- 1School of Chemical and Environmental Engineering, Shanghai Institute of Technology, Shanghai, China
- 2Key Laboratory of Inorganic-Organic Hybrid Functional Material Chemistry, Ministry of Education, Tianjin Key Laboratory of Structure and Performance for Functional Molecules, College of Chemistry, Tianjin Normal University, Tianjin, China
- 3Department of Chemistry, Center for Supramolecular Chemistry and Catalysis, Shanghai University, Shanghai, China
Here we report a new class of synthetic receptors, acyclic pillar[n]naphthalene (n = 2–4, Dimer, Trimer, and Tetramer) oligomers, which are made up of 2,3-diethoxynaphthalene units linked by methylene bridges at the 1- and 4-positions. They can be synthesized through a one-step condensation of 2,3-diethoxynaphthalene monomer and paraformaldehyde in the presence of BF3•(Et)2O catalyst. The crystal structure of Tetramer has an interesting pseudo-cycle shaped structure in the solid state. Their complexation behaviors toward several organic ammonium cations (1+-15+) and electron–deficient neutral guests (16–17), were examined by means of 1H NMR spectroscopy. Tetramer shows good host-guest properties toward the ammonium guests, giving association constants (Ka) in the magnitude of 102-104 M−1, which are comparable with those for some macrocyclic hosts.
Introduction
Since the discover of crown ethers, the development of hosts for recognizing various guest species has mainly focused on macrocyclic structures (Cram, 1988; Lehn, 1988; Pedersen, 1988; Gong et al., 2010; Chun et al., 2013; Jurícek et al., 2014; Liu et al., 2019). Methylene–bridged macrocyclic arenes, for example calixarenes (Baldini et al., 2007; Guo and Liu, 2012), pillararenes (Ogoshi et al., 2008; Xue et al., 2012; Wang et al., 2016; Yang et al., 2016), coronarenes (Wang, 2018), helic[6]arene (Zhang et al., 2016), biphenarenes (Chen et al., 2015; Dai et al., 2017; Li et al., 2019; Wang et al., 2019b), and etc. (Guo et al., 2018; Luo et al., 2018; Ma et al., 2018) have been widely used in host-guest chemistry, self-assembly materials, and biomedical field (Song and Yang, 2015; Alsbaiee et al., 2016; Li et al., 2017; Jie et al., 2018; Chen et al., 2019; Yang et al., 2019). Naphthalene-based macrocyclic arenes, termed as calixnaphthalenes, have also been produced (Poh et al., 1989; Andreetti et al., 1993; Shorthill et al., 2004; AlHujran et al., 2012; Avetta et al., 2012). However, calixnaphthalenes have not become highly popular receptors because they do not have unique molecular recognition properties. Considering that pillararenes with pillar-shape topologic structures have shown nice host-guest properties, we wondered whether we can create acyclic pillarnaphthalenes (Scheme 1), which would have deep, pillar-shape, and π-rich cavities, and maybe better binding abilities than calixnaphthalenes. As detailed below, we did not get such macrocycles, but succeed in making acyclic pillarnaphthalene oligomers.
Acyclic hosts that contain partially enclosed cavities capable of binding guests provided alternatives with unique synthetic and functional advantages (Goodman et al., 2007; Seebach and Gardiner, 2008; Pan et al., 2017; Wang et al., 2019a). For example, foldamers may provide cavities that are adaptive in recognizing different guest molecules (Zhang et al., 2012; Yashima et al., 2016). Molecular tweezers have made the way from a supramolecular host to a drug candidate, due to their ability to inhibit peptide and protein aggregation through the complexation toward amino acids (Sinha et al., 2011; Schrader et al., 2016).
Isaacs and his co-workers created acyclic cucurbit[n]uril-type receptors, which can function as solubilizing agents for insoluble drugs. Interestingly, the solubility of paclitaxel was increased 2,750 times through the formation of soluble container–drug complex (Ma et al., 2012). These highly soluble acyclic cucurbiturils could also solubilize individual single-walled carbon nanotubes (SWNTs) in water even at a concentration 100–1,000 times lower than typically required for surfactants (Shen et al., 2012). The groups of Schrader and Yoshizawa synthesized beautiful water-soluble clip and tweezer-shaped hosts based on norbornene and anthracene building blocks (Bier et al., 2013; Jono et al., 2017).
Herein, we wish to report the synthesis of a new type of receptors, acyclic pillar[n]naphthalene (n = 2–4, Dimer, Trimer, and Tetramer) oligomers, which are made up of 2,3-diethoxynaphthalene units linked by methylene bridges at the 1- and 4-positions. Tetramer, bearing a pseudo-cavity, has good host-guest properties toward a series of model organic cationic guests.
Materials and Methods
All the reagents involved in this research were commercially available and used without further purification unless otherwise noted. 1H NMR, 13C NMR, 2D NOESY, and COSY spectra (see Supplementary Material) were recorded with a Bruker AVANCE III 500 MHz instrument. Chemical shifts were referred to TMS. Highresolution mass spectra (HRMS) were determined on a Bruker Daltonics, Inc. APEXIII 7.0 TESLA FTMS instrument. The single crystal X-ray data were measured by direct methods using SHELXS-971 and refined by fullmatrix least-squares procedures on F2 with SHELXL-97.2. All non-hydrogen atoms were obtained from the difference Fourier map and subjected to anisotropic refinement by full-matrix least squares on F2. Hydrogen atoms were obtained geometrically and treated as riding on the parent atoms or were constrained in the locations during refinements. Test parameters and detailed experimental data are shown in the Supplementary Material.
Synthesis and Characterization
To the solution of 2,3-diethoxy naphthalene (2.6 g, 12 mmol) in CHCl3 (150 mL) was added paraformaldehyde (0.36 g, 12 mmol). Boron trifluoride diethyl etherate (2.5 ml, 20 mmol) was then added to the reaction mixture. The mixture was stirred at 25°C for 1 h. Then the reaction was quenched by addition of 50 mL water. The organic phase was separated and washed with saturated aqueous NaHCO3, and water. The organic layer was dried over anhydrous Na2SO4 and concentrated. The residue was purified by column chromatography on silica gel (eluent: 1/1, v/v, dichloromethane: petrol ether) to afford Dimer (21%), Trimer (9%), and Tetramer (15%), as white solids.
Dimer. m.p. 155–156°C. 1H NMR (500 MHz, CDCl3, 298 K): δ (ppm): 8.10 (d, J = 8.4 Hz, 2H), 7.61 (d, J = 7.7 Hz, 2H), 7.28–7.24 (m, 2H), 7.23–7.20 (m, 2H), 7.07 (s, 2H), 5.00 (s, 2H), 4.22 (q, J = 7.0 Hz, 4H), 4.02 (q, J = 7.0 Hz, 4H), 1.57 (t, J = 7.0 Hz, 6H), 1.33 (t, J = 7.0 Hz, 6H). 13C NMR (125 MHz, CDCl3, 298 K): δ (ppm): 151.36, 146.37, 131.43, 129.97, 128.57, 126.80, 124.88, 124.65, 123.71, 106.85 (C of acyclic dimer), 69.11, 63.80 (C of methylene in ethoxy group), 23.55 (C of methylene bridge of acyclic dimer), 15.58, 14.86 (C of methyl in ethoxy group). HRMS (ESI): C29H32O4, calcd m/z 462.2644; found m/z 462.2641.
Trimer. m.p. 171–172°C. 1H NMR (500 MHz, CDCl3, 298 K): δ (ppm): 8.17 (d, J = 8.6 Hz, 2H), 8.04 (dd, J = 6.5, 3.3 Hz, 2H), 7.57 (d, J = 7.8 Hz, 2H), 7.23 (dd, J = 11.0, 4.0 Hz, 2H), 7.14–7.09 (m, 4H), 7.01 (s, 2H), 4.93 (s, 4H), 4.20 – 4.11 (m, 8H), 3.87 (q, J = 7.0 Hz, 4H), 1.51 (t, J = 7.0 Hz, 6H), 1.35 (t, J = 7.0 Hz, 6H), 1.13 (t, J = 7.0 Hz, 6H). 13C NMR (125 MHz, CDCl3, 298 K): δ (ppm): 151.37, 148.98, 146.30, 131.42, 130.70, 130.15, 128.93, 128.49, 126.81, 125.16, 124.90, 124.53, 124.39, 123.24, 106.78 (C of acyclic trimer), 69.21, 69.10, 63.79 (C of methylene in ethoxy group), 23.37 (C of methylene bridge of acyclic trimer), 15.77, 15.43, 14.84, 14.22 (C of methyl in ethoxy group). HRMS (ESI): C44H48O6, calcd m/z 690.3795; found m/z 690.3786.
Tetramer. m.p. 212–213°C. 1H NMR (500 MHz, CDCl3, 298 K): δ (ppm): 8.19 (d, J = 8.2 Hz, 2H), 8.13 (d, J = 8.5 Hz, 2H), 8.02 (d, J = 8.2 Hz, 2H), 7.58 (d, J = 8.0 Hz, 2H), 7.23 (t, J = 7.6 Hz, 2H), 7.16–7.04 (m, 6H), 7.02 (s, 2H), 4.92 (s, 4H), 4.89 (s, 2H), 4.17 (q, J = 7.0 Hz, 4H), 4.13 (q, J = 7.0 Hz, 4H), 3.95 (q, J = 7.0 Hz, 4H), 3.88 (q, J = 7.0 Hz, 4H), 1.52 (t, J = 6.9 Hz, 6H), 1.33 (t, J = 7.0 Hz, 3H), 1.16 (t, J = 7.0 Hz, 6H), 1.05 (t, J = 7.0 Hz, 6H). 13C NMR (125 MHz, CDCl3, 298 K): δ (ppm): 151.36, 149.00, 148.89, 146.25, 131.42, 130.71, 130.66, 130.17, 129.29, 128.78, 128.46, 126.80, 125.21, 125.19, 124.91, 124.56, 124.29, 123.92, 123.18, 106.72 (C of acyclic tetramer), 69.25, 69.13, 69.08, 63.77 (C of methylene in ethoxy group), 23.34 (C of methylene bridge of acyclic tetramer), 15.78, 15.48, 15.46, 14.85 (C of methyl in ethoxy group). HRMS (ESI): C59H64O8, calcd m/z 918.4945; found m/z 918.4922.
Results and Discussion
2,3-Diethoxy naphthalene was selected as the building block to condense with paraformaldehyde. Due to the electron-donating ethoxy groups, great regioselectivity can be rationalized, and the reactive sites should be 1- and 4-positions in Friedel–Crafts reaction. It was expected to produce pillar-shape macrocycles, pillar[n]naphthalenes. However, no cyclic oligomers have been obtained after many attempts; a possible reason is that big naphthalene units make the final cyclization quite difficult due to the steric hindrance. Fortunately, we got acyclic pillar[n]naphthalenes (n = 2–4).
Using BF3·(Et)2O as the catalyst, the condensation reaction of 2,3-diethoxy naphthalene and paraformaldehyde in CHCl3 at room temperature (Scheme 2) produced oligomers Dimer, Trimer, and Tetramer with yields of 21, 9, and 15%, respectively. Other Lewis acid catalysts, for example TfOH, FeCl3, and AlCl3, could also work, but the reaction yields were lower than that for BF3·(Et)2O. The synthesis was considerably easy since it just involved a one–step reaction of commercial starting materials and the isolation was also convenient by column chromatography on silica gel.
Dimer, Trimer, and Tetramer were well characterized by 1H NMR, 13C NMR, NOESY, and COSY spectra (Figure 1 and Supplementary Figures 1–11), and high-resolution mass spectrometry (HRMS). They have rather complex patterns of aromatic and ethoxy peaks in 1H NMR spectra (Figure 1) because they are not highly symmetrical macrocycles, but acyclic oligomers with low symmetry.
Single crystals of Dimer, Trimer, and Tetramer suitable for X-ray analysis were obtained by diffusion of hexane into a solution of the compounds in dichloromethane at room temperature (Figure 2). As expected, these three acyclic oligomers had the same connecting style, i.e., 2,3-diethoxy naphthalene units were connected by methylene at 1,4-positions. As shown in Figures 2A,B, the acyclic Dimer and Trimer, possessing two and three naphthalene moieties, have ill–defined cavities. Particularly, the Tetramer exhibits a pseudocycle–shaped structure, with all the methylene bridges being orientated outwardly. There exist intramolecular sextuple C–H···π interactions, with H···ring center distances of 2.75–3.23 Å (Supplementary Figure 12), between the middle two ethoxy groups and naphthalenes, resulting in the formation of a pseudo cycle rather than a zigzag structure. More interestingly, the single crystal structures of Tetramer molecules exist in a pair of enantiomers in the solid state (Figure 2D).
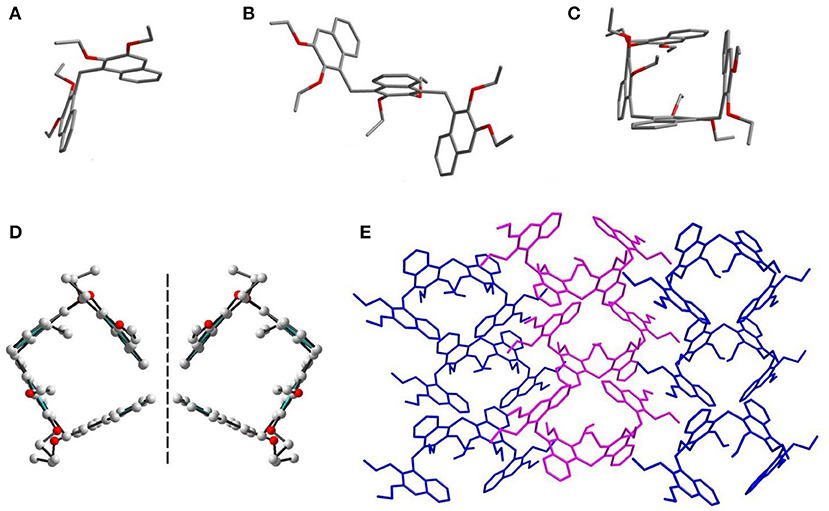
Figure 2. Crystal structures of Dimer (A), Trimer (B), and Tetramer (C). (D) A pair of enantiomers of Tetramer. (E) Packing mode of Tetramer.
The host-guest properties of the acyclic receptors were then tested. Since they possess π-rich cavities, several cationic guests (1+-15+) and electron–deficient neutral guests (16–17) (Scheme 3) were chosen as model guest molecules to investigate their host-guest chemistry. In most cases, CDCl3 was used as solvent during the 1H NMR experiments of host-guest mixture and following NMR titrations; for guests 72+, 92+, and 102+, CD2Cl2 was used because of their poor solubility in CDCl3.
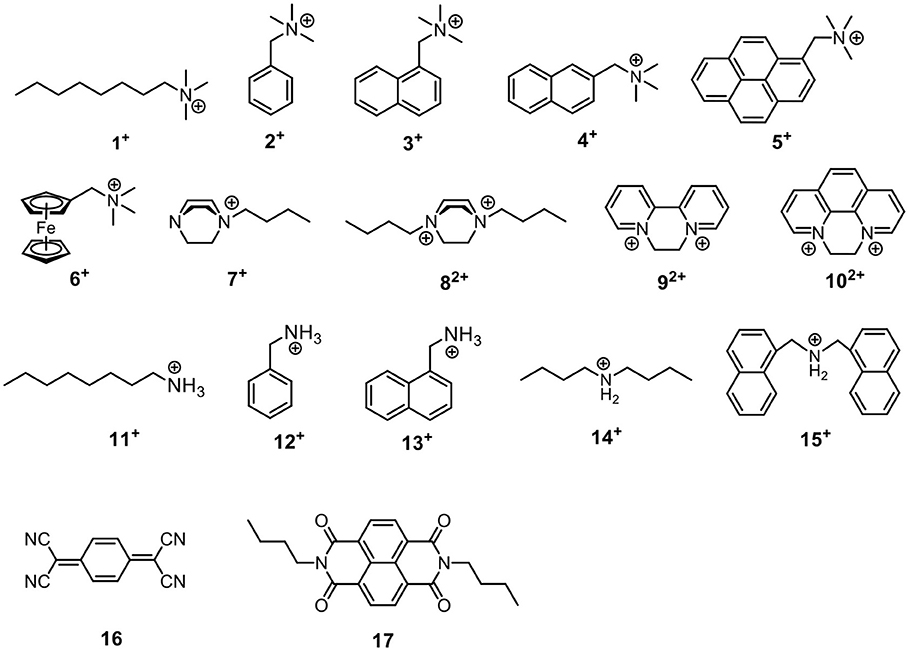
Scheme 3. Structures of guest molecules. The counter anions of 1+-15+ are tetrakis[3,5-bis(trifluoromethyl)phenyl] borate (BArF−).
Figure 3 shows the 1H NMR spectra recorded for quarternary ammonium guest 1+ in the absence and presence of Tetramer. As can be readily seen, upon addition of Tetramer, protons Ha, Hb and Hc of 1+ display substantial upfield shifts (Δδ = −0.39, −0.29, and −0.21 ppm) due to complexation–induced shielding effects, indicating that 1+ was located inside the acyclic host's pseudo-cavity to form a host-guest inclusion complex, and the main binding site is the N+(Me)3 moiety. In contrast, protons Hh−i undergo indistinct NMR changes, suggesting they are located outside the cavity of Tetramer. [24] In the NOESY spectrum of 1+ and Tetramer, NOE correlations were observed between methyl protons Ha of the guest and the aromatic protons H5, H7 and H8 of Tetramer, also suggesting the host-guest encapsulation (Supplementary Figure 13). The formation of 1+•Tetramer complex was further supported by ESI mass spectrometry analysis of an equimolar mixture of 1•BArF and Tetramer, where an intense peak for the 1:1 complex (m/z 1072.66, calcd. for 1+•Tetramer = 1072.67) was observed (Supplementary Figure 14). The encapsulation could also be rationalized by energy-minimized molecular modeling (Figure 3D): the oligomers wrapped around the guest to enhance the host-guest contacts driven by cation···π/ C–H···π interactions.
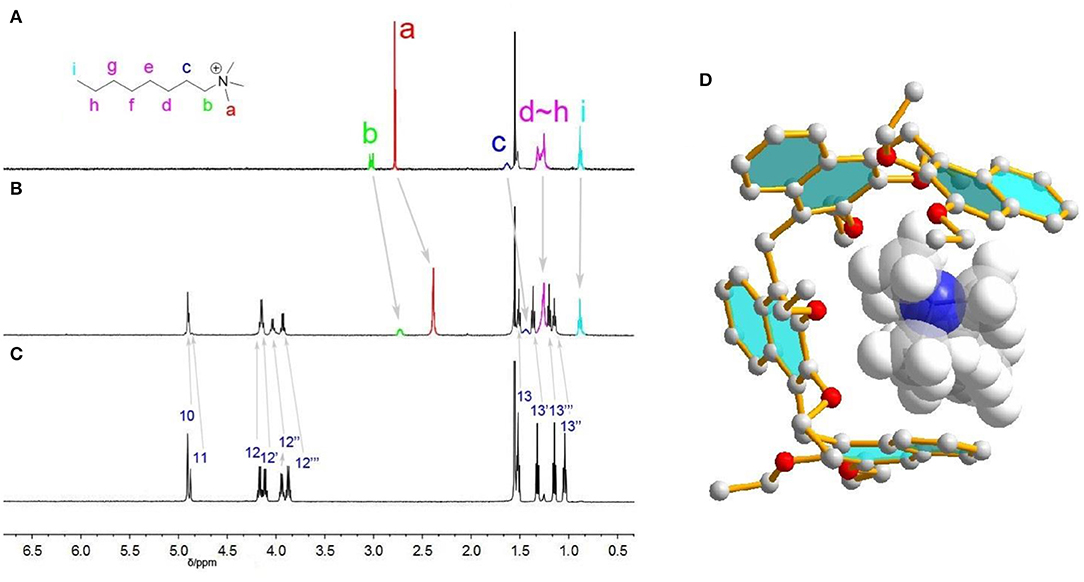
Figure 3. 1H NMR spectra (CDCl3, 298 K, 1.0 mmol) of (A) guest 1+, (B) 1+ and Tetramer (1:1 mixture), (C) Tetramer. (D) Energy-minimized structures of 1+•Tetramer at the semiempirical PM6 level of theory.
The addition of Dimer and Trimer could also induce the upfield shifts of guest 1+, but the Δδ values are smaller than those for Tetramer (Supplementary Figure 15). These results indicated relatively weak binding interactions occurred for Dimer and Trimer in comparison with Tetramer. These observations were consistent with the association constants (Ka) obtained from 1H NMR titration experiments. As shown in Table 1, the Ka value of 1+ with Tetramer [(4.4±0.6) × 102 M−1] is 18 times larger than that for Trimer, and the affinity for Dimer was too small to be accurately calculated (< 5 M−1).
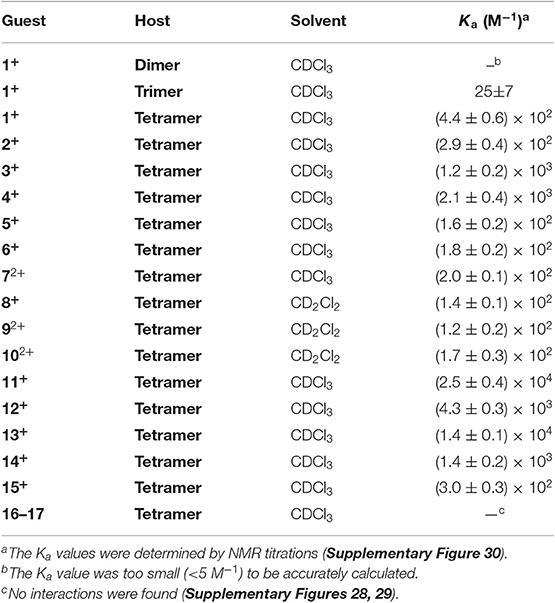
Table 1. Association constants (M−1) of Dimer, Trimer, and Tetramer with different guests (500 MHz, 298 K).
Since Tetramer showed interesting structure and good recognition behavior, we then examined its binding capacity toward other cationic guests (Table 1 and Supplementary Figures 16–29), revealing that Tetramer can form host–guest complexes with them but the binding affinities are totally different. For the trimethyl ammonium guests 1+-6+, 3+ [Ka = (1.2 ± 0.2) × 103 M−1] and 4+ [Ka = (2.1±0.4) × 103 M−1] bearing naphthyl moieties give stronger affinities, which should be due to host-guest fitted π ··· π interactions and large contacts. The substitution of naphthyl for smaller phenyl or bigger pyrenyl in 3+ and 4+, affording 1+ or 5+, considerably decreases the association constants by one order of magnitude.
Binding affinities of Tetramer toward primary ammonium guests 11+–13+ were stronger than those of the corresponding quaternary ammonium guests 1+–3+. For example, the Ka value of Tetramer and octylammonium 11+ [(2.5±0.4) × 104 M−1] is about 56-fold higher than that for trimethyloctylammonium 1+ [(4.4±0.6) × 102 M−1]. Similarly, the selectivity factors of 12+/2+ and 13+/2+ are 15 and 12, respectively. The reason for such high selectivity would be that big and spherical N+(Me)3 group is too larger compared with Tetramer's size, and small is a suitable one. It should be noted that the binding affinities of Tetramer and organic ammonium salts, with Ka values in the magnitude of 102-104 M−1, are comparable to those for macrocyclic arenes such as pillararenes and biphenarenes.
Due to its π-electron rich cavity, the complexation of Tetramer and two π-deficient neutral guests, 16 and 17, were also investigated. From Supplementary Figures 28, 29, no obvious NMR changes were detected, indicating no stable complexes can be formed.
Conclusions
In summary, acyclic pillarnaphthalenes with 2,3-diethoxynaphthalene units bridged by methylenes at 1,4-positions were synthesized through a one-pot reaction of 2,3-diethoxy naphthalene monomer and paraformaldehyde by using Lewis acid as the catalyst. Acyclic pillar[4]naphthalene Tetramer is able to interact organic ammonium guests cations by wrapping around them, giving association constants in the magnitude of 102-104 M−1. We expect that Tetramer bearing pseudo-cycle cavity, could have significant potential for the applications in host-guest chemistry and self-assembly.
Data Availability Statement
All datasets generated for this study are included in the article/Supplementary Material.
Author Contributions
CL, BW, and YJ conceived this project and designed the experiments. YJ and MD contributed to most of the experimental work. CL, MD, and BW co-wrote the paper. All authors discussed and commented on the paper and analyzed the data.
Funding
This work was supported by the National Natural Science Foundation of China (21772118 and 21472122), the Shanghai Pujiang Program (16PJD024), and the Shuguang Program.
Conflict of Interest
The authors declare that the research was conducted in the absence of any commercial or financial relationships that could be construed as a potential conflict of interest.
Supplementary Material
The Supplementary Material for this article can be found online at: https://www.frontiersin.org/articles/10.3389/fchem.2019.00828/full#supplementary-material
References
AlHujran, T. A., Dawe, L. N., and Georghiou, P. E. (2012). Synthesis of functionalized acenaphthenes and a new class of homooxacalixarenes. Org. Lett. 14, 3530–3533. doi: 10.1021/ol301538s
Alsbaiee, A., Smith, B. J., Xiao, L., Ling, Y., Helbling, D. E., and Dichtel, W. R. (2016). Rapid removal of organic micropollutants from water by a porous β-cyclodextrin polymer. Nature 529, 190–194. doi: 10.1038/nature16185
Andreetti, G. D., Boehmer, V., Jordon, J. G., Tabatabai, M., Ugozzoli, F., Vogt, W., et al. (1993). Dissymmetric calix [4] arenes with C4-and C2-symmetry. Synthesis, X-ray structures, conformational fixation, and proton NMR spectroscopic studies. J. Org. Chem.58, 4023–4032. doi: 10.1021/jo00067a040
Avetta, C. T., Shorthill, B. J., Ren, C., and Glass, T. E. (2012). Molecular tubes for lipid sensing: tube conformations control analyte selectivity and fluorescent response. J. Org. Chem. 77, 851–857. doi: 10.1021/jo201791a
Baldini, L., Casnati, A., Sansone, F., and Ungaro, R. (2007). Calixarene-based multivalent ligands. Chem. Soc. Rev. 36, 254–266. doi: 10.1039/B603082N
Bier, D., Rose, R., Bravo-Rodriguez, K., Bartel, M., Ramirez-Anguita, J. M., Dutt, S., et al. (2013). Molecular tweezers modulate 14-3-3 protein–protein interactions. Nat. Chem. 5, 234–239. doi: 10.1038/nchem.1570
Chen, H., Fan, J., Hu, X., Ma, J., Wang, S., Li, J., et al. (2015). Biphen[n]arenes. Chem. Sci. 6, 197–202. doi: 10.1039/C4SC02422B
Chen, J., Ni, H., Meng, Z., Wang, J., Huang, X., Dong, Y., et al. (2019). Supramolecular trap for catching polyamines in cells as an anti-tumor strategy. Nat. Commun. 10:3546. doi: 10.1038/s41467-019-11553-7
Chun, Y., Singh, N. J., Hwang, I. C., Lee, J. W., Yu, S. U., and Kim, K. S. (2013). Calix [n] imidazolium as a new class of positively charged homo-calix compounds. Nat. Commun. 4:1797. doi: 10.1038/ncomms2758
Cram, D. J. (1988). The design of molecular hosts, guests, and their complexes. Science 240, 760–767. doi: 10.1126/science.3283937
Dai, L., Ding, Z. J., Cui, L., Li, J., Jia, X., and Li, C. (2017). 2, 2′-Biphen[n]arenes (n = 4–8): one-step, high-yield synthesis, and host–guest properties. Chem. Commun. 53, 12096–12099. doi: 10.1039/C7CC06767D
Gong, H. Y., Rambo, B. M., Karnas, E., Lynch, V. M., and Sessler, J. L. (2010). A ‘Texas-sized'molecular box that forms an anion-induced supramolecular necklace. Nat. Chem. 2, 406–409. doi: 10.1038/nchem.597
Goodman, C. M., Choi, S., Shandler, S., and DeGrado, W. F. (2007). Foldamers as versatile frameworks for the design and evolution of function. Nat. Chem. Biol. 3, 252–262. doi: 10.1038/nchembio876
Guo, D. S., and Liu, Y. (2012). Calixarene-based supramolecular polymerization in solution. Chem. Soc. Rev. 41, 5907–5921. doi: 10.1039/C2CS35075K
Guo, S., Song, Y., He, Y., Hu, X. Y., and Wang, L. (2018). Highly efficient artificial light-harvesting systems constructed in aqueous solution based on supramolecular self-assembly. Angew. Chem. Int. Ed. 57, 3163–3167. doi: 10.1002/anie.201800175
Jie, K., Zhou, Y., Li, E., and Huang, F. (2018). Nonporous adaptive crystals of pillararenes. Acc. Chem. Res. 51, 2064–2072. doi: 10.1021/acs.accounts.8b00255
Jono, K., Suzuki, A., Akita, M., Albrecht, K., Yamamoto, K., and Yoshizawa, M. (2017). A polyaromatic molecular clip that enables the binding of planar, tubular, and dendritic compounds. Angew. Chem. Int. Ed. 129, 3570–3574. doi: 10.1002/anie.201612489
Jurícek, M., Strutt, N. L., Barnes, J. C., Butterfield, A. M., Dale, E. J., Baldridge, K. K., et al. (2014). Induced-fit catalysis of corannulene bowl-to-bowl inversion. Nat. Chem. 6, 222–228. doi: 10.1038/nchem.1842
Lehn, J. M. (1988). Supramolecular chemistry—scope and perspectives molecules, supermolecules, and molecular devices (Nobel Lecture). Angew. Chem. Int. Ed. 27, 89–112. doi: 10.1002/anie.198800891
Li, B., Meng, Z., Li, Q., Huang, X., Kang, Z., Dong, H., et al. (2017). A pH responsive complexation-based drug delivery system for oxaliplatin. Chem. Sci. 8, 4458–4464. doi: 10.1039/c7sc01438d
Li, B., Wang, B., Huang, X., Dai, L., Cui, L., Li, J., et al. (2019). Terphen[n]arenes and quaterphen[n]arenes (n = 3–6): one-pot synthesis, self-assembly into supramolecular gels, and iodine capture. Angew. Chem. Int. Ed. 58, 3885–3889. doi: 10.1002/anie.201813972
Liu, Y., Zhao, W., Chen, C. H., and Flood, A. H. (2019). Chloride capture using a C–H hydrogen bonding cage. Science 365, 159–161. doi: 10.1126/science.aaw5145
Luo, J., Ao, Y. F., Wang, Q. Q., and Wang, D. X. (2018). Diversity-oriented construction and interconversion of multicavity supermacrocycles for cooperative anion–π binding. Angew. Chem. Int. Ed. 57, 15827–15831. doi: 10.1002/anie.201810836
Ma, D., Hettiarachchi, G., Nguyen, D., Zhang, B., Wittenberg, J. B., Zavalij, P. Y., et al. (2012). Acyclic cucurbit [n] uril molecular containers enhance the solubility and bioactivity of poorly soluble pharmaceuticals. Nat. Chem. 4, 503–510. doi: 10.1038/nchem.1326
Ma, Y. L., Ke, H., Valkonen, A., Rissanen, K., and Jiang, W. (2018). Achieving strong positive cooperativity through activating weak non-covalent interactions. Angew. Chem. Int. Ed. 57, 709–713. doi: 10.1002/anie.201711077
Ogoshi, T., Kanai, S., Fujinami, S., Yamagishi, T. A., and Nakamoto, Y. (2008). para-Bridged symmetrical pillar [5] arenes: their Lewis acid catalyzed synthesis and host–guest property. J. Am. Chem. Soc. 130, 5022–5023. doi: 10.1021/ja711260m
Pan, S. J., Ye, G., Jia, F., He, Z., Ke, H., Yao, H., et al. (2017). Regioselective synthesis of methylene-bridged naphthalene oligomers and their host–guest chemistry. J. Org. Chem. 82, 9570–9575. doi: 10.1021/acs.joc.7b01579
Pedersen, C. J. (1988). The discovery of crown ethers. Science 241, 536–540. doi: 10.1126/science.241.4865.536
Poh, B. L., Lim, C. S., and Khoo, K. S. (1989). A water-soluble cyclic tetramer from reacting chromotropic acid with formaldehyde. Tetrahedron Lett. 30, 1005–1008. doi: 10.1016/S0040-4039(00)95302-4
Schrader, T., Bitan, G., and Klärner, F. G. (2016). Molecular tweezers for lysine and arginine–powerful inhibitors of pathologic protein aggregation. Chem. Commun. 52, 11318–11334. doi: 10.1039/C6CC04640A
Seebach, D., and Gardiner, J. (2008). β-peptidic peptidomimetics. Acc. Chem. Res. 41, 1366–1375. doi: 10.1021/ar700263g
Shen, C., Ma, D., Meany, B., Isaacs, L., and Wang, Y. (2012). Acyclic cucurbit [n] uril molecular containers selectively solubilize single–walled carbon nanotubes in water. J. Am. Chem. Soc. 134, 7254–7257. doi: 10.1021/ja301462e
Shorthill, B. J., Avetta, C. T., and Glass, T. E. (2004). Shape-selective sensing of lipids in aqueous solution by a designed fluorescent molecular tube. J. Am. Chem. Soc. 126, 12732–12733. doi: 10.1021/ja047639d
Sinha, S., Lopes, D. H., Du, Z., Pang, E. S., Shanmugam, A., Lomakin, A., et al. (2011). Lysine-specific molecular tweezers are broad-spectrum inhibitors of assembly and toxicity of amyloid proteins. J. Am. Chem. Soc. 133, 16958–16969. doi: 10.1021/ja206279b
Song, N., and Yang, Y. W. (2015). Molecular and supramolecular switches on mesoporous silica nanoparticles. Chem. Soc. Rev. 44, 3474–3504. doi: 10.1039/C5CS00243E
Wang, M. X. (2018). Coronarenes: recent advances and perspectives on macrocyclic and supramolecular chemistry. Sci. China Chem. 61, 993–1003. doi: 10.1007/s11426-018-9328-8
Wang, Y., Liu, T., Jiang, J., Chen, Y., Cen, M., Lu, D., et al. (2019a). Syntheses of water-soluble acyclic naphthalene oligomers and their applications in water. Dalton Trans. 48, 6333–6336. doi: 10.1039/C9DT00709A
Wang, Y., Ping, G., and Li, C. (2016). Efficient complexation between pillar [5] arenes and neutral guests: from host–guest chemistry to functional materials. Chem. Commun. 52, 9858–9872. doi: 10.1039/C6CC03999E
Wang, Y., Xu, K., Li, B., Cui, L., Li, J., Jia, X., et al. (2019b). Efficient separation of cis-and trans-1, 2-dichloroethene isomers by adaptive biphen[3]arene crystals. Angew. Chem. Int. Ed. 58, 10281–10284. doi: 10.1002/anie.201905563
Xue, M. I. N., Yang, Y., Chi, X., Zhang, Z., and Huang, F. (2012). Pillararenes, a new class of macrocycles for supramolecular chemistry. Acc. Chem. Res. 45, 1294–1308. doi: 10.1021/ar2003418
Yang, B., Zhang, X. D., Li, J., Tian, J., Wu, Y. P., Yu, F. X., et al. (2019). In situ loading and delivery of short single-and double-stranded dna by supramolecular organic frameworks. CCS Chem. 1, 156–165 doi: 10.31635/ccschem.019.20180011
Yang, K., Pei, Y., Wen, J., and Pei, Z. (2016). Recent advances in pillar [n] arenes: synthesis and applications based on host–guest interactions. Chem. Commun. 52, 9316–9326. doi: 10.1039/C6CC03641D
Yashima, E., Ousaka, N., Taura, D., Shimomura, K., Ikai, T., and Maeda, K. (2016). Supramolecular helical systems: helical assemblies of small molecules, foldamers, and polymers with chiral amplification and their functions. Chem. Rev. 116, 13752–13990. doi: 10.1021/acs.chemrev.6b00354
Zhang, D. W., Zhao, X., Hou, J. L., and Li, Z. T. (2012). Aromatic amide foldamers: structures, properties, and functions. Chem. Rev. 112, 5271–5316. doi: 10.1021/cr300116k
Keywords: pillararenes, calixarenes, acyclic hosts, molecular recognition, host-guest chemistry
Citation: Jia Y, Dong M, Wang B and Li C (2019) Synthesis and Host–Guest Properties of Acyclic Pillar[n]naphthalenes. Front. Chem. 7:828. doi: 10.3389/fchem.2019.00828
Received: 15 August 2019; Accepted: 14 November 2019;
Published: 03 December 2019.
Edited by:
De-Xian Wang, Institute of Chemistry (CAS), ChinaReviewed by:
Lyle Isaacs, University of Maryland, College Park, United StatesLiu-Pan Yang, Southern University of Science and Technology, China
Copyright © 2019 Jia, Dong, Wang and Li. This is an open-access article distributed under the terms of the Creative Commons Attribution License (CC BY). The use, distribution or reproduction in other forums is permitted, provided the original author(s) and the copyright owner(s) are credited and that the original publication in this journal is cited, in accordance with accepted academic practice. No use, distribution or reproduction is permitted which does not comply with these terms.
*Correspondence: Bin Wang, aHh4eXdhbmdiQG1haWwudGpudS5lZHUuY24=; Chunju Li, Y2psaUBzaHUuZWR1LmNu
†These authors have contributed equally to this work