- 1College of Science, Nanjing Forestry University, Nanjing, China
- 2College of Materials Science and Engineering, Nanjing Forestry University, Nanjing, China
- 3Key Laboratory of Flexible Electronics (KLOFE) & Institute of Advanced Materials (IAM), Jiangsu National Synergetic Innovation Center for Advanced Materials (SICAM), Nanjing Tech University (NanjingTech), Nanjing, China
With the development of organic optoelectronic materials and bioimaging technology, to exploit organic luminescent materials with high luminescent efficiency in aggregation-state has become a research hotspot. BODIPYs have become one of the research objects of this kind of material because of their obvious advantages. This review focuses on the design and synthesis of AIE-type BODIPYs, the mechanism of AIE properties and their applications in recent years. Through classification, analysis, and summary, this review aims to explore the structure-activity relationship of AIE-type BODIPYs and to provide ideas for the further design and potential applications of AIE-active fluorescent materials.
Introduction
Organic luminescent materials (OLMs) are widely used in chemo/biosensors and light-emitting devices in light of their rich advantages, which include great diversity, easily modified structures, rich colors, and low environmental pollution (Chan et al., 2012; Uoyama et al., 2012; Yan et al., 2018). Since most of these applications heavily depend on their luminescent capabilities in the condensed state, the development of luminophores with excellent photophysical properties in the aggregation state is highly required. Traditional organic fluorescent dyes with a π-conjugated structure show excellent luminescent properties in dilute solution but become weakly or non-emissive in high concentration solutions or the aggregation-state, which is called the aggregation-caused emission quenching (ACQ) effect (Förster and Kasper, 1954). This effect is due to the collision between the ground state and the excited state of the fluorescent molecule at high concentration, which leads to the non-radiation deactivation process, or because the strong interaction between the planar π-conjugated structures leads the formation of excimers or exciplexes, and the energy of the excited state decays through the non-radiative form. The ACQ effect greatly limits the practical application of OLMs because the aggregated states are unavoidable for both light-emitting devices and fluorescent sensors.
Aggregation-induced emission (AIE), which was first introduced by Tang et al. has been widely accepted as a novel strategy to mitigate the ACQ effect on OLMs (Mei et al., 2015). Generally, AIE molecules such as 1,1,2,3,4,5-hexaphenylsilole (HPS, Figure 1) and tetraphenylethylene (TPE, Figure 1) usually possess highly twisted structures and show weak fluorescence in diluted solutions due to non-radiative transition induced by intramolecular motion (IM) in their excited state. In the aggregation state, such IM progress is effectively suppressed, resulting in their enhanced emission. Additionally, the highly twisted structure can effectively inhibit the π-π interactions between AIE molecules, which is conducive to improving their solid-state luminescence efficiency. Based on the widely accepted restriction of intramolecular motion (RIM) mechanism, AIE materials, including not only various newly designed molecules but also classical fluorophores including coumarins, pyrene, squaraines, cyanies, perleneimides, and BODIPYs, have been developed and applied in bioimaging, data encryption/decryption, OLEDs, and stimuli-responsive materials (Mei et al., 2015; Kokado and Sada, 2019).
As a classical luminophore, BODIPY dyes (boron dipyrromethene and its analogs, Figure 1) have achieved great development in the field of fluorescent sensing and bioimaging because of their excellent photophysical properties, including large molar extinction coefficients, high fluorescence quantum yields, tunable emission from visible light to near-infrared (NIR), and high photo- and chemical-stability (Loudet and Burgess, 2007; Boens et al., 2012; Lu et al., 2014; Kowada et al., 2015; Fan et al., 2016; Ge and O'shea, 2016; Wang et al., 2017). Unfortunately, in contrast to their excellent luminescence in a solution, most BODIPYs suffer from the ACQ effect and show weak fluorescence in the aggregation state. This is mainly because of the self-absorption and strong intermolecular interactions (π-π stacking, etc.) induced by their small Stokes shift and planar π-conjugated structures. These problems greatly restrict the further applications of BODIPYs as solid-state emitters. Therefore, the development of BODIPYs with aggregation-state fluorescence has received intense attention in the past decades.
AIE has been proved to be an efficient strategy for the construction of BODIPYs with efficient fluorescence in the aggregation state. A number of AIE-active BODIPYs have been rationally designed by various strategies, such as the direct integration of AIE molecules with the BODIPY skeleton (Hu et al., 2012; Gomez-Duran et al., 2015), J-type aggregation (Choi et al., 2014; Kim et al., 2015), and dipyrromethene bidentate ligand modification (Yang et al., 2012; Wang et al., 2015b). Taking the advantages of AIE, the intense aggregation-state fluorescence of BODIPYs has been successfully achieved. Moreover, their application as aggregation-state emitters for bioimaging, stimuli-response switches as well as OLEDs has been demonstrated (Mei et al., 2015; Baysec et al., 2018; Che et al., 2019). This mini review focuses on providing an overview of the design, mechanism and application of AIE-active BODIPYs and BODIPY analogs so as to facilitate their future application in the solid-state luminescence field. For the convenience of explanation, AIE-active BODIPYs are divided into two categories: one is based on the boron dipyrromethene platform (classical BODIPYs); the other is BODIPY analogs based on heterocycle-based bidentate chelates. The key photophysical data of each compound discussed are listed in Table 1. Moreover, boron difluoride complexes based on β–diketonate, ketoiminate, and diiminate will not be discussed in this review. A review that summarizes the photophysical properties and applications of these complexes would be helpful to readers (Tanaka and Chujo, 2015).
Classical BODIPYs with AIE
AIE-Active BODIPYs Based on TPE
Due to the planar π-conjugated structure of boron dipyrromethene core, strong intermolecular interactions such as π-π stacking and hydrogen bonds are usually observed in the aggregation state of BODIPYs, leading to distinct emission quenching. In order to suppress the strong intermolecular interactions, the well-known AIE luminescent element, TPE, has been successfully integrated with BODIPYs; thus, both AIE and intense aggregation-state emission were achieved. Tang et al. first reported TPE-containing BODIPYs (1–3, Figure 2) with AIE effect (Hu et al., 2012). In compounds 1–3, TPE was simply introduced to the meso-position of the BODIPY core via a palladium-catalyzed cross-coupling reaction. In tetrahydrofuran (THF)-water mixture, both locally excited (LE) state, and twisted intramolecular charge transfer (TICT) state emission bands were observed in the emission spectra of compounds 1–3. Compound 1 showed ACQ with the increment of the fraction of water (fw) in THF. In contrast, the intensity of the TICT emission band of compounds 2 and 3 was increased dramatically and accompanied by the decrement of the LE emission band. Meanwhile, the fluorescence quantum yields (Φf) of compounds 2 and 3 in the solid state were determined to be 27 and 7.5%, respectively, which are higher than those of obtained in THF solution (Φf < 1%). Clearly, the relative stronger TICT effect of compounds 2 and 3 compared to compound 1 should be responsible for their different AIE and ACQ behavior.
The AIE behavior of TPE-BODIPY is highly dependent on the position and the number of TPE units attached to the BODIPY core. For example, Wu et al. (Chua et al., 2015), Scherf et al. (Baysec et al., 2018), and Atilgan et al. (Baglan et al., 2013) have reported that BODIPY derivatives with TPE or triphenylethene units at 2,6- or 2,6,8-positions (4–5, Figure 2) could effectively inhibit ACQ and act as aggregation-induced emission enhancement (AIEE). However, the conjugating of TPE at 3,5-position of the BODIPY core resulted in large π-conjugated structures with ACQ (6–7) (Gomez-Duran et al., 2015).
AIE-Active BODIPYs Based on Triphenylamine (TPA)
Designing the propeller-shaped BODIPY molecules to consist of electron donor (D) and acceptor (A) units is another method to hinder the ACQ effect. Tang et al. developed a group of AIE-active BODIPYs with a D-A structure (8–11, Figure 3). Due to the strong electronic interaction between TPA (D) and BODIPY (A), compounds 8–11 displayed TICT and AIE properties. When the water was added to the THF solution of 8–11, the LE emission intensity decreased with an increment of fw, accompanied by the red-shift of emission. This progress is mainly dominated by the polarity effect. However, when the fw reached the point of aggregation, the rotation of the aromatic rings was efficiently restricted, resulting in blue-shifted emission, and AIE (Figure 4) (Hu et al., 2009; Lager et al., 2009). Moreover, compounds 12–14 with TPA unit incorporated into the 2-, 2,6-, 2,6,8-positions showed more enhanced TICT effect than compounds 8–11, and only aggregation-induced emission enhancement of TICT was observed (Bui et al., 2019).
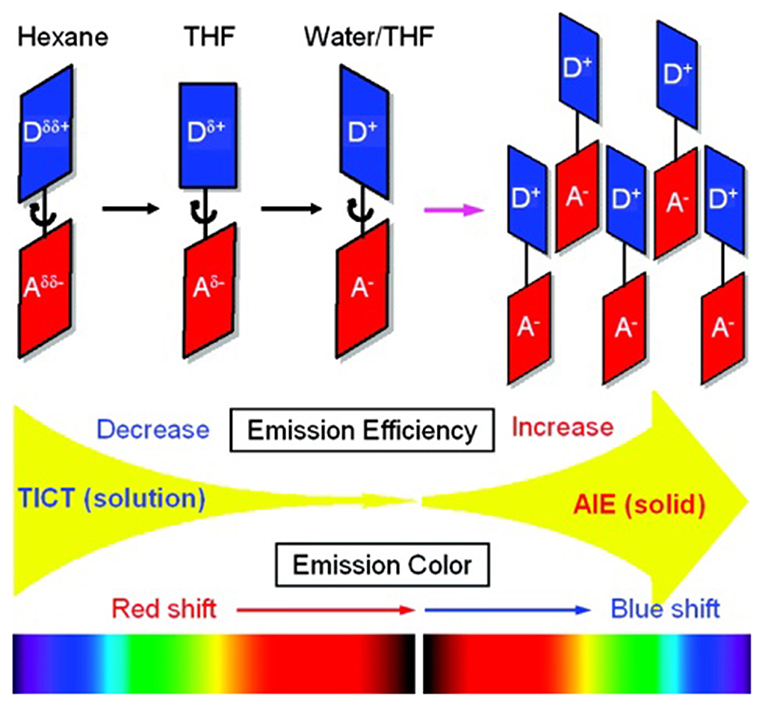
Figure 4. Proposed mechanism for TICT and AIE behaviors in AIE-active BODIPYs based on TPA (Reprinted from Hu et al., 2009. Copyright 2009 American Chemical Society).
AIE-Active BODIPYs Based on J-aggregation
Most BODIPYs tend to form H-type (face to face) aggregates in the aggregated state, which leads to fluorescence quenching. Recent studies indicated that the packing of BODIPYs could be engineered to favor the formation of J-type (head to tail) aggregates, and they would then give out red-shifted emission in comparison to their respective monomers in solution. Under this aggregation, the transition dipoles of the monomers aligned in a coplanar inclined way with a slip angle <54.7° to form dimers, trimers or even larger J-aggregates (Wurthner et al., 2011; Choi et al., 2014; Tian et al., 2018).
Johansson et al. first evidenced the formation of non-fluorescent BODIPY H-dimers in double-labeled proteins and emissive J-dimers in labeled lipid vesicles (Bergström et al., 2002). Vu et al. found that bulky substituents at the 3- and 5-positions of the BODIPY core, such as paracyclophane (15, Figure 5) (Vu et al., 2009) and the adamantyl group (16) (Vu et al., 2013), could facilitate the formation of emissive J-aggregates in the aggregation state. To elucidate the factors that govern the formation of emissive J-aggregates of BODIPYs, Kim et al. carried out a systematic study of the substitution effect on the meso-position (Choi et al., 2014; Kim et al., 2015). For 1,3,5,7-tetramethyl derivatives, the meso-substituents 17, that are -CF3, -COOMe, -COOtBu, and -iPr, demonstrably formed emissive J-aggregates. Meanwhile, other meso-substituents, such as -CH3, -CHO, -CN, and -Cl, exhibited the ACQ effect or were fluorescent in the solid state without forming J-aggregates (Figure 6). The formation of emissive J-aggregates is quite sensitive to minute structural changes. J-aggregations were not encountered in the closely related 3,5-dimethyl derivatives. Both the electron-withdrawing meso-substituents and flanking methyl groups are necessary for the formation of emissive BODIPY J-aggregates. Moreover, by using the AIEE-type meso-ester-substituted BODIPY probe 17b, they realized the need to detect specifically HOBr generated by eosinophil peroxidase (EPO) for a clean turn-on signal: the red emissive (621 nm) J-aggregates of 2,6-dibrominated 17b self-assembled into orange emissive (581 nm) J-aggregates (Kim et al., 2018).
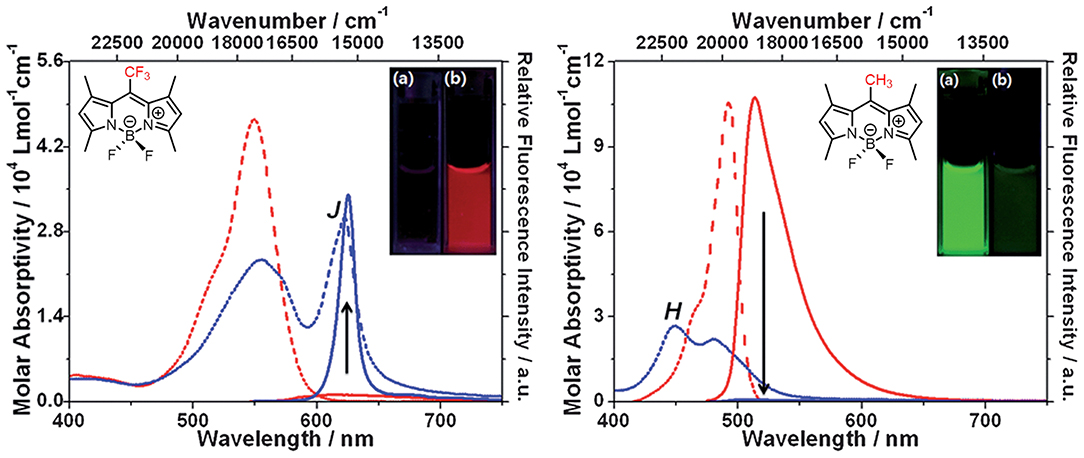
Figure 6. Different aggregation behavior of 17a (2 × 10−5 mol L−1) and it's analog with a methyl group at the meso-position in acetonitrile and acetonitrile-water mixtures (1:99, v: v). Dotted and solid lines refer to the absorption and emission spectra of BODIPYs, respectively (adapted with permission from Choi et al., 2014, Copyright 2014, Royal Chemistry Society).
Besides the abovementioned J-aggregation tuning tuned via variation the meso-substitutions, the modification of BF2 moiety with a diacyloxyl or diaryl substituent should be another potential strategy. For example, AIE behavior induced by J-aggregation of BODIPY in pure organic solvents was described by Chiara et al. in O-BODIPYs with a B-spiranic 4,4-diacyloxyl substitution pattern (18, 19, Figure 5). The high conformational rigidity of this design along with the orthogonal disposition of the B-diacyloxyl substituent and the meso-aryl group were analyzed to be the key factors of the J-aggregation process (Manzano et al., 2016). Wang et al. investigated spiro-BODIPYs with a diaryl chelate unit that could form J-aggregates in the alcohol-water mixture. The J-aggregates of 20a showed increased emission efficiency while those of 21a and 22a indicated decreased emission efficiency, suggesting that the change in emission intensity is not a reliable indicator for the formation of J-aggregates. An important detail to mention is that similar structures substituted by phenyl at meso-position (20b–22b) were not observed in the J-aggregation formation of the alcohol-water mixture or in the tetrahydrofuran-water mixture (Yuan et al., 2017).
Bodipy Analogs with AIE
AIE strategy, relying on the RIM, has produced numerous systems with high emission in the aggregation state. Except for classical BODIPYs, the development of new members of BODIPYs family viz. BODIPY analogs with AIE-active will undoubtedly contribute to a better understanding of the phenomena and lead to novel applications.
In the process of elucidating the optical properties of benzo[c, d]indole-containing aza-BODIPYs, Kobayashi et al. found that the photophysical property of aza-BODIPYs could be tuned by incorporating heteroaromatic moieties in place of pyrrole or isoindole rings. Moreover, they reported the first aza-BODIPY (23, Figure 7) exhibiting AIEE behavior. Compound 23 showed weak fluorescence in a diluted solution (Φf = 2% in THF solution), however, fluorescence enhancement was observed both in film-state (drop-cast film, Φf = 14%) and aggregation-state (fw = 90%, Φf = 23%). Clearly, the restricted molecular dynamics induced by the non-conjugated moiety should be responsible for such AIEE phenomenon (Shimizu et al., 2015).
Similar to the strategy mentioned in section AIE-active BODIPYs Based on TPE, the AIE property of NIR-emissive aza-BODIPYs based on a diketopyrrolopyrrole-benzo[d]thiazole ligand was realized by linking one or two TPE moieties to its planar π-conjugated structure (Li et al., 2017). Compounds 24 and 25 showed weak fluorescence with Φf of 0.7 and 0.4% in diluted dichloromethane solution. After the adding of hexane as a poor solvent to the dichloromethane solution, great fluorescence enhancement of around 690 nm was observed (21.9% for compound 24, and 18.8% for compound 25) due to the formation of high emissive aggregates. Moreover, the imaging ability of 25-NPs, which was prepared from compound 25 and Pluronics 127, has been proved in HeLa cells.
By incorporating two phenothiazine units into the biquinoline-based ligand, an AIE-active aza-BODIPY with highly twisted structure (26, Figure 7) was reported by Zhu et al. (2014). Compound 26 showed weak blue emission at 480 nm in THF solution. The addition of water to the THF solution (fw ≤ 50%) first induced emission quenching due to the enhanced TICT effect. Then, great red-shifted emission from 480 to 610 nm accompanied by emission enhancement was observed because of the formation of aggregates.
Besides the strategies of incorporation of AIE units into the BODIPY core and J-aggregation engineering, modification of the dipyrromethene bidentate to give BODIPY analogs with desymmetrized and propeller-shaped structure has also proved to be an efficient method to achieve AIE-active BODIPYs with high aggregation-state Φf. Generally, these BODIPY analogs usually show a larger Stokes shift than classical BODIPY, which is helpful for suppressing the self-absorption in the condensed phase. Moreover, as a benefit of their high twisted structure, the strong π-π interaction can be efficiently avoided. Based on the above conception, various AIE-active BODIPY analogs with the propeller-shaped structure have been developed by replacing the dipyrrole units to various heterocycles such as pyridine, benzo[d]thiazole, quinoline, etc.
Heterocycle-hydrazone-based boron difluoride complexes, which were first reported by Aprahamian et al. are a new class of AIE-active BODIPY analogs (27, Figure 8) (Yang et al., 2012). Due to the desymmetrized and propeller-shaped structure, compound 27a showed weak fluorescence at 512 nm (Φf < 10%) with a large Stokes shift (101,010 cm−1) in dichloromethane. After restricting the intramolecular rotations, enhanced emission both in the film and crystalline state was observed. Most importantly, the AIE mechanism of pyridine-hydrazone-based boron difluoride complexes was rationalized by TD-DFT calculations (Qian et al., 2017). The calculated results demonstrated that the emission of these compounds was not generated from the S1 state but from the other excited states with higher energy (>S1). The authors also suggested that suppression of Kasha's rule should be the real mechanism responsible for emission in the solid state.
Inspired by the AIE-active BOIDPY analogs based on pyridine-hydrazone ligands, a group of new AIE-active monoboron (28) and bisboron (29) difluoride complexes was developed by adopting benzo[d]thiazole-hydrozone as the chelates (Duan et al., 2018). Similar to compound 27a, these complexes showed weak emission (Φf < 1%) and large Stokes shifts (up to 7,400 cm−1) in a diluted solution and AIEE in the aggregation state. The AIE behavior of these complexes was investigated and found to be closely related to the RIR of the aromatic rings.
In consideration of the attractive properties of heterocycle-amidine and heterocycle-hydrazone ligands based BODIPY analogs, it is worth to further expand the family of these BODIPY analogs so as to develop more AIE-active functional materials. In this case, various BODIPY analogs based on heterocycle-enolate ligands have been successfully developed. Matsui et al. synthesized a boron difluoride complexes derivate from βbenzo[d]thiazole-enolate ligands (30, Figure 8) (Kubota et al., 2012). The desymmetrized structure and AIEE effect make these complexes show high emission with Φf up to 60% in the solid state. Based on this pioneering work, Matsui et al. also investigated the photophysical and AIE properties of monoboron and bisboron complexes based on pyrimidine-enolate ligands (31, Figure 8) (Kubota et al., 2013). By tuning the CT and conjugation effect, an intense solid-state emission maximum from 488 to 641 nm was achieved (Φf = 7–20%). Next, a number of propeller-shaped BODIPY analogs based on pyridine- (Wu et al., 2015), quinoxaline- (Liao et al., 2015), pyrimidine- (Qi et al., 2016), and benzo[d]thiazole-enolate (Gong et al., 2015) ligands (32–34, Figure 8) were reported by different groups. Like HPS and TPE, the propeller-shaped structure quenched the emission of these complexes in a diluted solution, and, after restricting the intramolecular rotations by aggregation, distinct AIE behavior was observed.
By reacting pyridine- and benzo[d]thiazole-enolate ligands with arylamine, our group developed a series of pyridyl- and benzothiazole-enamide N∧N-bidentate ligands, which facilitated the generation of a new family of propeller-shaped BODIPY analogs (Liu et al., 2015; Wang et al., 2015a,b). Similarly to the abovementioned compounds 27–34, compounds 35 and 36 showed very weak emission in low-viscosity solvents and displayed AIE in the aggregation state. All of these compounds showed large Stokes shifts and very weak intermolecular interactions in the aggregation state, resulting in high Φf. Moreover, the applications of these compounds as solid-emitters for acid gas and pressure sensing were also demonstrated.
Applications of AIE-active BODIPYs
Although BODIPYs have gained great success in biological sensing and imaging, their application as the emitter in the aggregation state was rarely reported in the past decades. This obstacle would be well-solved by the rational designing of aggregation-state emissive BODIPYs and their analogs. Indeed, taking the advantages of AIE, the application scope of BODIPYs has been successfully expanded from solution state to aggregation state in recent years.
Fluorescent Imaging
Fluorescence imaging technology has been demonstrated as a powerful tool for investigating biological processes in living cells and clinical diagnostics because of its high specificity and sensitivity, high resolution, and its nondestructive properties (Johnson and Spence, 2010). Numerous fluorescent materials such as fluorescent proteins, quantum dots, polymer dots, and small organic fluorescent molecules with photo-active or photoswitch properties have been developed (Lavis and Raines, 2008; Chan et al., 2012; Li et al., 2014). Compared with the above fluorescent materials, AIE materials have distinct advantages, including high emission at high concentration or in the aggregation state, low toxicity and good anti-photobleaching ability, which make them hold great potential as candidates for fluorescent imaging (Chen et al., 2016). As an important member of AIE materials, AIE-active BODIPYs have been employed as imaging agents in biological sensing and imaging.
Tang et al. first used the aggregates of compound 3 for fluorescence imaging in living HeLa cells. After staining living cells with the aggregates, both the LE emission, and TICT emission were detected (Hu et al., 2012). After that, the biocompatible AIE dots that were prepared from AIE-active BODIPY molecules were applied for intracellular fluorescent imaging. For example, red emissive nanoparticles of compound 4 were obtained by encapsulating compound 4 with 1,2-sistearoyl-sn -glycero-3-phosphoethanolamine-N-[methoxy(polyethylene glycol)-2000] (DSPE-PEG2000). Benefitting from their good biocompatibility and two-photon absorption and excited fluorescence (TPEF), these AIE NPs were further applied in TPEF cellular imaging and mouse brain blood vascular visualization, suggesting their potential application in TPEF sensing and imaging (Zhao et al., 2014).
Very recently, the efficient imaging capabilities of TPA- and carbazoyl-based AIE-active BODIPYs have also been reported by Tang and Su. After fabricating in the presence of DSPE-PEG2000, the NPs of compound 12 were obtained with intense far-red emission (around 650 nm) and excellent photostability. Moreover, these NPs showed an ultrafast cell staining time of a few seconds and excellent cell imaging ability. More importantly, these NPs can be used for long term imaging both in vitro and in vivo (Figure 9), demonstrating their great potential imaging abilities in the practical biological applications (Che et al., 2019).
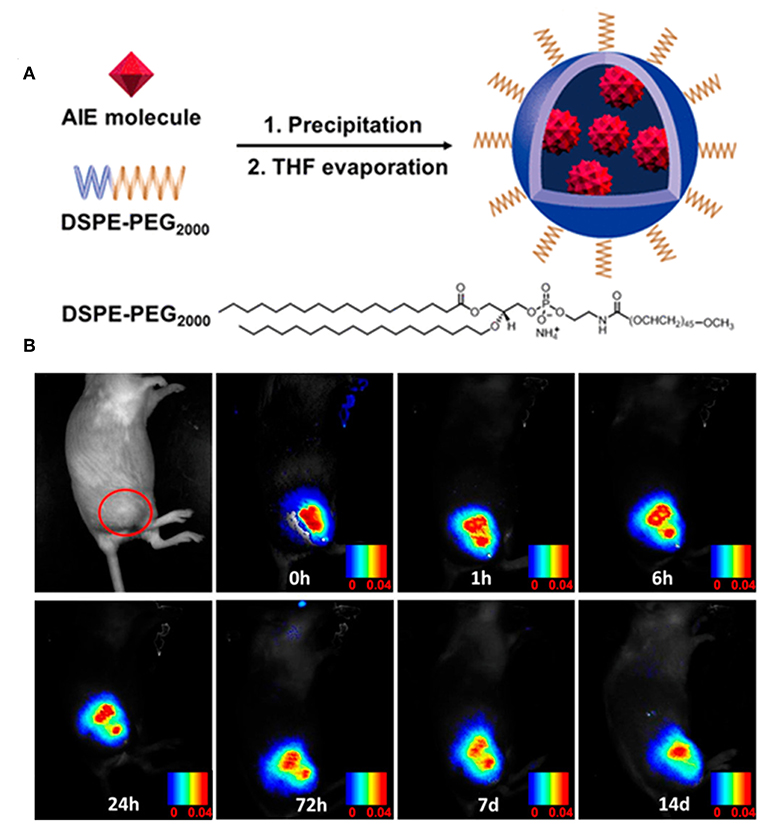
Figure 9. (A) Preparation of the AIE NPs of compound 12. (B) In vivo confocal images of the tumor-bearing mouse with AIE NPs of compound 12 from day 0 to day 14 (adapted with permission from Che et al., 2019, Copyright 2019, Royal Chemistry Society).
Mechanofluorochromic (MFC) Materials
MFC materials that change their luminescence upon mechanical grinding/shearing have been attracting a great deal of interest owing to their promising applications (Sagara et al., 2016). Generally, AIE molecules with a strong twisted skeleton with rotatable aryl units, resulting in the stacking of loose molecules in the crystal state, can be easily destroyed by mechanical stimulation, resulting in a change of luminescence color. Thus, twisted π-conjugated AIE-active BODIPY analogs have been used as potential candidates for promising MFC materials. For example, the yellow powder of compound 26 showed bright fluorescence with λem at 540 nm. After grinding with the motor, the yellow emissive powder immediately changed its emission color to red (λem = 635 nm), resulting in a 95 nm red-shift of emission. Moreover, the mechanic-induced color change can be switched back via dichloromethane fuming. The crystalline-amorphous-crystalline state transformation of 26 during the grinding and fuming stimuli processes has been demonstrated by powder X-ray diffraction (PXRD) (Figure 10) (Zhu et al., 2014).
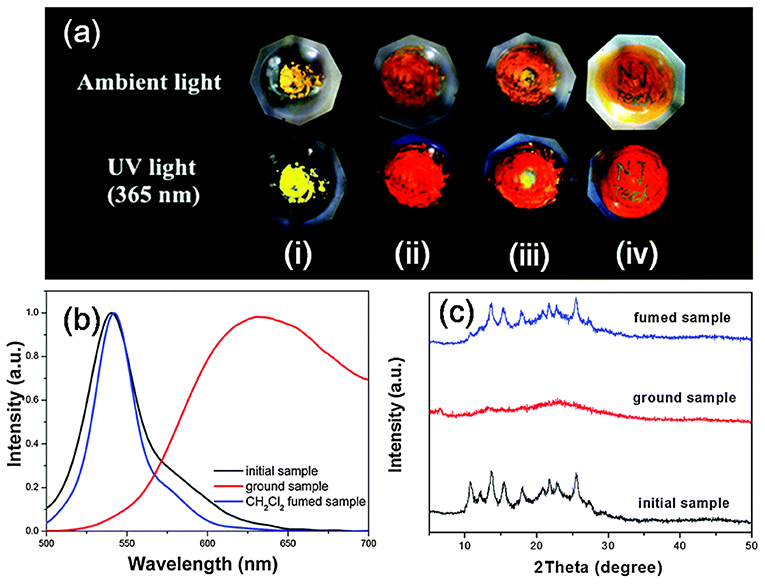
Figure 10. (a) Photographs of the powder of compound 26 before and after grinding under ambient and light UV light (365 nm): (i) crystalline powder, (ii) ground powder, (iii) ground powder upon adding of a drop of CH2Cl2, and (iv) the letters “NJ Tech” were written on the ground powder using CH2Cl2. (b) Emission spectra and (C) PXRD patterns of the powder, after being ground and CH2Cl2-fumed (Reprinted from Zhu et al., 2014, Copyright 2014, Royal Chemistry Society).
Upon mechanical grinding or hydrostatic compression, compounds 35e and 35f displayed red-shift emission under high pressure, while 35f with ICT effects showed a more sensitive piezochromic response at low pressure (<1.5 GPa), which implied that the pressure-dependent π-π intermolecular interaction and the intramolecular CT effect were efficient in inducing piezochromic luminescence (Figure 11) (Wang et al., 2015a). The distinct piezochromic effect of 35f at low compression pressure suggested that the propeller-shaped AIE luminophore with the ICT effect could be a valuable basis upon which to design MFCs with high sensitivity. In the process of studying pyrimidine-based BF2 complexes, 33a–d, we found that only 33d showed distinct luminescence change upon mechanical stimuli. 33d underwent red-shift from 491 to 509 nm on mechanical grinding, while it recovered to the original state when exposed to dichloromethane vapor for 10 min. In addition to the XRD characteristic, we rationalized that the mechanochromism is attributed to the desymmetric propeller-shaped configuration and donor-acceptor character of 33d (Qi et al., 2016).
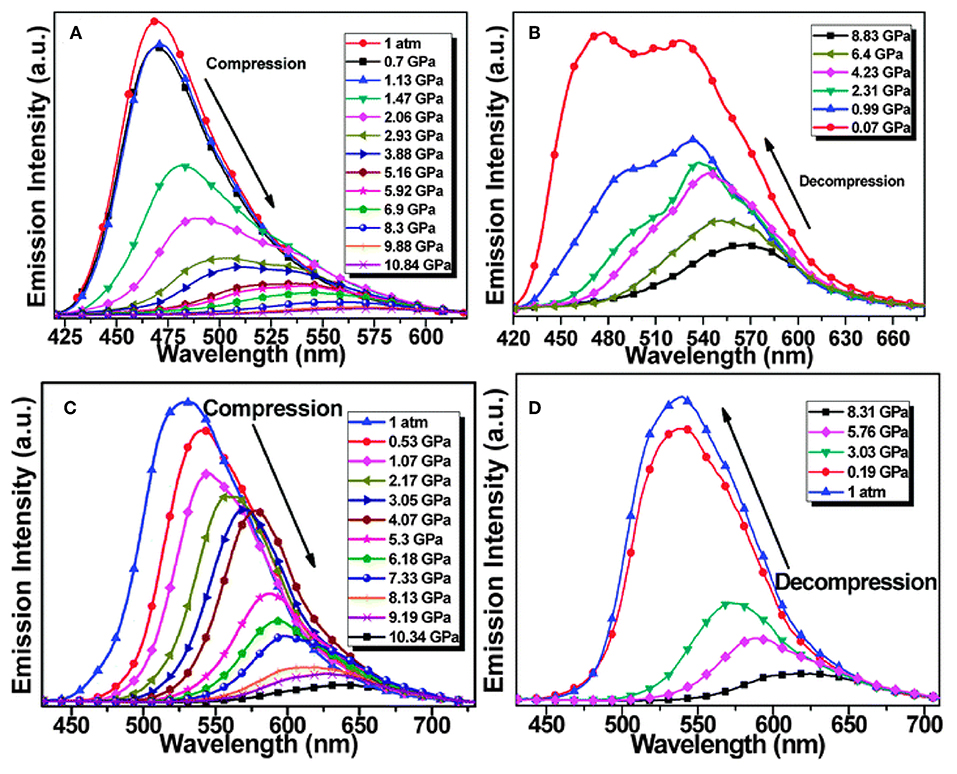
Figure 11. Emission spectra of compounds 35e (A,B) and 35f (C,D) upon the compressing (A,C) and following decompressing processes (B,D) (Reprinted from Wang et al. (2015a), Copyright 2015, Royal Chemistry Society).
Gas Sensors
Due to their strong fluorescent properties in the solid state, AIE-active BODIPYs and their analogs are proposed to be an ideal candidate for gas sensing. A number of compounds have been reported as the fluorescent switch for organic solvent, acid and base gases. For example, compounds 34a–e possessed unusual acidochromic behavior triggered by acid vapor (Liao et al., 2015). After exposure to trifluoroacetic acid (TFA) vapors, the colors of 34a-e turned obscure, and absorption spectra were red-shifted and accompanied by strong quenching of luminescence. The effect of fluorescence quenching upon acid fuming should be attributed to the synergistic effects of the protonation of nitrogen resulting from the pyrazine segment-induced push-pull effect and the changes of intermolecular packing and molecular conformation upon acid protonation (Figure 12).
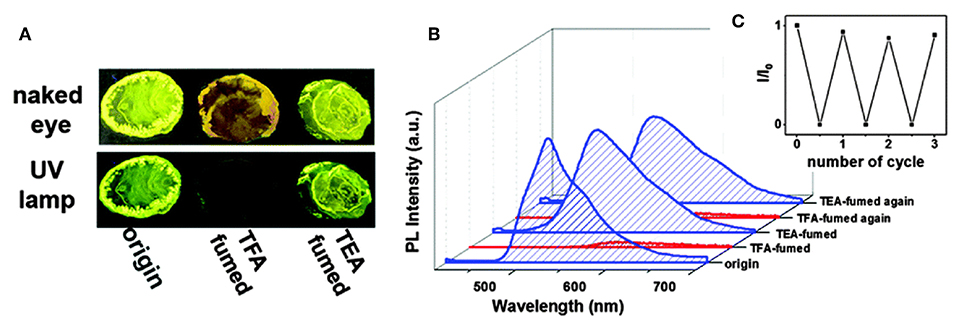
Figure 12. (A) Photographs of compound 34d on sliding glass taken under ambient and UV light (λex = 365 nm). (B) Emission spectra of compound 34d after treating with TEA–TFA vapors in solid-state. (C) Recycling of the emission switching of the power of compound 34d upon fuming with TFA and TEA vapors (Reprinted from Liao et al., 2015, Copyright 2015, Royal Chemistry Society).
Another example is that the intense solid-state emission of compound 26 could be switched by multiple external stimuli, including grinding, organic solvent vapors as well as acid and base vapors (Zhu et al., 2014). Fumigation of 26 with HCl–TEA vapors exhibited an off/on switching fluorescence effect. Based on the protonation–deprotonation stimuli luminescence property of 26, a simple, convenient and efficient piece of technology for data encryption and decryption was designed. All these comprehensive investigations suggested that complex 26 was a very promising candidate for application in sensing, detection, and security protection.
The introduction of the N,N-dimethylamino group as an acid-sensitive group to the π-conjugated structure of AIE-active BODIPYs has been demonstrated to be an efficient strategy for achieving highly sensitive acidic vapor sensing. Taking compound 35b as an example, when exposed to HCl vapors for a few seconds, 35b exhibited blue-shifted emission with the color changing from yellow to cyan (547–518 nm). The protonated powder samples gradually recovered their original color and fluorescence when they were treated with NH3 vapor for a few minutes. Such an acidic/basic gas-triggered solid-state emission change was also observed in compounds 35d, 36b, and 36d (Liu et al., 2015; Wang et al., 2015b).
Summary and outlook
Among many organic fluorescent molecules, the synthesis of BODIPY fluorescent molecules and their analogs is relatively simple, and the advantages of photophysical properties are prominent, such as a high molar extinction coefficient, high quantum yield, tunable emission wavelength, and high stability. The methods of achieving AIE activity of classical BODIPY and BODIPY analogs mainly include linking AIE-active molecules on the chromophore core, the J-aggregation method, and designing fluorescent molecules into propeller-shaped structures. Because of their high luminescence efficiency in aggregates and solid-state, these molecules have been successfully applied to bioimaging, solid-state stimulus-responsive materials, OLEDs and other fields. However, some challenges still exist for the design and application of the AIE-active BODIPYs.
Fundamental understanding of the aggregation effect on photophysical property is not yet satisfactory. Mechanisms based on RIM and J-aggregation are generally applied in molecular design, and in some cases they do not work as well as expected yet. For example, integrating the TPE unit to BODIPY is generally thought to induce AIE, but ACQ behavior toward some BODIPYs bearing TPE units was reported (Gomez-Duran et al., 2015). On the other hand, J-aggregation of BODIPYs should result in emissive J-aggregates in the aggregation state; however, we recently demonstrated that the J-aggregation could generate multiple emissions across the red to NIR region (Tian et al., 2018).
Improving the fluorescent efficiency in the aggregation state is highly required. Although the ACQ effect of BODIPYs was suppressed by introducing an AIE unit, the aggregation-state Φf for most of AIE-active BODIPYs remained low, which restricted their further applications. Exploring a new strategy or a proper platform to achieve intense aggregation-state emission of BODIPYs remains a challenge.
There is huge scope in exploring AIE-active BODIPYs with NIR emission (700–1,700 nm). Compared to the large number of AIE-active BODIPYs with a short emission wavelength, successful examples of NIR emission are rather limited. Exploring the suitable building block and fine-tuning of the π-conjugated structures should be helpful for achieving NIR aggregation-state emission. Aggregation-state emissive BODIPYs, especially those with NIR-II (1,000–1,700 nm) emission, could have a bright future for in vivo and clinical imaging (Zhu et al., 2019).
Taken together, under the guidance of AIE, efficient aggregation-state emissive BODIPYs with diverse chemical structures and intriguing photophysical properties will be developed. These BODIPY derivatives will undoubtedly show their capabilities in various application fields.
Author Contributions
ZL and XW designed this proposal, revised the manuscript, and determined the contents. ZJ and MY drew the chemical structures and prepared the figures. All authors contributed to the writing of the manuscript.
Funding
This research was funded by the National Natural Science Foundation of China (21971115 and 21501085) and the Key University Science Research Project of Jiangsu Province (17KJA150004).
Conflict of Interest
The authors declare that the research was conducted in the absence of any commercial or financial relationships that could be construed as a potential conflict of interest.
Acknowledgments
We thank the National Natural Science Foundation of China and the Key University Science Research Project of Jiangsu Province.
References
Baglan, M., Ozturk, S., Gur, B., Meral, K., Bozkaya, U., Bozdemir, O. A., et al. (2013). Novel phenomena for aggregation induced emission enhancement: highly fluorescent hydrophobic tpe-bodipy couples in both organic and aqueous media. RSC Adv. 3, 15866–15874. doi: 10.1039/c3ra40791h
Baysec, S., Minotto, A., Klein, P., Poddi, S., Zampetti, A., Allard, S., et al. (2018). Tetraphenylethylene-bodipy aggregation-induced emission luminogens for near-infrared polymer light-emitting diodes. Sci. China Chem. 61, 932–939. doi: 10.1007/s11426-018-9306-2
Bergström, F., Mikhalyov, I., Hägglöf, P., Wortmann, R., Ny, T., and Johansson, L. B. (2002). Dimers of dipyrrometheneboron difluoride (bodipy) with light spectroscopic applications in chemistry and biology. J. Am. Chem. Soc. 124, 196–204. doi: 10.1021/ja010983f
Boens, N., Leen, V., and Dehaen, W. (2012). Fluorescent indicators based on bodipy. Chem. Soc. Rev. 41, 1130–1172. doi: 10.1039/C1CS15132K
Bui, H. T., Mai, D. K., Kim, B., Choi, K. H., Park, B. J., Kim, H. J., et al. (2019). Effect of substituents on the photophysical properties and bioimaging application of bodipy derivatives with triphenylamine substituents. J. Phys. Chem. B 123, 5601–5607. doi: 10.1021/acs.jpcb.9b04782
Chan, J., Dodani, S. C., and Chang, C. J. (2012). Reaction-based small-molecule fluorescent probes for chemoselective bioimaging. Nat. Chem. 4, 973–984. doi: 10.1038/nchem.1500
Che, W., Zhang, L., Li, Y., Zhu, D., Xie, Z., Li, G., et al. (2019). Ultrafast and non-invasive long-term bioimaging with highly stable red aggregation-induced emission nanoparticles. Anal. Chem. 91, 3467–3474. doi: 10.1021/acs.analchem.8b05024
Chen, S. J., Wang, H., Hong, Y. N., and Tang, B. Z. (2016). Fabrication of fluorescent nanoparticles based on aie luminogens (aie dots) and their applications in bioimaging. Mater. Horiz. 3, 283–293. doi: 10.1039/C6MH00060F
Choi, S., Bouffard, J., and Kim, Y. (2014). Aggregation-induced emission enhancement of a meso-trifluoromethyl bodipy via j-aggregation. Chem. Sci. 5, 751–755. doi: 10.1039/C3SC52495G
Chua, M. H., Ni, Y., Garai, M., Zheng, B., Huang, K. W., Xu, Q. H., et al. (2015). Towards meso-ester bodipys with aggregation-induced emission properties: the effect of substitution positions. Chem. Asian J. 10, 1631–1634. doi: 10.1002/asia.201500420
Duan, W., Liu, Q., Huo, Y., Cui, J., Gong, S., and Liu, Z. (2018). Aie-active boron complexes based on benzothiazole-hydrazone chelates. Org. Biomol. Chem. 16, 4977–4984. doi: 10.1039/C8OB00755A
Fan, C., Wu, W., Chruma, J. J., Zhao, J., and Yang, C. (2016). Enhanced triplet-triplet energy transfer and upconversion fluorescence through host-guest complexation. J. Am. Chem. Soc. 138, 15405–15412. doi: 10.1021/jacs.6b07946
Förster, T., and Kasper, K. (1954). Ein konzentrationsumschlag der fluoreszenz des pyrens. Z. Phys. Chem. 1, 275–277. doi: 10.1524/zpch.1954.1.5_6.275
Ge, Y., and O'shea, D. F. (2016). Azadipyrromethenes: from traditional dye chemistry to leading edge applications. Chem. Soc. Rev. 45, 3846–3864. doi: 10.1039/C6CS00200E
Gomez-Duran, C. F., Hu, R., Feng, G., Li, T., Bu, F., Arseneault, M., et al. (2015). Effect of aie substituents on the fluorescence of tetraphenylethene-containing bodipy derivatives. ACS Appl Mater Interfaces 7, 15168–15176. doi: 10.1021/acsami.5b05033
Gong, S., Liu, Q., Wang, X., Xia, B., Liu, Z., and He, W. (2015). Aie-active organoboron complexes with highly efficient solid-state luminescence and their application as gas sensitive materials. Dalton Trans. 44, 14063–14070. doi: 10.1039/C5DT01525A
Hu, R., Gomez-Duran, C. F., Lam, J. W., Belmonte-Vazquez, J. L., Deng, C., Chen, S., et al. (2012). Synthesis, solvatochromism, aggregation-induced emission and cell imaging of tetraphenylethene-containing bodipy derivatives with large stokes shifts. Chem. Commun. 48, 10099–10101. doi: 10.1039/c2cc35188a
Hu, R. R., Lager, E., Aguilar-Aguilar, A., Liu, J. Z., Lam, J. W. Y., Sung, H. H. Y., et al. (2009). Twisted intramolecular charge transfer and aggregation-induced emission of bodipy derivatives. J. Phys. Chem. C 113, 15845–15853. doi: 10.1021/jp902962h
Johnson, I., and Spence, M. T. Z. (2010). The Molecular Probes Hand-Book: A Guide to Fluorescent Probes and Labeling Technologies. Grand Island, NE: Life Technologies Corporation.
Kim, S., Bouffard, J., and Kim, Y. (2015). Tailoring the solid-state fluorescence emission of bodipy dyes by meso substitution. Chem. Eur. J. 21, 17459–17465. doi: 10.1002/chem.201503040
Kim, T. I., Hwang, B., Lee, B., Bae, J., and Kim, Y. (2018). Selective monitoring and imaging of eosinophil peroxidase activity with a j-aggregating probe. J. Am. Chem. Soc. 140, 11771–11776. doi: 10.1021/jacs.8b07073
Kokado, K., and Sada, K. (2019). Consideration of molecular structure in the excited state to design new luminogens with aggregation-induced emission. Angew. Chem. Int. Ed. 58, 8632–8639. doi: 10.1002/anie.201814462
Kowada, T., Maeda, H., and Kikuchi, K. (2015). Bodipy-based probes for the fluorescence imaging of biomolecules in living cells. Chem. Soc. Rev. 44, 4953–4972. doi: 10.1039/C5CS00030K
Kubota, Y., Ozaki, Y., Funabiki, K., and Matsui, M. (2013). Synthesis and fluorescence properties of pyrimidine mono- and bisboron complexes. J. Org. Chem. 78, 7058–7067. doi: 10.1021/jo400879g
Kubota, Y., Tanaka, S., Funabiki, K., and Matsui, M. (2012). Synthesis and fluorescence properties of thiazole-boron complexes bearing a beta-ketoiminate ligand. Org. Lett. 14, 4682–4685. doi: 10.1021/ol302179r
Lager, E., Liu, J., Aguilar-Aguilar, A., Tang, B. Z., and Pena-Cabrera, E. (2009). Novel meso-polyarylamine-bodipy hybrids: synthesis and study of their optical properties. J. Org. Chem. 74, 2053–2058. doi: 10.1021/jo802519b
Lavis, L. D., and Raines, R. T. (2008). Bright ideas for chemical biology. ACS Chem Biol 3, 142–155. doi: 10.1021/cb700248m
Li, L., Wang, L., Tang, H., and Cao, D. (2017). A facile synthesis of novel near-infrared pyrrolopyrrole aza-bodipy luminogens with aggregation-enhanced emission characteristics. Chem. Commun. 53, 8352–8355. doi: 10.1039/C7CC04568A
Li, X., Gao, X., Shi, W., and Ma, H. (2014). Design strategies for water-soluble small molecular chromogenic and fluorogenic probes. Chem. Rev. 114, 590–659. doi: 10.1021/cr300508p
Liao, C. W., Rao, M. R., and Sun, S. S. (2015). Structural diversity of new solid-state luminophores based on quinoxaline-beta-ketoiminate boron difluoride complexes with remarkable fluorescence switching properties. Chem. Commun. 51, 2656–2659. doi: 10.1039/C4CC08958H
Liu, Q., Wang, X., Yan, H., Wu, Y., Li, Z., Gong, S., et al. (2015). Benzothiazole-enamide-based bf2 complexes: luminophores exhibiting aggregation-induced emission, tunable emission and highly efficient solid-state emission. J. Mater. Chem. C 3, 2953–2959. doi: 10.1039/C4TC02876G
Loudet, A., and Burgess, K. (2007). Bodipy dyes and their derivatives: syntheses and spectroscopic properties. Chem. Rev. 107, 4891–4932. doi: 10.1021/cr078381n
Lu, H., Mack, J., Yang, Y., and Shen, Z. (2014). Structural modification strategies for the rational design of red/nir region bodipys. Chem. Soc. Rev. 43, 4778–4823. doi: 10.1039/C4CS00030G
Manzano, H., Esnal, I., Marqués-Matesanz, T., Bañuelos, J., López-Arbeloa, I., Ortiz, M. J., et al. (2016). Unprecedented j-aggregated dyes in pure organic solvents. Adv. Funct. Mater. 26, 2756–2769. doi: 10.1002/adfm.201505051
Mei, J., Leung, N. L., Kwok, R. T., Lam, J. W., and Tang, B. Z. (2015). Aggregation-induced emission: together we shine, united we soar! Chem. Rev. 115, 11718–11940. doi: 10.1021/acs.chemrev.5b00263
Qi, F., Lin, J., Wang, X., Cui, P., Yan, H., Gong, S., et al. (2016). New aie-active pyrimidine-based boronfluoride complexes with high solid-state emission and reversible mechanochromism luminescence behavior. Dalton Trans. 45, 7278–7284. doi: 10.1039/C6DT00292G
Qian, H., Cousins, M. E., Horak, E. H., Wakefield, A., Liptak, M. D., and Aprahamian, I. (2017). Suppression of kasha's rule as a mechanism for fluorescent molecular rotors and aggregation-induced emission. Nat. Chem. 9, 83–87. doi: 10.1038/nchem.2612
Sagara, Y., Yamane, S., Mitani, M., Weder, C., and Kato, T. (2016). Mechanoresponsive luminescent molecular assemblies: an emerging class of materials. Adv. Mater. 28, 1073–1095. doi: 10.1002/adma.201502589
Shimizu, S., Murayama, A., Haruyama, T., Iino, T., Mori, S., Furuta, H., et al. (2015). Benzo[c,d]indole-containing aza-bodipy dyes: asymmetrization-induced solid-state emission and aggregation-induced emission enhancement as new properties of a well-known chromophore. Chem. Eur. J. 21, 12996–13003. doi: 10.1002/chem.201501464
Tanaka, K., and Chujo, Y. (2015). Recent progress of optical functional nanomaterials based on organoboron complexes with β-diketonate, ketoiminate and diiminate. NPG Asia Mater. 7:e223. doi: 10.1038/am.2015.118
Tian, D., Qi, F., Ma, H., Wang, X., Pan, Y., Chen, R., et al. (2018). Domino-like multi-emissions across red and near infrared from solid-state 2-/2,6-aryl substituted bodipy dyes. Nat. Commun. 9:2688. doi: 10.1038/s41467-018-05040-8
Uoyama, H., Goushi, K., Shizu, K., Nomura, H., and Adachi, C. (2012). Highly efficient organic light-emitting diodes from delayed fluorescence. Nature 492, 234–238. doi: 10.1038/nature11687
Vu, T. T., Badré, S., Dumas-Verdes, C. C., Vachon, J.-J., Julien, C., Audebert, P., et al. (2009). New hindered bodipy derivatives: solution and amorphous state fluorescence properties. J. Phys. Chem. C 113, 11844–11855. doi: 10.1021/jp9019602
Vu, T. T., Dvorko, M., Schmidt, E. Y., Audibert, J.-F., Retailleau, P., Trofimov, B. A., et al. (2013). Understanding the spectroscopic properties and aggregation process of a new emitting boron dipyrromethene (bodipy). J. Phys. Chem. C 117, 5373–5385. doi: 10.1021/jp3097555
Wang, X., Liu, Q., Yan, H., Liu, Z., Yao, M., Zhang, Q., et al. (2015a). Piezochromic luminescence behaviors of two new benzothiazole-enamido boron difluoride complexes: intra- and inter-molecular effects induced by hydrostatic compression. Chem. Commun. 51, 7497–7500. doi: 10.1039/C5CC01902H
Wang, X., Wu, Y., Liu, Q., Li, Z., Yan, H., Ji, C., et al. (2015b). Aggregation-induced emission (aie) of pyridyl-enamido-based organoboron luminophores. Chem. Commun. 51, 784–787. doi: 10.1039/C4CC07451C
Wang, Z., Zhao, J., Barbon, A., Toffoletti, A., Liu, Y., An, Y., et al. (2017). Radical-enhanced intersystem crossing in new bodipy derivatives and application for efficient triplet-triplet annihilation upconversion. J. Am. Chem. Soc. 139, 7831–7842. doi: 10.1021/jacs.7b02063
Wu, Y., Li, Z., Liu, Q., Wang, X., Yan, H., Gong, S., et al. (2015). High solid-state luminescence in propeller-shaped aie-active pyridine-ketoiminate-boron complexes. Org. Biomol. Chem. 13, 5775–5782. doi: 10.1039/C5OB00607D
Wurthner, F., Kaiser, T. E., and Saha-Moller, C. R. (2011). J-aggregates: from serendipitous discovery to supramolecular engineering of functional dye materials. Angew. Chem. Int. Ed. 50, 3376–3410. doi: 10.1002/anie.201002307
Yan, J., Lee, S., Zhang, A., and Yoon, J. (2018). Self-immolative colorimetric, fluorescent and chemiluminescent chemosensors. Chem. Soc. Rev. 47, 6900–6916. doi: 10.1039/C7CS00841D
Yang, Y., Su, X., Carroll, C. N., and Aprahamian, I. (2012). Aggregation-induced emission in bf2-hydrazone (bodihy) complexes. Chem. Sci. 3, 610–613. doi: 10.1039/C1SC00658D
Yuan, K., Wang, X., Mellerup, S. K., Kozin, I., and Wang, S. (2017). Spiro-bodipys with a diaryl chelate: impact on aggregation and luminescence. J. Org. Chem. 82, 13481–13487. doi: 10.1021/acs.joc.7b02602
Zhao, Z. J., Chen, B., Geng, J. L., Chang, Z. F., Aparicio-Ixta, L., Nie, H., et al. (2014). Red emissive biocompatible nanoparticles from tetraphenylethene-decorated bodipy luminogens for two-photon excited fluorescence cellular imaging and mouse brain blood vascular visualization. Part. Part. Syst. Charact. 31, 481–491. doi: 10.1002/ppsc.201300223
Zhu, S., Tian, R., Antaris, A. L., Chen, X., and Dai, H. (2019). Near-infrared-ii molecular dyes for cancer imaging and surgery. Adv. Mater. 31, e1900321. doi: 10.1002/adma.201900321
Keywords: BODIPY, aggregation-induced emission, fluorescence, bioimaging, sensor
Citation: Liu Z, Jiang Z, Yan M and Wang X (2019) Recent Progress of BODIPY Dyes With Aggregation-Induced Emission. Front. Chem. 7:712. doi: 10.3389/fchem.2019.00712
Received: 02 August 2019; Accepted: 09 October 2019;
Published: 25 October 2019.
Edited by:
Hua Lu, Hangzhou Normal University, ChinaReviewed by:
Shaomin Ji, Guangdong University of Technology, ChinaWanhua Wu, Sichuan University, China
Copyright © 2019 Liu, Jiang, Yan and Wang. This is an open-access article distributed under the terms of the Creative Commons Attribution License (CC BY). The use, distribution or reproduction in other forums is permitted, provided the original author(s) and the copyright owner(s) are credited and that the original publication in this journal is cited, in accordance with accepted academic practice. No use, distribution or reproduction is permitted which does not comply with these terms.
*Correspondence: Zhipeng Liu, zpliu@njfu.edu.cn; Xiaoqing Wang, xqwang@njfu.edu.cn