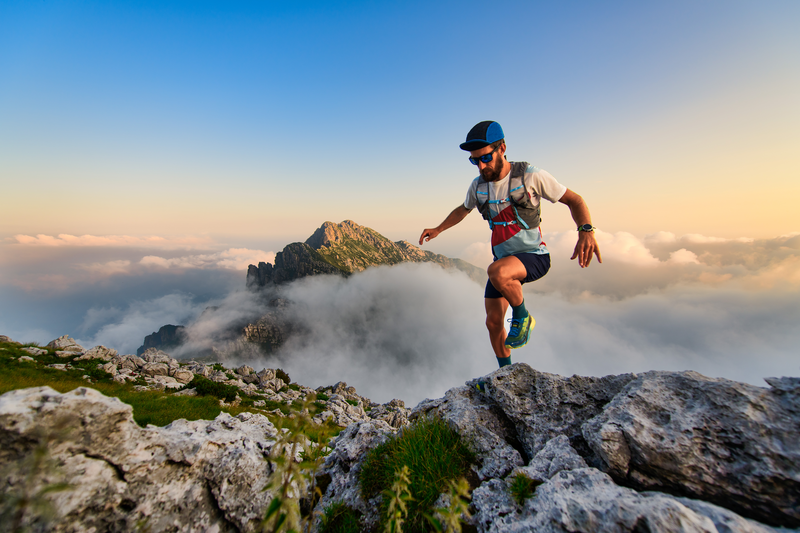
94% of researchers rate our articles as excellent or good
Learn more about the work of our research integrity team to safeguard the quality of each article we publish.
Find out more
ORIGINAL RESEARCH article
Front. Chem. , 26 November 2019
Sec. Organic Chemistry
Volume 7 - 2019 | https://doi.org/10.3389/fchem.2019.00814
A new microwave method (MM) has been developed for the synthesis of a series of 16 substituted ferrocenyl chalcones using acetylferrocene (1) with different aldehydes (2a-2p) and comparing it with conventional method (CM). The synthesized compounds were characterized by various spectroscopic techniques viz IR, HR-MS, 1H NMR, and 13C NMR. The time required for completion of reaction in MM varied from 1 to 5 min as compared to CM which required 10–40 h. All the synthesized compounds were screened for antifungal activity against Sclerotium rolfsii and Alternaria solani. In vitro fungicidal activity revealed that compound 3o (ED50 = 23.24 mg L−1) was found to be most active against S. rolfsii. But in case of A. solani, compound 3c (ED50 = 29.9 mg L−1) showed highest activity. The nematicidal activity revealed that the compound 3b was more potent with LC50 values of 10.67, 7.30, and 4.55 ppm at 24, 48, and 72 h, respectively. 2D-Quantitative Structural Activity Relationship (2D-QSAR) analysis of these ferrocenyl chalcones was carried out by developing three different models namely Partial Least Squares (PLS, Model 1), Multiple Linear Regression (MLR, Model 2) and Principal Component Regression (PCR, Model 3). Statistical significance and predictive ability of these models were assessed by internal and external validation and also verified by leave one-out cross-validation. QSAR study revealed that MLR for S. rolfsii (r2 = 0.999, q2 = 0.996), PLS for A. solani (r2 = 0.934, q2 = 0.749) and PCR for M. incognita (r2 = 0.878, q2 = 0.772) were the best model. The physico-chemical parameters were calculated using VLife MDS 4.6 software. QSAR study could be employed for structure optimization to achieve better activity.
Chalcones and their corresponding heterocyclic analogs were reported to exhibit several biological activities such as antibacterial, antifungal, insecticidal, nematicidal, anti-oxidant, antiplasmodial, antitumor, and anthelmintic due to occurrence of highly reactive unsaturated carbonyl moiety in skeleton (Vibhute and Baseer, 2003; Alam, 2004; Bag et al., 2009; Kalirajan et al., 2009; Shakil et al., 2010, 2011, 2013; Caboni et al., 2016). Although chalcones do exhibit promising activity against various pests, but have not been explored in agricultural practices to that extent due to their poor water solubility, which can be overcome by making suitable formulations. However, to develop formulations, there is a need to incorporate auxiliaries, adjuvants, other materials and also the process of developing formulation is cumbersome as well as costly to end users.
Ferrocene based organometallic compounds are important because of their interference to the biological system. Due to greater stability, easy availability of derivatives, and favorable electrochemical properties, ferrocene derivatives have been used prominently (Nolte and Salmain, 2008). Wu et al. (2002) synthesized ferrochloroquine, which is the ferrocenyl analog of chloroquine and reported its antimalarial activity against Plasmodium falciparum.
Ferrochloroquine
In recent time, microwave heating has taken an incontestable place in analytical and organic laboratories practice as a very effective and non-polluting method of activation (Arends et al., 1997). Synthesis through microwave, has several advantages viz., shorter reaction times, higher yields, ease of manipulation, and lower costs. Rate enhancement of organic reactions is due to the superheating of the solvent (Gedye et al., 1986; Mayo et al., 2002).
The early blight disease caused by Alternaria solani is most threatening and severely influenced the tomato productivity across the world and accounts for 35–78% loss in fruit yield (Grigolli et al., 2011; Song et al., 2011). Another widely distributed, soil-borne fungal pathogen, affecting a large number of horticultural, and agricultural crops is Sclerotium rolfsii. In tomato, they cause tomato blight resulting in damping-off and collar rot (Abeysinghe, 2009).
Plant parasitic nematodes are most important soil borne pests that cause damage to almost all species of crop plant. Meloidogyne spp. (Root-knot nematodes) are the most destructive pathogens in terms of yield loss due to their broad host range i.e., vegetables, cereals, pulses, etc. (Abad et al., 2008). M. incognita is one of the important pest of vegetables belonging to Solanaceous crop (brinjal, tomato, chili etc.). They have been reported to cause extensive damage to the tune of 20–32% (Jain et al., 2007).
Chalcones and their derivatives are conventionally known to possess biological activity, but their poor water solubility hamper their utility, both in pharmaceuticals and agricultural field. Through this study, efforts have been made to improve the solubility/bioavailability of substituted chalcones by the introduction of ferrocene group. Also, new microwave method was developed for synthesizing these molecules in reduced time as compared to conventional method. Synthesized ferrocenyl chalcones have been evaluated for their fungicidal and nematicidal activity against S. rolfsii, A. solani, and M. incognita. QSAR analysis was carried out to recognize the molecular properties which effect the fungicidal and nematicidal activities the most.
Acetylferrocene and different benzaldehydes were procured from Aldrich. All other solvents and chemicals were of analytical grade and were used as received unless otherwise noted. Reactions were observed by thin layer chromatography (TLC) on pre-coated Merck silica gel 60F254, 200 mm thick aluminum sheets and the spots were visualized under UV-light. Reverse-phase UFLC SHIMADZU, C-18 Shim-pack column (5 μm, 4.6 × 250 mm) with PDA detector using isocratic solvent system (methanol-water: 98:2) was used for determining the purity of synthesized ferrocenyl chalcones. 1H-NMR and 13C-NMR studies were carried out on JEOL 400 MHz Spectrospin spectrometer instrument, data were processed using Delta software and tetramethylsilane (TMS) was used as internal standard.
High Resolution Mass Spectrometry (HRMS) was performed by AB SCIEX Triple TOF™ 5,600+ equipped with TurboIonSpray (TIS), SCIEX ExionLC, and PDA detector. C-18 column (2.7 μm, 4.6 × 100 mm) was used for separation of compounds. The column was eluted with methanol and water (98:2, v/v) with 0.1% formic acid at a flow rate of 0.3 mL/min. The column oven temperature was set at 40°C. Infrared (IR) spectra were recorded on Bruker alpha FT-IR spectrophotometer, values were expressed as υmax cm−1. Melting point apparatus was used for determination of melting points. ED50 and LC50 values were calculated by SPSS statistical package. All computational activities were performed by using Vlife MDS QSAR plus 4.6 software in ASUS VivoBook (windows 10 OS and Intel Core i5).
The synthesis of the ferrocenyl chalcones series was done by the base-catalyzed Claisen-Schmidt reaction according to the method reported in literature. The general method of synthesis is reproduced below (Wu et al., 2002).
A series of ferrocenyl chalcones [except 3-hydroxybenzaldehyde (2i), 4-hydroxybenzaldehyde (2p)] was synthesized as follows: Acetylferrocene (684 mg, 3 mmol) and KOH (0.2 g) were dissolved in ethanol (5 mL) in a round bottom flask and stirred at room temperature for 10 min, followed by drop wise addition of ethanolic solution of equimolar amounts of different benzaldehydes (2a-2o), (3 mmol, 5 mL) with continuous stirring. The stirring ranged between 1 and 40 h for different reactions at room temperature (Scheme 1). The reaction was monitored by TLC in ethyl acetate: hexane, (1:4 solvent systems). After completion of reaction, the reaction mixture was neutralized with 2 M HCl to get the dark red/red/orange/chocolate precipitate. The precipitate was separated by filtration and washed with cold water. In case of absence of precipitate, the solution was extracted with ethyl acetate (30 mL × 3). The organic layer was dried with anhydrous Na2SO4 and solvent removed using rotary evaporator to give a viscous residue. The crude product was purified by column chromatography on silica gel using hexane-ethyl acetate as eluent of increasing polarity. The desired compound was eluted in 10% ethyl acetate: hexane. Characterization of all compounds was done by IR, HR-MS, 1H-NMR, and 13C-NMR (Supplementary Data Sheets 1–3).
Scheme 1. General method for the synthesis of ferrocenyl (Wu et al., 2002).
3i and 3p were synthesized as follows: The hydroxy derivatives of benzaldehydes (2i and 2p) (366 mg, 3 mmol), 2H-3, 4-dihydropyran (8 mmol), and pyridinium p-toluenesulfonate (0.2 mmol) were taken in DCM (10 mL) and kept for stirring at room temperature for 4 h (Scheme 2). After completion of reaction, crude tetrahydropyranyl ether was obtained as yellow solid by washing the reaction mixture with Na2CO3 (1 M, 20 mL × 2), followed by removal of solvent under vacuum. These protected benzaldehydes (2i and 2p) were then treated with acetylferrocene as per the method described above to get the corresponding ferrocenyl chalcones.
Scheme 2. Method for the synthesis of 3p, ferrocenyl (Wu et al., 2002).
Equimolar amounts of acetylferrocene (100 mg, 0.438 mmol) in 5% ethanolic KOH (5 mL) with minimum ethanolic solution of the different benzaldehydes were added in microwave vial (10 mL). The vial was placed in microwave synthesis reactor (Anton Paar, Monowave 300) with the conditions; Temp. 100°C, rpm-600, and time 1–5 min. Monitoring of reaction was carried out by TLC in ethyl acetate: hexane (1:4 solvent systems). Reaction mixture was worked up as described above.
It was obtained as a red colored solid in 81% yield; m.p.:140–142°C, Rf: 0.50 (ethyl acetate: hexane, 1:4). IR (cm−1): 1,645 (CO), 1,593 (C = C). 1H-NMR (400 MHz, ACN-d3): δ 4.22 (5H, s, H-6″), 4.63 (2H, s, H-3″ & H-4″), 4.94 (2H, s, H-2″ & H-5″), 7.29 (1H, d, J = 16, H-α), 7.45 (3H, m, H-3′, H-4′ & H-5′), 7.67 (1H, d, J = 16, H-β), 7.76 (2H, d, J = 8, H-2′ & H-6′).13C- NMR (400 MHz, DMSO-d6): δ 69.65 (C-2″ & C-5″), 69.78 (C-6″), 72.74 (C-3″ & C-4″), 80.66 (C-1″), 123.64 (C-α), 128.61 (C-3′ & C-5′), 128.89 (C-2′ & C-6′), 130.11 (C-4′), 134.90 (C-1′), 139.75 (C-β), 191.96 (CO). HR-MS for C19H16FeO [M+H]+ m/z: Calcd 317.0623; Observed 317.0639.
It was obtained as an orange colored solid in 87% yield; m.p.:160–162°C, Rf: 0.42 (ethyl acetate: hexane, 1:4). IR (cm−1): 1,649 (CO), 1,591 (C = C). 1H-NMR (400 MHz, ACN-d3): δ 4.22 (5H, s, H-6″), 4.64 (2H, s, H-3″ & H-4″), 4.93 (2H, s, H-2″ & H-5″), 7.28 (1H, d, J = 16, H-α), 7.61 (2H, d, J = 8, H-2′ & H-6′), 7.63 (1H, d, J = 12, H-β), 7.68 (2H, d, J = 8, H-3′ & H-5′). 13C-NMR (400 MHz, DMSO-d6): δ 69.70 (C-2″ & C-5″), 69.80 (C-6″), 72.83 (C-3″ & C-4″), 80.59 (C-1″), 123.45 (C-α), 124.43(C-4′), 130.55 (C-2′ & C-6′), 131.82 (C-3′ & C-5′), 134.24 (C-1′), 138.41 (C-β), 191.88 (CO). HR-MS for C19H15BrFeO [M+H]+ m/z: Calcd 394.9728; Observed 394.9739.
It was obtained as a red colored solid in 79% yield; m.p.:138–140°C, Rf: 0.43 (ethyl acetate: hexane, 1:4). IR (cm−1): 1,649 (CO), 1,589 (C = C). 1H-NMR (400 MHz, ACN-d3): δ 4.22 (5H, s, H-6″), 4.63 (2H, s, H-3″ & H-4″), 4.93 (2H, s, H-2″ & H-5″), 7.20 (2H, d, J = 8, H-3′ & H-5′), 7.22 (1H, d, J = 16, H-α), 7.65 (1H, d, J = 16, H-β), 7.81 (2H, d, J = 8, H-2′ & H-6′).13C-NMR (400 MHz, DMSO-d6): δ 69.28 (C-2″ & C-5″), 69.99 (C-6″), 72.95 (C-3″ & C-4″), 80.87 (C-1″), 115.62 (C-3′ & C-5′), 123.79 (C-α), 130.60 (C-2′ & C-6′), 131.07 (C-1′), 138.34 (C-β), 163.64 (C-4′),192.45 (CO). HR-MS for C19H15FFeO [M+H]+ m/z: Calcd 335.0529; Observed 317.0544.
It was obtained as a chocolate colored solid in 80% yield; m.p.:125–126°C, Rf: 0.60 (ethyl acetate: hexane, 1:4). IR (cm−1): 1,653 (CO), 1,591 (C = C). 1H-NMR (400 MHz, ACN-d3): δ 4.19 (5H, s, H-6″), 4.52 (2H, s, H-3″ & H-4″), 4.62 (2H, s, H-2″ & H-5″), 6.64 (1H, d, J = 16, H-α), 7.24 (1H, d, J = 16, H-β), 7.42, 7.47, 7.51, 7.53 (4H, m, H-3′, H-4′, H-5′, & H-6′).13C-NMR (400 MHz, DMSO-d6): δ 68.24 (C-2″ & C-5″), 69.12 (C-6″), 72.99 (C-3″ & C-4″), 80.35 (C-1″), 124.22 (C-α), 125.89 (C-5′), 127.20 (C-6′), 128.56 (C-3′), 130.6 (C-4′), 133.73 (C-2′), 136.61 (C-1′), 139.85 (C-β), 192.87 (CO). HR-MS for C19H15ClFeO [M+H]+ m/z: Calcd 351.0233; Observed 351.0231.
It was obtained as a red colored solid in 83% yield; m.p.:120–122°C, Rf: 0.50 (ethyl acetate: hexane, 1:4). IR (cm−1): 1,653 (CO), 1,595 (C = C). 1H-NMR (400 MHz, ACN-d3): δ 4.24 (5H, s, H-6″), 4.66 (2H, s, H-3″ & H-4″), 4.94 (2H, s, H-2″ & H-5″), 7.24 (1H, d, J = 16, H-α), 7.31 (1H, t, H-4′), 7.44 (1H, t, H-5′), 7.69 (1H, d, J = 8, H-6′), 7.96 (1H, d, J = 4, H-3′), 7.97 (1H, d, J = 16, H-β).13C-NMR (400 MHz, DMSO-d6): δ 69.71 (C-2″ & C-5″), 69.86 (C-6″), 72.99 (C-3″ & C-4″), 80.35 (C-1″), 125.09 (C-2′), 126.51 (C-α), 128.20 (C-5′), 128.56 (C-4′), 131.66 (C-6′), 133.23 (C-3′), 134.21 (C-1′)137.14 (C-β), 191.70 (CO). HR-MS for C19H15BrFeO [M+H]+ m/z: Calcd 394.9728; Observed 394.9724.
It was obtained as an orange colored solid in 82% yield; m.p.:150–152°C, Rf: 0.28 (ethyl acetate: hexane, 1:4). IR (cm−1): 1,645 (CO), 1,585 (C = C). 1H-NMR (400 MHz, ACN-d3): δ 3.84 (3H, s, 4′, Ar-OCH3), 4.21 (5H, s, H-6″), 4.60 (2H, s, H-3″ & H-4″), 4.92 (2H, s, H-2″ & H-5″), 6.99 (2H, d, J = 8, H-3′ & H-5′), 7.17 (1H, d, J = 16, H-α), 7.63(1H, d, J = 16, H-β), 7.72 (2H, d, J = 8, H-2′ & H-6′).13C-NMR (400 MHz, DMSO-d6): δ 55.34 (4′, Ar-OCH3), 69.54 (C-2″ & C-5″), 69.70 (C-6″), 72.48 (C-3″ & C-4″), 80.87 (C-1″), 114.35 (C-3′ & C-5′), 121.26 (C-α), 127.56 (C-1′), 130.37 (C-2′ & C-6′), 139.65 (C-β), 160.92 (C-4′), 191.90 (CO). HR-MS for C20H18FeO2 [M+H]+ m/z: Calcd 347.0729; Observed 347.0749.
It was obtained as a chocolate colored solid in 78% yield; m.p.:140–142°C, Rf: 0.40 (ethyl acetate: hexane, 1:4). IR (cm−1): 1,639 (CO), 1,562 (C = C). 1H-NMR (400 MHz, ACN-d3): δ 3.01 (6H, s, 4′, Ar-N(CH3)2), 4.19 (5H, s, H-6″), 4.57 (2H, s, H-3″ & H-4″), 4.90 (2H, s, H-2″ & H-5″), 6.76 (2H, d, J = 8, H-3′ & H-5′), 7.08 (1H, d, J = 16, H-α), 7.58 (2H, d, J = 8, H-2′ & H-6′), 7.61 (1H, d, J = 16, H-β).13C-NMR (400 MHz, DMSO-d6): δ 40.65 (4′, Ar-N(CH3)2), 69. 68 (C-2″ & C-5″), 69.97 (C-6″), 72.28 (C-3″ & C-4″), 81.05 (C-1″), 111.79 (C-3′ & C-5′), 117.80 (C-α), 122.62 (C-1′), 130.17 (C-2′ & C-6′), 141.24 (C-β), 151.72 (C-4′), 192.92 (CO). HR-MS for C21H21FeNO [M+H]+ m/z: Calcd 360.1045; Observed 360.1049.
It was obtained as a chocolate colored solid in 80% yield; m.p.:145–146°C, Rf: 0.30 (ethyl acetate: hexane, 1:4). IR (cm−1): 1,643 (CO), 1,579 (C = C). 1H-NMR (400 MHz, ACN-d3): δ 3.77 (3H, s, 4′, Ar-OCH3), 3.90 (6H, s, 3′&5′, Ar-OCH3), 4.22 (5H, s, H-6″), 4.63 (2H, s, H-3″ & H-4″), 4.97 (2H, s, H-2″ & H-5″), 7.05 (2H, s, H-2′& H-6′), 7.21 (1H, d, J = 16, H-α), 7.60 (1H, d, J = 16, H-β). 13C-NMR (400 MHz, DMSO-d6): δ 55.31 (3′&5′, Ar-OCH3), 60.10 (4′, Ar-OCH3), 69.60(C-2″ & C-5″), 69.76(C-6″), 72.59 (C-3″ & C-4″), 80.95 (C-1″), 106.17 (C-2′ & C-6′), 122.82 (C-α), 129.56 (C-1′), 130.37 (C-4′), 140.21 (C-β), 153.07 (C-3′ & C-5′), 191.79 (CO). HR-MS for C22H22FeO4 [M+H]+ m/z:Calcd 407.0940; Observed 407.0931.
It was obtained as a dark red colored solid in 81% yield; m.p.:120–122°C, Rf: 0.37 (ethyl acetate: hexane, 1:4). IR (cm−1): 1,647 (CO), 1,585 (C = C). 1H-NMR (400 MHz, ACN-d3): δ 4.22 (5H, s, H-6″), 4.63 (2H, s, H-3″ & H-4″), 4.94 (2H, s, H-2″ & H-5″), 5.53 (1H, s, 3′, Ar-OH), 7.29 (1H, d, J = 16, H-α), 7.38 (4H, m, H-2′ H-4′, H-5′ & H-6′), 7.63 (1H, d, J = 16, H-β).13C-NMR (400 MHz, DMSO-d6): δ 69.51 (C-2″ & C-5″), 69.77 (C-6″), 72.74 (C-3″ & C-4″), 80.28 (C-1″), 116.21 (C-2′), 118.05 (C-4′), 122.21 (C-6′), 123.91 (C-α), 129.86 (C-5′), 136.15(C-1′), 139.62 (C-β), 157.17 (C-3′), 192.80 (CO). HR-MS for C19H16FeO2 [M+H]+ m/z: Calcd 333.0572; Observed 333.0588.
It was obtained as an orange colored solid in 82% yield; m.p.:140–142°C, Rf: 0.34 (ethyl acetate: hexane, 1:4). IR (cm−1): 1,645 (CO), 1,579 (C = C). 1H-NMR (400 MHz, ACN-d3): δ 4.21 (5H, s, H-6″), 4.60 (2H, s, H-3″ & H-4″), 4.92 (2H, s, H-2″ & H-5″), 5.16 (2H, s, 4′, Ar-OCH2−C6H5), 7.06 (2H, d, J = 20, H-3′ & H-5′), 7.16 (1H, d, J = 16, H-α), 7.41 (5H, s, Ar-OCH2−C6H5), 7.46 (2H, d, H-2′ & H-6′), 7.66 (1H, d, J = 16, H-β).13C-NMR (400 MHz, DMSO-d6): δ 69.53 (C-2″ & C-5″), 69.69 (C-6″), 72.48 (C-3″ & C-4″), 77.45 (4′, Ar-OCH2-Ar), 80.85 (C-1″), 115.19 (C-3′ & C-5′), 121.37 (C-α), 127.73 (C-1′, C-2′ & C-6′), 127.90 (4′, Ar-OCH2-Ar, C-2″ ′ & C-6″ ′), 128.44 (4′, Ar-OCH2-Ar, C-4″′), 130.36 (4′, Ar-OCH2-Ar, C-3″′ & C-5″′), 136.59 (4′, Ar-OCH2-Ar, C-1″′), 139.57 (C-β), 159.89 (C-4′), 191.94 (CO). HR-MS for C26H22FeO2 [M+H]+ m/z: Calcd 423.1042; Observed 423.1045.
It was obtained as dark viscous liquid in 71% yield; Rf: 0.25 (ethyl acetate: hexane, 1:4). IR (cm−1): 1,648 (CO), 1,594 (C = C). 1H-NMR (400 MHz, ACN-d3): δ 4.24 (5H, s, H-6″), 4.55 (2H, s, H-3″ & H-4″), 4.94 (2H, s, H-2″ & H-5″), 7.24 (1H, d, J = 16, H-α), 8.01 (1H, d, J = 16, H-β), 7.77 (3H, m, H-4′, H-5′ H-4′ & H-6′), 8.04 (1H, d, J = 12, H-3′). 13C-NMR (400 MHz, DMSO-d6): δ 69.64 (C-2″ & C-5″), 69.95 (C-6″), 72.91 (C-3″ & C-4″), 80.88 (C-1″), 125.68 (C-α), 126.81 (C-3′), 128.62 (C-6′), 129.98 (C-5′), 131.79 (C-1′), 134.12 (C-4′), 137.21 (C-β), 148.98 (C-2′), 192.25 (CO). HR-MS for C19H15FeNO3 [M+H]+ m/z: Calcd 362.0474; Observed 362.0476.
It was obtained as a red colored solid in 80% yield; m.p.:175–176°C, Rf: 0.31 (ethyl acetate: hexane, 1:4). IR (cm−1): 1,651 (CO), 1,595 (C = C). 1H-NMR (400 MHz, ACN-d3): δ 4.23 (5H, s, H-6″), 4.66 (2H, s, H-3″ & H-4″), 4.97 (2H, s, H-2″ & H-5″), 7.43 (1H, d, J = 16, H-α), 7.68–7.74 (2H, m, H-5′ & H-6′), 8.13 (1H, d, H-4′). 8.24 (1H, d, J = 16, H-β), 8.60 (1H, s, H-2′). 13C-NMR (400 MHz, DMSO-d6): δ 69.53 (C-2″ & C-5″), 69.82 (C-6″), 72.97 (C-3″ & C-4″), 80.41 (C-1″), 122.81 (C-2′), 124.21 (C-4′), 126.28 (C-α), 130.28 (C-5′), 134.79 (C-6′), 136.88 (C-1′), 137.38 (C-β), 148.40 (C-3′), 191.81 (CO). HR-MS for C19H15FeNO3 [M+H]+ m/z: Calcd 362.0474; Observed 362.0480.
It was obtained as a violet colored solid in 82% yield; m.p.:180–182°C, Rf: 0.33 (ethyl acetate: hexane, 1:4). IR (cm−1): 1,653 (CO), 1,597 (C = C). 1H-NMR (400 MHz, ACN-d3): δ 4.24 (5H, s, H-6″), 4.68 (2H, s, H-3″ & H-4″), 4.96 (2H, s, H-2″ & H-5″), 7.39 (1H, d, J = 16, H-α), 7.72 (1H, d, J = 16, H-β), 7.97 (2H, d, J = 8, H-2′ & H-6′), 8.27 (2H, d, J = 8, H-3′ & H-5′). 13C-NMR (400 MHz, DMSO-d6): δ 69.48 (C-2″ & C-5″), 69.85 (C-6″), 72.98 (C-3″ & C-4″), 80.67 (C-1″), 123.77 (C-3′ & C-5′), 125.72 (C-α), 129.20 (C-2′ & C-6′), 136.91 (C-β), 141.21 (C-1′), 148.67 (C-4′),192.41 (CO). HR-MS for C19H15FeNO3 [M+H]+ m/z: Calcd 362.0474; Observed 362.0478.
It was obtained as dark viscous liquid in 76% yield; Rf: 0.60 (ethyl acetate: hexane, 1:4). IR (cm−1): 1,651 (CO), 1,584 (C = C). 1H-NMR (400 MHz, ACN-d3): δ 4.24 (5H, s, H-6″), 4.66 (2H, s, H-3″ & H-4″), 4.88 (2H, s, H-2″ & H-5″), 7.29 (1H, d, J = 16, H-α), 7.31 (1H, d, J = 8, H-5′), 7.33 (1H, d, J = 8, H-6′), 7.49 (1H, s, H-3′), 7.66 (1H, d, J = 16, H-β).13C-NMR (400 MHz, DMSO-d6): δ 69.46 (C-2″ & C-5″), 69.85 (C-6″), 73.23 (C-3″ & C-4″), 79.83 (C-1″), 123.28 (C-α), 128.75 (C-5′), 130.62 (C-6′), 132.05 (C-3′), 132.34 (C-2′), 132.86 (C-1′), 133.78 (C-4′), 139.36 (C-β), 191.17 (CO). HR-MS for C19H14Cl2FeO [M+H]+ m/z: Calcd 384.9843; Observed 384.9813.
It was obtained as dark viscous liquid in 83% yield; Rf: 0.60 (ethyl acetate: hexane, 1:4). IR (cm−1): 1,654 (CO), 1,586 (C = C). 1H-NMR (400 MHz, ACN-d3): δ 4.23 (5H, s), 4.66 (2H, s, H-3″ & H-4″), 4.93 (2H, s, H-2″ & H-5″), 7.45 (1H, d, J = 16, H-α), 7.21–7.35 (1H, m, H-4′), 7.66 (1H, d, J = 16, H-β),7.94–7.98 (2H, m, H-3′& H-5′).13C-NMR (400 MHz, DMSO-d6): δ 69.72 (C-2″ & C-5″), 70.00 (C-6″), 73.14 (C-3″ & C-4″), 80.35 (C-1″), 124.24 (C-α), 128.07 (C-3′& C-5′), 129.03 (C-4′), 132.51 (C-2′ & C-6′), 135.22 (C-1′), 139.48 (C-β), 192.35 (CO). HR-MS for C19H14Cl2FeO [M+H]+ m/z: Calcd 384.9843; Observed 384.9856.
It was obtained as an orange colored solid in 80% yield; m.p.:115–120°C, Rf: 0.45 (ethyl acetate: hexane, 1:4). IR (cm−1): 1,645 (CO), 1,571 (C = C). 1H-NMR (400 MHz, ACN-d3): δ 4.21 (5H, s, H-6″), 4.60 (2H, s, H-3″ & H-4″), 4.92 (2H, s, H-2″ & H-5″), 5.52 (1H, s, 4′, Ar-OH), 7.08 (2H, d, J = 8, H-3′ & H-5′), 7.17 (1H, d, J = 16, H-α), 7.63 (1H, d, J = 16, H-β), 7.70 (2H, d, J = 8, H-2′ & H-6′).13C-NMR (400 MHz, DMSO-d6): δ 69.59 (C-2″ & C-5″), 69.89 (C-6″), 72.28 (C-3″ & C-4″), 80.37 (C-1″), 116.35 (C-3′ & C-5′), 120.78 (C-α), 127.06 (C-1′), 127.37 (C-2′ & C-6′), 139.80 (C-β), 156.92 (C-4′), 192.37 (CO). HR-MS for C19H16FeO2 [M+H]+ m/z: Calcd 333.0572; Observed 333.0579.
Two fungi, A. solani ITCC 4632 and S. rolfsii ITCC 6474 were procured from Indian Type Culture Collection (ITCC) center, Division of Plant Pathology, ICAR-Indian Agricultural Research Institute, New Delhi-110012, India and kept at 27°C for at least 4–7 days on Potato Dextrose Agar (PDA) slant. Sub-culturing was done in petriplates and before doing the antifungal activity.
In vitro antifungal activity of the all synthesized compounds was carried out on PDA medium by using poisoned food technique (Nene and Thapliyal, 1979). A stock solution of 10,000 ppm of each compound was prepared in DMSO (Dimethylsuphoxide). Five test concentrations viz. 100, 50, 25, 12.5, and 6.25 mg L−1 were prepared from stock solution by serial dilution. The commercial fungicides, Hexaconazole 5% SC (S. rolfsii) and Mancozeb 75% WP (A. solani) were taken as positive control. Percentage inhibition was calculated by Abott's formula (Abbott, 1925).
Corrected % inhibition (IC) was calculated by given formula
Where, I = Percentage inhibition, CF = (90-C)/C × 100, 90 is the diameter (mm) of the petriplate and C is the growth of the fungus (mm) in control.
ED50 (mg L−1) values (Effective Dose for 50% inhibition) were calculated using SPSS statistical Package (v16.0).
In vitro nematicidal activity of the all synthesized compounds was carried by water screening method. The root galls were obtained from M. incognita infected plants collected from the glass house of Division of Nematology, ICAR-IARI, New Delhi and were incubated for 2–3 days at 25–30°C for hatching of eggs. After hatching, the number of J2s per 1 mL of aliquot were calculated and diluted to 100 J2s per mL. Synthesized ferrocenyl chalcones (12 mg) were weighed separately and dissolved in 0.5 mL of DMSO and 24.5 mL distilled water with Tween 80 (3.0%) to get a stock solution of 500 ppm. Five test concentrations viz. 125, 62.5, 31.25 15.62, and 7.81 mg L−1 were prepared from stock solution by serial dilution. 1 mL of aliquot (100 J2s/mL) was taken in 24 well culture plate to which 1 mL of test concentrations was added (Figure 3). All treatments were carried out in triplicates separately for 24, 48, and 72 h. Mortality was recorded after 24, 48, and 72 h using a stereoscopic binocular microscope. The percent mortality and the corrected percent mortality was calculated using Abbott's formula.
Corrected mortality % = T – C/100 – C × 100 (where, T = Total mortality in treatment; C = Total mortality in control).
LC50 value (ppm) was calculated for each compound with the help SPSS statistical Package (v16.0) using ASUS VivoBook laptop.
QSAR study was performed by using negative logarithm of experimental LC50 (ppm) [pLC50 = –log (LC50)] as dependent variable and 2D descriptors (Table 5) as independent variables. ChemDraw Ultra 7.0 software was used for drawing 2D structures of compounds which were converted to mol structures. To describe several features of molecular structure 239 2D descriptors were taken and composed of structural, thermodynamic, electronic and spatial descriptors, e.g., element count, atomic valence connectivity index (chiV), refractivity, estate number, chain path count, retention index (chi), path cluster, semi-empirical path count, molecular cluster, topological index, molecular weight, and logP. The various descriptors were calculated by energy minimization and geometry optimization through batch energy minimization method using Merck Molecular Force Field (MMFF) by taking RMS gradient (0.01), number of cycles (max. 1,000) and distance dependent dielectric (1). Various Baumann alignment-independent (AI) descriptors were also calculated. All computational activities were performed by using Vlife MDS QSAR plus 4.6 software in ASUS VivoBook (windows 10 OS and Intel Core i5).
Entire data of 16 compounds was divided into two sets i.e., training set (11 compounds) and test set (5 compounds) with the help of sphere exclusion method (Hudson et al., 1996). Unicolumn statistics was done to establish the accuracy of selection of training and test sets (Tables 2B, 3B, 4B) as maximum value of the training set was higher than that of the test set and the minimum value of the training set was lower than that of the test set.
Ferrocenyl chalcones were prepared by condensation of acetylferrocene with different benzaldehydes both by conventional and microwave methods. It was found that the yield was higher in microwave method (78–92%) as compared to conventional method (71–87%) (Table 1). In MM, reaction was completed in 1–5 min as compared to CM requiring 10–40 h (Table 1). Peaks at δ 6.64–7.45 (1H, d, J = 16 Hz, H-α) and δ 7.24–8.24 (1H, d, J = 16 Hz, H-β) as double doublets for two protons with J = 16 Hz each, were characteristic peaks of olefinic bond in 1H-NMR spectrum of all the compounds (3a-3p) and established the condensation of carbonyl moiety of acetylferrocene with carbonyl moiety of different benzaldehydes. In 13C-NMR, the peaks at δ 117.80–126.28 (C-α), 136.91–141.24 (C-β) for HC = CH and at δ 191.17–192.92 for CO were prominent for all the compounds. In all compounds, the higher chemical shifts values of H-α and C-α as compared to H-β and C-β was due to carbonyl moiety which polarizes the C = C double bond (Solcaniova et al., 1980). Stretching of (CO) at 1,639–1,654 and (C = C) at 1,562–1,595 cm−1 in IR spectra justified the NMR data.
All the synthesized compounds showed antifungal activity (Tables 2A, 3A). The compound 3o i.e., (2E)-1-ferrocenyl-3-(2,6-dichlorophenyl)-prop-2-en-1-one was found to be most active against S. rolfsii, having ED50 value 23.24 mg L−1 as compared to commercial fungicide Hexaconazole 5% SC (ED50 = 8.5 mg L−1) (Figure 1). But in case of A. solani, compound 3c i.e., (2E)-1-ferrocenyl-3-(4-fluorophenyl)-prop-2-en-1-one, having value ED50 = 29.9 mg L−1 showed highest activity (Figure 2). The corresponding value of commercial fungicide Mancozeb 75% WP was 2.9 mg L−1.
Table 2A. Experimental and predicted fungicidal activity of ferrocenyl chalcones against S. rolfsii.
Table 2B. Unicolumn statistics of training and test sets for fungicidal activity against S. rolfsii.
Figure 1. Fungicidal activity against S. rolfsii of (2E)-1-ferrocenyl-3-(2,6-dichlorophenyl)-prop-2-en-1-one (3o).
Figure 2. Fungicidal activity against A. solani of (2E)-1-ferrocenyl-3-(4-fluorophenyl)-prop-2-en-1-one (3c).
Figure 3. In-vitro nematicidal bioassay well culture plate containing nematode suspension (1 mL) and different concentrations (1 mL) of test compounds.
Although all the synthesized compounds possessed nematicidal activity against M. incognita (Table 4A), but the trend of nematicidal bioassay revealed that the compound (2E)-1-ferrocenyl- 3-(4-bromophenyl)-prop-2-en-1-one (3b) was more potent with LC50 values of 10.67 ppm as compared to commercial nematicide Carbofuran 3G (LC50 = 4.78 ppm) and Velum Prime 500 SC (LC50 = 2.40 ppm). It was found that mortality was directly proportional to time of exposure and concentration.
Table 4A. Experimental and predicted nematicidal activity of ferrocenyl chalcones against M. incognita.
Table 4B. Unicolumn statistics of training and test sets for nematicidal activity against M. incognita.
Three statistically significant QSAR models viz. Model-1 (PLS), Model-2 (MLR), and Model-3 (PCR) were developed in 2D-QSAR analysis of fungicidal activity against S. rolfsii and A. solani and nematicidal activity against M. incognita. Fitness plot of each model was used for validation of statistical significance of model (Figures 4A, 5A, 6A).
Figure 4. (A) Graphs of experimental vs. predicted fungicidal activity of different models against S. rolfsii. (B) Contribution charts of 2D-QSAR model.
Figure 5. (A) Graphs of experimental vs. predicted fungicidal activity of different models against A. solani. (B) Contribution charts of 2D-QSAR models.
Figure 6. (A) Graphs of experimental vs. predicted nematicidal activity of different models against M. incognita. (B) Contribution charts of 2D-QSAR models.
S. rolfsii
Model-2 (MLR)
Where n = 11, DF = 8, r2 = 0.9997, q2 = 0.9962, F_test = 3036.53, r2_se = 0.0122, q2_se = 0.0464, pred_r2 = 0.6201, pred_r2se = 0.3985
A. solani
Model-1 (PLS)
Where n = 11, DF = 9, r2 = 0.9348, q2 = 0.7499, F_test = 50.2052, r2_se = 0.0727, q2_se = 0.2917, pred_r2 = 0.4856, pred_r2se = 0.2826
M. incognita
Model-3(PCR)
Where n = 11, DF = 8, r2 = 0.8784, q2 = 0.7728, F_test = 78.9078, r2_se = 0.0528, q2_se = 0.0722, pred_r2 = 0.4818, pred_r2se = 0.1703.
The biological activity variance for all QSAR models was estimated by multiplying the correlation coefficient (r2) with 100. The predictive power (q2) of models was determined by LOO (Left Out One) method. F denotes ratio of variance of models and that of error in regression. Models with higher F and lower SE of estimation viz. r2se and q2se were found statistically significant. External validation with pred_r2 > 0.3 confirmed the predictive power of QSAR model. The best model was determined by r2, q2, higher values of pred_r2 F_test and (Tables 2C, 3C, 4C). The best measure of authenticity of 2D QSAR is higher q2 value, because overfitting to data may result into higher r2 value. Mostly, a value of q2 >0.5 is found acceptable (Golbraikh and Tropsha, 2002; Doweyko, 2004; Ponce et al., 2004).
Alignment Independent (AI) descriptors were calculated as explained in Baumann's paper based on type of atom, bond and molecular topology (Balaban, 1982). Maximum three and minimum one attribute was given for every atom. First attribute “T” denotes molecule topology. Second attribute was denoted by atomic symbol and third by atoms with multiple (double or triple) bonds. Then selective distance count statistics, which counts all the fragments between first and last atom separated by graph distance. Graph distance is the minimum number of atoms along the path connecting two atoms in molecular structure. Topological indices are numerical values about chemical compositions which determines correlation between chemical structure and biological activity. AI descriptors were calculated by using attributes viz. 2 (atom with double bond), 3(atom with double bond), C (Carbon), N (Nitrogen), O (Oxygen), S (Sulfur), H (Hydrogen), F (Flourine), Cl (Chlorine), and Br (Bromine) with distance ranging from 0 to 7.
Model-2 (MLR) was found to be the best model for QSAR study molecules against S. rolfsii. The developed model indicated that descriptors, Nitrogens Count, Most+ve&–vePotential Distance, DeltaPsiA, and AI descriptor, T_T_C_3 were inversely related to the fungicidal activity (Figure 4B). The major descriptor (~50%) is Nitrogen Count which negatively influences the fungicidal activity of test compounds. Most+ve&–vePotential Distance is an electrostatic descriptor which denotes distance between points having the highest value of +ve and highest value of –ve electrostatic potential on van der Waals surface area of the molecule. The result showed that it negatively influences the fungicidal activity of compounds. Therefore, lesser negative potential distance resulted in higher fungicidal activity.
Model-1 (PLS) was found to be the best model for QSAR study molecules against A. solani.The developed model indicated that descriptors, T_N_O_6, chiV2, SKMostHydrophobic HydrophilicDistance were inversely related to the fungicidal activity (Figure 5B). The major descriptor (~50%) was T_N_O_6, which negatively influences the fungicidal activity of test compounds. But FluorinesCount positively influences the fungicidal activity of test compounds.
Model-3 (PCR) was found to be the best model for QSAR study molecules against M. incognita. The developed model indicated that descriptors, DeltaAlphaA positively influences the nematicidal activity of test compounds and SaaCHcount & SsOHE-index were negatively influences the activity (Figure 6B). These two (DeltaPsiA & SaaCHcount) are major descriptors for nematicidal activity. DeltaAlphaA indicate that a greater number of non-hydrogen heteroatoms will be more nematicidal activity. SaaCHcount indicate that the total number of carbon atoms connected with a hydrogen along with two aromatic bonds will be less nematicidal activity.
This is the first report of microwave assisted synthesis and bioefficacy (fungicidal and nematicidal) evaluations of ferrocenyl chalcones. Out of 16 synthesized compounds (2E)-1-ferrocenyl-3-(3-hydroxyphenyl)-prop-2-en-1-one (3i) is new molecule which was characterized by various spectroscopic techniques. The compound 3o was found to be most active against S. rolfsii and the compound 3c showed highest activity against A. solani. The compounds 3b, 3m, 3o, 3f, and 3h were most active against root-knot nematode M. incognita. QSAR studies was performed to find quantitative relationship between fungicidal and nematicidal activity and physicochemical/structural properties of the synthesized chalcones. The developed models were evaluated and validated for their external predictive power and statistical significance. Among the three 2D-QSAR models (MLR, PCR, and PLS), MLR, PLS, and PCR analysis showed significant predictive power and reliability for S. rolfsii, A. solani, and M. incognita, respectively. Information and understanding of descriptors affecting fungicidal and nematicidal activity of these ferrocenyl chalcones be used for structure optimization to attain higher activity.
All datasets generated for this study are included in the article/Supplementary Material.
NS: conceptualization, draft editing, and supervision. DY: methodology, data creation, analysis, and original draft writing. PK: methodology and analysis. P: methodology and nematicidal analysis. VR: draft editing and supervision. DKa: methodology and fungicidal analysis. DKh: analysis and funding.
The authors declare that this study received funding from PI Industries, Udaipur as part of Prime Minister Fellowship. The funder was not involved in the study design, collection, analysis, interpretation of data, the writing of this article or the decision to submit it for publication.
The authors declare that the research was conducted in the absence of any commercial or financial relationships that could be construed as a potential conflict of interest.
The authors thank the Head, Division of Agricultural Chemicals, ICAR-IARI, New Delhi for providing facilities to carry out the research work. DY thanks DST, PI Industries Ltd., CII, New Delhi and Prime Minister's Fellowship for financial support (DST/SSK/SERB-CII-Fell/2014).
The Supplementary Material for this article can be found online at: https://www.frontiersin.org/articles/10.3389/fchem.2019.00814/full#supplementary-material
Supplementary Data Sheet 1. 1H-NMR, 13C-NMR, IR, and HR-MS spectra of compounds synthesized by Scheme 1.
Supplementary Data Sheet 2. 1H-NMR, 13C-NMR, IR, and HR-MS spectra of compound synthesized by Scheme 2.
Supplementary Data Sheet 3. Enlarge view of NMR spectra.
HR-MS, High Resolution-Mass Spectrometry; 1H-NMR, Proton Nuclear Magnetic Resonance; 13C-NMR, Carbon-13 Nuclear Magnetic Resonance; MM, Microwave Method; CM, Conventional Method; QSAR, Quantitative Structural Activity Relationship; TLC, Thin Layer Chromatography; TMS, Tetramethylsilane; PDA, Photo Diode Array; FT-IR, Fourier-transform infrared; ITCC, Indian Type Culture Collection; PDA, Potato Dextrose Agar; PLS, Partial Least Squares; MLR, Multiple Linear Regression; PCR, Principal Component Regression; UFLC, Ultra-Fast Liquid Chromatography.
Abad, P., Gouzy, J., Aury, J. M., Castagnone-Sereno, P., Danchin, E. G., Deleury, E., et al. (2008). Genome sequence of the metazoan plant-parasitic nematode Meloidogyne incognita. Nat. Biotechnol. 26:909. doi: 10.1038/nbt.1482
Abbott, W. S. (1925). A method of computing the effectiveness of an insecticide. J. Econ. Entomol. 18, 265–267. doi: 10.1093/jee/18.2.265a
Abeysinghe, S. (2009). Efficacy of combine use of biocontrol agents on control of Sclerotium rolfsii and Rhizoctonia solani of Capsicum annuum. Arch. Phytopathol. Plant Protect. 42, 221–227. doi: 10.1080/03235400600999406
Alam, S. (2004). Synthesis, antibacterial and antifungal activity of some derivatives of 2-phenyl-chromen-4-one. J. Chem. Sci. 116, 325–331. doi: 10.1007/BF02711433
Arends, I. W., Sheldon, R. A., Wallau, M., and Schuchardt, U. (1997). Oxidative transformations of organic compounds mediated by redox molecular sieves. Angew. Chem. Int. Ed. Engl. 36, 1144–1163. doi: 10.1002/anie.199711441
Bag, S., Ramar, S., and Degani, M. S. (2009). Synthesis and biological evaluation of α, β-unsaturated ketone as potential antifungal agents. Med. Chem. Res. 18, 309–316. doi: 10.1007/s00044-008-9128-x
Balaban, A. T. (1982). Highly discriminating distance-based topological index. Chem. Phys. Lett. 19, 399–404. doi: 10.1016/0009-2614(82)80009-2
Caboni, P., Aissani, N., Demurtas, M., Ntalli, N., and Onnis, V. (2016). Nematicidal activity of acetophenones and chalcones against Meloidogyne incognita and structure–activity considerations. Pest Manag. Sci. 72, 125–130. doi: 10.1002/ps.3978
Doweyko, A. M. (2004). 3D-QSAR illusions. J. Comput. Aided Mol. Des. 18, 587–596. doi: 10.1007/s10822-004-4068-0
Gedye, R., Smith, F., Westaway, K., Ali, H., Baldisera, L., Laberge, L., et al. (1986). The use of microwave ovens for rapid organic synthesis. Tetrahedron Lett. 27, 279–282. doi: 10.1016/S0040-4039(00)83996-9
Golbraikh, A., and Tropsha, A. (2002). Beware of q2! J. Mol. Graph. Model. 20, 269–276. doi: 10.1016/S1093-3263(01)00123-1
Grigolli, J. F., Kubota, M. M., Alves, D. P., Rodrigues, G. B., Cardoso, C. R., Silva, D. J., et al. (2011). Characterization of tomato accessions for resistance to early blight. Appl. Biotechnol. 11:174. doi: 10.1590/S1984-70332011000200010
Hudson, B. D., Hyde, R. M., Rahr, E., Wood, J., and Osman, J. (1996). Parameter based methods for compound selection from chemical databases. Quant. Struct. Act. Relat. 15, 285–289. doi: 10.1002/qsar.19960150402
Jain, R. K., Mathur, K. N., and Singh, R. V. (2007). Estimation of losses due to plant parasitic nematodes on different crops in India. Indian J. Nematol. 37, 219–220.
Kalirajan, R., Sivakumar, S. U., Jubie, S., Gowramma, B., and Suresh, B. (2009). Synthesis and biological evaluation of some heterocyclic derivatives of chalcones. Int. J. Chemtech. Res. 1, 27–34.
Mayo, K., Nearhoof, E. H., and Kiddle, J. J. (2002). Microwave-accelerated ruthenium-catalyzed olefin metathesis. Org. Lett. 4, 1567–1570. doi: 10.1021/ol025789s
Nene, Y. L., and Thapliyal, P. N. (1979). “Fungicides in plant disease control,” Evaluation of Fungicides, eds Y. L. Nene and P. N. Thapliyal (Oxford and IBH Publishing Co.): New Delhi, 406–428.
Nolte, N. M., and Salmain, M. (2008). “In ferrocenes ligands, materials and biomolecules,” ed P. Stepnicka (West Sussex: John Wiley and Sons), 499–639.
Ponce, Y., Garit, J., Torrens, F., Zaldivar, V., and Castro, E. (2004). Atom, atom-type, and total linear indices of the “molecular pseudograph's atom adjacency matrix”: application to QSPR/QSAR studies of organic compounds. Molecules 9, 1100–1123. doi: 10.3390/91201100
Shakil, N. A., Singh, M. K., Kumar, J., Sathiyendiran, M., Kumar, G., Singh, M., et al. (2010). Microwave synthesis and antifungal evaluations of some chalcones and their derived diaryl-cyclohexenones. J. Environ. Sci. Health 45, 524–530. doi: 10.1080/03601234.2010.493482
Shakil, N. A., Singh, M. K., Sathiyendiran, M., and Kumar, J. (2011). Microwave accelerated solvent-free synthesis and antifungal evaluations of flavanones. Arch. Phytopathol. Plant Protect. 44, 1958–1965. doi: 10.1080/03235408.2010.544467
Shakil, N. A., Singh, M. K., Sathiyendiran, M., Kumar, J., and Padaria, J. C. (2013). Microwave synthesis, characterization and bio-efficacy evaluation of novel chalcone based 6-carbethoxy-2-cyclohexen-1-one and 2H-indazol-3-ol derivatives. Eur. J. Med. Chem. 59, 120–131. doi: 10.1016/j.ejmech.2012.10.038
Solcaniova, E., Toma, S., and Fiedlerova, A. (1980). Investigation of substituent effects on the 1H and 13C NMR spectra of ferrocene analogues of chalcones. Org. Magn. Resonance. 14, 181–185. doi: 10.1002/mrc.1270140307
Song, W., Ma, X., Tan, H., and Zhou, J. (2011). Abscisic acid enhances resistance to Alternaria solani in tomato seedlings. Plant Physiol. Biochem. 49, 693–700. doi: 10.1016/j.plaphy.2011.03.018
Vibhute, Y. B., and Baseer, M. A. (2003). Synthesis and activity of a new series of Chalcones as antibacterial agents. Indian. J. Chem. 42, 202–205. doi: 10.1002/chin.200314102
Keywords: ferrocenyl chalcones, antifungal activity, Sclerotium rolfsii, Alternaria solani, root-knot nematode, Meloidogyne incognita, QSAR
Citation: Yadav DK, Kaushik P, Pankaj, Rana VS, Kamil D, Khatri D and Shakil NA (2019) Microwave Assisted Synthesis, Characterization and Biological Activities of Ferrocenyl Chalcones and Their QSAR Analysis. Front. Chem. 7:814. doi: 10.3389/fchem.2019.00814
Received: 20 September 2019; Accepted: 11 November 2019;
Published: 26 November 2019.
Edited by:
Greta Varchi, Consiglio Nazionale delle Ricerche, ItalyReviewed by:
Hitendra M. Patel, Sardar Patel University, IndiaCopyright © 2019 Yadav, Kaushik, Pankaj, Rana, Kamil, Khatri and Shakil. This is an open-access article distributed under the terms of the Creative Commons Attribution License (CC BY). The use, distribution or reproduction in other forums is permitted, provided the original author(s) and the copyright owner(s) are credited and that the original publication in this journal is cited, in accordance with accepted academic practice. No use, distribution or reproduction is permitted which does not comply with these terms.
*Correspondence: Najam A. Shakil, aWFtc2hha2lsQGdtYWlsLmNvbQ==
Disclaimer: All claims expressed in this article are solely those of the authors and do not necessarily represent those of their affiliated organizations, or those of the publisher, the editors and the reviewers. Any product that may be evaluated in this article or claim that may be made by its manufacturer is not guaranteed or endorsed by the publisher.
Research integrity at Frontiers
Learn more about the work of our research integrity team to safeguard the quality of each article we publish.