- 1Graphene Research Institute, Sejong University, Seoul, South Korea
- 2Department of Nano and Advanced Materials Engineering, Sejong University, Seoul, South Korea
- 3Division of Electronics and Electrical Engineering, Dongguk University-Seoul, Seoul, South Korea
- 4Department of Physics, Sejong University, Seoul, South Korea
- 5Thin Film Materials Research Center, Korea Research Institute of Chemical Technology, Daejeon, South Korea
The synthesis of low cost, high efficacy, and durable hydrogen evolution electrocatalysts from the non-noble metal group is a major challenge. Herein, we establish a simple and inexpensive chemical reduction method for producing molybdenum carbide (Mo2C) and tungsten carbide (W2C) nanoparticles that are efficient electrocatalysts in alkali and acid electrolytes for hydrogen evolution reactions (HER). Mo2C exhibits outstanding electrocatalytic behavior with an overpotential of −134 mV in acid medium and of −116 mV in alkaline medium, while W2C nanoparticles require an overpotential of −173 mV in acidic medium and −130 mV in alkaline medium to attain a current density of 10 mA cm−2. The observed results prove the capability of high- and low-pH active electrocatalysts of Mo2C and W2C nanoparticles to be efficient systems for hydrogen production through HER water electrolysis.
Introduction
A direct and effective route to clean and renewable hydrogen (H2) production by water splitting requires a robust catalyst to ensure sustainable efficiency (Vikraman et al., 2017). Platinum (Pt)-based systems are recognized as highly energetic HER catalysts that boast various pH tolerances and almost zero overpotential, but their high cost, originating from the scarcity of platinum, severely hinders their extensive use (Jacobsson et al., 2013; Peng et al., 2014). To reduce the use of Pt, intensive research has been conducted to prepare low-cost, highly active, and electrochemically stable HER electrocatalysts comprised of abundant elements as an alternative (Vikraman et al., 2018; Hussain et al., 2019a). Numerous types of non-scarce transition metal compounds, such as layered transition metal dichalcogenides (LTMDs) (Laursen et al., 2012; Seok et al., 2017; Hussain et al., 2018), phosphides (Lv and Wang, 2017; Pei et al., 2018), transition metal carbides (TMC) (Huang et al., 2016; Shi et al., 2017), and their composites (Ren et al., 2018; Vikraman et al., 2019b) have been shown to produce excellent HER activities because of their unique electronic structures. Various molybdenum selenide (MoSe2)-based materials, in particular, have been reported as being suited for replacing Pt in HER electrocatalysts (Ren et al., 2018; Hussain et al., 2019a; Vikraman et al., 2019a). In addition, attaching Mo2C and W2C to a carbon matrix has been shown to produce high-rate charge transfer properties during HER and to alleviate surface aggregation (Pan et al., 2014; Youn et al., 2014; Wu et al., 2016). Previous reports suggested that the HER electrocatalytic performance of Mo2C- and W2C-based catalysts mainly results from the morphology (Ang et al., 2016; Ishii et al., 2016; Peng et al., 2017), crystalline phases (Wan et al., 2014; Lin et al., 2017), and composition (Yu et al., 2018; Zhang et al., 2018) of the catalysts and the synthetic protocol. However, the critical challenge is to design and develop carbide-based catalysts with comparable catalytic performance to Pt for practical applications. Until now, carbide-based materials have shown inferior catalytic properties due to their poor activities (Wu et al., 2016; Yu et al., 2018; Zhang et al., 2018). An earlier study reported the HER behavior of commercially available Mo2C meshes in both basic and acidic solutions, showing a comparatively high overpotential of −190~-230 mV at a cathodic current of 10 mAcm−2 (Vrubel and Hu, 2012). Performance has since been upgraded by tuning its nanocrystal size (Ma R. et al., 2015; Chen et al., 2016). Hence, Mo2C and W2C nanoparticles with high catalytic activity and robustness remain open for future research.
Various methods have been employed to prepare TMC-based catalysts (Wu et al., 2016; Zhu et al., 2016; An and Xu, 2019). Gong et al. obtained WCx/MoCx-based electrocatalysts by carbonizing W/WO3 or Mo/MoO3 under the flow of carbon precursors (C2H6, CH4, or CO, and H2), but very low surface areas were obtained (Gong et al., 2016). Tantalum carbide nanocrystals have been prepared via a refined Cl2-assisted “micro-cutting-fragmentation” approach and produced an overpotential of ~-146 mV@10 mA cm−2 for HER (Kou et al., 2017). Different methodologies have been used to prepare Mo2C and its hybrids for HER applications (Pu et al., 2016; Kou et al., 2018; Liang et al., 2019). Tungsten carbides can exist in different phases, such as WC, metastable W2C, and WC1−x, but during the last decade, most researchers have exclusively focused on the WC phase instead of the W2C phase (Neylon et al., 1999; Ishii et al., 2016). W/WC synthesized by Kou et al. (2019) showed an overpotential of 159 mV @ 10 mA cm−2. As per experimental and theoretical calculations, the W2C phase exhibits more HER active catalytic properties than does WC, with a low negative Gibbs free energy (ΔGH) for hydrogen adsorption and high Fermi level electronic density of states (DOS) (Colton et al., 1975; Gong et al., 2016). β-phase Mo2C and the W2C phase, in particular, have been validated as effective HER electrocatalysts despite their bulky natures, and their activity could be further increased by engineering with appropriate nanosize structures (Huang et al., 2016).
Herein, we have successfully synthesized Mo2C and W2C nanoparticles for HER application via a simple and economical chemical reduction method. The electrochemical results indicate that Mo2C nanoparticles exhibit superior HER electrocatalytic behavior over W2C in both alkaline and acid solutions, with small Tafel slopes and distinct solidity. We believe that our present investigation provides effective strategies for designing and preparing the new nanostructured TMC-based HER catalysts.
Results and Discussion
The chemically reduced synthesis scheme and the atomic structures of the Mo2C and W2C nanoparticles are pictorially represented in Figure 1. Raman characterization was performed for structural confirmation of commercial and chemically reduced Mo2C and W2C nanoparticles. Figure 2A shows the Raman spectra of commercial Mo2C and W2C and chemically reduced Mo2C and W2C. The commercial Mo2C revealed unclear peaks, whereas the chemically reduced Mo2C produced distinctive peaks at 661, 818, and 990 cm−1, credited to β-Mo2C (Hussain et al., 2019b). In addition, the G band was positioned at 1,582 cm−1 and the D band was positioned at 1,349 cm−1, which corresponds to the sp2− bonded carbon atoms and disarranged graphite carbon, respectively (Ma F. et al., 2015). The intensity ratio of the D-band to the G-band (i.e., ID/IG) is 0.85 for Mo2C, comparable to long-time carbonized graphene layers with high conductivity, which may facilitate charge transfer during HER (Li et al., 2016; Lv et al., 2017; Wang et al., 2017). For commercial and chemically reduced W2C, the strong peaks observed at 693 and 807 cm−1 originate from the stretching vibration of W-C (Yan et al., 2017). The D and G bands were exhibited at 1,351 and 1,583 cm−1 for the chemically reduced W2C (Yan et al., 2017; Zhang et al., 2018). The observed results are in good agreement with previous reports (Yan et al., 2017; Hussain et al., 2019a).
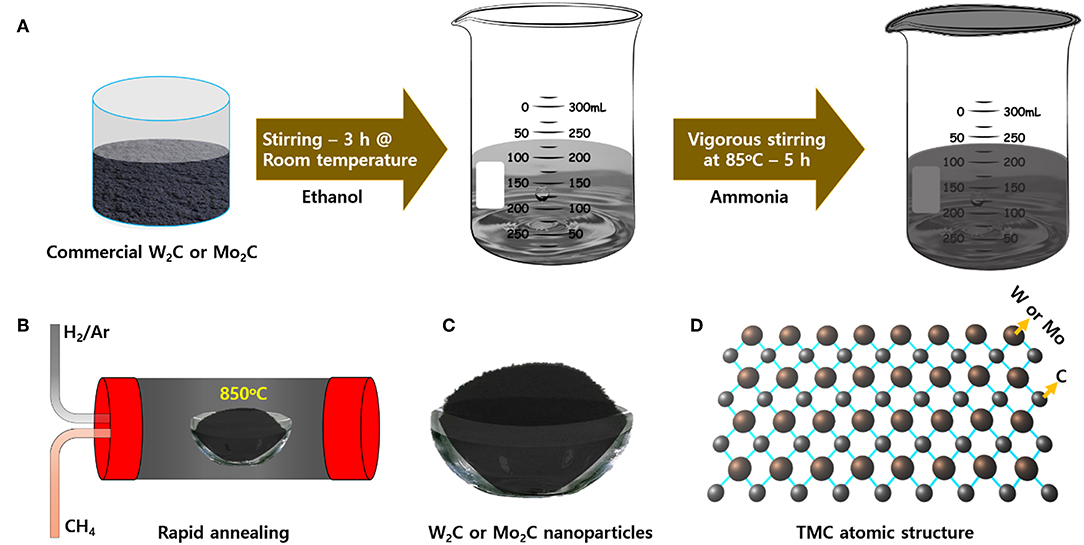
Figure 1. Schematic representation of the (A) chemically reduction, (B) thermal annealing at 850°C, (C) prepared powders, and (D) atomic structure of Mo2C and W2C nanoparticles.
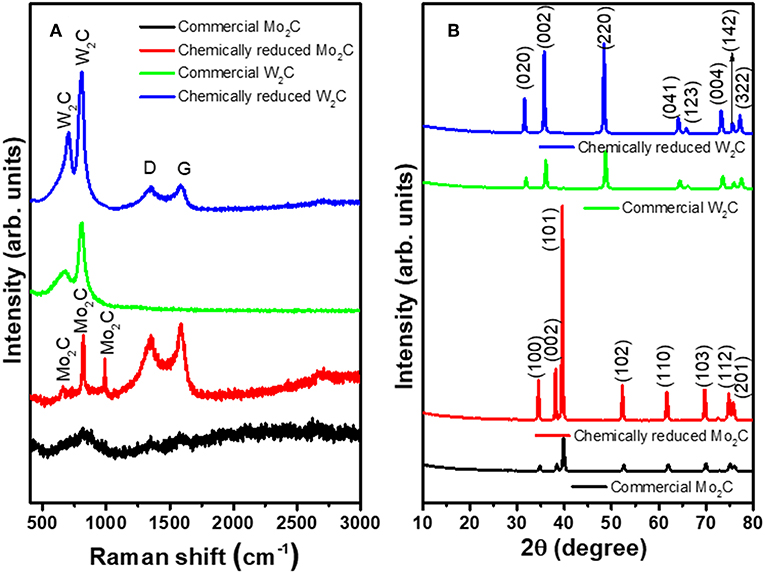
Figure 2. (A) XRD patterns and (B) Raman spectra of commercial Mo2C and W2C and chemically reduced Mo2C and W2C.
X-ray diffraction (XRD) was investigated to reveal the material structure of the commercial and chemical Mo2C and W2C nanoparticles (Figure 2B). For Mo2C, the XRD patterns revealed reflections at 34.5, 38.1, 39.5, 52.3, 61.7, 69.6, 74.7, and 75.7°, which were indexed to the (100), (002), (101), (102), (110), (103), (112), and (201) planes of β-Mo2C (JCPDS: 35-0787), which is the most active phase of Mo2C for HER. In the case of W2C, the XRD patterns produced peaks at 31.5, 35.1, 48.8, 64.1, 65.8, 73.2, 75.6, and 77.1° due to the (020), (002), (220), (041), (123), (004), (142), and (322) lattice planes, respectively (JCPDS: 89-2371). Compared with commercial samples, the chemically reduced samples showed higher-intensity peaks, which can be attributed to the enriched crystallinity in the nanoparticles compared to in bulk (Hussain et al., 2019a). No discernible peaks in either the Mo2C or W2C samples could be assigned to Mo/W, carbide, or other Mo/W non-stoichiometric phases and impurities, indicating the capability of the synthetic method to prepare effective nanostructures from bulk.
Morphology and microstructure modifications were inspected using field emission scanning electron microscopy (FESEM) and transmission electron microscopy (TEM). Figures 3a–c show FESEM images for reduced Mo2C nanoparticles. The observed images clearly indicated that larger sized grains were constructed through the accumulation of spherically shaped nanoparticles. The composition of reduced Mo2C nanoparticles was examined by energy dispersive spectroscopy (EDS), as shown in Figure S1. The observed composition of the chemically reduced sample is in good agreement with the claim of Mo2C formation, and EDS mapping images for the nanoparticles are provided in Figure S2. TEM images at different magnifications of Mo2C nanoparticles are provided in Figures 3d–g. The large grains are clearly seen in the lower-magnification TEM image (Figure 3d), and the typical 2D layered structures are shown in the higher-magnification images. A high-resolution TEM image showing a fingerprint-like structure is shown in Figure 3g, and its fast Fourier transform (FFT) and inverse FFTs are inserted. The intensity profile extracted proved the existence of (101) lattice plane spacing (0.228 nm) between the fringes, as shown in Figure 3h. Hence, the microscopic images clearly proved the formation of Mo2C nanoparticles.
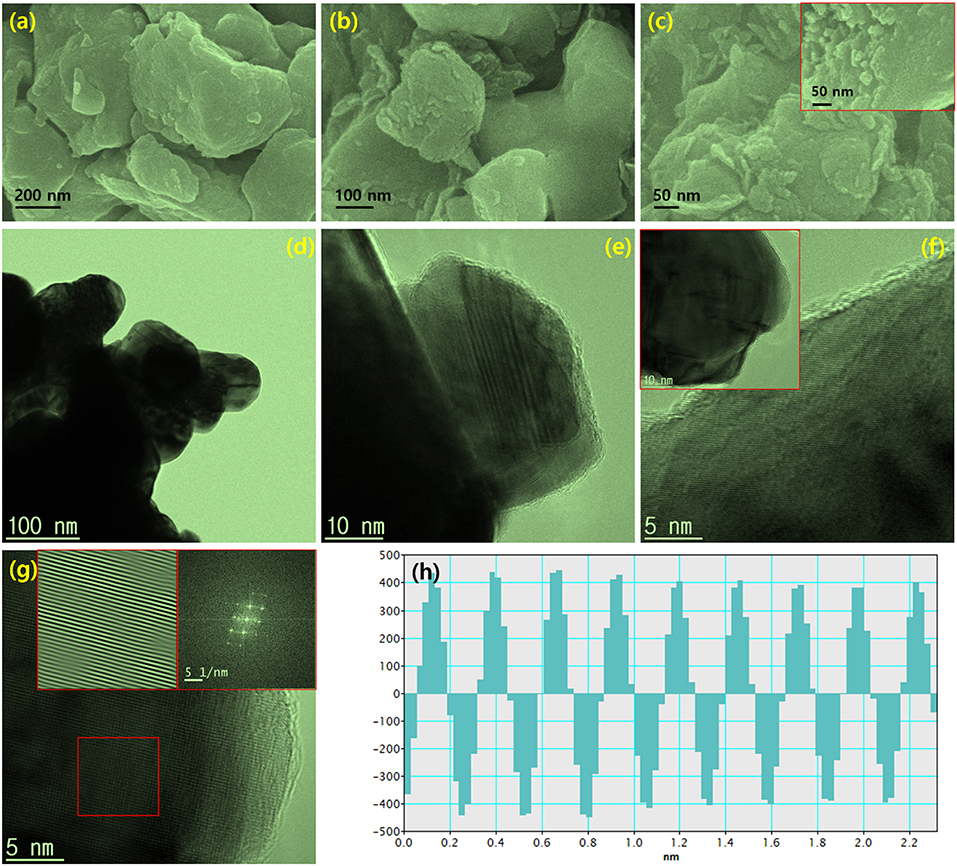
Figure 3. (a–c) FESEM images at different magnifications for chemically reduced Mo2C nanoparticles, (d–h) TEM micrographs of Mo2C nanoparticles, (d–f) Different magnifications of TEM images of dispersed nanosheet structures, (g) High-resolution TEM image with the typical fingerprint-type layered structure, with insets of FFT and inverse FFT patterns obtained via point mask mode, and (h) intensity profile.
Figure 4 shows FESEM and TEM images of chemically reduced W2C nanoparticles. The nanoparticles consist of inhomogeneous grains (Figures 4a–c). The EDS spectrum confirmed the existence of W2C nanoparticles (Figure S3), and their mapping images further supported the claim of W2C nanoparticle formation (Figure S4). The TEM images provided valuable insights regarding the W2C nanoparticles (Figures 4d–g). The irregularly sized nanograins were clearly seen in the low- and higher-magnification TEM images (Figures 4f,g). The FFT image showed the (002) W2C plane (0.26 nm spacing) (Figure 4h). The observed results are well-correlated with the XRD results.
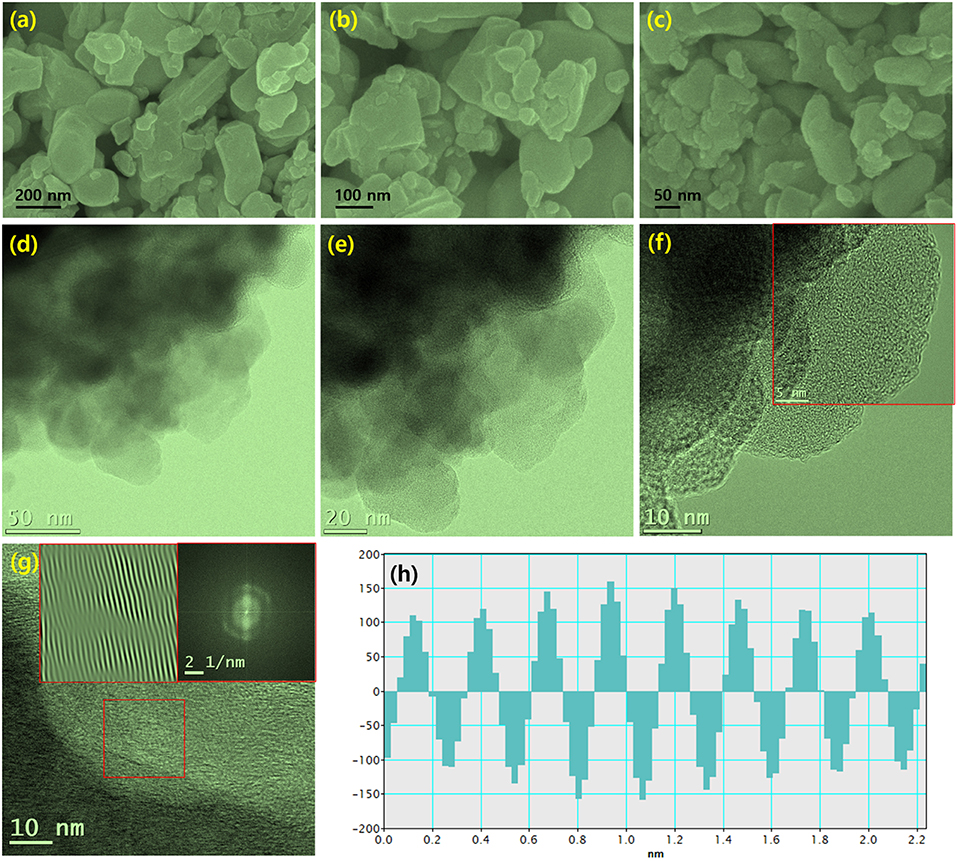
Figure 4. (a–c) FESEM images of different magnification for chemically reduced W2C nanoparticles, (d–h) TEM micrographs of W2C nanoparticles (d–e) Different magnifications of TEM images of accumulated nanosheet structures, (f,g) high-resolution TEM images of interconnected layers with a fingerprint structure, with an inset showing the inverse FFT pattern obtained via point mask mode, and (h) intensity profile.
An X-ray photoelectron spectroscopy (XPS) study was conducted to validate the composition of reduced Mo2C and W2C. The XPS survey spectra revealed the occurrence of Mo2C (C and Mo)- and W2C (C and W)-based elements, as shown in Figures S5A,B. Figure 5A shows the de-convoluted high-resolution region for Mo 3d, with the Mo 3d5/2 and Mo 3d3/2 peaks at 228.6 and 231.9 eV, which reveals the carbidic Mo phase. The peaks at 233.3 and 235.9 eV are from molybdenum oxide (Mo6+), indicating surface oxidation in an air environment (Cui et al., 2014; Tang et al., 2015; Huang et al., 2016; Fan et al., 2018; Hussain et al., 2019a). The C 1s spectrum (Figure 5B) at a 284.8 eV binding energy is characteristic of the sp2 carbon relation in Mo2C, whereas a satellite peak emerges at 288.8 eV due to C–O bonding (Wu et al., 2016). Figure 5C shows the high-resolution W 4f XPS spectrum from chemically reduced W2C. The deconvoluted peaks revealed at 31.64 and 33.81 eV were credited to 4f7/2 and 4f5/2, respectively, for the W 4f atom. The high-resolution C 1s spectrum (Figure 5D) of W2C exposed the sp2 graphitic carbon peak at 283.02 eV and C = O peak at 285.1 eV (Berglund et al., 2014; Ko et al., 2017). The observed results confirmed the formation of Mo2C and W2C, which is in good agreement with the earlier literature (Ma F. et al., 2015; Yan et al., 2017). The surface area modifications were measured by the nitrogen (N2) adsorption/desorption isotherms for chemically reduced Mo2C and W2C nanoparticles via the Brunauer Emmett Teller (BET) method (Figures S6A,B). Surface area of 0.91 and 1.75 m2·g−1 were observed for the chemically reduced Mo2C and W2C, respectively, compared with the reported values of their commercial samples (Gao et al., 2014; Hussain et al., 2019a). In addition, the pore diameter vs. pore volume profile (Figure S6B) shows the mesoporous nature of chemically reduced Mo2C and W2C, with pore diameters of 14.9 and 21.4 nm and pore volumes of 0.003 and 0.009 cm3·g−1, respectively.
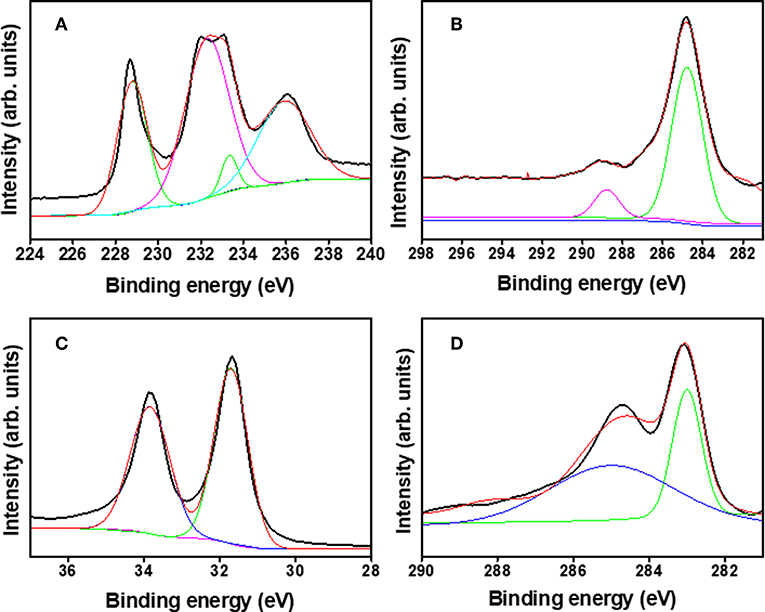
Figure 5. X-ray photoemission spectra: (A) Mo 3d, (B) C 1s binding energy spectra of Mo2C nanoparticles, (C) W 4f, and (D) C 1s binding energy spectra of W2C nanoparticles.
The HER electrocatalytic activities of Mo2C- and W2C-coated nickel foam (NF) electrodes were analyzed by linear sweep voltammogram (LSV) in 1 M KOH and 0.5 M H2SO4 electrolyte solutions (scan rate of 10 mV s−1, three-electrode setup). For the evaluation, a commercial Pt/C was used as an electrode, and the results were as follows. Figure 6A shows the LSV curves of commercial Pt/C and reduced Mo2C and W2C catalysts in a 0.5 M H2SO4 solution. As anticipated, the commercial Pt/C catalyst showed the highest HER activity, which had an overpotential of ~-49 mV. For bare NF, Mo2C, and W2C, overpotentials of −423, −134, and −170 mV were needed to achieve 10 mAcm−2 in acidic electrolyte, respectively, lower than those of the commercial samples (Vrubel and Hu, 2012; Chen et al., 2013). The overpotentials of the chemically reduced Mo2C and W2C were much closer to the recently reported values for carbide-based materials in acid medium, such as Mo2C encapsulated by nitrogen and phosphorus codoped carbon shells (@-260 mV) (Li et al., 2016), Mo2C nanoparticles embedded in chain-like Ketjenblack carbon (@-221 ~263 mV) (Wang et al., 2017), porous MoCx nano octahedrons (@-142 mV) (Wu et al., 2015), 3D porous scaffold-like Mo2C/C nanosheet hybrids (@-233 mV) (Wang et al., 2018), reduced graphene oxide-based Mo2C composites (@-206 mV) (Ojha et al., 2016), Mo2C nanoparticle-decorated graphitic carbon sheets (@-200~210 mV) (Cui et al., 2014), Mo2C particles embedded in a sulfur and nitrogen codoped mesoporous carbon matrix (@-146 mV) (An et al., 2017), and Mo2C NCs on vertically aligned graphene (@-152 mV) (Fan et al., 2017). The electrocatalytic properties of Pt/C, Mo2C, and W2C catalysts were appraised in a 1 M KOH medium (Figure 6B). Similarly, the Pt/C, Mo2C, and W2C electrocatalysts produced −48, −116, and −130 mV overpotentials to drive the 10 mAcm−2 reaction in an alkaline electrolyte. The bare NF did not produce viable HER properties in a 1 M KOH medium. The observed results constitute a considerable advance over earlier results.
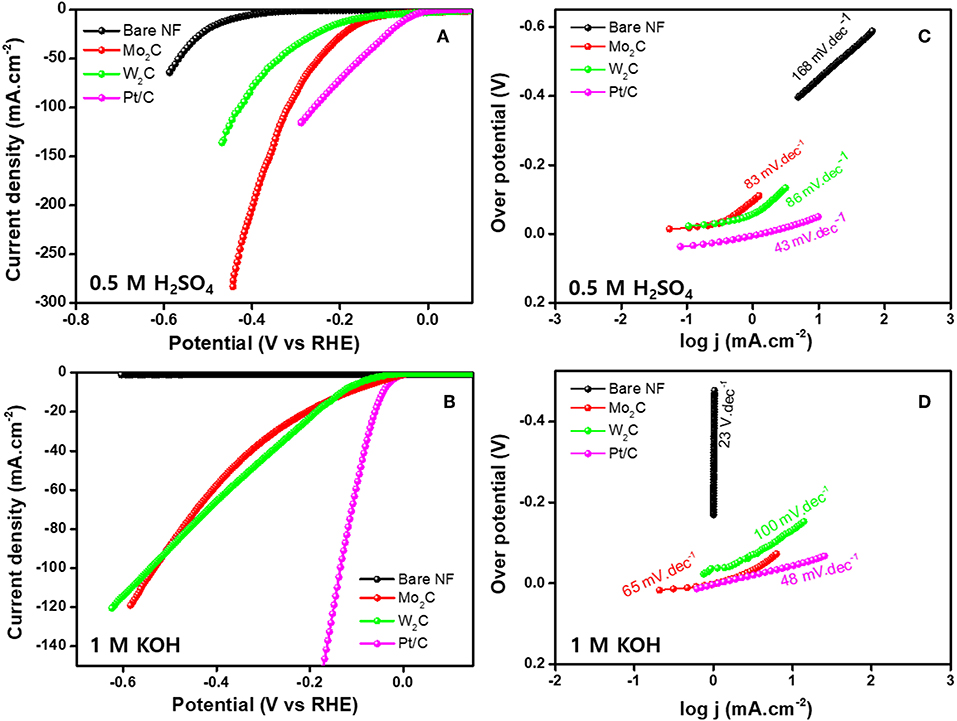
Figure 6. HER performance of bare NF, Pt/C, Mo2C, and W2C nanoparticle-decorated NF. (A,C) LSV profiles and their Tafel plots in 0.5 M H2SO4 and (B,D) LSV profiles and their Tafel plots in 1.0 M KOH.
The Tafel slope signifies the characteristic activity acquired of an electrocatalyst and is derived using the Tafel equation for the HER. As shown in Figures 6C,D, Pt/C offers Tafel slopes of 43 and 48 mV.dec−1 for acidic and alkaline electrolytes, respectively, which is comparable with the reported results (Fan et al., 2018). The extracted slope values from the Tafel plots were 83 and 86 mV dec−1 for the acidic and 65 and 100 mV.dec−1 for the alkali for reduced Mo2C and W2C, respectively. These values indicate that the Volmer-Heyrovsky reaction step is obeyed for the whole HER process (Bockris and Potter, 1952; Yuan et al., 2018). Another important parameter, the exchange current density (j0), was extracted to evaluate HER activity by extrapolating the linear region of the Tafel plot. A chemically reduced Mo2C catalyst displays j0 values of 0.846 and 0.131 mA cm−2 in alkali and acidic media, respectively, while a chemically reduced W2C catalyst shows j0 values of 0.438 and 0.194 mA cm−2. The observed parameters are provided in Table 1. A detailed comparison of chemically reduced Mo2C and W2C HER parameters, with the reported carbide-based materials in alkaline and acidic media, are provided in Tables S1, S2, respectively.
The observed HER properties are credited to the reduced sizes of Mo2C and W2C nanostructures and their improved morphological properties in terms of edge sites.
High electrical conductivity allows for rapid ion/electron transfer between the active electrocatalyst edge sites and also provides a high interaction area between the electrolyte and the electrode, which in turn enhances the electrochemical performance. The charge transport mechanism was clarified by employing electrochemical impedance spectroscopy (EIS) studies to understand the HER mechanism of Mo2C and W2C nanoparticles at the interface between electrodes and electrolytes. The charge transfer properties with resistance (Rct) are exhibited for Pt/C in acidic and alkaline media as shown in Figures S7A,B. The lower Rct values for chemically reduced Mo2C and W2C in acidic and alkaline media confirmed that they favor rapid electron transport in H2 evolution (Figures S7A,B). We estimated the electrochemically active surface area (ECSA) through cyclic voltammetry (CV), which was accomplished at different scan rates from 10 to 100 mVs−1 in the non-Faradaic regions for Mo2C and W2C, as shown in Figures S8A–D, respectively (Zhou et al., 2016; Hussain et al., 2019a; Vikraman et al., 2019a). The double-layer capacitance (Cdl) was extracted from the fitted slope value of the current differences (Δja−c) of the cathodic and anodic peaks of CV profiles at 0.24 V vs. RHE (Figure S8E). The extracted Cdl values were 2.13 and 1.42 mF.cm−2 in acid medium and 2.90 and 1.86 mF.cm−2 in alkaline medium for Mo2C and W2C, respectively. The ECSA values were assessed through a previously described procedure (Vikraman et al., 2019a) and were 60.8 and 40.6 cm2 in an acidic and 72.5 and 46.5 cm2 in a alkaline medium for Mo2C and W2C, respectively, indicating higher HER activity for the Mo2C system. We estimated the amount of surface active sites using the method reported by Fei et al. (2015). The turnover frequency (TOF) values of Mo2C and W2C were calculated in the acidic and alkaline media. The TOF values of Mo2C are 0.005 and 0.037 H2.s−1 at overpotentials of −134 and −116 mV, and those of W2C are 0.015 and 0.034 H2.s−1 at overpotentials of −173 and −130 mV in acidic and alkaline media, respectively. The observed TOF values are comparable with previous reports (Chen et al., 2011; Fei et al., 2015; Ma L. et al., 2015; Zhang et al., 2017).
The robustness of the Mo2C and W2C catalysts was analyzed by carrying out chronoamperometric performances in 0.5 M H2SO4 and in 1 M KOH for 20 h; the results are shown in Figures 7A–D. The LSV profiles of Mo2C and W2C catalysts were examined after 20 h of HER operation (−0.6 to 0.15 V vs. RHE at a scan rate of 10 mV s−1) in alkaline and acid media (Figure 7). The polarization curves revealed the robust nature of Mo2C after 20 h of working operation, whereas W2C showed slight degradation. Hence, the observed deliverables established the capability of chemically reduced Mo2C catalysts with long-term durability in alkaline and acidic media, which makes them interchangeable for high-cost materials.
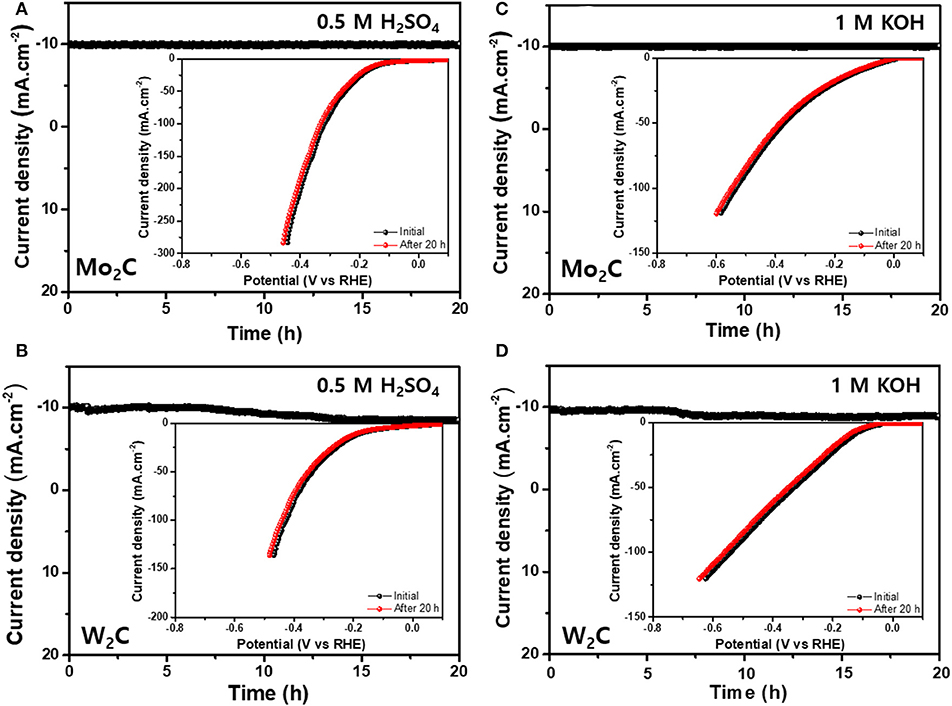
Figure 7. Long-term stability tests (A,B) chronoamperometric curves for Mo2C- and W2C-loaded NF electrocatalyst in 0.5 M H2SO4 (inset: corresponding LSV profiles for initial and after 20 h of HER performance) and (C,D) Chronoamperometric curves for Mo2C- and W2C-loaded NF electrocatalyst in 1.0 M KOH (inset: corresponding LSV profiles for initial and after 20 h of HER performance).
Furthermore, the stability of the Mo2C and W2C catalysts was investigated by XPS and FESEM analysis after continuous 20 h-HER operation in electrolytic solution (acidic medium); the results are shown in Figures S9, S10. The observed results also confirm no dramatic changes in XPS and FESEM data after continuous 20 h HER operation.
Conclusions
Chemically reduced Mo2C and W2C produced using an economical reduction method were successfully employed as electrocatalysts for application in HER. The observed HER results revealed that Mo2C and W2C nanoparticles had low overpotentials ( = −134 and −116 mV and −173 and −130 mV for Mo2C and W2C nanoparticles, respectively) with small Tafel slopes (83 and 65 mV.dec−1 and 86 and 100 mV.dec−1 for Mo2C and W2C nanoparticles, respectively) in a 0.5 M H2SO4 and in 1 M KOH electrolyte media. Both carbide catalysts showed strong stability in the alkaline and acidic media for over 20 h of operation. Thus, this work shows a viable way to synthesize nanostructured TMC-based electrocatalysts for hydrogen production.
Experimental Section
Materials and Methods
The commercial Mo2C and W2C chemicals of reagent grade were acquired from Sigma-Aldrich and were used without further purification. The following procedure was followed to prepare the chemically reduced Mo2C and W2C nanostructures (Hussain et al., 2019a). First, 2 g of commercial powder was well-disseminated in 100 mL of ethanol in a beaker to form a clear solution with the assistance of a room temperature stirring process. Subsequently, 50 mL of liquid ammonia solution was mixed with the black solution mixture, which was followed by a magnetic stirring at 85°C until the ethanol was completely evaporated from the mixture. The settled residue was then purified with de-ionized water and alcohol, sometimes using a centrifuge process, and the resultant material was placed in an oven at 60°C for 6 h. Finally, the collected black powders were placed in a quartz tube, and their temperature was raised with a heating rate of 5°C/min to attain 850°C with the support of a CH4/Ar/H2-mixture gas flow (50 sccm). The final chemically reduced Mo2C and W2C samples were kept in a vacuum desiccator for further analysis.
Electrochemical Measurements
The electrocatalytic HER properties were examined in an acid medium (0.5 M H2SO4 solution) and a alkaline medium (1 M KOH) by using a typical three-electrode setup. A saturated calomel electrode (SCE) and a carbon rod were used as the reference and counter electrodes, respectively. For the preparation of a working electrode, a 10:80:10 weight ratio of poly (vinylidene fluoride), active materials (W2C and Mo2C), and carbon black was used with an N-methyl-2-pyrrolidone solvent. The resultant sample was coated onto NF and dried overnight at 90°C. Mo2C- and W2C-loaded NF was employed as the working electrode. The LSV was recorded using a scan rate of 10 mV s−1 at room temperature. All the LSV performances were completed using SCE and then referenced to a reversible hydrogen electrode (RHE) scale using the following equation: E (RHE) = E (SCE) + E° (SCE) + 0.059 pH. An electrochemical impedance spectroscopy (EIS) study was performed within the 0.1 Hz to 1 MHz frequency with a perturbation voltage of 10 mV.
Characterization
Mo2C and W2C nanoparticles were studied using Raman spectroscopy (Renishaw Invia RE04, Ar laser−512 nm), FE-SEM (HITACHI S-4700), a Rigaku Ultima IV diffractometer with Cu-Kα radiation (0.154 nm), JEOL-2010F TEM with the help of Gatan DM software (version 3.0), PHI 5000 Versa Probe XPS, and a 3Flex surface characterization analyzer for nitrogen adsorption and desorption measurement at 77 K (Micromeritics, USA).
Data Availability Statement
All datasets generated for this study are included in the article/Supplementary Material.
Author Contributions
SH and JJ prepared the manuscript. SH performed the material synthesis. DV and AF actively took part in the characterization of catalysts. WS and K-SA performed XPS measurement and physical characterization of synthesized materials. H-SK, S-HC, and JJ did planning, design experimental work, and discussion. JJ edited the paper.
Funding
This research was supported by the Basic Science Research Program through the National Research Foundation of Korea (NRF), funded by the Ministry of Education (2015M3A7B7045194, 2017R1C1B5076952, and 2016M3A7B4909942), by the MOTIE (10052928) and the KSRC (Korea Semiconductor Research Consortium) support programs for the development of future semiconductor devices. This work was supported by the Korea Institute of Energy Technology Evaluation and Planning (KETEP) and the Ministry of Trade, Industry & Energy (MOTIE) of the Republic of Korea (20172010106080).
Conflict of Interest
The authors declare that the research was conducted in the absence of any commercial or financial relationships that could be construed as a potential conflict of interest.
Supplementary Material
The Supplementary Material for this article can be found online at: https://www.frontiersin.org/articles/10.3389/fchem.2019.00716/full#supplementary-material
References
An, K., and Xu, X. (2019). Mo2C based electrocatalyst with nitrogen doped three-dimensional mesoporous carbon as matrix, synthesis and HER activity study. Electrochim. Acta 293, 348–355. doi: 10.1016/j.electacta.2018.10.050
An, K., Xu, X., and Liu, X. (2017). Mo2C-based electrocatalyst with biomass-derived sulfur and nitrogen co-doped carbon as a matrix for hydrogen evolution and organic pollutant removal. ACS Sustain. Chem. Eng. 6, 1446–1455. doi: 10.1021/acssuschemeng.7b03882
Ang, H., Wang, H., Li, B., Zong, Y., Wang, X., and Yan, Q. (2016). 3D hierarchical porous Mo2C for efficient hydrogen evolution. Small 12, 2859–2865. doi: 10.1002/smll.201600110
Berglund, S. P., He, H., Chemelewski, W. D., Celio, H., Dolocan, A., and Mullins, C. B. (2014). p-Si/W2C and p-Si/W2C/Pt photocathodes for the hydrogen evolution reaction. J. Am. Chem. Soc. 136, 1535–1544. doi: 10.1021/ja411604k
Bockris, J. M., and Potter, E. (1952). The mechanism of the cathodic hydrogen evolution reaction. J. Electrochem. Soc. 99, 169–186. doi: 10.1149/1.2779692
Chen, W. F., Wang, C. H., Sasaki, K., Marinkovic, N., Xu, W., Muckerman, J., et al. (2013). Highly active and durable nanostructured molybdenum carbide electrocatalysts for hydrogen production. Energy Environ. Sci. 6, 943–951. doi: 10.1039/c2ee23891h
Chen, Y. Y., Zhang, Y., Jiang, W. J., Zhang, X., Dai, Z., Wan, L. J., et al. (2016). Pomegranate-like N, P-doped Mo2C@ C nanospheres as highly active electrocatalysts for alkaline hydrogen evolution. ACS Nano 10, 8851–8860. doi: 10.1021/acsnano.6b04725
Chen, Z., Cummins, D., Reinecke, B. N., Clark, E., Sunkara, M. K., and Jaramillo, T. F. (2011). Core–shell MoO3-MoS2 nanowires for hydrogen evolution: a functional design for electrocatalytic materials. Nano Lett. 11, 4168–4175. doi: 10.1021/nl2020476
Colton, R. J., Huang, J. T. J., and Rabalais, J. W. (1975). Electronic structure of tungsten carbide and its catalytic behavior. Chem. Phys. Lett. 34, 337–339. doi: 10.1016/0009-2614(75)85287-0
Cui, W., Cheng, N., Liu, Q., Ge, C., Asiri, A. M., and Sun, X. (2014). Mo2C nanoparticles decorated graphitic carbon sheets: biopolymer-derived solid-state synthesis and application as an efficient electrocatalyst for hydrogen generation. ACS Catal. 4, 2658–2661. doi: 10.1021/cs5005294
Fan, M., Zheng, Y., Li, A., Ma, Y., Huo, Q., Qiao, Z. A., et al. (2018). Sprout-like growth of mesoporous Mo2C/NC nanonetworks as efficient electrocatalysts for hydrogen evolution. ChemCatChem 10, 625–631. doi: 10.1002/cctc.201701417
Fan, X., Liu, Y., Peng, Z., Zhang, Z., Zhou, H., Zhang, X., et al. (2017). Atomic H-induced Mo2C hybrid as an active and stable bifunctional electrocatalyst. ACS Nano 11, 384–394. doi: 10.1021/acsnano.6b06089
Fei, H., Dong, J., Arellano-Jiménez, M. J., Ye, G., Dong Kim, N., Samuel, E. L. G., et al. (2015). Atomic cobalt on nitrogen-doped graphene for hydrogen generation. Nat. Commun. 6:8668. doi: 10.1038/ncomms9668
Gao, Q., Zhao, X., Xiao, Y., Zhao, D., and Cao, M. (2014). A mild route to mesoporous Mo2C–C hybrid nanospheres for high performance lithium-ion batteries. Nanoscale 6, 6151–6157. doi: 10.1039/c3nr06678a
Gong, Q., Wang, Y., Hu, Q., Zhou, J., Feng, R., Duchesne, P. N., et al. (2016). Ultrasmall and phase-pure W 2 C nanoparticles for efficient electrocatalytic and photoelectrochemical hydrogen evolution. Nat. Commun. 7:13216. doi: 10.1038/ncomms13216
Huang, Y., Gong, Q., Song, X., Feng, K., Nie, K., Zhao, F., et al. (2016). Mo2C nanoparticles dispersed on hierarchical carbon microflowers for efficient electrocatalytic hydrogen evolution. ACS Nano 10, 11337–11343. doi: 10.1021/acsnano.6b06580
Hussain, S., Akbar, K., Vikraman, D., Afzal, R. A., Song, W., An, K. S., et al. (2018). WS(1−x)Sex nanoparticles decorated three-dimensional graphene on nickel foam: a robust and highly efficient electrocatalyst for the hydrogen evolution reaction. Nanomaterials 8:929. doi: 10.3390/nano8110929
Hussain, S., Vikraman, D., Akbar, K., Naqvi, B. A., Abbas, S. M., Kim, H. S., et al. (2019a). Fabrication of MoSe2 decorated three-dimensional graphene composites structure as a highly stable electrocatalyst for improved hydrogen evolution reaction. Renew. Energy 143, 1659–1669. doi: 10.1016/j.renene.2019.05.126
Hussain, S., Zaidi, S. A., Vikraman, D., Kim, H. S., and Jung, J. (2019b). Facile preparation of molybdenum carbide (Mo2C) nanoparticles and its effective utilization in electrochemical sensing of folic acid via imprinting. Biosens. Bioelectron. 140:111330. doi: 10.1016/j.bios.2019.111330
Ishii, T., Yamada, K., Osuga, N., Imashiro, Y., and Ozaki., J. I. (2016). Single-step synthesis of W2C nanoparticle-dispersed carbon electrocatalysts for hydrogen evolution reactions utilizing phosphate groups on carbon edge sites. ACS Omega 1, 689–695. doi: 10.1021/acsomega.6b00179
Jacobsson, T. J., Fjällström, V., Sahlberg, M., Edoff, M., and Edvinsson, T. (2013). A monolithic device for solar water splitting based on series interconnected thin film absorbers reaching over 10% solar-to-hydrogen efficiency. Energy Environ. Sci. 6, 3676–3683. doi: 10.1039/c3ee42519c
Ko, Y. J., Cho, J. M., Kim, I., Jeong, D. S., Lee, K. S., Park, J. K., et al. (2017). Tungsten carbide nanowalls as electrocatalyst for hydrogen evolution reaction: new approach to durability issue. Appl. Catal. B Environ. 203, 684–691. doi: 10.1016/j.apcatb.2016.10.085
Kou, Z., Wang, T., Cai, Y., Guan, C., Pu, Z., Zhu, C., et al. (2018). Ultrafine molybdenum carbide nanocrystals confined in carbon foams via a colloid-confinement route for efficient hydrogen production. Small Methods 2:1700396. doi: 10.1002/smtd.201700396
Kou, Z., Wang, T., Pu, Z., Wu, L., Xi, K., and Mu, S. (2019). Realizing the extraction of carbon from WC for in situ formation of W/WC heterostructures with efficient photoelectrochemical hydrogen evolution. Nanoscale Horizons 4, 196–201. doi: 10.1039/C8NH00275D
Kou, Z., Xi, K., Pu, Z., and Mu, S. (2017). Constructing carbon-cohered high-index (222) faceted tantalum carbide nanocrystals as a robust hydrogen evolution catalyst. Nano Energy 36, 374–380. doi: 10.1016/j.nanoen.2017.04.057
Laursen, A. B., Kegnæs, S., Dahl, S., and Chorkendorff, I. (2012). Molybdenum sulfides—efficient and viable materials for electro-and photoelectrocatalytic hydrogen evolution. Energy Environ. Sci. 5, 5577–5591. doi: 10.1039/c2ee02618j
Li, J. S., Wang, Y., Liu, C. H., Li, S. L., Wang, Y. G., Dong, L. Z., et al. (2016). Coupled molybdenum carbide and reduced graphene oxide electrocatalysts for efficient hydrogen evolution. Nat. Commun. 7:11204. doi: 10.1038/ncomms11204
Liang, Q., Jin, H., Wang, Z., Xiong, Y., Yuan, S., Zeng, X., et al. (2019). Metal-organic frameworks derived reverse-encapsulation Co-NC@ Mo2C complex for efficient overall water splitting. Nano Energy 57, 746–752. doi: 10.1016/j.nanoen.2018.12.060
Lin, Z., Cai, L., Lu, W., and Chai, Y. (2017). Phase and facet control of molybdenum carbide nanosheet observed by in situ TEM. Small 13:1700051. doi: 10.1002/smll.201700051
Lv, C., Huang, Z., Yang, Q., Wei, G., Chen, Z., Humphrey, M. G., et al. (2017). Ultrafast synthesis of molybdenum carbide nanoparticles for efficient hydrogen generation. J. Mater. Chem. A 5, 22805–22812. doi: 10.1039/C7TA06266D
Lv, Y., and Wang, X. (2017). Nonprecious metal phosphides as catalysts for hydrogen evolution, oxygen reduction and evolution reactions. Catal. Sci. Technol. 7, 3676–3691. doi: 10.1039/C7CY00715A
Ma, F. X., Wu, H. B., Xia, B. Y., Xu, C. Y., and Lou, X. W. (2015). Hierarchical β-Mo2C nanotubes organized by ultrathin nanosheets as a highly efficient electrocatalyst for hydrogen production. Angew. Chem. Int. Ed. 54, 15395–15399. doi: 10.1002/anie.201508715
Ma, L., Ting, L. R. L., Molinari, V., Giordano, C., and Yeo, B. S. (2015). Efficient hydrogen evolution reaction catalyzed by molybdenum carbide and molybdenum nitride nanocatalysts synthesized via the urea glass route. J. Mater. Chem. A 3, 8361–8368. doi: 10.1039/C5TA00139K
Ma, R., Zhou, Y., Chen, Y., Li, P., Liu, Q., and Wang, J. (2015). Ultrafine molybdenum carbide nanoparticles composited with carbon as a highly active hydrogen-evolution electrocatalyst. Angew. Chem. 127, 14936–14940. doi: 10.1002/ange.201506727
Neylon, M., Choi, S., Kwon, H., Curry, K., and Thompson, L. (1999). Catalytic properties of early transition metal nitrides and carbides: n-butane hydrogenolysis, dehydrogenation and isomerization. Appl. Catal. A Gen. 183, 253–263. doi: 10.1016/S0926-860X(99)00053-8
Ojha, K., Saha, S., Kolev, H., Kumar, B., and Ganguli, A. K. (2016). Composites of graphene-Mo2C rods: highly active and stable electrocatalyst for hydrogen evolution reaction. Electrochim. Acta 193, 268–274. doi: 10.1016/j.electacta.2016.02.081
Pan, L. F., Li, Y. H., Yang, S., Liu, P. F., Yu, M. Q., and Yang, H. G. (2014). Molybdenum carbide stabilized on graphene with high electrocatalytic activity for hydrogen evolution reaction. Chem. Commun. 50, 13135–13137. doi: 10.1039/C4CC05698A
Pei, Y., Cheng, Y., Chen, J., Smith, W., Dong, P., Ajayan, P. M., et al. (2018). Recent developments of transition metal phosphides as catalysts in the energy conversion field. J. Mater. Chem. A 6, 23220–23243. doi: 10.1039/C8TA09454C
Peng, L., Nie, Y., Zhang, L., Xiang, R., Wang, J., Chen, H., et al. (2017). Self-assembly-and preshaping-assisted synthesis of molybdenum carbide supported on ultrathin nitrogen-doped graphitic carbon lamellas for the hydrogen evolution reaction. ChemCatChem 9, 1588–1593. doi: 10.1002/cctc.201700239
Peng, S., Li, L., Han, X., Sun, W., Srinivasan, M., Mhaisalkar, S. G., et al. (2014). Cobalt sulfide nanosheet/graphene/carbon nanotube nanocomposites as flexible electrodes for hydrogen evolution. Angew. Chem. 126, 12802–12807. doi: 10.1002/ange.201408876
Pu, Z., Wang, M., Kou, Z., Amiinu, I. S., and Mu, S. (2016). Mo 2 C quantum dot embedded chitosan-derived nitrogen-doped carbon for efficient hydrogen evolution in a broad pH range. Chem. Commun. 52, 12753–12756. doi: 10.1039/C6CC06267A
Ren, X., Wei, Q., Ren, P., Wang, Y., and Chen, R. (2018). Synthesis of flower-like MoSe2@MoS2 nanocomposites as the high efficient water splitting electrocatalyst. Mater. Lett. 231, 213–216. doi: 10.1016/j.matlet.2018.08.049
Seok, J., Lee, J. H., Cho, S., Ji, B., Kim, H. W., Kwon, M., et al. (2017). Active hydrogen evolution through lattice distortion in metallic MoTe2, 2D. Materials 4:025061. doi: 10.1088/2053-1583/aa659d
Shi, Z., Nie, K., Shao, Z. J., Gao, B., Lin, H., Zhang, H., et al. (2017). Phosphorus-Mo2C@carbon nanowires toward efficient electrochemical hydrogen evolution: composition, structural and electronic regulation. Energy Environ. Sci. 10, 1262–1271. doi: 10.1039/C7EE00388A
Tang, C., Sun, A., Xu, Y., Wu, Z., and Wang, D. (2015). High specific surface area Mo2C nanoparticles as an efficient electrocatalyst for hydrogen evolution. J. Power Sour. 296, 18–22. doi: 10.1016/j.jpowsour.2015.07.016
Vikraman, D., Akbar, K., Hussain, S., Yoo, G., Jang, J. Y., Chun, S. H., et al. (2017). Direct synthesis of thickness-tunable MoS2 quantum dot thin layers: optical, structural and electrical properties and their application to hydrogen evolution. Nano Energy 35, 101–114. doi: 10.1016/j.nanoen.2017.03.031
Vikraman, D., Hussain, S., Akbar, K., Karuppasamy, K., Chun, S. H., Jung, J., et al. (2019a). Design of basal plane edges in metal-doped nanostripes-structured MoSe2 atomic layers to enhance hydrogen evolution reaction activity. ACS Sustain. Chem. Eng. 7, 458–469. doi: 10.1021/acssuschemeng.8b03921
Vikraman, D., Hussain, S., Akbar, K., Truong, L., Kathalingam, A., S.-,Chun, H., et al. (2018). Improved hydrogen evolution reaction performance using MoS2-WS2 heterostructures by physicochemical process. ACS Sustain. Chem. Eng. 6, 8400–8409. doi: 10.1021/acssuschemeng.8b00524
Vikraman, D., Hussain, S., Truong, L., Karuppasamy, K., Kim, H. J., Maiyalagan, T., et al. (2019b). Fabrication of MoS2 /WSe2 heterostructures as electrocatalyst for enhanced hydrogen evolution reaction. Appl. Surf. Sci. 480, 611–620. doi: 10.1016/j.apsusc.2019.02.236
Vrubel, H., and Hu, X. (2012). Molybdenum boride and carbide catalyze hydrogen evolution in both acidic and basic solutions. Angew. Chem. 124, 12875–12878. doi: 10.1002/ange.201207111
Wan, C., Regmi, Y. N., and Leonard, B. M. (2014). Multiple phases of molybdenum carbide as electrocatalysts for the hydrogen evolution reaction. Angew. Chem. 126, 6525–6528. doi: 10.1002/ange.201402998
Wang, D., Guo, T., and Wu, Z. (2018). Hierarchical Mo2C/C scaffolds organized by nanosheets as highly efficient electrocatalysts for hydrogen production. ACS Sustain. Chem. Eng. 6, 13995–14003. doi: 10.1021/acssuschemeng.8b02469
Wang, D., Wang, J., Luo, X., Wu, Z., and Ye, L. (2017). In situ preparation of Mo2C nanoparticles embedded in ketjenblack carbon as highly efficient electrocatalysts for hydrogen evolution. ACS Sustai. Chem. Eng. 6, 983–990. doi: 10.1021/acssuschemeng.7b03317
Wu, H. B., Xia, B. Y., Yu, L., Yu, X. Y., and Lou, X. W. D. (2015). Porous molybdenum carbide nano-octahedrons synthesized via confined carburization in metal-organic frameworks for efficient hydrogen production. Nat. Commun. 6:6512. doi: 10.1038/ncomms7512
Wu, Z. Y., Hu, B. C., Wu, P., Liang, H. W., Yu, Z. L., Lin, Y., et al. (2016). Mo2C nanoparticles embedded within bacterial cellulose-derived 3D N-doped carbon nanofiber networks for efficient hydrogen evolution. NPG Asia Mater. 8:e288. doi: 10.1038/am.2016.87
Yan, G., Wu, C., Tan, H., Feng, X., Yan, L., Zang, H., et al. (2017). N-Carbon coated P-W2C composite as efficient electrocatalyst for hydrogen evolution reactions over the whole pH range. J. Mater. Chem. A 5, 765–772. doi: 10.1039/C6TA09052D
Youn, D. H., Han, S., Kim, J. Y., Kim, J. Y., Park, H., Choi, S. H., et al. (2014). Highly active and stable hydrogen evolution electrocatalysts based on molybdenum compounds on carbon nanotube–graphene hybrid support. ACS Nano 8, 5164–5173. doi: 10.1021/nn5012144
Yu, F., Gao, Y., Lang, Z., Ma, Y., Yin, L., Du, J., et al. (2018). Electrocatalytic performance of ultrasmall Mo 2 C affected by different transition metal dopants in hydrogen evolution reaction. Nanoscale 10, 6080–6087. doi: 10.1039/C8NR00908B
Yuan, W., Huang, Q., Yang, X., Cui, Z., Zhu, S., Li, Z., et al. (2018). Two-dimensional lamellar Mo2C for electrochemical hydrogen production: insights into the origin of HER activity in acid and alkaline electrolytes. ACS Appl. Mater. Interfaces 10, 40500–40508. doi: 10.1021/acsami.8b13215
Zhang, L. N., Ma, Y. Y., Lang, Z. L., Wang, Y. H., Khan, S. U., Yan, G., et al. (2018). Ultrafine cable-like WC/W 2 C heterojunction nanowires covered by graphitic carbon towards highly efficient electrocatalytic hydrogen evolution. J. Mater. Chem. A 6, 15395–15403. doi: 10.1039/C8TA05007D
Zhang, R., Wang, X., Yu, S., Wen, T., Zhu, X., Yang, F., et al. (2017). Ternary NiCo2Px nanowires as pH-universal electrocatalysts for highly efficient hydrogen evolution reaction. Adv. Mater. 29:1605502. doi: 10.1002/adma.201605502
Zhou, H., Yu, F., Huang, Y., Sun, J., Zhu, Z., Nielsen, R. J., et al. (2016). Efficient hydrogen evolution by ternary molybdenum sulfoselenide particles on self-standing porous nickel diselenide foam. Nat. Commun. 7:12765. doi: 10.1038/ncomms12765
Keywords: Mo2C, W2C, nanoparticle, HER, electrocatalyst
Citation: Hussain S, Vikraman D, Feroze A, Song W, An K-S, Kim H-S, Chun S-H and Jung J (2019) Synthesis of Mo2C and W2C Nanoparticle Electrocatalysts for the Efficient Hydrogen Evolution Reaction in Alkali and Acid Electrolytes. Front. Chem. 7:716. doi: 10.3389/fchem.2019.00716
Received: 14 August 2019; Accepted: 10 October 2019;
Published: 25 October 2019.
Edited by:
Yao Zheng, University of Adelaide, AustraliaReviewed by:
Bao Yu Xia, Huazhong University of Science and Technology, ChinaShichun Mu, Wuhan University of Technology, China
Copyright © 2019 Hussain, Vikraman, Feroze, Song, An, Kim, Chun and Jung. This is an open-access article distributed under the terms of the Creative Commons Attribution License (CC BY). The use, distribution or reproduction in other forums is permitted, provided the original author(s) and the copyright owner(s) are credited and that the original publication in this journal is cited, in accordance with accepted academic practice. No use, distribution or reproduction is permitted which does not comply with these terms.
*Correspondence: Jongwan Jung, andqdW5nQHNlam9uZy5hYy5rcg==