservatory, ChinaCopyright © 2019 Ge, Mu, Tian, Huang, Ahmed and Hu. This is an open-access article distributed under the terms of the Creative Commons Attribution License (CC BY). The use, distribution or reproduction in other forums is permitted, provided the original author(s) and the copyright owner(s) are credited and that the original publication in this journal is cited, in accordance with accepted academic practice. No use, distribution or reproduction is permitted which does not comply with these terms.
*Correspondence: Guiyun Tian, Zy55LnRpYW5AbmNsLmFjLnVr
Disclaimer: All claims expressed in this article are solely those of the authors and do not necessarily represent those of their affiliated organizations, or those of the publisher, the editors and the reviewers. Any product that may be evaluated in this article or claim that may be made by its manufacturer is not guaranteed or endorsed by the publisher.
Research integrity at Frontiers
Learn more about the work of our research integrity team to safeguard the quality of each article we publish.