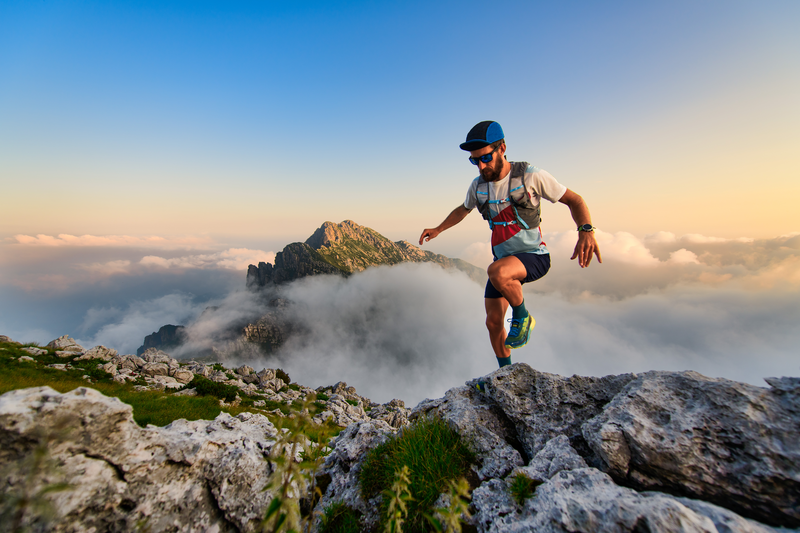
95% of researchers rate our articles as excellent or good
Learn more about the work of our research integrity team to safeguard the quality of each article we publish.
Find out more
ORIGINAL RESEARCH article
Front. Chem. , 23 October 2019
Sec. Green and Sustainable Chemistry
Volume 7 - 2019 | https://doi.org/10.3389/fchem.2019.00700
This article is part of the Research Topic Metal-Mediated and Metal-Catalyzed Reactions in Nonconventional Solvents View all 4 articles
A strong σ-donor mesoionic carbene ligand has been synthesized and applied to four different palladium-catalyzed cross-coupling transformations, proving the catalyst/medium compatibility and the increased activity of this system over previous reports in Deep Eutectic Solvent medium. Some cross-coupling processes could be carried out at room temperature and using aryl chlorides as starting materials. The possible implementation of multistep synthesis in eutectic mixtures has also been explored. The presence of palladium nanoparticles in the reaction media has been evaluated and correlated to the observed activity.
Organic Chemistry is, by definition, the study of carbon containing compounds. Thus, reactions that involve C-C bond formation have a great impact in this discipline. Due to this fact, C-C bond forming cross-coupling reactions have arisen as a main tool in Organic Synthesis. The importance and high applicability of this kind of reactions has been proven with the award of several Nobel Prizes in this area over the last years (Johansson Seechurn et al., 2012). Several metallic catalysts have been applied to these reactions, with palladium derived catalysts being the most employed (Biffis et al., 2018). This is the case for reactions as important as Suzuki (1985), Sonogashira (2002), Heck (1982), or Hiyama couplings (Hiyama, 2002).
Due to their enormous relevance, these reactions are performed nowadays in ton-scale, affording products of great importance in areas such as pharmaceuticals, fine chemical, and agrochemical industries (Torborg and Beller, 2009). For this reason, these reactions have been largely studied over the last decades and efficient catalysts have been developed. However, most of these cross-coupling reactions are performed using traditional volatile organic compounds (VOCs) as solvents. These solvents are usually flammable, toxic and persistent in the atmosphere, and their replacement by safer alternatives following Green Chemistry principles is highly desired (Anastas and Warner, 1998).
Some reports of cross-coupling reactions in neoteric solvents have been recently described, including the use of water (Chatterjee and Ward, 2016), γ-valerolactone (Ismalaj et al., 2014), glycerol (Reina et al., 2018), or supercritical carbon dioxide (Feng et al., 2010) as solvents. Recently, Deep Eutectic Solvents (DESs) have been explored as reaction media for cross-coupling reactions, with some advantages over the aforementioned solvents (García-Álvarez, 2014).
DESs are mixtures formed combining two or more components that display, usually strong hydrogen-bond interactions, affording a mixture with a melting point much lower than each of their individual components (Abbott et al., 2004). These solvents have a negligible vapor pressure and are non-flammable. Most of the components that are used to produce these eutectic mixtures are naturally-occurring, biodegradable, and biorenewable. Furthermore, there are supposed to exist more than one million possible mixtures (Hammond et al., 2017), affording the possibility of designing a solvent for each reaction. Due to their high versatility, tunability, and sustainability, their use as medium to carry out metal-catalyzed organic transformations has attracted the interest of organic chemists.
With DESs rising their popularity, it did not take long for cross coupling reactions to be tested in this novel reaction medium. Therefore, cross-coupling reactions in DESs have gained importance over the last few years as it is proven by the growing number of published articles in this research area. However, more efficient systems with broader applicability still have to be developed (Figure 1).
In order to improve the activity of a catalytic system, ligands are usually employed to tune the pre-catalyst properties. Considering the palladium-catalyzed cross coupling reactions in DES, first reports of the Suzuki (Imperato et al., 2006a), Sonogashira and Heck couplings (Ilgen and König, 2009) were performed in a ligand-free fashion, requiring high palladium loadings (up to 10 mol%). The Suzuki reaction in DES have been accomplished also with lower Pd(OAc)2 loadings by employing aryltrifluoroborates instead of boronic acids (Dilauro et al., 2018) as well as in the aminocarbonylation of aryl iodides (Messa et al., 2018). Our group have also contributed to this research field with the Hiyama reaction performed with a robust NCN-Pd pincer catalyst in ChCl:glycerol (Marset et al., 2018). However, the use of ionic phosphines seems to increase the compatibility of the palladium pre-catalysts with the reaction media, as it has been proven by the use of anionic TPPTS ligand, which was followed by enzymatic catalyzed reactions (Paris et al., 2018, 2019). Thus, the use of designed ligands seems mandatory for enhancing the activity of palladium catalysts in DES media. Early reports proved that arsines were better ligands than traditional phosphines when the Stille coupling was performed in DES (Imperato et al., 2006b). Our group also showed that α-cationic phosphine ligands greatly enhanced the activity of PdCl2 in the Suzuki, Heck and Sonogashira couplings performed in eutectic mixtures, while traditional non-ionic phosphines were not adequate (Marset et al., 2017). Those α-cationic phosphines have lower σ-donor ability compared to traditional phosphines, while they π-acceptor character is enhanced. This property could improve the catalyst activity in elementary steps such as reductive elimination or coordination of substrates to the metal center due to its electron-poor character (Alcarazo, 2016; Nicholls and Alcarazo, 2019).
The catalytic activity of metal complexes in cross-coupling reactions is often improved by the use of ligands with strong electron-donating properties. For this reason, in an attempt to increase the activity of DES-compatible catalysts the synthesis of a N-heterocyclic carbene (NHC)-Pd complex was carried out. NHC ligands, as well as phosphines, are strong σ-donors and weak π-acceptors. However, abnormal N-heterocyclic carbenes (aNHC) are reported to be even stronger σ-donors ligands (Crabtree, 2013). This stronger σ-donors ability increase the electron density at the metal center, enhancing the propensity of the metal to undergo oxidative addition (Krueger and Albrecht, 2011; Donnelly et al., 2013; Albrecht, 2014). To the best of our knowledge, the use of aNHC-Pd complexes in DESs as reaction medium has not been explored so far. For this reason, we aimed to synthetize an abnormal NHC-Pd complex and test it in different cross coupling reactions performed in DES.
Complex 6 was prepared according to literature procedures (Scheme 1). The first step involved a multicomponent click-type reaction to afford triazole 4, which was subjected to an alkylation reaction, yielding the salt 5. Ligand 5 was then, deprotonated with potassium tert-butoxide and treated with a palladium precursor. Finally, an excess of NaI was added to afford complex 6.
Once the catalyst was prepared, its ability to catalyze the Suzuki coupling was tested (Supplementary Table S1). The reaction between 4′-bromoacetophenone and phenylboronic acid was chosen as model reaction. A 40% yield of product 9a was obtained after 3 h using water as solvent, while only 12% yield was observed using ChCl:ethylene glycol (1:2) as reaction media. Water is, obviously, a non-toxic and inexpensive solvent, but dealing with aqueous residues is not an easy task. For this reason, the use of DESs as reaction media has an increased value. It is worth to mention that eutectic mixtures can maintain their structure even when small amounts of water are added to the mixture, while their physical properties are modulated (Hammond et al., 2017). For this reason, it was decided to add certain amounts of water to the reaction DES mixture, observing an increase in the reaction rate when 1 to 10 equivalents of water were added, while adding 20 equivalents decreased the reaction yield (Supplementary Table S1, entries 2–9). Then other solvents (entries 10–20) and bases (entries 21–27) were tested, obtaining the best results with ChCl:ethylene glycol, 10 equivalents of water and 1.5 equivalents of K2CO3, as base (99% yield). With the optimal conditions in hand, the scope of aryl halides was evaluated (Table 1). The reaction worked with moderate to excellent yields for aryl bromides bearing electro-withdrawing and electro-donating functional groups at different positions. Electron-poor aryl chlorides were also efficiently coupled with phenylboronic acids with moderate to good yields at room temperature in 3 h.
On the other hand, aryl boronic acids bearing different substituents, such as alkyl, hydroxyl or halide groups, were coupled with 4′-bromoacetophenone with good to excellent yields (Table 2).
Since recent reports have proven the compatibility of DES with the use of organometallic reagents such as organolithium or organomagnesium compounds (Vidal et al., 2014, 2016; García-Álvarez et al., 2015, 2018; Rodríguez-Álvarez et al., 2018), we decided to test the cross-coupling products 9b and 9c as substrates for the addition of different organometallic species in ChCl:ethyleneglycol as solvent. Aldehyde 9b could be reduced to the corresponding primary alcohol with NaBH4 (Table 3, entry 1) or coupled with n-BuLi (entry 4). Better results were obtained with ketone 9c, observing the aforementioned transformations (entries 5 and 8), as well as the addition of Grignard reagents (entries 6–7). These results highlighted the possibility of implementing DESs in a broad spectrum of different synthetic steps for the preparation of any product.
To further prove the activity of the catalyst, other cross-coupling reactions were tested. The Sonogashira reaction was optimized with catalyst 6 in DESs as reaction media, obtaining the best results with AcChCl:urea (1:2) as solvent and iPrNH2 as base in 2 h at room temperature, using only 1 mol% of complex 6 (95% yield, Supplementary Table S2). Then, the scope of the reaction was evaluated, obtaining moderate to excellent yields for the coupling of different aryl iodides with phenylacetylene (Table 4). 4′-Bromoacetophenone and 4′-chloroacetophenone were also submitted to the reaction with phenylacetylene but they failed. Excellent yields were obtained for electron-poor aryl iodides, although the yield was lower for neutral or electron rich aryl halides. However, results were improved by increasing the reaction time or the reaction temperature from rt to 80°C, which proved the stability of the catalyst at higher temperatures.
The Heck coupling was also evaluated. As in the previous reaction, AcChCl:urea (1:2) proved to be the best solvent after a thoughtful optimization process (99% yield, Supplementary Table S3). Good to excellent results were obtained for the coupling of electron-poor aryl iodides with different substitution patterns with methylacrylate, using 1.5 equiv. of NaOAc as base at 120°C and only 1 mol% of complex 6 (Table 5, entries 1–4). In the case of using electron-rich starting materials, the reaction time was increased to 16 h, obtaining the corresponding cross-coupling products with good to excellent yields (entries 6–8). Also, in this case aryl bromides (4′-bromoacetophenone) and aryl chlorides (4′-chloroacetophenone) failed.
The biaryl synthesis through cross-coupling reactions is usually performed under Suzuki conditions. Nevertheless, Hiyama reaction offers certain advantages from a sustainable and economic point of view, although organosilanes are typically less reactive than the corresponding boron counterparts. For this reason, it was decided to study the Hiyama reaction between aryl bromides and phenyltrimethoxysilane. The best result was obtained using ChCl:glycerol (1:2) as solvent, 1.5 equiv. of K2CO3 as base at 100°C for 24 h (70% yield, Supplementary Table S4). Good to excellent yields were observed for both, electron-poor (Table 6, entries 1–4) and electron-rich aryl bromides (entries 5–6). Furthermore, the reaction was compatible with heteroaryl bromides, affording the corresponding heterobiaryls in excellent yields (entries 7–9).
An attempt to reuse the catalyst and solvent by extracting the organic products with 2-MeTHF for the Suzuki and Hiyama reactions was performed. However, important drops in the reaction yields were observed in the second cycle (from 92 and 95% to 36 and 40%, respectively). Probably the poor activity of the recycled system could be explained by the formation of inactive palladium black, as well as the iodine poisoning of palladium nanoparticles (Cano et al., 2016).
In addition, with the aim of understanding better the catalytic process, a mercury poisoning test was performed. Thus, if the reactions were catalyzed by palladium nanoparticles (NPs), an important drop in the reaction yield should be noticed (Sigeev et al., 2015). The four cross-coupling reactions studied were analyzed again under optimal conditions but with the addition of 250 mol% of Hg(0). A complete inhibition of the catalytic activity was observed in the cases of the Suzuki, Sonogashira and Heck cross-coupling reactions. Surprisingly, no inhibition at all was observed in the case of the Hiyama coupling. A similar result was previously observed employing a Pd NCN-pincer in DES (Marset et al., 2018).
Since the formation of palladium nanoparticles seemed to be vital for the outcome of the desired organic transformations, the crude materials of the standard reactions were analyzed by electron transmission microscopy (TEM) to characterize those NPs (Figure 2). The size distributions of the observed nanoparticles are summarized in Table 7. Similar results were obtained in the cases of Suzuki and Sonogashira reactions. Although the solvent and base employed were different for each transformation (Suzuki and Sonogashira), a similar size of the nanoparticles was observed in both cases. In the case of Heck reaction, the average size of the nanoparticles was smaller, probably due to the higher temperature of the reaction and the different composition of the mixture. On the other hand, bigger NPs were found in the crude material of the Hiyama reaction (performed at 100°C). However, the longer reaction time required for this transformation could favor the NP aggregation, which may also explain the recyclability issues.
Figure 2. TEM images of the crude media after (a) Suzuki, (b) Sonogashira, (c) Heck, and (d) Hiyama cross-coupling reactions.
In order to better understanding the nature of the catalytic process kinetic studies and XPS analysis were performed (Figure 3). In the Suzuki kinetic plot an induction period was observed. This result seems to indicate that until palladium nanoparticles were not formed, the reaction did not occur. So, catalyst 6 plays a pre-catalyst role and palladium nanoparticles seems to be the real catalyst which is in consonance with the mercury test. However, in the Hiyama reaction any induction period was observed even decreasing the catalytic amount. Pre-formed palladium nanoparticles were employed to carry out Hiyama reaction under the optimized conditions but no catalysis was observed. So, this result seems to indicate that soluble palladium species with coordinated NHC ligands might be considered as the catalyst for the reaction through a Pd(II)-Pd(IV) catalytic process (Szulmanowicz et al., 2013; Modak et al., 2015; Mondal et al., 2018).
Figure 3. (A) Kinetic plot of the Suzuki cross-coupling reaction, (B) Kinect plot of the Hiyama cross-coupling reaction.
Also, XPS analysis (see Supplementary Material) shows that the oxidation state of the initial catalyst 6 changes completely to palladium(0) and palladium(II) oxide species, after the Suzuki reaction under the optimized conditions.
The use of a mesoionic carbene ligand in palladium-catalyzed cross-coupling reactions has been evaluated for the first time in Deep Eutectic Solvents as reaction media, proving to be an excellent and general pre-catalyst (it decomposes to PdNPs) for many different cross-coupling reactions. The electronic properties of the reported palladium complex have allowed to carry out the Suzuki cross-coupling reaction in the aforementioned neoteric solvents at room temperature, even using aryl chlorides as reagents. Furthermore, the Sonogashira, Heck and Hiyama reactions have been also optimized and their scope evaluated, proving the great applicability of the mesoionic carbene-palladium complex. In addition, the reaction media is compatible with the use of organometallic reagents, demonstrating the possibility of implementing DESs in a broad spectrum of synthetic steps. The low catalyst loading, the sustainable properties of the employed solvents and the vast scope analyzed demonstrate that the use of this catalyst in combination with DESs as reaction media is an interesting protocol for carrying out any type of Csp2-Csp2(sp) bond forming transformations. However, is still necessary to improve these protocols using DESs as solvents, to overcome the catalyst loading amount obtained in classical organic solvents.
All datasets for this study are included in the manuscript/Supplementary Material.
GG and DR contributed conception and design of the study. XM, BS, NG-G, AB, ML, and RL performed the synthetic experiments and characterization of products. XM, BS, and NG-G wrote the first draft of the manuscript. All authors contributed to manuscript revision, read, and approved the submitted version.
This work was supported by the Spanish Ministerio de Economía, Industria y Competitividad (CTQ2015-66624-P and PGC2018-096616-B-I00) and the University of Alicante (VIGROB-316).
The authors declare that the research was conducted in the absence of any commercial or financial relationships that could be construed as a potential conflict of interest.
XM and BS thank Generalitat Valenciana (ACIF/2016/057 and ACIF/2017/211) for their fellowships. ML thanks MINECO (PEJ-2014-P-00290) for his fellowship.
The Supplementary Material for this article can be found online at: https://www.frontiersin.org/articles/10.3389/fchem.2019.00700/full#supplementary-material
Abbott, A. P., Boothby, D., Capper, G., Davies, D. L., and Rasheed, R. K. (2004). Deep eutectic solvents formed between choline chloride and carboxylic acids: versatile alternatives to ionic liquids. J. Am. Chem. Soc. 126, 9142–9147. doi: 10.1021/ja048266j
Albrecht, M. (2014). Normal and abnormal N-heterocyclic carbene ligands: similarities and differences of mesoionic C-donor complexes. Adv. Organomet. Chem. 62, 111–158. doi: 10.1016/B978-0-12-800976-5.00002-3
Alcarazo, M. (2016). Synthesis, structure, and applications of α-cationic phosphines. Acc. Chem. Res. 49, 1797–1805. doi: 10.1021/acs.accounts.6b00262
Anastas, P. T., and Warner, J. C. (1998). Green Chemistry : Theory and Practice. Oxford: Oxford University Press.
Biffis, A., Centomo, P., Del Zotto, A., and Zecca, M. (2018). Pd metal catalysts for cross-couplings and related reactions in the 21st century: a critical review. Chem. Rev. 118, 2249–2295. doi: 10.1021/acs.chemrev.7b00443
Cano, R., Pérez, J. M., Ramón, D. J., and McGlacken, G. P. (2016). Impregnated palladium on magnetite as catalyst for direct arylation of heterocycles. Tetrahedron 72, 1043–1050. doi: 10.1016/j.tet.2015.12.039
Chatterjee, A., and Ward, T. R. (2016). Recent advances in the palladium catalyzed Suzuki-Miyaura cross-coupling reaction in water. Catal. Lett. 146, 820–840. doi: 10.1007/s10562-016-1707-8
Crabtree, R. H. (2013). Abnormal, mesoionic and remote N-heterocyclic carbene complexes. Coord. Chem. Rev. 257, 755–766. doi: 10.1016/j.ccr.2012.09.006
Dilauro, G., García, S. M., Tagarelli, D., Vitale, P., Perna, F. M., and Capriati, V. (2018). Ligand-free bioinspired suzuki-miyaura coupling reactions using aryltrifluoroborates as effective partners in deep eutectic solvents. ChemSusChem 11, 3495–3501. doi: 10.1002/cssc.201801382
Donnelly, K. F., Petronilho, A., and Albrecht, M. (2013). Application of 1,2,3-triazolylidenes as versatile NHC-type ligands: synthesis, properties, and application in catalysis and beyond. Chem. Commun. 49, 1145–1159. doi: 10.1039/C2CC37881G
Feng, X., Yan, M., Zhang, T., Liu, Y., and Bao, M. (2010). Preparation and application of SBA-15-supported palladium catalyst for Suzuki reaction in supercritical carbon dioxide. Green Chem. 12, 1758–1766. doi: 10.1039/c004250a
García-Álvarez, J. (2014). Deep eutectic solvents: environmentally friendly media for metal-catalyzed organic reactions. ACS Symp. Ser. 1186, 37–52. doi: 10.1021/bk-2014-1186.ch003
García-Álvarez, J., Hevia, E., and Capriati, V. (2015). Reactivity of polar organometallic compounds in unconventional reaction media: challenges and opportunities. Eur. J. Org. Chem. 2015, 6779–6799. doi: 10.1002/ejoc.201500757
García-Álvarez, J., Hevia, E., and Capriati, V. (2018). The future of polar organometallic chemistry written in bio-based solvents and water. Chem. Eur. J. 24, 14854–14863. doi: 10.1002/chem.201802873
Hammond, O. S., Bowron, D. T., and Edler, K. J. (2017). The effect of water upon deep eutectic solvent nanostructure: an unusual transition from ionic mixture to aqueous solution. Angew. Chem. Int. Ed. 56, 9782–9785. doi: 10.1002/anie.201702486
Heck, R. F. (1982). Palladium-catalyzed vinylation of organic halides. Org. React. 27, 345–390. doi: 10.1002/0471264180.or027.02
Hiyama, T. (2002). How I came across the silicon-based cross-coupling reaction. J. Organomet. Chem. 653, 58–61. doi: 10.1016/S0022-328X(02)01157-9
Ilgen, F., and König, B. (2009). Organic reactions in low melting mixtures based on carbohydrates and L-carnitine - a comparison. Green Chem. 11, 848–854. doi: 10.1039/b816551c
Imperato, G., Hoeger, S., Lenoir, D., and König, B. (2006a). Low melting sugar-urea-salt mixtures as solvents for organic reactions-estimation of polarity and use in catalysis. Green Chem. 8, 1051–1055. doi: 10.1039/B603660K
Imperato, G., Vasold, R., and König, B. (2006b). Stille reactions with tetraalkylstannanes and phenyltrialkylstannanes in low melting sugar-urea-salt mixtures. Adv. Synth. Catal. 348, 2243–2247. doi: 10.1002/adsc.200600248
Ismalaj, E., Strappaveccia, G., Ballerini, E., Elisei, F., Piermatti, O., Gelman, D., et al. (2014). γ-Valerolactone as a renewable dipolar aprotic solvent deriving from biomass degradation for the Hiyama reaction. ACS Sustain. Chem. Eng. 2, 2461–2464. doi: 10.1021/sc5004727
Johansson Seechurn, C. C., Kitching, M. O., Colacot, T. J., and Snieckus, V. (2012). Palladium-catalyzed cross-coupling: a historical contextual perspective to the 2010 nobel prize. Angew. Chem. Int. Ed. 51, 5062–5085. doi: 10.1002/anie.201107017
Krueger, A., and Albrecht, M. (2011). Abnormal N-heterocyclic carbenes: more than just exceptionally strong donor ligands. Aust. J. Chem. 64, 1113–1117. doi: 10.1071/CH11265
Marset, X., De Gea, S., Guillena, G., and Ramón, D. J. (2018). NCN-Pincer-Pd complex as catalyst for the hiyama reaction in biomass-derived solvents. ACS Sustain. Chem. Eng. 6, 5743–5748. doi: 10.1021/acssuschemeng.8b00598
Marset, X., Khoshnood, A., Sotorrios, L., Gómez-Bengoa, E., Alonso, D. A., and Ramón, D. J. (2017). Deep eutectic solvent compatible metallic catalysts: cationic pyridiniophosphine ligands in palladium catalyzed cross-coupling reactions. ChemCatChem 9, 1269–1275. doi: 10.1002/cctc.201601544
Messa, F., Perrone, S., Capua, M., Tolomeo, F., Troisi, L., Capriati, V., et al. (2018). Towards a sustainable synthesis of amides: chemoselective palladium-catalysed aminocarbonylation of aryl iodides in deep eutectic solvents. Chem. Commun. 54, 8100–8103. doi: 10.1039/C8CC03858A
Modak, S., Gangwar, M. K., Nageswar Rao, M., Madasu, M., Kalita, A. C. h., Dorcet, V., et al. (2015). Fluoride-free Hiyama coupling by palladium abnormal N-heterocyclic carbene complexes. Dalton Trans. 44, 17617–17628. doi: 10.1039/C5DT02317C
Mondal, T., De, S., Dutta, S., and Koley, D. (2018). Mechanistic exploration of the transmetalation and reductive elimination events involving PdIV–abnormal NHC complexes in Suzuki–Miyaura coupling reactions: a DFT study. Chem. Eur. J. 24, 6155–6168. doi: 10.1002/chem.201800024
Nicholls, L. D. M., and Alcarazo, M. (2019). Applications of α-cationic phosphines as ancillary ligands in homogeneous catalysis. Chem. Lett. 48, 1–13. doi: 10.1246/cl.180810
Paris, J., Ríos-Lombardia, N., Moris, F., Groeger, H., and Gónzalez-Sabin, J. (2018). Novel insights into the combination of metal- and biocatalysis: cascade one-pot synthesis of enantiomerically pure biaryl alcohols in deep eutectic solvents. ChemCatChem 10, 4417–4423. doi: 10.1002/cctc.201800768
Paris, J., Telzerow, A., Ríos-Lombardía, N., Steiner, K., Schwab, H., Morís, F., et al. (2019). Enantioselective one-pot synthesis of biaryl-substituted amines by combining palladium and enzyme catalysis in deep eutectic solvents. ACS Sustain. Chem. Eng. 7, 5486–5493. doi: 10.1021/acssuschemeng.8b06715
Reina, A., Serrano-Maldonado, A., Teuma, E., Martín, E., and Gómez, M. (2018). Palladium nanocatalysts in glycerol: tuning the reactivity by effect of the stabilizer. Catal. Commun. 104, 22–27. doi: 10.1016/j.catcom.2017.10.004
Rodríguez-Álvarez, M. J., García-Álvarez, J., Uzelac, M., Fairley, M., O'Hara, C. T., and Hevia, E. (2018). Introducing glycerol as a sustainable solvent to organolithium chemistry: ultrafast chemoselective addition of aryllithium reagents to nitriles under air and at ambient temperature. Chem. Eur. J. 24, 1720–1725. doi: 10.1002/chem.201705577
Sigeev, A. S., Peregudov, A. S., Cheprakov, A. V., and Beletskaya, I. P. (2015). The palladium slow-release pre-catalysts and nanoparticles in the “Phosphine-free” Mizoroki–Heck and Suzuki–Miyaura reactions. Adv. Synth. Catal. 357, 417–429. doi: 10.1002/adsc.201400562
Sonogashira, K. (2002). Development of Pd-Cu catalyzed cross-coupling of terminal acetylenes with sp2-carbon halides. J. Organomet. Chem. 653, 46–49. doi: 10.1016/S0022-328X(02)01158-0
Suzuki, A. (1985). Organoboron compounds in new synthetic reactions. Pure Appl. Chem. 57, 1749–1758. doi: 10.1351/pac198557121749
Szulmanowicz, M. S., Gniewek, A., Gil, W., and Trzeciak, A. M. (2013). Palladium(II) complexes with small N-heterocyclic carbene ligands as highly active catalysts for the Suzuki–Miyaura cross-coupling reaction. ChemCatChem 5, 1152–1160. doi: 10.1002/cctc.201200428
Torborg, C., and Beller, M. (2009). Recent applications of palladium-catalyzed coupling reactions in the pharmaceutical, agrochemical, and fine chemical industries. Adv. Synth. Catal. 351, 3027–3043. doi: 10.1002/adsc.200900587
Vidal, C., García-Álvarez, J., Hernán-Gómez, A., Kennedy, A. R., and Hevia, E. (2014). Introducing deep eutectic solvents to polar organometallic chemistry: chemoselective addition of organolithium and Grignard reagents to ketones in air. Angew. Chem. Int. Ed. 53, 5969–5973. doi: 10.1002/anie.201400889
Vidal, C., García-Álvarez, J., Hernán-Gómez, A., Kennedy, A. R., and Hevia, E. (2016). Exploiting deep eutectic solvents and organolithium reagent partnerships: chemoselective ultrafast addition to imines and quinolines under aerobic ambient temperature conditions. Angew. Chem. Int. Ed. 55, 16145–16148. doi: 10.1002/anie.201609929
Keywords: mesoionic carbene, palladium, Deep Eutectic Solvent, sustainability, cross-coupling reaction
Citation: Marset X, Saavedra B, González-Gallardo N, Beaton A, León MM, Luna R, Ramón DJ and Guillena G (2019) Palladium Mesoionic Carbene Pre-catalyst for General Cross-Coupling Transformations in Deep Eutectic Solvents. Front. Chem. 7:700. doi: 10.3389/fchem.2019.00700
Received: 11 June 2019; Accepted: 08 October 2019;
Published: 23 October 2019.
Edited by:
Vito Capriati, University of Bari Aldo Moro, ItalyReviewed by:
Viktoria H. Gessner, Ruhr University Bochum, GermanyCopyright © 2019 Marset, Saavedra, González-Gallardo, Beaton, León, Luna, Ramón and Guillena. This is an open-access article distributed under the terms of the Creative Commons Attribution License (CC BY). The use, distribution or reproduction in other forums is permitted, provided the original author(s) and the copyright owner(s) are credited and that the original publication in this journal is cited, in accordance with accepted academic practice. No use, distribution or reproduction is permitted which does not comply with these terms.
*Correspondence: Diego J. Ramón, ZGpyYW1vbkB1YS5lcw==; Gabriela Guillena, Z2FicmllbGEuZ3VpbGxlbmFAdWEuZXM=
Disclaimer: All claims expressed in this article are solely those of the authors and do not necessarily represent those of their affiliated organizations, or those of the publisher, the editors and the reviewers. Any product that may be evaluated in this article or claim that may be made by its manufacturer is not guaranteed or endorsed by the publisher.
Research integrity at Frontiers
Learn more about the work of our research integrity team to safeguard the quality of each article we publish.