- 1Department of Pharmacy and Biotechnology, University of Bologna, Bologna, Italy
- 2Department of Chemistry “G. Ciamician,” University of Bologna, Bologna, Italy
Among the other members of the adhesion molecules' family, α4β1 integrin, a heterodimeric receptor, plays a crucial role in inflammatory diseases, cancer development, metastasis and stem cell mobilization or retention. In many cases, its function in pathogenesis is not yet completely understood and investigations on ligand binding and related stabilization of active/inactive conformations still represent an important goal. For this reason, starting from the highlight of α4β1 functions in human pathologies, we report an overview of synthetic α4β1 integrin ligands under development as potential therapeutic agents. The small molecule library that we have selected represents a collection of lead compounds. These molecules are the object of future refinement in academic and industrial research, in order to achieve a fine tuning of α4β1 integrin regulation for the development of novel agents against pathologies still eluding an effective solution.
Introduction
Integrins represent one of the most important families of cell adhesion receptors that mediate cell-cell and cell-extracellular matrix interactions. Integrins are heterodimeric transmembrane proteins composed by stable non-covalent association between α and β subunit. In mammals, 24 possible heterodimers have been identified, deriving from differential combination of 18 α subunits and 8 β subunits (Humphries et al., 2006).
Integrins propagate signals bidirectionally across cell membranes (Abram and Lowell, 2009; Ley et al., 2016) (Figure 1A) and can be classified on the basis of the combination of α and β subunit (Tolomelli et al., 2017). The α4 subunit can couple with either β7 or β1 subunits. α4β1 integrin (also known as very late antigen-4, VLA-4) is expressed on leukocytes (lymphocytes, eosinophils, monocytes, macrophages, natural killer cells, basophils, and mast cells) and mediates homing, trafficking, differentiation, activation, and survival of α4β1 expressing cells (Hemler et al., 1987; Chan et al., 2001; Baiula et al., 2011; Mitroulis et al., 2015). VCAM-1 (vascular cell adhesion molecule-1), MAdCAM-1 (mucosal vascular addressin cell adhesion molecule-1), fibronectin and JAM-B (junctional adhesion molecule-B) are physiological ligands for α4β1 integrin (Imhof and Aurrand-Lions, 2004).
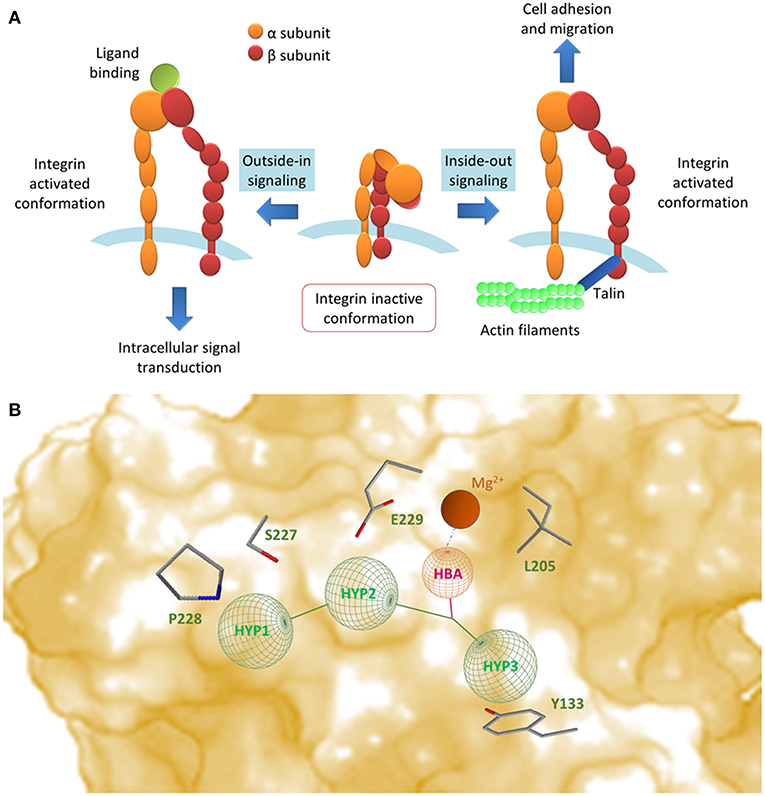
Figure 1. (A) Integrins propagate signals bidirectionally across cell membrane: conformational changes in their extracellular domains can occur as a consequence of signaling events happening inside the cells (inside-out signaling); these events lead to an increase in affinity (integrin activation) and therefore lead to ligand binding and cell adhesion. On the contrary, outside-in signaling represents the process in which ligand binding and ligand-induced integrin clustering lead to integrin-mediated intracellular signal transduction (Abram and Lowell, 2009; Ley et al., 2016). (B) Schematic representation of small molecule ligand binding mode to α4β1 integrin, obtained by combining the models reported in the literature. Side chains of selected residues in the β1 unit have been indicated. HBA, hydrogen bond acceptor; HYP1, valine mimetic hydrophobic pocket; HYP2-3, leucine mimetic hydrophobic pockets.
Targeting integrins has already proven to be a successful therapeutic strategy, with several agents approved for clinical practice. In this review, starting from the highlight of α4β1 functions in human pathology, we will give an overview of α4β1 integrin ligands under development as therapeutic agents.
α4β1 Integrin as Therapeutic Target in Inflammatory Disorders
The α4β1 integrin plays a crucial role in inflammation. Extravasation is a multistep process consisting of leukocytes recruitment to inflamed tissue. The α4β1 integrin is mainly involved in the phases of leukocytes tethering and rolling on activated endothelial cells and in arrest and adhesion strengthening through the interaction with adhesion molecules such as VCAM-1 (Herter and Zarbock, 2019). Moreover, α4β1 integrin can bind JAM-B, expressed on endothelial cells, to enable transendothelial migration (Imhof and Aurrand-Lions, 2004). Therefore, targeting α4β1 integrin could be valuable for the treatment of inflammatory disorder, since α4β1 represents an absolute requirement for extravasation.
Of interest is the role of α4β1 in leukocyte homing to the CNS: this integrin is required for T cell migration across the blood brain barrier to the brain, and blocking α4β1 resulted in the inhibition of experimental autoimmune encephalitis (Kanwar et al., 2000).
In the following sections we will present a brief overview of the pathogenesis of the main inflammatory disorders involving α4β1 integrin.
Multiple Sclerosis
Multiple sclerosis (MS) is a chronic inflammatory, autoimmune, demyelinating, and neurodegenerative disease of CNS. The pathogenesis of MS is very complex and not fully disclosed. Activated T lymphocytes are recruited from the blood into the CNS, through the interaction between α4β1 and VCAM-1, and release pro-inflammatory cytokines causing an inflammatory reaction that leads to neurodegeneration (Dargahi et al., 2017). Blocking α4 integrin results in inhibition of trafficking of T cells from the blood to CNS: natalizumab is a humanized monoclonal antibody (mAb) that binds to α4 subunit and thus blocks both α4β1 and α4β7; it has been approved for the treatment of highly active relapsing and remitting MS (Clerico et al., 2017) and Crohn's disease (see below). However, progressive multifocal encephalopathy (PML) occurred as a fatal adverse effect of natalizumab (Shirani and Stüve, 2017).
Inflammatory Bowel Diseases
Inflammatory bowel diseases (IBD), including ulcerative colitis (UC), and Crohn's disease (CD), are chronic relapsing inflammatory disorders of the gut (Zundler et al., 2017a). IBD pathogenesis is not completely understood, and comprises several factors: among them, infiltration of immune cells in the gut plays a pivotal role. T lymphocytes homing to the gut is mainly mediated by α4β7-MAdCAM-1 interaction. Consequently, vedolizumab, a humanized mAb anti-α4β7, has been developed and approved for the treatment of both UC and CD (Feagan et al., 2013; Sandborn et al., 2013). In addition, α4β1 integrin contributes to the infiltration of T cells to the inflamed intestinal tissue (Zundler et al., 2017b). Natalizumab, targeting α4 integrin subunit, has been approved for the treatment of MS and for CD, although due to fatal adverse reactions, its use is very limited for CD (Li et al., 2018; Nelson et al., 2018). Recently it has been observed that vedolizumab did not cause a decrement in homing of T cells into the gut, which instead was achieved by blocking α4β1. These data suggest that α4β1-dependent homing can represent a compensatory mechanism to evade α4β7 blockade. It is not still known if this mechanism is clinically relevant for CD (Zundler et al., 2017b).
Allergic Conjunctivitis
Allergic conjunctivitis is the most common form of ocular allergy (Baiula et al., 2011; Baiula and Spampinato, 2014). This disease is mainly characterized by an inflammatory response of the conjunctival mucosa that leads, through the interaction of integrins with adhesion molecules, to a long-term infiltration of neutrophils, eosinophils and T lymphocytes. Integrin α4β1 is strongly involved in the recruitment of circulating cells at the inflamed conjunctiva, contributing to both rolling and firm adhesion (Bacon et al., 1998). The reduction of α4β1 expression at conjunctival level, is part of the mechanism of action of the antihistamine levocabastine (Qasem et al., 2008).
Dry Eye Disease
Dry eye disease (DED) is a common cause of ocular discomfort and visual disturbance (Miljanović et al., 2007). DED is associated with ocular surface inflammation characterized by infiltration of T cells and overexpression of inflammatory mediators although the pathogenesis is not fully understood. α4β1 integrin blockade, using small molecule α4β1 antagonists, strongly reduced T cell infiltration into the ocular surface and ameliorated ocular signs in in vivo models of DED (Ecoiffier et al., 2008; Krauss et al., 2015).
Asthma
Asthma is a chronic inflammatory disease of the lower respiratory tract (Mims, 2015). α4β1 integrin, expressed on inflammatory cells, participates in the pathogenesis of asthma (Ohashi et al., 1992) and sarcoidosis, a disorder characterized by lymphocyte accumulation in the lung (Berlin et al., 1998). Several α4β1 antagonists have been developed but they lack efficacy in clinical trials (Teoh et al., 2015).
Stem Cell Mobilization or Retention
Hematopoietic stem cell (HSC) express several integrins, including α4β1 which is involved in the regulation of HSC homing and retention within the bone marrow niche (Grassinger et al., 2009). Novel agents able to mobilize HSC and progenitor cells are actively searched and clinically important to obtain cells from healthy donors for transplantation. The blockade of α4β1/α9β1 with a dual antagonist induced a rapid and transient mobilization of HSC (Cao et al., 2016). Moreover, bortezomib, a proteasome inhibitor that blocks the expression of VCAM-1, had a mobilizing effect by the modulation of α4β1/VCAM-1 axis (Ghobadi et al., 2014). This strategy based on the blockade of α4β1 integrin has shown great promise also for HSC transplantation in utero.
On the contrary, the activation of α4β1 may be a promising strategy to improve cell retention and engraftment in stem cell-based therapies (Vanderslice et al., 2013). The homing of endothelial progenitor cells to sites of ischemia, regulated by α4β1 integrin, has been shown to promote neovascularization in ischemic tissue (Duan et al., 2006).
Cancer and Metastasis
Several types of tumor cells express α4β1 integrin and the interaction with its ligand VCAM-1 increases transendothelial migration and contributes to metastasis to distant organs (Schlesinger and Bendas, 2015). Moreover, an aberrant expression of VCAM-1 has been observed in tumor cells. In breast cancer cells, VCAM-1 seems to confer an increased ability to metastasize to the bones and the lungs (Vanharanta and Massagu, 2013). In addition, α4β1 plays an important role in tumor angiogenesis, as do other integrins (Gentilucci et al., 2010), and in the development of drug resistance (Schlesinger and Bendas, 2015).
Recent evidences hypothesize an apparent tumor-protective role of α4β1 integrin in a mouse model of colon adenocarcinoma: when α4β1 was depleted, an accelerated tumor growth was observed (Oh et al., 2018). Considering these preliminary results, authors suggest manipulation of α4β1 levels could be achieved using small molecule agonists.
Small Molecules Targeting α4β1 Integrin
Small molecules selectively binding to α4β1 integrin have been designed on the basis of the minimal recognition sequences with the extracellular matrix proteins. In particular, the tripeptide LDV (Leu-Asp-Val) that has been recognized as the binding sequence found in the alternatively spliced connecting segment (CS1) region of fibronectin (Komoriya et al., 1991), is homologous and quite isosteric to the fragment IDS (Ile-Asp-Ser), present in the binding site of VCAM-1 to α4β1.
Due to the lack of crystal structures of ligand-receptor complexes (Jones et al., 1995), suggestions on the required three-dimensional features that may ensure optimal affinity have been deduced only by combining homology models deduced by the template of β2 integrins (CD11A/CD11B) (You et al., 2002), QSAR studies on small library of LDV mimicking ligands (Singh et al., 2002; Hutt et al., 2011; Thangapandian et al., 2011; Amin et al., 2018), molecular dynamics and ligand-receptor docking studies (Silva et al., 2010). In general, effective ligands should possess a hydrogen bond acceptor, typically a carboxylate moiety, to coordinate the metal cation in the β unit and lipophilic groups that find accommodation into the pockets usually occupied by valine and leucine side chains (Figure 1B). Three complete and detailed overviews on α4β1 synthetic ligands have already been reported (Jackson, 2002; Tilley, 2002; Huryn et al., 2004), but a collection of the more recent results in the design and synthesis of these bioactive compounds is lacking. We report herein a selection of the most recent examples of bioactive small molecule ligands to α4β1 integrin. The structures of the cited compounds are reported in Table 1.
N-benziloxycarbamido Phenylurea (PUPA) Containing Ligands
Starting from the LDV recognition sequence, Adams et al. (Lin et al., 1999) synthesized a small library of oligopeptides which had the terminal amino group capped with benziloxycarbamido phenylurea (PUPA). Introduction of this moiety allowed to identify compound BIO1211 (N-PUPA-LDVP), which is 106 fold more potent than the corresponding peptide and possesses an enhanced resistance toward enzymatic hydrolysis. On this basis, N-PUPA containing linear peptidomimetics, respecting the fundamental requirements for affinity, showed micromolar inhibitory activity on fibronectin adhesion to human T lymphoblast like cells (Gérard et al., 2012a). Introduction of five membered rings as amide bond isosters and conformational restraints have been exploited by several groups. Recently, 5-aminomethyloxazolidine-2,4-dione (Amo) dipeptide scaffold, analog of the well-known Freidinger lactam, was successfully introduced as a central core into peptidomimetics, designed to maintain a 14-bond carboxylate-urea distance, displaying nanomolar IC50 in cell adhesion assays (De Marco et al., 2015). On the other hand, since proline has been often inserted into peptide sequences to induce specific conformations, (D)-configured β2-proline-containing ligand DS70 was synthesized and successfully tested in cell assays and in a guinea pig model of allergic conjunctivitis (Dattoli et al., 2018). A small library of compounds containing the rigid dehydro-β-proline ring also showed excellent affinity to α4β1 integrin (Tolomelli et al., 2015). For these compounds, a strong dependence on the stereochemistry of the heterocyclic central core was observed, thus suggesting a specific disposition of the lipophilic chain for the two enantiomers. Decorating the simpler four membered β-lactam scaffold also afforded effective ligands. In particular, agonists and antagonists to α4β1 were identified, by evaluating their ability to inhibit or activate cell adhesion. This behavior was ascribed to their ability to promote and stabilize active or inactive conformations of the receptor (Baiula et al., 2016). A previous study already suggested that small modifications in ligand structure could induce dramatic effect on their agonist/antagonist behavior. Compound TBC3486, a potent nanomolar antagonist selective for α4β1 integrin (Vanderslice et al., 2010), was converted into THI0019, a micromolar agonist, simply by introducing an oxymethylene bond and protecting the carboxylic terminal as methyl ester. Stabilization of an active conformation by the agonist, followed by its fast displacement was suggested to justify this result (Vanderslice et al., 2013).
Increased bioavailability was obtained by Daiichi Sankyo Ltd. researchers by linking fluoro-prolinol derivatives to halogen or alkyl substituted aromatic PUPA fragment (Muro et al., 2009). Benzoic or cyclohexanecarboxylic acids were introduced in this class of compounds as metal binding pharmacophores (Setoguchi et al., 2012). Modifications to the polar surface and the number of hydrogen bonds by changing PUPA with other lipophilic groups has also been explored (Setoguchi et al., 2013). Selected members of this library are currently in clinical development (Kapp et al., 2013). Another PUPA-containing ligand, HMR 1031, was enrolled by Aventis Pharmaceuticals to phase II clinical trials, but the suspect risk of teratogenicity decreased the interest on this compound (Crofts et al., 2004). Anyway, a similar molecule was lately reported to prevent development of arthritis in Lyme disease infection (Gläsner et al., 2005).
N-PUPA Derivatives for Imaging Application
In the last few years, due to the drawbacks of some promising integrin targeting compounds in vivo, beside the therapeutic applications, the use of selective ligands as imaging agents has been widely explored. For instance, the peptidomimetic LLP2A, displaying extraordinarily high affinity (IC50 = 2 pM) to the α4β1 integrin receptor (Peng et al., 2006), was conjugated with NIR tags through a PEG linker and applied to the detection of MOLT-4 tumor xenografts. Similar derivatives were linked to radioactive metal chelators for in vivo imaging (Denardo et al., 2009; Gai et al., 2018). More recently, 18F-labeled LLP2A-trifluoroborate bioconjugates were successfully evaluated in α4β1 integrin-overexpressing tumor models (Walker et al., 2016). Bioconjugation of BIO1211 derivatives with biotin though linear spacer arms allowed the exploitation of the extraordinary affinity with streptavidin/avidin coated supports to detect and capture leukemia cells overexpressing α4β1 (Gérard et al., 2012b).
Proline-Phenylalanine Dipeptide Deriving Ligands
A second important family of small molecule ligands possesses, as a common feature, the presence of an N-acylated para-substituted phenylalanine core. Among them, proline-phenylalanine derivatives showed excellent activity, lacking unfortunately of satisfactory bioavailability due to their peptidic nature. For this reason, much attention has been devoted to obtain a better pharmacokinetic profile and impart oral availability. Arylsolfonamide proline dipeptides (Hagmann et al., 2001), discovered from directed screening of a combinatorial library, showed picomolar α4β1 affinity, but unfortunately these compounds were very rapidly cleared from plasma. Thus, a novel series of potent prolyl dipeptide α4β1 antagonists containing fluorinated cyclic tertiary amines at the proline 4-position was developed. In general, the fluorinated compounds provided improved potency when compared with their des-fluoro analogs (Venkatraman et al., 2009). The highly potent BIO5192 (Ramirez et al., 2009) ligand shares some common features with the above reported molecules, as it is a chimera between arylsolfonamide proline dipeptides and PUPA-substituted compounds. This molecule displayed the ability to mobilize hematopoietic stem and progenitor cells (HSPC) but was not selected for clinical development. Conjugation of already reported N-phenylsulfonyl proline-based integrin antagonists (Pepinsky et al., 2002) to PEG-linker or fluorophore afforded novel compounds, exhibiting high nanomolar dual binding affinities to α9β1 and α4β1 integrins. Furthermore, these ligands are capable of binding haemopoietic progenitor cells and HSC within mice bone marrow in vivo (Cao et al., 2014).
By replacing the proline ring with a 3-alkyl-isoxazoline-5-carboxamide, ligands showing nanomolar activity and possessing stability in microsomes were obtained (Soni et al., 2013). Compound TR14035, first reported in 2002 by Tanabe, is a dichloro-substituted benzamides of biphenylalanine scaffold. This molecule displayed nanomolar IC50, but acted as a dual ligand, being active both on α4β1 and α4β7 integrins. Anyway, it represented the lead compound for the development of refined derivatives, such as AJM-300, developed by Ajinomoto, where the biphenyl chain was replaced by a phenyl-pirimidindione. This compound is currently in clinical phase III for ulcerative colitis (Sugiura et al., 2013). Firategrast (Kawaguchi et al., 2002), developed by Tanabe and GSK, belonging to the same class of compounds, reached phase II trials for MS and is currently studied as a facilitator in the “in utero” hematopoietic cell transplantation (IUHCT), a pioneering approach for critical fetal diseases treatment (Kim et al., 2016). By refining the structure of an already reported antagonist α4β1, the researchers of Elan Pharmaceuticals faced the limited bioavailability of their lead compound, by introducing N-arylated heterocycles to mimic the carboxamide (Semko et al., 2011). This last moiety was indeed considered partially responsible for the poor pharmacokinetic profile. As a result, they identified a novel compound displaying a greatly improved pharmacokinetic profile and robust efficacy in a sheep asthma model (Xu et al., 2013a). In further investigations, the same structure was properly modified achieving excellent bioavailability and, in some cases, dual affinity for α4β1 and α4β7 integrins (Xu et al., 2013b). Linking these molecules at each of the termini of a three-arm branched PEG provided potent in vivo α4 integrin inhibitors (Smith et al., 2013). In general, due to the high similarity of the α4β1 and α4β7 integrins receptors, several cases of dual ligands have been reported in the literature (Tilley et al., 2013). Finally, among the phenylalanine containing dipeptides, particular interest has been recently paid to GW559090, for its effects on corneal staining and ocular surface inflammation in murine model of DED (Krauss et al., 2015).
Tellurium Compound
AS101 [ammonium trichloro(dioxoethylene-o,o′)tellurate], a small telluriumIV compound, has been shown to inhibit α4β1 function by redox inactivation of adjacent thiols in the extracellular domain of α4β1 (Smith et al., 2002; Chigaev et al., 2004). Since this small molecule is not mimicking the recognition sequences in the extracellular matrix proteins, it has no structural similarity with all the other ligands. Through the regulation of integrin functions and immunomodulatory properties, AS101 significantly reduced clinical manifestations of IBD and other autoimmune and inflammatory diseases (Halpert et al., 2014).
Ligands Indirectly Regulating α4β1 Integrin Activity
The activity of α4β1 integrin may also be controlled by acting on other biomolecules. For instance, orally active ER464195-01, an antagonist to calreticulin (CRT), has been reported as an inhibitor of VCAM-1 mediated cell adhesion (Ohkuro et al., 2018), having an IC50 in the μM range. The effect is indirect since CRT is a calcium binding chaperone involved in integrin α subunit activation. On the other hand, targeting γ-parvin, a component of focal adhesions involved in the downstream of α4 integrin, allowed for the identification of compound JK273 (Lee et al., 2009), as an alternative modulator of α4 integrin mediated leukocyte trafficking.
Concluding Remarks
Involvement of α4β1 integrin in several diseases still waiting for an efficacious treatment and for a clear understanding of their pathogenesis, confirms the importance of this receptor as a therapeutic target. Already developed agents in late-phase clinical trial offer great expectations to patients, but the discovery of novel small molecule ligands, targeting not only binding but also selective conformation in active/inactive state, may offer great potential for novel treatments. Improvement of pharmacokinetic and pharmacodynamic properties of compounds that could allow managing of oral therapies in home environment is still an issue. Anyway, the use of α4β1 integrin ligands in bioconjugation with imaging agents is also a field of growing interest.
Finally, the recognized role of α4β1 integrin in the regulation of HSC homing, retention and engraftment suggests a paramount role of these receptor in the future development of post-transplantation treatments and prenatal therapy of fetus pathologies.
Author Contributions
MB, SS, and AT equally contributed to the preparation of the manuscript and LG approved the final version.
Funding
This study has been carried out with the fundamental contribution of MIUR (PRIN project 20157WW5EH), University of Bologna FARB (FFBO 125290), RFO2017 and RFO2018, Fondazione Cassa di Risparmio in Bologna (2018/0347).
Conflict of Interest Statement
The authors declare that the research was conducted in the absence of any commercial or financial relationships that could be construed as a potential conflict of interest.
Abbreviations
VLA-4, very late antigen-4; VCAM-1, vascular cell adhesion molecule-1; MAdCAM-1, mucosal vascular addressin cell adhesion molecule-1; JAM-B, junctional adhesion molecule-B; MS, multiple sclerosis; IBD, inflammatory bowel diseases; DED, dry eye disease; CNS, central nervous system; PML, progressive multifocal encephalopathy; UC, ulcerative colitis; CD, Crohn's disease; mAb, monoclonal antibody; ICAM-1, intercellular adhesion molecule-1; HSC, hematopoietic stem cell; LDV, Leu-Asp-Val; IDS, Ile-Asp-Ser; QSAR, quantitative structure-activity relationship; PUPA, benziloxycarbamido phenylurea; PEG, polyethylene glycol; HSPC, hematopoietic stem and progenitor cells; IUHCT, in utero hematopoietic cell transplantation; CRT, calreticulin.
References
Abram, C. L., and Lowell, C. A. (2009). The ins and outs of leukocyte integrin signaling. Annu. Rev. Immunol. 27, 339–362. doi: 10.1146/annurev.immunol.021908.132554.
Amin, S. A., Adhikari, N., Bhargava, S., Gayen, S., and Jha, T. (2018). An integrated QSAR modeling approach to explore the structure–property and selectivity relationships of N-benzoyl-l-biphenylalanines as integrin antagonists. Mol. Divers. 22, 129–158. doi: 10.1007/s11030-017-9789-9.
Bacon, A. S., McGill, J. I., Anderson, D. F., Baddeley, S., Lightman, S. L., and Holgate, S. T. (1998). Adhesion molecules and relationship to leukocyte levels in allergic eye disease. Invest. Ophthalmol. Vis. Sci. 39, 322–30.
Baiula, M., Galletti, P., Martelli, G., Soldati, R., Belvisi, L., Civera, M., et al. (2016). New β-lactam derivatives modulate cell adhesion and signaling mediated by RGD-binding and leukocyte integrins. J. Med. Chem. 59, 9721–9742. doi: 10.1021/acs.jmedchem.6b00576
Baiula, M., and Spampinato, S. (2014). Phase II drugs under investigation for allergic conjunctivitis. Expert. Opin. Investig. Drugs 23, 1671–1686. doi: 10.1517/13543784.2014.944640
Baiula, M., Spart,à, A., Bedini, A., Carbonari, G., Bucolo, C., Ward, K. W., et al. (2011). Eosinophil as a cellular target of the ocular anti-allergic action of mapracorat, a novel selective glucocorticoid receptor agonist. Mol. Vis. 17, 3208–3223.
Berlin, M., Lundahl, J., Sköld, C. M., Grunewald, J., and Eklund, A. (1998). The lymphocytic alveolitis in sarcoidosis is associated with increased amounts of soluble and cell-bound adhesion molecules in bronchoalveolar lavage fluid and serum. J. Intern. Med. 244, 333–40.
Cao, B., Hutt, O. E., Zhang, Z., Li, S., Heazlewood, S. Y., Williams, B., et al. (2014). Design, synthesis and binding properties of a fluorescent α9β1/α4β1 integrin antagonist and its application as an in vivo probe for bone marrow haemopoietic stem cells. Org. Biomol. Chem. 12, 965–978. doi: 10.1039/c3ob42332h
Cao, B., Zhang, Z., Grassinger, J., Williams, B., Heazlewood, C. K., Churches, Q. I., et al. (2016). Therapeutic targeting and rapid mobilization of endosteal HSC using a small molecule integrin antagonist. Nat. Commun. 7:11007. doi: 10.1038/ncomms11007
Chan, J. R., Hyduk, S. J., and Cybulsky, M. I. (2001). Chemoattractants induce a rapid and transient upregulation of monocyte alpha4 integrin affinity for vascular cell adhesion molecule 1 which mediates arrest: an early step in the process of emigration. J. Exp. Med. 193, 1149–58.
Chigaev, A., Zwartz, G. J., Buranda, T., Edwards, B. S., Prossnitz, E. R., and Sklar, L. A. (2004). Conformational regulation of alpha 4 beta 1-integrin affinity by reducing agents. "Inside-out" signaling is independent of and additive to reduction-regulated integrin activation. J. Biol. Chem. 279, 32435–32443. doi: 10.1074/jbc.M404387200.
Clerico, M., Artusi, C., Liberto, A., Rolla, S., Bardina, V., Barbero, P., et al. (2017). Natalizumab in multiple sclerosis: long-term management. Int. J. Mol. Sci. 18:940. doi: 10.3390/ijms18050940
Crofts, F., Pino, M., DeLise, B., Guittin, P., Barbellion, S., Brunel, P., et al. (2004). Different embryo-fetal toxicity effects for three VLA-4 antagonists. Birth Defects Res. B. Dev. Reprod. Toxicol. 71, 55–68. doi: 10.1002/bdrb.20001
Dargahi, N., Katsara, M., Tselios, T., Androutsou, M.-E., de Courten, M., Matsoukas, J., et al. (2017). Multiple sclerosis: immunopathology and treatment update. Brain Sci. 7:78. doi: 10.3390/brainsci7070078
Dattoli, S. D., Baiula, M., De Marco, R., Bedini, A., Anselmi, M., Gentilucci, L., et al. (2018). DS-70, a novel and potent α4 integrin antagonist, is an effective treatment for experimental allergic conjunctivitis in guinea pigs. Br. J. Pharmacol. 175, 3891–3910. doi: 10.1111/bph.14458
De Marco, R., Mazzotti, G., Dattoli, S. D., Baiula, M., Spampinato, S., Greco, A., et al. (2015). 5-aminomethyloxazolidine-2,4-dione hybrid α/β-dipeptide scaffolds as inductors of constrained conformations: applications to the synthesis of integrin antagonists. Biopolymers 104, 636–649. doi: 10.1002/bip.22704
Denardo, S. J., Liu, R., Albrecht, H., Natarajan, A., Sutcliffe, J. L., Anderson, C., et al. (2009). 111In-LLP2A-DOTA Polyethylene Glycol-Targeting {alpha}4{beta}1 Integrin: comparative pharmacokinetics for imaging and therapy of lymphoid malignancies. J. Nucl. Med. 50, 625–634. doi: 10.2967/jnumed.108.056903
Duan, H., Cheng, L., Sun, X., Wu, Y., Hu, L., Wang, J., et al. (2006). LFA-1 and VLA-4 involved in human high proliferative potential-endothelial progenitor cells homing to ischemic tissue. Thromb. Haemost. 96, 807–15.
Ecoiffier, T., Annan, J., El, R.ashid, S., Schaumberg, D., and Dana, R. (2008). Modulation of integrin α4β1 (VLA-4) in dry eye disease. Arch. Ophthalmol. 126:1695. doi: 10.1001/archopht.126.12.1695
Feagan, B. G., Rutgeerts, P., Sands, B. E., Hanauer, S., Colombel, J.-F., Sandborn, W. J., et al. (2013). Vedolizumab as induction and maintenance therapy for ulcerative colitis. N. Engl. J. Med. 369, 699–710. doi: 10.1056/NEJMoa1215734
Gai, Y., Sun, L., Lan, X., Zeng, D., Xiang, G., and Ma, X. (2018). Synthesis and Evaluation of new bifunctional chelators with phosphonic acid arms for gallium-68 based PET imaging in melanoma. Bioconjug. Chem. 29, 3483–3494. doi: 10.1021/acs.bioconjchem.8b00642
Gentilucci, L., Cardillo, G., Spampinato, S., Tolomelli, A., Squassabia, F., De Marco, R., et al. (2010). Antiangiogenic effect of dual/selective α5 β1/αvβ3 integrin antagonists designed on partially modified retro-inverso cyclotetrapeptide mimetics. J. Med. Chem. 53, 106–118. doi: 10.1021/jm9013532
Gérard, E., Meulle, A., Feron, O., and Marchand-Brynaert, J. (2012a). Diaryl ureaLDV peptidomimetics as α 4 β 1 integrin antagonists: synthesis, adhesion inhibition and toxicity evaluation on CCRF-CEM cell line. Med. Chem. Commun. 3, 199–212. doi: 10.1039/C1MD00229E
Gérard, E., Meulle, A., Feron, O., and Marchand-Brynaert, J. (2012b). LDV peptidomimetics equipped with biotinylated spacer-arms: synthesis and biological evaluation on CCRF-CEM cell line. Bioorg. Med. Chem. Lett. 22, 586–590. doi: 10.1016/j.bmcl.2011.10.078
Ghobadi, A., Rettig, M. P., Cooper, M. L., Holt, M. S., Ritchey, J. K., Eissenberg, L., et al. (2014). Bortezomib is a rapid mobilizer of hematopoietic stem cells in mice via modulation of the VCAM-1/VLA-4 axis. Blood 124, 2752–2754. doi: 10.1182/blood-2014-08-595967
Gläsner, J., Blum, H., Wehner, V., Stilz, H. U., Humphries, J. D., Curley, G. P., et al. (2005). A small molecule alpha 4 beta 1 antagonist prevents development of murine Lyme arthritis without affecting protective immunity. J. Immunol. 175, 4724–4734. doi: 10.4049/jimmunol.175.7.4724
Grassinger, J., Haylock, D. N., Storan, M. J., Haines, G. O., Williams, B., Whitty, G. A., et al. (2009). Thrombin-cleaved osteopontin regulates hemopoietic stem and progenitor cell functions through interactions with alpha9beta1 and alpha4beta1 integrins. Blood 114, 49–59. doi: 10.1182/blood-2009-01-197988
Hagmann, W., Durette, P., Lanza, T., Kevin, N., de, S. L., Kopka, I., et al. (2001). The discovery of sulfonylated dipeptides as potent VLA-4 antagonists. Bioorg. Med. Chem. Lett. 11, 2709–2713. doi: 10.1016/S0960-894X(01)00544-3
Halpert, G., Eitan, T., Voronov, E., Apte, R. N., Rath-Wolfson, L., Albeck, M., et al. (2014). Multifunctional activity of a small tellurium redox immunomodulator compound, AS101, on dextran sodium sulfate-induced murine colitis. J. Biol. Chem. 289, 17215–17227. doi: 10.1074/jbc.M113.536664
Hemler, M. E., Huang, C., and Schwarz, L. (1987). The VLA protein family. Characterization of five distinct cell surface heterodimers each with a common 130,000 molecular weight beta subunit. J. Biol. Chem. 262, 3300–9.
Herter, J., and Zarbock, A. (2019). Recruitment integrin regulation during leukocyte. J. Immunol. 190, 4451–4457. doi: 10.4049/jimmunol.1203179
Humphries, J. D., Byron, A., and Humphries, M. J. (2006). Integrin ligands at a glance. J. Cell Sci. 119, 3901–3903. doi: 10.1242/jcs.03098
Huryn, D., Konradi, A., Ashwell, S., Freedman, S., Lombardo, L., Pleiss, M., et al. (2004). The identification and optimization of orally efficacious, small molecule VLA-4 antagonists. Curr. Top. Med. Chem. 4, 1473–1484. doi: 10.2174/1568026043387467
Hutt, O. E., Saubern, S., and Winkler, D. A. (2011). Modeling the molecular basis for α4β1 integrin antagonism. Bioorg. Med. Chem. 19, 5903–5911. doi: 10.1016/j.bmc.2011.08.011
Imhof, B. A., and Aurrand-Lions, M. (2004). Adhesion mechanisms regulating the migration of monocytes. Nat. Rev. Immunol. 4, 432–444. doi: 10.1038/nri1375
Jones, E. Y., Harlos, K., Bottomley, M. J., Robinson, R. C., Driscoll, P. C., Edwards, R. M., et al. (1995). Crystal structure of an integrin-binding fragment of vascular cell adhesion molecule-1 at 1.8 A resolution. Nature 373, 539–544. doi: 10.1038/373539a0
Kanwar, J. R., Harrison, J. E., Wang, D., Leung, E., Mueller, W., Wagner, N., et al. (2000). Beta7 integrins contribute to demyelinating disease of the central nervous system. J. Neuroimmunol. 103, 146–52.
Kapp, T. G., Rechenmacher, F., Sobahi, T. R., and Kessler, H. (2013). Integrin modulators: a patent review. Expert Opin. Ther. Pat. 23, 1273–1295. doi: 10.1517/13543776.2013.818133
Kawaguchi, T., Nomura, S., Tsukimoto, M., Kume, T., and Sircar, H. (2002). Inhibitors of Alpha4 Mediated Cell Adhesion. WO 02/18320 A3.
Kim, A. G., Vrecenak, J. D., Boelig, M. M., Eissenberg, L., Rettig, M. P., Riley, J. S., et al. (2016). Enhanced in utero allogeneic engraftment in mice after mobilizing fetal HSCs by α4β1/7 inhibition. Blood 128, 2457–2461. doi: 10.1182/blood-2016-06-723981
Komoriya, A., Green, L. J., Mervic, M., Yamada, S. S., Yamada, K. M., and Humphries, M. J. (1991). The minimal essential sequence for a major cell type-specific adhesion site (CS1) within the alternatively spliced type III connecting segment domain of fibronectin is leucine-aspartic acid-valine. J. Biol. Chem. 266, 15075–9.
Krauss, A. H., Corrales, R. M., Pelegrino, F. S. A., Tukler-Henriksson, J., Pflugfelder, S. C., and de Paiva, C. S. (2015). Improvement of outcome measures of dry eye by a novel integrin antagonist in the murine desiccating stress model. Invest. Ophthalmol. Vis. Sci. 56, 5888–5895. doi: 10.1167/iovs.15-17249
Lee, J., Hong, J., Nam, T.-G., Peters, E. C., Orth, A. P., Geierstanger, B. H., et al. (2009). A small molecule inhibitor of alpha4 integrin-dependent cell migration. Bioorg. Med. Chem. 17, 977–980. doi: 10.1016/j.bmc.2008.02.067
Ley, K., Rivera-Nieves, J., Sandborn, W. J., and Shattil, S. (2016). Integrin-based therapeutics: biological basis, clinical use and new drugs. Nat. Rev. Drug Discov. 15, 173–183. doi: 10.1038/nrd.2015.10
Li, H., Huang, S.-Y., Shi, H., Gu, Z.-C., Zhang, S.-G., and Wei, J.-F. (2018). α4β7 integrin inhibitors: a patent review. Expert Opin. Ther. Pat. 28, 903–917. doi: 10.1080/13543776.2018.1549227
Lin, K., c, Ateeq, H. S., Hsiung, S. H., Chong, L. T., Zimmerman, C. N., Castro, A., et al. (1999). Selective, tight-binding inhibitors of integrin alpha4beta1 that inhibit allergic airway responses. J. Med. Chem. 42, 920–934. doi: 10.1021/jm980673g
Miljanović, B., Dana, R., Sullivan, D. A., and Schaumberg, D. A. (2007). Impact of Dry Eye Syndrome on Vision-Related Quality of Life. Am. J. Ophthalmol. 143, 409–415.
Mims, J. W. (2015). Asthma: definitions and pathophysiology. Int. Forum Allergy Rhinol. 5, S2–S6. doi: 10.1002/alr.21609
Mitroulis, I., Alexaki, V. I., Kourtzelis, I., Ziogas, A., Hajishengallis, G., and Chavakis, T. (2015). Leukocyte integrins: role in leukocyte recruitment and as therapeutic targets in inflammatory disease. Pharmacol. Ther. 147, 123–135. doi: 10.1016/j.pharmthera.2014.11.008
Muro, F., Iimura, S., Sugimoto, Y., Yoneda, Y., Chiba, J., Watanabe, T., et al. (2009). Discovery of trans-4-[1-[[2,5-Dichloro-4-(1-methyl-3-indolylcarboxamido)phenyl]acetyl]-(4S)-methoxy-(2S)-pyrrolidinylmethoxy]cyclohexanecarboxylic acid: an orally active, selective very late antigen-4 antagonist. J. Med. Chem. 52, 7974–7992. doi: 10.1021/jm901154c
Nelson, S. M., Nguyen, T. M., McDonald, J. W., and MacDonald, J. K. (2018). Natalizumab for induction of remission in Crohn's disease. Cochr. Database Syst. Rev. 8:CD006097. doi: 10.1002/14651858.CD006097.pub3
Noborio-Hatano, K., Kikuchi, J., Takatoku, M., Shimizu, R., Wada, T., Ueda, M., et al. (2009). Bortezomib overcomes cell-adhesion-mediated drug resistance through downregulation of VLA-4 expression in multiple myeloma. Oncogene 28, 231–242. doi: 10.1038/onc.2008.385
Oh, J., Magnuson, A., Benoist, C., Pittet, M. J., and Weissleder, R. (2018). Age-related tumor growth in mice is related to integrin α 4 in CD8+ T cells. JCI Insight 3:e122961. doi: 10.1172/jci.insight.122961
Ohashi, Y., Motojima, S., Fukuda, T., and Makino, S. (1992). Airway hyperresponsiveness, increased intracellular spaces of bronchial epithelium, and increased infiltration of eosinophils and lymphocytes in bronchial mucosa in asthma. Am. Rev. Respir. Dis. 145, 1469–1476. doi: 10.1164/ajrccm/145.6.1469
Ohkuro, M., Kim, J.-D., Kuboi, Y., Hayashi, Y., Mizukami, H., Kobayashi-Kuramochi, H., et al. (2018). Calreticulin and integrin alpha dissociation induces anti-inflammatory programming in animal models of inflammatory bowel disease. Nat. Commun. 9:1982. doi: 10.1038/s41467-018-04420-4
Peng, L., Liu, R., Marik, J., Wang, X., Takada, Y., and Lam, K. S. (2006). Combinatorial chemistry identifies high-affinity peptidomimetics against alpha4beta1 integrin for in vivo tumor imaging. Nat. Chem. Biol. 2, 381–389. doi: 10.1038/nchembio798
Pepinsky, R. B., Mumford, R. A., Chen, L. L., Leone, D., Amo, S. E., Riper, G., et al. (2002). Comparative assessment of the ligand and metal ion binding properties of integrins alpha9beta1 and alpha4beta1. Biochemistry 41, 7125–7141. doi: 10.1021/bi020024d
Qasem, A. R., Bucolo, C., Baiula, M., Spart,à, A., Govoni, P., Bedini, A., et al. (2008). Contribution of α4β1 integrin to the antiallergic effect of levocabastine. Biochem. Pharmacol. 76, 751–762. doi: 10.1016/j.bcp.2008.07.007
Ramirez, P., Rettig, M. P., Uy, G. L., Deych, E., Holt, M. S., Ritchey, J. K., et al. (2009). BIO5192, a small molecule inhibitor of VLA-4, mobilizes hematopoietic stem and progenitor cells. Blood 114, 1340–1343. doi: 10.1182/blood-2008-10-184721
Sandborn, W. J., Feagan, B. G., Rutgeerts, P., Hanauer, S., Colombel, J.-F., Sands, B. E., et al. (2013). Vedolizumab as induction and maintenance therapy for Crohn's disease. N. Engl. J. Med. 369, 711–721. doi: 10.1056/NEJMoa1215739
Schlesinger, M., and Bendas, G. (2015). Contribution of very late antigen-4 (VLA-4) integrin to cancer progression and metastasis. Cancer Metastasis Rev. 34, 575–591. doi: 10.1007/s10555-014-9545-x
Semko, C. M., Chen, L., Dressen, D. B., Dreyer, M. L., Dunn, W., Farouz, F. S., et al. (2011). Discovery of a potent, orally bioavailable pyrimidine VLA-4 antagonist effective in a sheep asthma model. Bioorg. Med. Chem. Lett. 21, 1741–1743. doi: 10.1016/j.bmcl.2011.01.075
Setoguchi, M., Iimura, S., Sugimoto, Y., Yoneda, Y., Chiba, J., Watanabe, T., et al. (2012). Identification of trans-4-[1-[[7-fluoro-2-(1-methyl-3-indolyl)-6-benzoxazolyl]acetyl]-(4S)-fluoro-(2S)-pyrrolidinylmethoxy]cyclohexanecarboxylic acid as a potent, orally active VLA-4 antagonist. Bioorg. Med. Chem. 20, 1201–1212. doi: 10.1016/j.bmc.2011.12.045
Setoguchi, M., Iimura, S., Sugimoto, Y., Yoneda, Y., Chiba, J., Watanabe, T., et al. (2013). A novel, potent, and orally active VLA-4 antagonist with good aqueous solubility: trans-4-[1-[[2-(5-Fluoro-2-methylphenylamino)-7-fluoro-6-benzoxazolyl]acetyl]-(5S)-[methoxy(methyl)amino]methyl-(2S)-pyrrolidinylmethoxy]cyclohexanecarboxylic acid. Bioorg. Med. Chem. 21, 42–61. doi: 10.1016/j.bmc.2012.11.003
Shirani, A., and Stüve, O. (2017). Neuroimmunology in point for the impact of translational natalizumab for multiple sclerosis: a case. J. Immunol. 198, 1381–1386. doi: 10.4049/jimmunol.1601358
Silva, J. H. M., da Dardenne, L. E., Savino, W., and Caffarena, E. R. (2010). Analysis of α4 β1integrin specific antagonists binding modes: structural insights by molecular docking, molecular dynamics and linear interaction energy method for free energy calculations. J. Braz. Chem. Soc. 21, 546–555. doi: 10.1590/S0103-50532010000300022
Singh, J., van Vlijmen, H., Liao, Y., Lee, W.-C., Cornebise, M., Harris, M., et al. (2002). Identification of potent and novel α4 β1 antagonists using in silico screening. J. Med. Chem. 45, 2988–2993. doi: 10.1021/jm020054e
Smith, J. L., Rossiter, K. I., Semko, C. M., Xu, Y.-Z., Quincy, D. A., Jagodzinski, J., et al. (2013). PEG conjugates of potent α4 integrin inhibitors, maintaining sustained levels and bioactivity in vivo, following subcutaneous administration. Bioorg. Med. Chem. Lett. 23, 4117–4119. doi: 10.1016/j.bmcl.2013.05.048
Smith, J. W., Yan, B., Landry, F., and Lombardo, C. R. (2002). Redox control of integrin adhesion receptors. Methods Enzymol. 353, 156–63.
Soni, A., Rehman, A., Naik, K., Dastidar, S., Alam, M. S., Ray, A., et al. (2013). Synthesis and evaluation of 4,5-dihydro-5-methylisoxazolin-5-carboxamide derivatives as VLA-4 antagonists. Bioorg. Med. Chem. Lett. 23, 1482–1485. doi: 10.1016/j.bmcl.2012.12.043
Sugiura, T., Kageyama, S., Andou, A., Miyazawa, T., Ejima, C., Nakayama, A., et al. (2013). Oral treatment with a novel small molecule alpha 4 integrin antagonist, AJM300, prevents the development of experimental colitis in mice. J. Crohns. Colitis 7, e533–e542. doi: 10.1016/j.crohns.2013.03.014
Teoh, C. M., Tan, S. S. L., and Tran, T. (2015). Integrins as Therapeutic Targets for Respiratory Diseases. Available online at: https://www.ncbi.nlm.nih.gov/pmc/articles/PMC5427774/pdf/CMM-15-714.pdf (accessed April 9, 2019).
Thangapandian, S., John, S., Sakkiah, S., and Lee, K. W. (2011). Discovery of potential integrin VLA-4 antagonists using pharmacophore modeling, virtual screening and molecular docking studies. Chem. Biol. Drug Des. 78, 289–300. doi: 10.1111/j.1747-0285.2011.01127.x
Tilley, J. W. (2002). VLA-4 antagonists. Expert Opin. Ther. Pat. 12, 991–1008. doi: 10.1517/13543776.12.7.991
Tilley, J. W., Sidduri, A., Lou, J., Kaplan, G., Tare, N., Cavallo, G., et al. (2013). Identification of N-acyl 4-(3-pyridonyl)phenylalanine derivatives and their orally active prodrug esters as dual acting α4β1 and α4β7 receptor antagonists. Bioorg. Med. Chem. Lett. 23, 1036–1040. doi: 10.1016/j.bmcl.2012.12.019
Tolomelli, A., Baiula, M., Viola, A., Ferrazzano, L., Gentilucci, L., Dattoli, S. D., et al. (2015). Dehydro-β-proline containing α 4 β 1 integrin antagonists: stereochemical recognition in ligand–receptor interplay. ACS Med. Chem. Lett. 6, 701–706. doi: 10.1021/acsmedchemlett.5b00125
Tolomelli, A., Galletti, P., Baiula, M., and Giacomini, D. (2017). Can integrin agonists have cards to play against cancer? A Literature Survey of Small Molecules Integrin Activators. Cancers. 9:78. doi: 10.3390/cancers9070078
Vanderslice, P., Biediger, R. J., Woodside, D. G., Brown, W. S., Khounlo, S., Warier, N. D., et al. (2013). Small molecule agonist of very late antigen-4 (VLA-4) integrin induces progenitor cell adhesion. J. Biol. Chem. 288, 19414–19428. doi: 10.1074/jbc.M113.479634
Vanderslice, P., Woodside, D. G., Caivano, A. R., Decker, E. R., Munsch, C. L., Sherwood, S. J., et al. (2010). Potent in vivo suppression of inflammation by selectively targeting the high affinity conformation of integrin α4β1. Biochem. Biophys. Res. Commun. 400, 619–624. doi: 10.1016/j.bbrc.2010.08.114
Vanharanta, S., and Massagu,é, J. (2013). Origins of metastatic traits. Cancer Cell. 24, 410–421. doi: 10.1016/j.ccr.2013.09.007
Venkatraman, S., Lebsack, A. D., Alves, K., Gardner, M. F., James, J., Lingham, R. B., et al. (2009). Discovery of N-{N-[(3-cyanobenzene) sulfonyl]-4(R)-(3,3-difluoropiperidin-1-yl)-(l)-prolyl}-4-[(3',5'-dichloro-isonicotinoyl) amino]-(l)-phenylalanine (MK-0617), a highly potent and orally active VLA-4 antagonist. Bioorg. Med. Chem. Lett. 19, 5803–5806. doi: 10.1016/j.bmcl.2009.07.111
Walker, D., Li, Y., Roxin, Á., Schaffer, P., Adam, M. J., and Perrin, D. M. (2016). Facile synthesis and 18F-radiolabeling of α4β1-specific LLP2A-aryltrifluoroborate peptidomimetic conjugates. Bioorg. Med. Chem. Lett. 26, 5126–5131. doi: 10.1016/j.bmcl.2016.08.011
Xu, Y., Smith, J. L., Semko, C. M., Rossiter, K. I., Fukuda, J. Y., Dappen, M. S., et al. (2013b). Orally available and efficacious α4β1/α4β7 integrin inhibitors. Bioorg. Med. Chem. Lett. 23, 4370–4373. doi: 10.1016/j.bmcl.2013.05.076
Xu, Y.-Z., Konradi, A. W., Bard, F., Dappen, M., Dofiles, L., Dreyer, M., et al. (2013a). Arylsulfonamide pyrimidines as VLA-4 antagonists. Bioorg. Med. Chem. Lett. 23, 3070–3074. doi: 10.1016/j.bmcl.2013.03.010
You, T. J., Maxwell, D. S., Kogan, T. P., Chen, Q., Li, J., Kassir, J., et al. (2002). A 3D structure model of integrin alpha 4 beta 1 complex: I. Construction of a homology model of beta 1 and ligand binding analysis. Biophys. J. 82, 447–57. doi: 10.1016/S0006-3495(02)75409-X
Zundler, S., Becker, E., Weidinger, C., and Siegmund, B. (2017a). Anti-adhesion therapies in inflammatory bowel disease—molecular and clinical aspects. Front. Immunol. 8:891. doi: 10.3389/fimmu.2017.00891
Keywords: α4β1 integrin, agonist, antagonist, small molecules, inflammatory disorders
Citation: Baiula M, Spampinato S, Gentilucci L and Tolomelli A (2019) Novel Ligands Targeting α4β1 Integrin: Therapeutic Applications and Perspectives. Front. Chem. 7:489. doi: 10.3389/fchem.2019.00489
Received: 14 May 2019; Accepted: 25 June 2019;
Published: 09 July 2019.
Edited by:
Simona Rapposelli, University of Pisa, ItalyReviewed by:
Benjamin Sredni, Bar-Ilan University, IsraelSalvatore Di Maro, Second University of Naples, Italy
Copyright © 2019 Baiula, Spampinato, Gentilucci and Tolomelli. This is an open-access article distributed under the terms of the Creative Commons Attribution License (CC BY). The use, distribution or reproduction in other forums is permitted, provided the original author(s) and the copyright owner(s) are credited and that the original publication in this journal is cited, in accordance with accepted academic practice. No use, distribution or reproduction is permitted which does not comply with these terms.
*Correspondence: Santi Spampinato, santi.spampinato@unibo.it; Luca Gentilucci, luca.gentilucci@unibo.it