- 1Institute of Advanced Wear & Corrosion Resistant and Functional Materials, Jinan University, Guangzhou, China
- 2Department of Chemistry, Jinan University, Guangzhou, China
Nowadays, due to the worldwide growth demand of energy, over consumption of fossil fuel as well as their accompanying increased negative environmental impacts, the development of renewable energy systems, such as fuel cells and water electrolyzers, is becoming one of the “holy grail” for researchers. However, their large-scale applications have been severely limited by precious and unsustainable noble-metal electrocatalysts. Hence, it is highly desirable to develop robust electrocatalysts composed exclusively of low-cost and earth-abundant elements, to reduce or replace expensive and scarce noble-metals. Carbon-based nanomaterials, including heteroatoms-doped carbons and carbon-encapsulated metal materials, have recently attracted great interests because they show remarkable electrocatalytic performance and long-term stability for energy-related reactions, such as oxygen reduction reaction (ORR), hydrogen and oxygen evolution reactions (OER), hydrazine oxidation reaction (HzOR), etc. This review summarizes the recent progress in heteroatoms-doped carbon and carbon-encapsulated metal materials, highlighting the promise as cost-efficient electrocatalysts. Finally, a prospective on the future development of these promising materials is offered.
Introduction
The over consumption of fossil fuel reserves (natural gas, petroleum, coal, etc.) and their ongoing negative environmental impacts (e.g., water, air, and soil pollution) have driven the development of alternative, environmentally-friendly energy systems to reduce and/or eliminate our over-reliance on fossil fuels (Chow et al., 2003; Barnett et al., 2012; Subbaraman et al., 2012). Fuel cells, which can directly generate electricity from chemical fuels such as hydrogen and liquid hydrazine, and water electrolyzer that can generate the hydrogen fuel from water, are the promising energy conversion systems. However, the large-scale commercialization of fuel cells and water electrolyzer has so far been hampered by expensive and unsustainable electrocatalysts used for boosting the sluggish reactions at both anode and cathode, such as oxygen reduction reaction (ORR), hydrogen oxidation reaction (HOR), and water splitting including oxygen evolution reaction (OER) and hydrogen evolution reactions (HER) (Yang et al., 2008; Bhowmik et al., 2016; Cheng et al., 2016; Guo et al., 2016; Huang et al., 2018). In recent years, great efforts have been made to develop low-cost and earth-abundant electrocatalysts to promote the above-mentioned energy-related reactions, such as the carbon-based materials and transition metal sulfides and carbides (Feng et al., 2015; Hu et al., 2015; Liu et al., 2015; Huang C. et al., 2019; Wang X.-T. et al., 2019). Among the various electrocatalysts being investigated, carbon-based materials, including heteroatoms-doped carbons and carbon-encapsulated metal materials, have drawn increasing attentions due to their low-cost, high-efficiency, and good long-term durability (Figure 1). In this review, we will discuss the synthetic procedure of heteroatoms-doped carbons and carbon-encapsulated metal materials, as well as their characterizations and electrochemical performance.
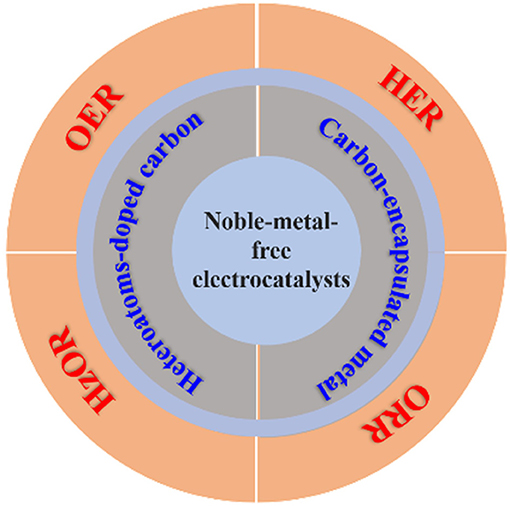
Figure 1. Summary illustration of the carbon-based noble-metal-free electrocatalysts and their applications.
Fuel Cell Reactions
ORR is the reaction occurred on cathode in fuel cells, which involves multiple electrochemical processes. The ORR can either proceed through a two-step, a two electron (2e) process with the formation of intermediate in alkaline, and H2O2 in acidic media, or via a more efficient four-electron (4e) pathway to directly reduce O2 into OH− in alkaline and H2O in acidic media (Dai et al., 2015). The sluggish kinetic of ORR, the high cost and low durability of the Pt-based electrocatalysts are the current bottlenecks, which are needed to be addressed to enlarge the application of fuel cells (Liang et al., 2011; Huang et al., 2015b). Nitrogen and other heteroatoms-doped carbon materials, as well as carbon-encapsulated metal materials have shown remarkable electrocatalytic activity and durability toward ORR (Geng et al., 2011; Lin et al., 2012).
Hydrazine oxidation reaction (HzOR) is a vital reaction in direct liquid fuel cells (DLFCs) taking place at the anode. Due to its more favorable energy and power densities compared to hydrogen fuel, as well as no harmful and/or carbon-containing byproducts, HzOR has been becoming one of the great scientific and technological interest (Yamada et al., 2003; Sanabria-Chinchilla et al., 2011). Unfortunately, electrochemical conversion of hydrazine molecular to nitrogen on catalytic surface in DLFCs is kinetically sluggish and requires a relatively high over potential at electrodes, which is not well-suited for quantitation via conventional electrochemical approaches. In order to address the facing problem, extensively studies have been made in last decades to lower the overpotential of the hydrazine oxidation and improve the conversion efficiency. Currently, electrodes such as platinum, gold, silver, palladium, and alloys have been reported to be the electrocatalysts for the electrochemical oxidation of hydrazine (Gao et al., 2007; Rosca and Koper, 2008; Ye et al., 2008; Yi and Yu, 2009). However, these materials are tending to agglomerate or leach out during reaction and lose/reduce their activity. Others have found that carbon-based materials such as modified carbon nanotube and reduced graphene nanosheets can effectively electrocatalyze hydrazine oxidation, which paved a way to find the novel high-performance, inexpensive, sustainable metal-free HzOR electrocatalysts (Zhao et al., 2002; Wang et al., 2010).
Water-Splitting Reactions
Electrolysis of water is widely considered to be the green and efficient approach to produce hydrogen fuel. The electrochemical water-splitting consists of two half reactions: the cathodic hydrogen evolution reaction (HER, 2H+ (aq) + 2e− → H2 (g)) and the anodic oxygen evolution reaction (OER, 2H2O (l) → 4e− + 4H+ (aq) + O2 (g)) (Shi and Zhang, 2016). To successfully conduct electrochemical water splitting, the applied voltages for both reactions must be above the thermodynamic potential values corresponding to the intrinsic activation barriers (i.e., overpotential, η) (Yan et al., 2014; Zou et al., 2014). Moreover, OER requires a higher overpotential (higher energy) to overcome the kinetic barrier to occur than that of HER due to the four-electron transfer involved in OER and gives the inherent sluggish kinetic (Xu et al., 2016). Hence, electrocatalysts for both the HER and OER are essential to reduce the overpotentials and consequently increase the energy conversion efficiency. Currently, the most effective electrocatalysts for the HER and OER are noble metal/metal oxides, such as Pt, RuO2, and IrO2 (Lee et al., 2012). However, the high cost and element scarcity of noble-metal based materials limited their wide application. Therefore, it is highly desired to develop effective alternative water splitting electrocatalysts with low cost and high abundance.
Heteroatoms-Doped Carbons
As one of the most important classes of noble metal-free materials, carbon-based nanomaterials have drawn much attention because of their unique chemical, optical, electrical, and physical properties. Heteroatoms doping (e.g., nitrogen, boron, oxygen, sulfur, phosphorous) in carbon structure can affect various physicochemical properties of sp2 carbon materials, and hence lead to significant changes in local graphitic structure, hardness, electrical conductivity, and chemical reactivity (Wiggins-Camacho and Stevenson, 2009). Thereby, the structural incorporation of foreign atoms in graphitized carbon has received increasing attention nowadays due to its enhanced physicochemical properties and electrocatalytic performance.
N-doped carbon materials, owing to their unique electronic properties and structural features, have been reported to exhibit not only efficient ORR performance comparable to that of commercial Pt/C (20 wt.%), but also good long-term stability, and excellent resistance to methanol crossover effects that are superior to Pt/C (Tang et al., 2009; Chen et al., 2012; Sharifi et al., 2012; Hou et al., 2015). The research area on this kind of metal-free electrocatalyst actually dates back to several decades ago, particularly to the seminal work reported by Dai, in which nitrogen doped carbon nanotube arrays were reported to remarkably electrocatalyze ORR in alkaline fuel cells with high long-term stability and good methanol crossover tolerance (Gong et al., 2009). Following this report, many other related materials without any metals, such as nitrogen-doped graphene and nitrogen-doped mesoporous carbons, were developed to show remarkable electrocatalytic activity and high durability for the ORR (Qu et al., 2010). Hu and his co-authors have prepared boron doped carbon nanotubes (BCNT) and directly used as electrocatalysts for ORR in alkaline medium (Yang et al., 2011). The result illustrated that the ORR performance of the synthesized BCN was related with the boron dopant amount. Specifically, the ORR activity increased with the increasing boron content, illustrating the importance of the boron moieties (Figure 2). Moreover, BCN gave good ORR selectivity and methanol crossover resistance, qualifying the B-doped nanotubes can serve as promising ORR metal-free electrocatalysts. Moreover, carbon materials with phosphorus dopants also have shown strikingly ORR activity in alkaline medium (Liu et al., 2011). Ordered mesoporous carbons with a small amount of P doping, prepared by Yu group, demonstrated their promise as a metal-free electrocatalyst for ORR, which featured excellent electrocatalytic activity via four-electron pathway in alkaline medium, enhanced stability, and excellent alcohol tolerance, compared to those of Pt/C (Yang D.-S. et al., 2012).
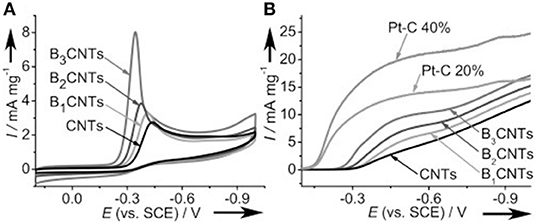
Figure 2. Electrocatalytic capabilities of the BCNT catalysts for the ORR in O2-saturated 1.0 M NaOH electrolyte. (A) CV curves (scan rate 50 mVs−1). (B) RDE voltammetry with a rotation speed of 2,500 rpm (scan rate 10 mV s−1). For comparison, corresponding examinations for CNTs and commercial Pt/C catalysts (20 and 40 wt % Pt loading) were also carried out. Re-printed with permission from Wiley-VCH Publications 2011 (Yang et al., 2011).
Along with single heteroatoms doped carbon materials, dual or multi heteroatoms doped carbons, which constitute of two or three kinds of heteroatoms in the carbon, were also investigated as metal-free electrocatalysts for the ORR. Wang et al. reported that vertically aligned B and N co-doped carbon nanotubes electrode, which prepared by pyrolysis of melamine diborate, gave a higher electrocatalytic activity for ORR in alkaline media than the one singly doped with boron or nitrogen (Wang et al., 2011). They believed that the promoted activity was mainly attributed to the synergetic effect arising from the co-doping of boron and nitrogen. On the other hand, nitrogen and sulfur co-doped carbon aerogels have improved the overall electrocatalytic activity in both basic and acidic media, compared to the corresponding carbon solely doped with nitrogen, suggesting sulfur co-doping can further enhance the ORR activity (Wohlgemuth et al., 2012). Recently, researchers also reported the tri (N, B, P)-doped carbon can significantly improve the performance of nitrogen doped carbons and show remarkable ORR performance (Choi et al., 2012). We have synthesized N-, O-, and S-tridoped, polypyrrole-derived mesoporous carbons (NOSCs) and studied their ORR performance using colloidal silica as template. The synthesized NOSCs exhibited good catalytic activity toward ORR with low onset potential and low Tafel slope (Meng et al., 2014b). Moreover, the electron transfer numbers and H2O/H2O2 ratios as product of the electrocatalytic reaction were found to be tuneable by the amount of colloidal silica and their synergistic effect of N, S, and O tri-doping (Figure 3).
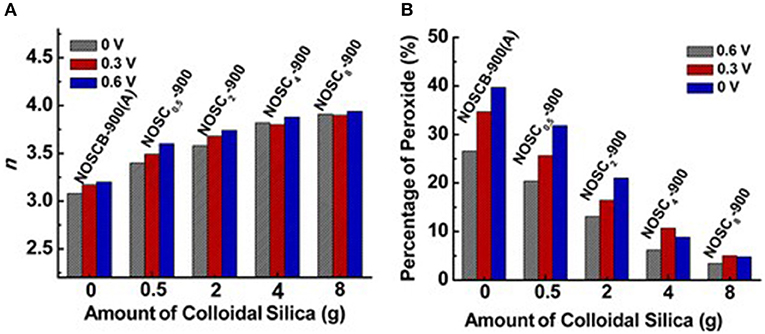
Figure 3. (A) Electron-transfer number (n) and (B) % peroxide formed at three different potentials vs. amount of colloidal silica used as templates for a series of NOSCx-900 materials. Re-printed with permission from the American Chemical Society Publications 2014 (Meng et al., 2014a).
Additionally, heteroatoms doped carbons can also serve as metal-free electrocatalysts for HzOR, HER and OER. N-doped carbon nanoneedles (CNNs) with well-organized graphitic multi-layers and large proportions of N-doped edge planes (Figure 4) have been proven to be efficient metal-free electrocatalysts for hydrazine oxidation (Silva et al., 2012). The high catalytic activity toward HzOR was mostly due to their unique structures and large proportions of exposed edge planes. Later, the same group also synthesized other carbon-based materials by carbonization of cellulose filter paper, rice, yeast cell, and polypyrrole, all of which showed remarkable HzOR activity (Huang et al., 2015a; Koh et al., 2016; Martins et al., 2016). In the case of nitrogen and oxygen doped carbons, they believe that the presence of dopants and defects aid the effective adsorption of hydrazine onto the catalytic surfaces, then the hydrazine molecule was effectively dissociated/oxidized by the flow of electrons, giving a good electrocatalytic performance (Figure 5) (Meng et al., 2014b). During the oxidation process, carbon atoms with the relatively positive charge in the graphitic structure, facilitated the flow of electrons, which allowed the oxidation of N2H4 molecular to form N2 and H+. H+ was then react with OH− to form water. Subsequently, the water and nitrogen products were desorbed and fresh hydrazine molecules was adsorbed on the surface to keep the catalytic cycle running.
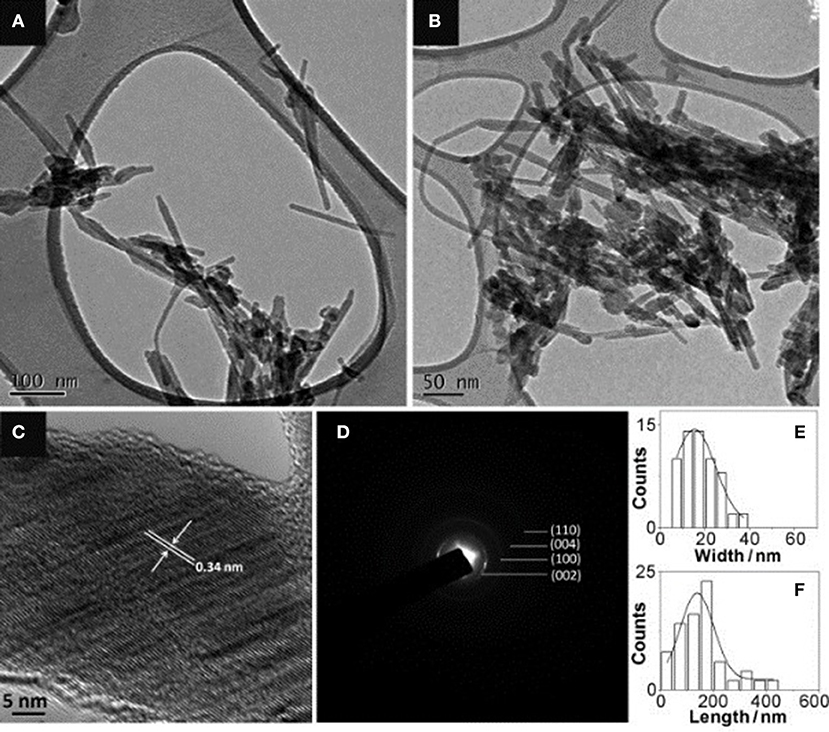
Figure 4. Characterization of N-doped carbon nanoneedles: (A) low magnification and (B) slightly higher magnification TEM images. (C) HRTEM and (D) SAED. (E) Their width distribution and (F) length distribution. Re-printed with permission from Wiley-VCH Publications 2012 (Silva et al., 2012).
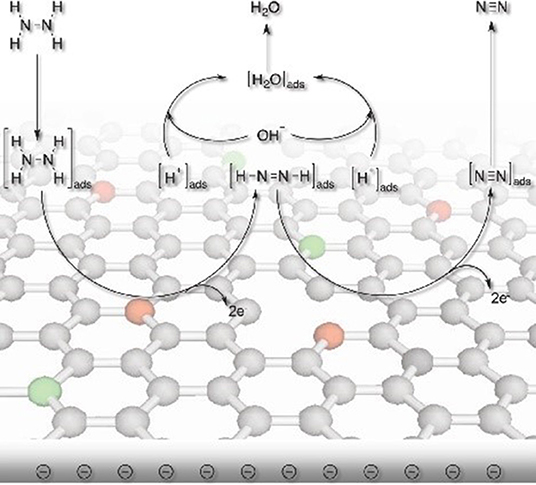
Figure 5. Schematic illustration showing the proposed electrocatalytic processes over nitrogen and oxygen doped carbon. Re-printed with permission from Wiley-VCH Publications 2014 (Meng et al., 2014b).
With the high rapid development of graphene and carbon-based materials, heteroatoms doped carbons were also investigated as OER and HER electrocatalysts in water-splitting reactions. Zhao et al reported that the nitrogen-doped carbon materials function as the efficient oxygen evolution electrocatalysts with good stability (Zhao et al., 2013). The material gave a current density of 10 mA cm−2 at the overpotential of 0.38 V in alkaline electrolytes, which was comparable to those of iridium and cobalt oxide catalysts. The electrochemical and physical results displayed that the good oxygen evolution activity of the nitrogen/carbon materials was associated with the pyridinic-N and quaternary-N. Nitrogen and phosphorus co-doped mesoporous carbon foam with a large surface area of ~1,663 m2 g−1 was reported to act as bi-functional electrocatalyst for OER and ORR with good electrocatalytic properties (Zhang et al., 2015). The N and P co-doping and the highly porous network of the carbon foam were the main reason for the good bifunctional activity. Later, Dai et al. reported that N, S co-doped graphitic sheets with stereoscopic holes (SHG) can efficiently serve as tri-functional electrocatalysts for the ORR, OER, and HER, simultaneously (Hu and Dai, 2017). The SHG electrode showed a remarkable OER activity with a small onset potential of 1.49 V and overpotential of 1.56 V vs. RHE at a current density of 10 mA cm−2 in 0.1 M KOH electrolyte, which were comparable to those of commercially available RuO2 electrocatalyst, respectively. In the case of HER, SHG electrode exhibited a similar activity of metal-containing HER electrocatalysts, giving positive onset potential, large current density and small Tafel slope. The multifunctional electrocatalytic activities were attributed to the synergistic effect of N, S co-doping and the large surface area derived from this unique SHG architecture, which provided efficient pathways for electron and electrolyte/reactant transports. B-substitute graphene (B-SuG) was also found to electrocatalyze HER in acidic medium (Sathe et al., 2014). In comparison with the graphene without B doping, B-SuG performed a better activity, with more positive onset potential and larger current density. Jiao et al. have synthesized a series of heteroatoms doped graphene and investigated their electrochemical performance as metal-free electrocatalyst for HER (Jiao et al., 2016). Density functional theory (DFT) calculations illustrated that heteroatoms doping can significantly increase the hydrogen adsorption strength of graphene, lower the adsorption free energy of H (Δ) and thus enhance the HER activity, especially for the dual doping ones.
Synthesis of Heteroatoms-Doped Carbon Materials
The preparation of heteroatom-doped carbon nanomaterials involves one-pot synthesis by in-situ incorporating heteroatoms during the formation of carbon nanomaterials or the post-synthesis through the post-treatment of performed carbon nanomaterials with the heteroatoms-containing precursor. The in-situ doping, direct carbonization of the dopant-containing precursors and carbon precursors together, can ensure the dopants structural incorporation into the carbon framework with a homogeneous distribution. In recent years, several heteroatom-doped carbon materials have been fabricated by this direct method. Nitrogen-doped carbon nanotube and nanofiber have been prepared from nitrogen-containing precursors, such as melamine (Terrones and Terrones, 1999; Terrones et al., 1999), benzylamine (Munoz-Sandoval et al., 2017), acetonitrile (Xia and Mokaya, 2004). Moreover, other synthetic substances, possessing large amount of dopants atoms, were also used to prepare heteroatoms-doped mesoporous carbons, including dicyandiamide (Liu R. et al., 2010; Liu Z.-W. et al., 2010), triphenylphosphine (Yang S. et al., 2012), polyaniline (Ajayan et al., 2007; Vinu et al., 2008; Lei et al., 2009), polypyrrole (Chang et al., 2007; Shrestha and Mustain, 2010), and so on. Asefa group has used polyaniline as the carbon and nitrogen precursor to synthesize the nitrogen and oxygen co-doped mesoporous carbons (PDMCs), which showed remarkable ORR performance with positive onset potential, large current density, high electron transfer number and long-term stability, due to the structural doping of heteroatoms into the carbon framework (Silva et al., 2013). Recently, our group have synthesized N, O, and P tri-doped hollow carbons (NOPHC), which served as bifunctional metal-free electrocatalysts for HER and ORR, using polypyrrole as precursor and Co2P as template (Huang S. et al., 2019). N, O and P were “in-situ” incorporated into the carbon structure during pyrolysis process, while hollow structure formed after removal of Co2P template (Figure 6). Additionally, Antonietti and others also employed non-volatile ionic liquids (ILs), composed of an organic N-containing cation and a bulky inorganic anion, as excellent direct precursors for synthesis of nitrogen (Paraknowitsch et al., 2010a,b), phosphorous (Paraknowitsch et al., 2013), and/or sulfur-doped (Wang and Dai, 2010; Paraknowitsch et al., 2012) carbon materials with high amount of dopants. Post-synthesis method was also widely employed to fabricate heteroatoms doped carbons. Yang and co-workers have successfully synthesized N (or S) doped graphene with large surface area via pyrolysis of graphene oxide in presence of guest gases (NH3 or H2S). When the annealing temperature was in the range of 500–1,000°C, both N and S were doped into the graphitic carbon, forming different binding configurations at the edges or on the planes of the graphene (Yang S. et al., 2012).
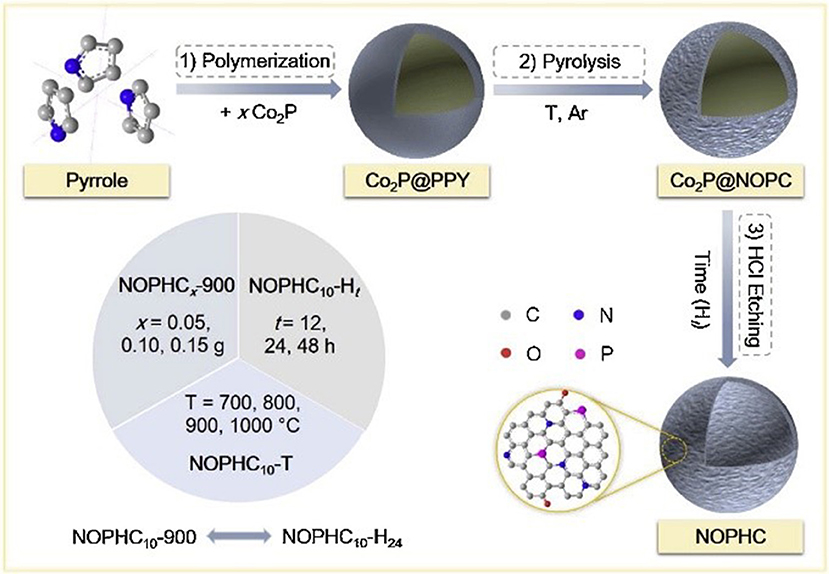
Figure 6. Synthetic procedure used to prepare NOPHCs electrocatalysts. Re-printed with permission from Elsevier Publications 2019 (Huang S. et al., 2019).
Carbon-Encapsulated Metal Materials
In addition to heteroatoms-doped carbons, carbon-encapsulated metals materials were also widely investigated as promising alternatives of the noble-metal based electrocatalysts for the energy conversion systems (Wang et al., 2018; Ouyang et al., 2019; Wang J.-Y. et al., 2019). Bao group has encapsulated 3d transition metals Fe, Co, and the FeCo alloy into nitrogen-doped carbon nanotubes (NCNTs) through a chemical vapor deposition (CVD) method (Deng et al., 2014). The synthesized NCNTs encapsulating 3d TM Fe, Co, and FeCo alloy nanoparticles (NPs) displayed good activity and long-term durability toward HER in acidic medium. DFT calculations, combining with the experimental results, illustrated that the introduction of metal and nitrogen in the carbon can synergistically enhance the HER activity. Our group also prepared N-, O-, and S-tridoped carbon-encapsulated Co9S8 materials (Co9S8@NOSCs) which have proven to act as noble metal-free bifunctional electrocatalysts for HER and OER in alkaline media (Huang et al., 2017). The Co9S8@NOSC nanocomposite materials were fabricated by preparing a S- and Co(II)-containing polypyrrole solid precursors (S-Co-PPY), carbonizing the S-Co-PPY precursor at different high temperatures, and then removal of surface bound metallic species on the carbonized products in concentrated HCl solution, as illustrated in Figure 7. Regarding to OER, Co9S8@NOSC-900 nanomaterials, obtained at pyrolysis temperature of 900°C, gave an efficient electrocatalytic activity, with a small overpotential of 340 mV at current density of 10 mA cm−2, high anodic current density, low Tafel slope (68 mV dec−1) as well as high (nearly 100%) Faradic efficiency. The excellent electrocatalytic activity can be derived from the synergistic effects between the heteroatom-doped carbon layers and the Co9S8 cores in the materials. Later, N-, O-, and S-doped carbon-encapsulated Ni3S2 and NiS core-shell architectures were prepared using the similar method with S- and Ni(II)-containing polypyrrole solid precursors (S-Ni-PPY) (Cao et al., 2018). The materials can bifunctionally electrocatalyze HER and ORR in alkaline media with good activity and long-term stability.
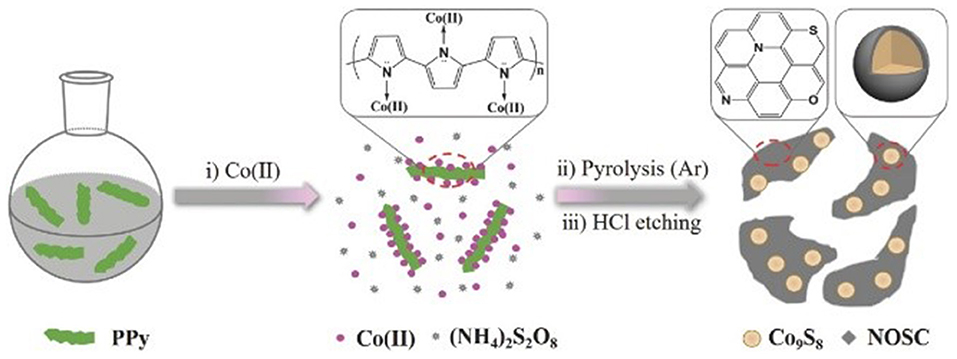
Figure 7. Synthetic procedures used to synthesize Co9S8@NOSC hybrid electrocatalysts. Re-printed with permission from Wiley-VCH Publications 2017 (Huang et al., 2017).
Conclusions, Future Outlook, and Perspectives
Over the last few years, great effort has been made to develop the carbon-based materials as (noble) metal-free electrocatalyst for the ORR, HzOR, HER, and OER for fuel cells and water electrolyzer. Although huge progress has been achieved, there is still more research needed to be given for carbon-based materials. For example, the electrocatalytic performance for most (noble) metal-free electrocatalysts is difficult to compete the noble-based counterparts, which require further improvement. Also, the electrocatalytic mechanism and the exact active sites are not well-known, both of which are needed to be explored. In addition, there is difficulty in obtaining heteroatoms-doped carbon with high density of dopants.
To overcome the above-mentioned shortcomings, new precursors or synthetic methods are demanded to fabricate the carbons with high density of heteroatoms or large number of active sites, which in turn can obtain the carbon materials with enhanced catalytic activity. We believed that by combining various experimental approaches, state-of-the-art characterizations and powerful computational calculations, the new, low-cost (noble) metal-free carbon electrocatalysts with high electrocatalytic activity and clear catalytic mechanism can be accomplished for clean energy systems.
Author Contributions
All authors listed have made a substantial, direct and intellectual contribution to the work, and approved it for publication.
Conflict of Interest
The authors declare that the research was conducted in the absence of any commercial or financial relationships that could be construed as a potential conflict of interest.
Acknowledgments
We gratefully appreciate the financial support from the National Natural Science Foundation of China (21701199 and 21773093), the Starting Project for Doctoral Fellows Sponsored by the Natural Science Foundation of Guangdong Province (2017A030310503), the Fundamental Research Funds for the Central Universities of China (21619417).
References
Ajayan, V., Pavuluri, S., Toshiyuki, M., Toshio, S., Anjana, A., Katsuhiko, A., et al. (2007). Novel hexagonally ordered nitrogen-doped mesoporous carbon from SBA-15/polyaniline nanocomposite. Chem. Lett. 36, 770–771. doi: 10.1246/cl.2007.770
Barnett, S. M., Goldberg, K. I., and Mayer, J. M. (2012). A soluble copper-bipyridine water-oxidation electrocatalyst. Nat. Chem. 4, 498–502. doi: 10.1038/NCHEM.1350
Bhowmik, T., Kundu, M. K., and Barman, S. (2016). Growth of one-dimensional RuO2 nanowires on g-carbon nitride: an active and stable bifunctional electrocatalyst for hydrogen and oxygen evolution reactions at all pH value. ACS Appl. Mater. Interfaces 8, 28678–28688. doi: 10.1021/acsami.6b10436.
Cao, Y., Meng, Y., Huang, S., He, S., Li, X., Tong, S., et al. (2018). Nitrogen-oxygen and sulfure-doped carbon-encapsulated Ni3S2 and NiS core-shell architectures: bifunctional electrocatalysts for hydrogen evolution and oxygen reduction reactions. ACS Sustainable Chem. Eng. 6, 15582–15590. doi: 10.1021/acssuschemeng.8b04029
Chang, H., Joo, S. H., and Pak, C. (2007). Synthesis and characterization of mesoporous carbon for fuel cell applications. J. Mater. Chem. 17, 3078–3088. doi: 10.1039/B700389G
Chen, S., Bi, J., Zhao, Y., Yang, L., Zhang, C., Ma, Y., et al. (2012). Nitrogen-doped carbon nanocages as efficient metal-free electrocatalysts for oxygen reduction reaction. Adv. Mater. 24, 5593–5597. doi: 10.1002/adma.201202424
Cheng, N., Stambula, S., Wang, D., Banis, M. N., Liu, J., Riese, A., et al. (2016). Platinum single-atom and cluster catalysis of the hydrogen evolution reaction. Nature 7, 13638–13647. doi: 10.1038/ncomms13638
Choi, C. H., Park, S. H., and Woo, S. I. (2012). Binary and ternary doping of nitrogen, boron, and phosphorus into carbon for enhancing electrochemical oxygen reduction activity. ACS Nano 6, 7084–7091. doi: 10.1021/nn3021234
Chow, J., Kopp, R. J., and Portney, P. R. (2003). Energy resources and global development. Science 28, 1528–1531. doi: 10.1126/science.1091939
Dai, L., Xue, Y., Qu, L., Choi, H.-J., and Baek, J.-B. (2015). Metal-free catalysts for oxygen reduction reaction. Chem. Rev. 115, 4823–4892. doi: 10.1021/cr5003563
Deng, J., Ren, P., Deng, D., Yu, L., Yang, F., and Bao, X. (2014). Highly active and durable non-precious-metal catalysts encapsulated in carbon nanotubes for hydrogen evolution reaction. Energy Environ. Sci. 7, 1919–1923. doi: 10.1039/C4EE00370E
Feng, L.-L., Yu, G., Wu, Y., Li, G.-D., Liu, H., Sun, Y., et al. (2015). High-index faceted Ni3S2 nanosheet arrays as highly active and ultrastable electrocatalysts for water splitting. J. Am. Chem. Soc. 137, 4023–14026. doi: 10.1021/jacs.5b08186
Gao, G., Guo, D., Wang, C., and Li, H. (2007). Electrocrystallized Ag nanoparticle on functional multi-walled carbon nanotube surfaces for hydrazine oxidation. Electrochem. Commun. 9, 1582–1586. doi: 10.1016/j.elecom.2007.02.026
Geng, D., Chen, Y., Chen, Y., Li, Y., Li, R., Sun, X., et al. (2011). High oxygen-reduction activity and durability of nitrogen-doped graphene. Energy Environ. Sci. 4, 760–764. doi: 10.1039/C0EE00326C
Gong, K., Du, F., Xia, Z., Durstock, M., and Dai, L. (2009). Nitrogen-doped carbon nanotube arrays with high electrocatalytic activity for oxygen reduction. Science 323, 760–764. doi: 10.1126/science.1168049
Guo, D., Shibuya, R., Akiba, C., Saji, S., Kondo, T., and Nakamura, J. (2016). Active sites of nitrogen-doped carbon materials for oxygen reduction reaction clarified using model catalysts. Science 351, 361–365. doi: 10.1126/science.aad0832
Hou, J., Cao, C., Idrees, F., and Ma, X. (2015). Hierarchical porous nitrogen-doped carbon nanosheets derived from silk for ultrahigh-capacity battery anodes and supercapacitors. ACS Nano 9, 2556–2564. doi: 10.1021/nn506394r
Hu, C., and Dai, L. (2017). Multifunctional carbon-based metal-free electrocatalysts for simultaneous oxygen reduction, oxygen evolution, and hydrogen evolution. Adv. Mater. 29:1604942. doi: 10.1002/adma.201604942
Hu, C., Yu, C., Li, M., Wang, X., Dong, Q., Wang, G., et al. (2015). Nitrogen-doped carbon dots decorated on graphene: a novel all-carbon hybrid electrocatalyst for enhanced oxygen reduction reaction. Chem. Commun. 51, 3419–3422. doi: 10.1039/C4CC08735F
Huang, C., Ouyang, T., Zou, Y., Li, N., and Liu, Z.-Q. (2018). Ultrathin NiCo2Px nanosheets strongly coupled with CNTs as efficient and robust electrocatalysts for overall water splitting, J. Mater. Chem. A 6, 7420–7427. doi: 10.1039/C7TA11364A
Huang, C., Zou, Y., Ye, Y.-Q., Ouyang, T., Xiao, K., and Liu, Z.-Q. (2019). Unveiling the active sites of Ni-Fe phosphide/metaphosphate for efficient oxygen evolution under alkaline conditions. Chem. Commun. 55, 7687–7690. doi: 10.1039/C9CC03024G
Huang, S., Meng, Y., Cao, Y., He, S., Li, X., Tong, S., et al. (2019). N-, O- and P-doped hollow carbons: Metal-free bifunctional electrocatalysts for hydrogen evolution and oxygen reduction reactions. Appl. Catal. B Environ. 248, 239–248. doi: 10.1016/j.apcatb.2019.01.080
Huang, S., Meng, Y., He, S., Goswami, A., Wu, Q., Li, J., et al. (2017). N-, O-, and S-tridoped carbon-encapsulated Co9S8 nanomaterials: efficient bifunctional electrocatalysts for overall water splitting. Adv. Funct. Mater. 27:1606585. doi: 10.1002/adfm.201606585
Huang, X., Zhao, Z., Cao, L., Chen, Y., Zhu, E., Lin, Z., et al. (2015a). High-performance transition metal-doped Pt3Ni octahedra for oxygen reduction reaction. Science 348, 1230–1234. doi: 10.1126/science.aaa8765
Huang, X., Zou, X., Meng, Y., Mikmeková, E., Chen, H., Voiry, D., et al. (2015b). Yeast cells-derived hollow core/shell heteroatom-doped carbon microparticles for sustainable electrocatalysis. ACS Appl. Mater. Interface 7, 1978–1986. doi: 10.1021/am507787t
Jiao, Y., Zheng, Y., Davey, K., and Qiao, S.-Z. (2016). Activity origin and catalyst design principles for electrocatalytic hydrogen evolution on heteroatom-doped graphene. Nat. Energy 1, 16130–16138. doi: 10.1038/nenergy.2016.130
Koh, K., Meng, Y., Huang, X., Zou, X., Chhowalla, M., and Asefa, T. (2016). N- and O-doped mesoporous carbons derived from rice grains: efficient metal-free electrocatalysts for hydrazine oxidation. Chem. Commun. 52, 13588–13591. doi: 10.1039/C6CC06140K
Lee, Y., Suntivich, J., May, K. J., Perry, E. E., and Shao-Horn, Y. (2012). Synthesis and activities of rutile IrO2 and RuO2 nanoparticles for oxygen evolution in acid and alkaline solutions. J. Phys. Chem. Lett. 3, 399–404. doi: 10.1021/jz2016507
Lei, Z., An, L., Dang, L., Zhao, M., Shi, J., Bai, S., et al. (2009). Highly dispersed platinum supported on nitrogen-containing ordered mesoporous carbon for methanol electrochemical oxidation. Micropor. Mesopor. Mater. 119, 30–38. doi: 10.1016/j.micromeso.2008.09.033
Liang, Y., Li, Y., Wang, H., Zhou, J., Wang, J., Regier, T., et al. (2011). Co3O4 nanocrystals on graphene as a synergistic catalyst for oxygen reduction reaction. Nat. Mater. 10, 780–786. doi: 10.1038/NMAT3087
Lin, Z., Waller, G., Liu, Y., Liu, M., and Wong, C.-P. (2012). Facile synthesis of nitrogen-doped graphene via pyrolysis of graphene oxide and urea, and its electrocatalytic activity toward the oxygen-reduction reaction. Adv. Energy Mater. 2, 884–888. doi: 10.1002/aenm.201200038
Liu, R., Wu, D., Feng, X., and Müllen, K. (2010). Nitrogen-doped ordered mesoporous graphitic srrays with high electrocatalytic activity for oxygen reduction. Angew. Chem. Int. Ed. 49, 2565–2569. doi: 10.1002/anie.200907289
Liu, Y., Yu, G., Li, G.-D., Sun, Y., Asefa, T., Chen, W., et al. (2015). Coupling Mo2C with nitrogen-rich nanocarbon leads to efficient hydrogen-evolution electrocatalytic sites. Angew. Chem. Int. Ed. 54, 10752–10757. doi: 10.1002/anie.201504376
Liu, Z., Peng, F., Wang, H., Yu, H., Tan, J., and Zhu, L. (2011). Novel phosphorus-doped multiwalled nanotubes with high electrocatalytic activity for O2 reduction in alkaline medium. Catal. Commun. 16, 35–38. doi: 10.1016/j.catcom.2011.08.038
Liu, Z.-W., Peng, F., Wang, H.-J., Yu, H., Zheng, W.-X., and Yang, J. (2010). Phosphorus-doped graphite layers with high electrocatalytic activity for the O2 reduction in an alkaline medium. Angew. Chem. Int. Ed. 50, 3257–3261. doi: 10.1002/anie.201006768
Martins, A. C., Huang, X., Goswami, A., Koh, K., Meng, Y., Almeida, V. C., et al. (2016). Fibrous porous carbon electrocatalysts for hydrazine oxidation by using cellulose filter paper as precursor and self-template. Carbon 102, 97–105. doi: 10.1016/j.carbon.2016.02.028
Meng, Y., Voiry, D., Goswami, A., Zou, X., Huang, X., Chhowalla, M., et al. (2014a). N-, O-, and S-tridoped nanoporous carbons as selective catalysts for oxygen reduction and alcohol oxidation reactions. J. Am. Chem. Soc. 136, 13554–13557. doi: 10.1021/ja507463w
Meng, Y., Zou, X., Huang, X., Goswami, A., Liu, Z., and Asefa, T. (2014b). Polypyrrole-derived nitrogen and oxygen co-doped mesoporous carbons as efficient metal-free electrocatalyst for hydrazine oxidation. Adv. Mater. 26, 6510–6516. doi: 10.1002/adma.201401969
Munoz-Sandoval, E., Cortes-López, A. J., Flores-Gómez, B., Fajardo-Díaz, J. L., Sánchez-Salas, R., and López-Urías, F. (2017). Carbon sponge-type nanostructures based on coaxial nitrogen-doped multiwalled carbon nanotubes grown by CVD using benzylamine as precursor. Carbon 115, 409–421. doi: 10.1016/j.carbon.2017.01.010
Ouyang, T., Ye, Y.-Q., Wu, C.-Y., Xiao, K., and Liu, Z.-Q. (2019). Heterostructures composed of N-doped carbon nanotubes encapsulating cobalt and β-Mo2C nanoparticles as bifunctional electrodes for water splitting. Angew. Chem. Int. Ed. 58, 4923–4928. doi: 10.1002/anie.201814262
Paraknowitsch, J. P., Thomasa, A., and Antonietti, M. (2010a). A detailed view on the polycondensation of ionic liquid monomers towards nitrogen doped carbon materials. J. Mater. Chem. 20, 6746–6758. doi: 10.1039/C0JM00869A
Paraknowitsch, J. P., Wienert, B., Zhang, Y., and Thomas, A. (2012). Intrinsically sulfur- and nitrogen-co-doped carbons from thiazolium salts. Chem. Eur. J. 18, 15416–15423. doi: 10.1002/chem.201202445
Paraknowitsch, J. P., Zhang, J., Su, D., Thomas, A., and Antonietti, M. (2010b). Ionic liquids as precursors for nitrogen-doped graphitic carbon. Adv. Mater. 22, 87–92. doi: 10.1002/adma.200900965
Paraknowitsch, J. P., Zhang, Y., Wienerta, B., and Thomas, A. (2013). Nitrogen- and phosphorus-co-doped carbons with tunable enhanced surface areas promoted by the doping additives. Chem. Commun. 49:1208. doi: 10.1039/C2CC37398J
Qu, L., Liu, Y., Baek, J.-B., and Dai, L. (2010). Nitrogen-doped graphene as efficient metal-Free electrocatalyst for oxygen reduction in fuel cells. ACS Nano 4, 1321–1326. doi: 10.1021/nn901850u
Rosca, V., and Koper, M. T. M. (2008). Electrocatalytic oxidation of hydrazine on platinum electrodes in alkaline solutions. Electrochim. Acta 53, 5199–5205. doi: 10.1016/j.electacta.2008.02.054
Sanabria-Chinchilla, J., Asazawa, K., Sakamoto, T., Yamada, K., Tanaka, H., and Strasser, P. (2011). Noble metal-free hydrazine fuel cell catalysts: EPOC effect in competing chemical and electrochemical reaction pathways. J. Am. Chem. Soc. 133, 5425–5431. doi: 10.1021/ja111160r
Sathe, B. R., Zou, X., and Asefa, T. (2014). Metal-free B-doped graphene with efficient electrocatalytic activity for hydrogen evolution reaction. Catal. Sci. Technol. 4, 2023–2030. doi: 10.1039/C4CY00075G
Sharifi, T., Hu, G., Jia, X., and Wågberg, T. (2012). Formation of active sites for oxygen reduction reactions by transformation of nitrogen functionalities in nitrogen-doped carbon nanotubes. ACS Nano 6, 8904–8912. doi: 10.1021/nn302906r
Shi, Y., and Zhang, B. (2016). Recent advances in transition metal phosphide nanomaterials: synthesis and applications in hydrogen evolution reaction. Chem. Soc. Rev. 45, 1529–1541. doi: 10.1039/C5CS00434A
Shrestha, S., and Mustain, W. E. (2010). Properties of nitrogen-functionalized ordered mesoporous carbon prepared using polypyrrole precursor. J. Electrochem. Soc. 157, B1665–B1672. doi: 10.1149/1.3489412
Silva, R., Voiry, D., Chhowalla, M., and Asefa, T. (2013). Efficient metal-free electrocatalysts for oxygen reduction: polyaniline-derived N- and O-doped mesoporous carbons. J. Am. Chem. Soc. 135, 7823–7826. doi: 10.1021/ja402450a
Silva, R, Al-Sharab, J., and Asefa, T. (2012). Edge-plane-rich nitrogen-doped carbon nanoneedles and efficient metal-free electrocatalysts. Angew. Chem. Int. Ed. 51, 7171–7175. doi: 10.1002/anie.201201742.
Subbaraman, R., Tripkovic, D., Chang, K.-C., Strmcnik, D., Paulikas, A. P., Hirunsit, P., et al. (2012). Trends in activity for the water electrolyser reactions on 3d M (Ni, Co, Fe, Mn) hydr(oxy)oxide catalysts. Nat. Mater. 11, 550–557. doi: 10.1038/NMAT3313
Tang, Y., Allen, B. L., Kauffman, D. R., and Star, A. (2009). Electrocatalytic activity of nitrogen-doped carbon nanotube cups. J. Am. Chem. Soc. 131, 13200–13201. doi: 10.1021/ja904595t
Terrones, M., Redlich, P., Grobert, N., Trasobares, S., Hsu, W.-K., Terrones, H., et al. (1999). Carbon nitride nanocomposites: formation of aligned CxNy nanofibers. Adv. Mater. 11, 655–658. doi: 10.1002/(SICI)1521-4095(199906)11:8<655::AID-ADMA655>3.0.CO;2-6
Terrones, M., and Terrones, H. (1999). Efficient route to large arrays of CNx nanofibers by pyrolysis of ferrocene/melamine mixtures. Appl. Phys. Lett. 75, 3932–3934. doi: 10.1063/1.125498
Vinu, A., Anandan, S., Anand, C., Srinivasu, P., Ariga, K., and Mori, T. (2008). Fabrication of partially graphitic three-dimensional nitrogen-doped mesoporous carbon using polyaniline nanocomposite through nanotemplating method. Micropor. Mesopor. Mater. 109, 398–404. doi: 10.1016/j.micromeso.2007.05.037
Wang, J.-Y., Ouyang, T., Deng, Y.-P., Hong, Y.-S., and Liu, Z.-Q. (2019). Metallic Mo2C anchored pyrrolic-N induced N-CNTs/NiS2 for efficient overall water electrolysis, J. Power Sources 420, 108–117. doi: 10.1016/j.jpowsour.2019.02.098
Wang, J.-Y., Ouyang, T., Li, N., Ma, T., and Liu, Z.-Q. (2018). S, N co-doped carbon nanotube-encapsulated core-shelled CoS2@Co nanoparticles: efficient and stable bifunctional catalysts for overall water splitting. Sci. Bull. 63, 1130–1140. doi: 10.1016/j.scib.2018.07.008
Wang, S., Iyyamperumal, E., Roy, A., Xue, Y., Yu, D., and Dai, L. (2011). Vertically aligned BCN nanotubes as efficient metal-free electrocatalysts for the oxygen reduction reaction: a synergetic effect by co-doping with boron and nitrogen. Angew. Chem. Int. Ed. 50, 11756–11760. doi: 10.1002/anie.201105204
Wang, X., and Dai, S. (2010). Ionic liquids as versatile precursors for functionalized porous carbon and carbon-oxide composite materials by confined carbonization. Angew. Chem. Int. Ed. 49, 6664–6668. doi: 10.1002/anie.201003163
Wang, X.-T., Ouyang, T., Wang, L., Zhong, J.-H., Ma, T., and Liu, Z.-Q. (2019). Redox-inert Fe3+ ions in octahedral sites of Co-Fe spinel oxides with enhanced oxygen catalytic activity for rechargeable zinc-air batteries. Angew.Chem. Int. Ed. 131, 13425–13430. doi: 10.1002/anie.201907595
Wang, Y., Wan, Y., and Zhang, D. (2010). Reduced graphene sheets modified glassy carbon electrode for electrocatalytic oxidation of hydrazine in alkaline media. Electrochem. Commun. 12, 187–190. doi: 10.1016/j.elecom.2009.11.019
Wiggins-Camacho, J. D., and Stevenson, K. J. (2009). Effect of nitrogen concentration on capacitance, density of states, electronic conductivity, and morphology of N-doped carbon nanotube electrodes. J. Phys. Chem. C 113, 19082–19090. doi: 10.1021/jp907160v
Wohlgemuth, S.-A., White, R. J., Willinger, M.-G., Titirici, M.-M., and Antonietti, M. (2012). A one-pot hydrothermal synthesis of sulfur and nitrogen doped carbon aerogels with enhanced electrocatalytic activity in the oxygen reduction reaction. Green Chem. 14, 1515–1523. doi: 10.1039/C2GC35309A
Xia, Y., and Mokaya, R. (2004). Synthesis of ordered mesoporous carbon and nitrogen-doped carbon materials with graphitic pore walls via a simple chemical vapor deposition method. Adv. Mater. 16, 1553–1558. doi: 10.1002/adma.200400391
Xu, L., Jiang, Q., Xiao, Z., Li, X., Huo, J., Wang, S., et al. (2016). Plasma-engraved Co3O4 nanosheets with oxygen vacancies and high surface area for the oxygen evolution reaction. Angew. Chem. Int. Ed. 55, 5277–5281. doi: 10.1002/anie.201600687
Yamada, K., Yasuda, K., Fujiwara, N., Siroma, Z., Tanaka, H., Miyazaki, Y., et al. (2003). Potential application of anion-exchange membrane for hydrazine fuel cell electrolyte. Electrochem. Commun. 5, 892–896. doi: 10.1016/j.elecom.2003.08.015
Yan, Y., Xia, B. Y., Xu, Z., and Wang, X. (2014). Recent development of molybdenum sulfides as advanced electrocatalysts for hydrogen evolution reaction. ACS Catal. 4, 1693–1705. doi: 10.1021/cs500070x
Yang, D.-S., Bhattacharjya, D., Inamdar, S., Park, J., and Yu, J.-S. (2012). Phosphorus-doped ordered mesoporous carbons with different lengths as efficient metal-free electrocatalysts for oxygen reduction reaction in alkaline media. J. Am. Chem. Soc. 134, 16127–16130. doi: 10.1021/ja306376s
Yang, G.-W., Gao, G.-Y., Wang, C., Xu, C.-L., and Li, H.-L. (2008). Controllable deposition of Ag nanoparticles on carbon nanotubes as a catalyst for hydrazine oxidation. Carbon 46, 747–752. doi: 10.1016/j.carbon.2008.01.026
Yang, L., Jiang, S., Zhao, Y., Zhu, L., Chen, S., Wang, X., et al. (2011). Boron-doped carbon nanotubes as metal-free electrocatalysts for the oxygen reduction reaction. Angew. Chem. Int. Ed. 50, 7132–7135. doi: 10.1002/anie.201101287
Yang, S., Zhi, L., Tang, K., Feng, X., Maier, J., and Müllen, K. (2012). Efficient synthesis of heteroatom (N or S)-doped graphene based on ultrathin graphene oxide-porous silica sheets for oxygen reduction reactions. Adv. Funct. Mater. 22, 3634–3640. doi: 10.1002/adfm.201200186
Ye, W., Yang, B., Cao, G., Duan, L., and Wang, C. (2008). Electrocatalytic oxidation of hydrazine compound on electroplated Pd/WO3 film. Thin Solid Films 516, 2957–2961. doi: 10.1016/j.tsf.2007.10.096
Yi, Q., and Yu, W. (2009). Nanoporous gold particles modified titanium electrode for hydrazine oxidation. J. Electroanal. Chem. 633, 159–164. doi: 10.1016/j.jelechem.2009.05.008
Zhang, J., Zhao, Z., Xia, Z., and Dai, L. (2015). A metal-free bifunctional electrocatalyst for oxygen reduction and oxygen evolution reactions. Nat. Nanotech. 10, 444–452. doi: 10.1038/NNANO.2015.48
Zhao, Y., Nakamura, R., Kamiya, K., Nakanishi, S., and Hashimoto, K. (2013). Nitrogen-doped carbon nanomaterials as non-metal electrocatalysts for water oxidation. Nat. Commun. 4, 2390–2396. doi: 10.1038/ncomms3390
Zhao, Y.-D., Zhang, W.-D., Chen, H., and Luo, Q.-M. (2002). Anodic oxidation of hydrazine at carbon nanotube powder microelectrode and its detection. Talanta 58, 529–534. doi: 10.1016/S0039-9140(02)00318-1
Keywords: carbon-based nanomaterials, fuel cell, water splitting, energy-related reactions, doping effect
Citation: Meng Y, Huang X, Lin H, Zhang P, Gao Q and Li W (2019) Carbon-Based Nanomaterials as Sustainable Noble-Metal-Free Electrocatalysts. Front. Chem. 7:759. doi: 10.3389/fchem.2019.00759
Received: 08 August 2019; Accepted: 22 October 2019;
Published: 12 November 2019.
Edited by:
Hao Yu, South China University of Technology, ChinaReviewed by:
Zhao-Qing Liu, Guangzhou University, ChinaYuefeng Liu, Chinese Academy of Sciences, China
Copyright © 2019 Meng, Huang, Lin, Zhang, Gao and Li. This is an open-access article distributed under the terms of the Creative Commons Attribution License (CC BY). The use, distribution or reproduction in other forums is permitted, provided the original author(s) and the copyright owner(s) are credited and that the original publication in this journal is cited, in accordance with accepted academic practice. No use, distribution or reproduction is permitted which does not comply with these terms.
*Correspondence: Yuying Meng, bWVuZ3l1eSYjeDAwMDQwO2pudS5lZHUuY24=; Qingsheng Gao, dHFzZ2FvJiN4MDAwNDA7am51LmVkdS5jbg==