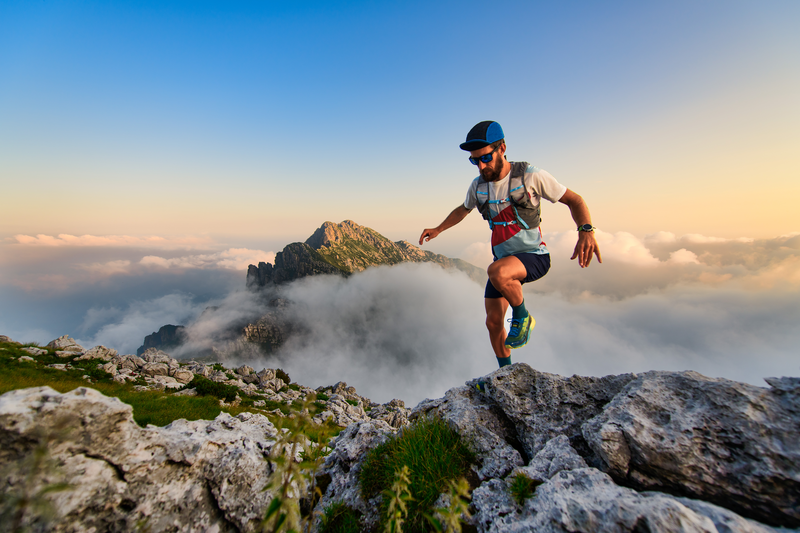
94% of researchers rate our articles as excellent or good
Learn more about the work of our research integrity team to safeguard the quality of each article we publish.
Find out more
ORIGINAL RESEARCH article
Front. Chem. , 14 June 2019
Sec. Organic Chemistry
Volume 7 - 2019 | https://doi.org/10.3389/fchem.2019.00433
This article is part of the Research Topic Isocyanide-based Multicomponent Reactions View all 10 articles
A palladium-catalyzed three-component reaction between 5-(2-chloroquinolin-3-yl) oxazoles, isocyanides, and water to yield 3-(oxazol-5-yl)quinoline-2-carboxamides is described. Interestingly, sulfonylation occurred when the same reaction was performed with toluenesulfonylmethyl isocyanide (TosMIC) as an isocyanide source. The reaction with 5-(2-chloroquinolin-3-yl)oxazoles and TosMIC in the presence of Cs2CO3 in DMSO afforded 5-(2-Tosylquinolin-3-yl)oxazoles. In basic media, TosMIC probably decomposed to generate Ts− species, which were replaced with Cl−. Tandem oxazole formation with subsequent sulfonylation of 2-chloroquinoline-3-carbaldehydes to form directly 5-(2-tosylquinolin-3-yl)oxazoles was also investigated.
Quinolines are heterocyclic compounds exhibiting diverse and well-documented bioactivity and physical properties as well as existing as scaffolds in complex structures of natural products (Michael, 2002; Hranjec et al., 2017). Accordingly, quinoline synthesis, and functionalization has attracted much attention from synthetic organic chemists (Marco-Contelles et al., 2009; Shiri et al., 2011; Prajapati et al., 2014; Sharma et al., 2018; Nainwal et al., 2019).
However, isocyanides play an important role in synthesizing N-containing heterocycles and are particularly widely applied in Ugi and Passirini reactions (Domling and Ugi, 2000; Domling, 2006). Amides are especially valuable as precursors in synthesizing of bioactive and natural structures, in medicinal chemistry as well as protein synthesis (Bode, 2006; Rönn et al., 2008).
Many natural products such as urukthapelstatin A have been isolated from marine sources, these contains, several aminocarbonyl and oxazole functional groups with cytotoxic activity against human lung cancer (Yu et al., 2009). Similarly, venturamides A and B showed in vitro antimalarial activity (Linington et al., 2007; Davyt and Serra, 2010). Additionally, aerucyclamid C, a hexameric cyclopeptide, extremely active against T. brucei rhodesiense, which causes sleeping sickness, and exhibits lower activity against P. falciparum, the deadliest species of Plasmodium, which causes malaria (Davyt and Serra, 2010). Microcyclamide A is a cyclic hexapeptide with three five-membered heterocycles. It is isolated from cyanobacterium M. aeruginosa, and it has exhibited cytotoxic effects against P388 murine leukemia (Ishida et al., 2000; Davyt and Serra, 2010; Raveh et al., 2010) (Figure 1).
Classical procedures for amide bond formation include reactions between carboxylic acids and amines (Linington et al., 2007; Davyt and Serra, 2010). Another route involves reactions of acyl halides, acyl azides, acyl imidazoles, anhydrides, or esters (activated carboxylic acid species) with amines (Ulijn et al., 2002; Montalbetti and Falque, 2005). As well as classical routes for amides synthesis which have own merits and demerits, direct aminocarbonylation from aryl halides in metal-catalyzed reactions has attracted attention from chemists due to its significant advantages benzamide preparation (Åkerbladh et al., 2017). In this regard, many synthetic methods have been introduced, including copper-catalyzed reactions of aryl halides and isocyanides in DMSO (Yavari et al., 2014). A palladium-catalyzed reaction was developed for amidation of aryl halides (Jiang et al., 2011), as well as the synthesis of 4-aminophthalazin-1(2H)-ones in a palladium-catalyzed reaction with isocyanide insertion in a multi-component reaction, which is difficult to achieve via a classical route (Vlaar et al., 2011). Palladium-catalyzed isocyanide insertion was applied to a carboxamidation/hydroamidation reaction to synthesize isoindolin-1-one derivatives (Pathare et al., 2016). Another example is the synthesis of isoquinolin-1(2H)-one derivatives via a palladium-catalyzed cascade reaction from isocyanide and amides (Wang et al., 2002; Tyagi et al., 2012; Chaudhary et al., 2013). Very recently, Guan et al reported an efficient method for the synthesis of multisubstituted 1H-imidazo-[4,5-c]quinoline derivatives via sequential van Leusen/Staudinger/aza-Wittig/carbodiimide-mediated cyclization (Guan et al., 2018).
The solvents and chemicals purchased from Merck and Aldrich chemical companies. Unless otherwise mentioned they used without further purification. Melting points are taken on an Electrothermal 9100 apparatus and are uncorrected. IR spectras recorded on a Shimadzu Infra-Red Spectroscopy IR-435. Nuclear magnetic resonance (NMR) spectra recorded on a Bruker AVANCE Spectrometer (400 MHz for 1H, 100 MHz for 13C) in DMSO-d6 and CDCl3 as solvent, TMS used as internal standard. The elemental analysis carried out with a Leco CHNS model 932. Mass spectra recorded on Agilent Technology (HP) 5973 Network Mass Selective Detector operating at an ionization potential of 70 eV.
A mixture of 2-chloro-quinoline-3-carbaldehyde 2a (191 mg, 1.0 mmol), p-toluenesulfonylmethyl isocyanide 1 (234 mg, 1.2 mmol) and K2CO3 (341 mg, 2.5 equiv.) was added to EtOH (5.0 ml) and stirred for 3.5 h, at room temperature. After completion of the reaction, monitored by TLC, the mixture poured into cool water and stirred for 30 min. The product 3a filtered, washed with water two times and dried on the air.
A mixture of 5-(2-chloroquinolin-3-yl)oxazole 3a (230 mg, 1.0 mmol), of Pd(OAc)2 (11 mg, 5 mol%) and Cs2CO3 (325 mg, 1.0 mmol) stirred in DMSO:H2O, 9:1 (5 mL) at 80°C for 15 min. Cyclohexyl isocyanide 4a (120 mg, 1.1 mmol) was added and the reaction stirred for 4 h. After completion of the reaction (the progress of the reaction was monitored by TLC) organic layer was extracted by DCM, washed with brine, dried over Na2SO4 and its solvent evaporated on a rotary evaporator. The residue was washed with 2-propanol and recrystallized in methanol to give 5a. It is noteworthy that 5a and 5h purified by washing with 2-propanol but other derivatives were purified by a column chromatography (n-hexan: ethyl acetate 3:1) and recrystallized in EtOH.
A mixture of 2-chloro-quinoline-3-carbaldehyde 2a (191 mg, 1.0 mmol), p-toluenesulfonylmethyl isocyanide 1 (468 mg, 2.4 mmol) and Cs2CO3 (810 mg, equiv.) was added to DMSO (5.0 ml) and stirred for 5 h at 80°C. After completion of the reaction and monitored by TLC, the mixture poured into cool water and stirred for 30 min, then extracted with DCM. The product 6a purified by a column chromatography (n-hexan: ethyl acetate 4:1).
Copies of NMR spectra are provided as Supplementary Materials. White powder, mp.: 123–128°C. 1H-NMR (400 MHz, CDCl3): δ = 1.25–1.52 (m, 5H), 1.68–171 (m, 1H), 1.80–1.85 (m, 2H), 2.10–213 (m, 2H), 3.99–4.02 (m, 1H), 7.60 (s, 1H), 7.67 (t, J = 8.0 Hz, 2H), 7.82 (t, J = 7.8 Hz, 1H), 7.90 (d, J = 8.4 Hz, 1H), 8.03 (s, 1H), 8.14 (d, J = 8.4 Hz, 1H), 8.44 (s, 1H) ppm. 13C-NMR (100 MHz, CDCl3): δ = 25.0, 25.6, 33.0, 48.6, 120.4, 125.9, 127.9, 128.0, 128.6, 129.5 131.0, 137.9, 145.9, 148.5, 148.8, 151.0, 164.3 ppm. Mass: m/z 321 (M+) (calcd. For C19H19N3O2: 321.37). FT-IR (KBr): νmax: 1604, 3444 cm−1. Anal. calcd. for C19H19N3O2: C, 71.01; H, 5.96; N, 13.08. Found: C,71.10; H,5.81; N,13.17.
White powder, mp: 181–185°C. 1H-NMR (400 MHz, DMSO-d6): δ = 1.15–1.74 (m, 10H), 2.75 (s, 3H), 3.83 (m, 1H), 7.52 (s, 1H), 7.63 (d, J = 8.0 Hz, 1H), 7.73 (d, J = 6.8 Hz, 1H), 7.97 (d, J = 8.0 Hz, 1H), 8.60 (s, 1H), 8.74 (s, 1H) ppm. 13C-NMR (100 MHz, DMSO-d6): δ = 17.8, 25.0, 25.6, 25.8, 32.5, 33.8, 48.5, 118.6, 121.8, 125.0, 126.7, 127.6, 128.3, 131.4, 135.2, 136.9, 145.0, 147.8, 153.0, 167.0 ppm. Mass: m/z 335 (M+) (calcd. for C20H21N3O2: 335.40). FT-IR (KBr): νmax: 1646, 2853, 2922, 3310, 3444 cm−1. Anal. calcd. for C20H21N3O2: C, 71.62; H, 6.31; N, 12.53%. Found: C, 71.55; H, 6.47; N, 12.42%.
White powder, mp: 124–127°C. 1H-NMR (400 MHz, CDCl3): δ = 1.01 (t, J = 7.2 Hz, 3H), 1.45–1.54 (m, 2H), 1.67–1.74 (m, 2H), 2.61 (s, 3H), 3.53 (dd, J = 13.4 Hz, J = 6.8 Hz, 2H), 7.60 (s, 1H), 7.65 (s, 1H), 7.68 (s, 1H), 7.77 (s, 1H), 8.03 (t, J = 5.2 Hz, 2H), 8.37 (s, 1H) ppm. 13C-NMR (100 MHz, CDCl3): δ = 13.8, 20.2, 21.8, 31.7, 39.5, 120.4, 125.7, 125.8, 126.5, 126.6, 128.1, 129.0, 133.4, 137.2, 138.9, 142.9, 150.9, 165.2 ppm. Mass: m/z 309 (M+) (calcd for C18H19N3O2: 309.36). FT-IR (KBr): νmax: 1542, 1652, 2858, 2928, 2956, 2922, 3114, 3299 cm−1. Anal. calcd. for: C18H19N3O2, C, 69.88; H, 6.19; N, 13.58%. Found C, 67.03; H, 6.24; N, 13.65%.
White powder, mp: 157–162°C. 1H-NMR (400 MHz, DMSO-d6): δ = 1.04–1.27 (m, 5H), 1.57–1.74 (m, 5H), 3.93(s, 3H), 3.93 (m, 1H), 7.52 (dd, J = 9.2, J = 2.8 Hz, 1H), 7.61 (d, J = 2.8 Hz, 1H), 7.92 (d, J = 9.2 Hz, 1H), 7.96 (s, 1H), 8.71 (s, 1H), 8.77 (s,1H) ppm. 13C-NMR (100 MHz, DMSO-d6): δ = 24.9, 25.8, 30.8, 31.2, 33.8, 47.9, 56.2, 106.7, 115.8, 121.3, 124.6, 127.5, 128.3, 129.6, 135.9, 142.4, 143.1, 146.5, 153.4, 158.7 ppm. Mass: m/z 351 (M+) (calcd. for C20H21N3O3:351.40). FT-IR (KBr): νmax: 1666, 2927, 2966, 3286, 3423 cm−1. Anal. calcd. for C20H21N3O3: C, 68.36; H, 6.02; N, 11.96%. Found: C, 68.47; H, 6.14; N, 12.11%.
White powder, mp: 224–267°C. 1H-NMR (400 MHz, CDCl3): δ = 1.28–1.58 (m, 6H), 1.85–1.88 (m, 2H), 2.16–2.19 (m, 2H), 4.07- 4.10 (m, 1H), 7.30 (s, 1H), 7.74 (d, J = 2.4 Hz, 1H), 7.79-7.85 (m, 3H), 7.93 (d, J = 8.8 Hz, 1H), 7.97 (d, J = 7.6 Hz, 1H), 8.07 (s, 1H), 8.49 (s, 1H), 9.19 (d, J = 7.6 Hz, 1H) ppm. 13C-NMR (100 MHz, CDCl3): δ = 24.9, 25.7, 29.7, 33.2, 48.5, 77.2, 121.3, 124.3, 124.7, 126.6, 126.9, 127.7, 128.2, 129.1, 130.1, 130.6, 134.1, 137.5, 144.2, 146.7, 148.5, 151.1, 164.5 ppm. Mass: m/z 371 (M+) (calcd for C23H21N3O2:371.43). FT-IR (KBr): νmax: 1646, 2852, 2936, 3119, 3294, 3448 cm−1. Anal. calcd. for: C23H21N3O2, C, 74.37; H, 5.70; N, 11.31%. Found: C, 74.29; H, 5.84; N, 11.52%.
White powder, mp: 226–232°C. 1H-NMR (400 MHz, CDCl3): δ = 1.25–1.52 (m, 5H), 1.68–1.83 (m, 3H), 2.09–2.12 (m, 2H), 3.94–4.04 (m, 1H), 7.54 (d, J = 8.0 Hz, 1H), 7.63 (s, 1H), 7.71 (dd, J = 9.0 Hz, J = 2.4 Hz, 1H), 7.86 (d, J = 2.4 Hz, 1H), 8.02 (s, 1H), 8.05 (d, J = 9.2 Hz, 1H), 8.34 (s, 1H) ppm. 13C-NMR (100 MHz, CDCl3): δ = 24.9, 25.6, 33.0, 48.7, 77.3, 121.2, 126.4, 126.6, 128.5, 131.0, 131.9, 134.5, 136.3, 144.1, 147.9, 149.0, 151.2, 164.2 ppm. Mass: m/z 355 (M+) (calcd. for C19H18 ClN3O2: 355.82). FT-IR (KBr): νmax: 663, 1649, 2852, 2923, 3279, 3443 cm−1. Anal. calcd. for: C19H18ClN3O2, C, 64.13; H, 5.10; N, 11.81%. Found: C, 64.20; H, 5.16; N, 11.70%.
White powder, mp: 190–197°C. 1H-NMR (400 MHz, DMSO-d6): δ = 1.43 (s, 9H), 7.75 (s, 1H), 7.86 (dd, J = 9.2 Hz, J = 2.4 Hz, 1H), 8.14 (d, J = 8.8 Hz, 1H), 8.28 (d, J = 2.4 Hz, 1H), 8.46 (s, 1H), 8.64 (s, 1H), 8.76 (s, 1H) ppm. 13C-NMR (100 MHz, DMSO-d6): δ = 28.8, 51.5, 119.5, 125.5, 127.4, 128.3, 131.3, 131.8, 132.7, 133.8, 144.5, 147.4, 153.4, 167.1 ppm. Mass: m/z 329.78 (M+) (calcd. for C17H16N3O2: 329.09). FT-IR (KBr): νmax: 1626, 2852, 2928, 3334, 3423 cm−1. Anal. calcd. for C17H16 ClN3O2: C, 61.91; H, 4.89; N, 12.74. Found: C, 62.04; H, 4.95; N, 12.89.
White powder, mp: 102–106°C. 1H-NMR (400 MHz, DMSO-d6): δ = 1.43 (s, 9H), 7.56 (s, 1H), 7.72 (t, J = 7.4 Hz, 1H), 7.87 (t, J = 7.6 Hz, 1H), 8.13 (t, J = 8.8 Hz, 2H), 8.41 (s, 1H), 8.61(s, 1H), 8.77 (s, 1H) ppm. 13C-NMR (100 MHz, DMSO-d6): δ = 28.8, 51.4, 118.7, 125.1, 127.5, 128.4, 128.8, 129.1, 131.4, 134.7, 146.0, 147.8, 153.0, 153.1, 167.4 ppm. Mass: m/z 295 (M+) (calcd. for C17H17N3O2: 295.34). FT-IR (KBr): νmax: 1658, 2904, 2966, 3422 cm−1. Anal. calcd. for C17H17N3O2: C, 69.14; H, 5.80; N, 14.23%. Found: C, 69.20; H, 5.87; N, 14.35%.
White powder, mp: 149–152°C. 1H-NMR (400 MHz, DMSO-d6): δ = 2.46 (s, 3H), 7.50 (d, J = 7.2 Hz, 2H), 7.77–7.90 (m, 6H), 8.18 (d, J = 7.6 Hz, 1H), 8.69 (s, 1H), 8.92 (s, 1H) ppm. 13C-NMR (100 MHz, DMSO-d6): δ = 21.6, 118.2, 127.6, 128.2, 128.9, 129.5, 129.6, 130.1, 130.6, 132.9, 135.5, 141.1, 144.9, 145.3, 145.6, 153.4, 155.1 ppm. Mass: m/z 350 (M+) (calcd for C19H14N2O3S:350.39). FT-IR (KBr): νmax: 683, 1073, 1103, 2851, 2920 cm−1. Anal. calcd. for: C19H14N2O3S, C, 65.13; H, 4.03; N, 7.99; S, 9.15%. Found: C, 65.23; H, 4.14; N, 8.06; S, 9.21%.
White powder, mp: 162–167°C. 1H-NMR (400 MHz, DMSO-d6): δ = 2.18 (s, 3H), 2.50 (s, 3H), 7.55 (d, J = 8.0 Hz, 1H), 7.71 (d, J = 6.8 Hz, 2H), 7.88 (s, 1H), 7.90 (d, J = 7.6 Hz, 2H), 8.03 (dd, J = 6.8 Hz, J = 2.8 Hz, 1H), 8.73 (s, 1H), 8.95 (s, 1H) ppm. 13C-NMR (100 MHz, DMSO-d6): δ = 16.5, 21.7, 117.5, 126.6, 127.7, 128.3, 129.9, 130.1, 130.4, 132.5, 135.3, 137.4, 140.3, 143.5, 145.3, 145.5, 153.6, 153.8 ppm. Mass: m/z 364 (M+) (calcd for C20H16N2O3S:364.42). FT-IR (KBr): νmax: 1294, 1379, 2852, 2922 cm−1. Anal. calcd. for: C20H16N2O3S, C, 65.92; H, 4.43; N, 7.69; S, 8.80%. Found: C, 66.03; H, 4.49; N, 7.78; S, 8.93%.
White powder, mp: 124–127°C. 1H-NMR (400 MHz, CDCl3): δ = 2.51 (s, 3H), 2.59 (s, 3H), 7.30 (s, 1H), 7.38 (d, J = 6.4 Hz, 2H), 7.62 (d, J = 8.4 Hz, 1H), 7.68 (s, 1H), 7.84 (d, J = 8.0 Hz, 1H), 7.89 (d, J = 7.6 Hz, 2H), 8.10 (s, 1H), 8.44 (s, 1H) ppm. 13C-NMR (100 MHz, DMSO-d6): δ = 21.6, 21.7, 118.3, 127.3, 127.5, 128.2, 129.2, 129.5, 130.0, 135.1, 135.7, 140.2, 140.8, 142.9, 143.6, 145.2, 145.7, 153.3 ppm. Mass: m/z 364 (M+) (calcd for C20H16N2O3S:364.42). FT-IR (KBr): νmax: 823, 1375, 2853, 2923 cm−1. Anal. calcd. for: C20H16N2O3S, C, 65.92; H, 4.43; N, 7.69; S, 8.80%. Found: C, 65.98; H, 4.49; N, 7.77; S, 8.86%.
White powder, mp: 187–190°C. 1H-NMR (400 MHz, CDCl3): δ = 2.51 (s, 3H), 3.99 (s, 3H), 7.16 (d, J = 8.8 Hz, 2H), 7.30 (s, 1H), 7.39 (s, 1H), 7.58 (d, J = 8.8 Hz, 2H), 7.84 (d, J = 9.2 Hz, 2H), 7.90 (d, J = 8.4 Hz, 1H), 8.10 (s, 1H), 8.42 (s, 1H) ppm. 13C-NMR (100 MHz, CDCl3): δ = 37.1, 55.8, 104.6, 119.1, 124.0, 124.4, 124.8, 129.2, 129.6, 131.6, 135.6, 137.5, 138.4, 138.5, 144.5, 147.1, 147.6, 147.7 ppm. Mass: m/z 380 (M+) (calcd for C20H16N2O4S: 380.42). FT-IR (KBr): νmax: 1037, 117, 2850, 2920 cm−1. Anal. calcd. for: C20H16N2O4S, C, 63.14; H, 4.24; N, 7.36; S, 8.43%. Found: C, 63.21; H, 4.29; N, 7.43; S, 8.55%.
White powder, mp: 202–207°C. 1H-NMR (400 MHz, CDCl3): δ = 2.60 (s, 3H), 7.30 (s, 1H), 7.52 (d, J = 8.0 Hz, 2H), 7.57 (t, J = 7.2 Hz, 1H), 7.70 (t, J = 6.8 Hz, 1H), 7.73 (d, J = 8.8 Hz, 1H), 7.89 (d, J = 8.4 Hz, 1H), 7.92 (d, J = 9.2 Hz, 1H), 8.04 (d, J = 8.4 Hz, 2H), 8.16 (d, J = 5.6 Hz, 1H), 8.20 (d, J = 8.4 Hz, 1H), 8.60 (s, 1H) ppm. 13C-NMR (100 MHz, CDCl3): δ = 21.8, 119.0, 124.1, 124.4, 127.0, 127.8, 128.0, 128.7, 129.3, 129.3, 130.2, 130.5, 131.1, 134.0, 135.3, 137.5, 134.3, 144.8, 145.5, 151.6, 153.3 ppm. Mass: m/z 400 (M+) (calcd for C23H16N2O3S: 400.45). FT-IR (KBr): νmax: 1313, 1400, 2853, 2920 cm−1. Anal. calcd. for: C23H16N2O3S, C, 68.98; H, 4.03; N, 7.00; S, 8.01%. Found: 69.06; H, 4.11; N, 7.12; S, 8.14%.
In continuation of our interest in quinoline chemistry (Shiri et al., 2012, 2016a, 2017a) and isocyanide reactions (Shiri et al., 2016b, 2017b; Salehi and Shiri, 2019), we began our investigation with 2-chloroquinoline-3-carbaldehyde (1) and its two step reaction with two different isocyanides. In the presence of K2CO3, 2-chloroquinoline-3-carbaldehyde (1) and 4-toluenesulfonylmethyl isocyanide (TosMIC) (2) furnished 5-(2-chloroquinolin-3-yl)oxazole (3a). Several 5-(2-chloroquinolin-3-yl)oxazoles (3) were prepared under the same conditions. The reaction of quinoline 3a with cyclohexyl isocyanide 4a was selected as a model reaction in the presence of Pd(OAc)2, Ph3P, and Cs2CO3, in 1,4-dioxane with a few drops of H2O as solvent at 80 °C. Desired product 5a was obtained in 86% yield (Table 1). Solvent screening showed that DMSO is the best solvent (Table 1, entry 6). Other Pd sources such as Pd(PPh3)4 and PdCl2 did not improve the product yield, however, the best yield was obtained with 5 mol% of Pd(OAc)2 even without PPh3 (Table 1, entries 7–9). Without palladium, the reaction did not occur (Table 1, entry 10). Moreover, the effect of base is crucial for reaction completion. Hence, different bases were investigated, including K2CO3, NaOAc, (CH3)3OK, DABCO, and Et3N (Table 1, entries 14–18). In this survey, it was found that increasing the temperatures or the reaction time decreased the product yield.
Table 1. Optimization of the reaction condition for the synthesis of N-cyclohexyl-3-(oxazol-5-yl)quinoline-2-carboxamide 5a from 3a.
With the optimized reaction conditions in hand (Pd(OAc)2 (5 mol%), Cs2CO3 (1 equiv.), DMSO + H2O (9:1), 80°C), the generality of the reaction was explored (Table 2). A range of quinolines 3 bearing electron-donating groups, such as Me, and OMe and electron-withdrawing groups, such as Cl and benzo, reacted with cyclohexyl isocyanide and n-butylisocyanide to afford the corresponding 3-(oxazol-5-yl)quinoline-2-carboxamides 5a-f in 70%-94% yields (Table 2). Moreover, the bulky tert-butyl isocyanide smoothly participated in this reaction to furnish 5g and 5h in 67 and 76% yield, respectively. The yield with 1,1,3,3-tetramethylbutyl isocyanide was too low to allow its isolation and characterization.
The scope of the reaction was explored using tosylmethyl isocyanide (TosMIC) 1 as another isocyanide source. Surprisingly, the reaction of 3a with TosMIC under the optimized conditions afforded 5-(2-tosylquinolin-3-yl)oxazole (6a) (Scheme 1).
Although the reaction proceeded well without a palladium source, the presence of base is crucial. Among the bases Cs2CO3, K2CO3, NaOAc, t-BuOK, and DABCO, Cs2CO3 gave the best results.
Encouraged by the tosylation results with TosMIC, we explored extending the reaction to tandem oxazole formation as well as tosylation of 2-chloroquinoline-3-carbaldehyde. Subjecting 2-chloroquinoline-3-carbaldehyde and TosMIC to the standard reaction conditions yielded 5-(2-tosylquinolin-3-yl)oxazole (6a) in 83% yield after 8 h (Scheme 2). Notably, sulfones are present in different bioactive compounds (Metzner and Thuillier, 1994; Fang et al., 2016); however, well-known sulfonylating agents include sulfonyl halides (Tocco et al., 2013; Zhang et al., 2015), sulfonyl hydrazides (Yuan et al., 2018; Zhang et al., 2018), and sodium sulfinate (Sun et al., 2017; Smith et al., 2018). A few studies used TosMIC as a sulfonyl precursor (Liu et al., 2014; Phanindrudu et al., 2016; Kadari et al., 2017). Furthermore, Bounar et al. reacted tosylmethyl isocyanide (TosMIC) with propargylic alcohols in the presence of silver acetate to efficiently yield (E)-vinyl sulfones (Bounar et al., 2015); this is the only study in which TosMIC plays a dual role as both an amide and a sulfonyl source.
The above cascade oxazole formation and sulfonylation strategy could be extended to other 2-chloroquinoline-3-carbaldehyde derivatives (Figure 2). A methyl group was tolerated on positions 6 and 8 of 2 to afford 6b and 6c, respectively, in 82 and 62% yields. Furthermore, quinoline 2d reacted with TosMIC, affording 6d in good yield. Product 6e, existing an alternative decoration of the quinoline ring, was obtained in 85% yield.
Our proposed mechanism for the tosylation of quinoline involved in situ Ts− generation by decomposition of p-toluenesulfonylmethyl-isocyanide 1 in the presence of base with subsequent aromatic nucleophilic substitution to form 2-sulfonyl quinoline 6. Although application of TosMIC as a sulfonyl source was reported by Liu et al. for synthesizing sulfonyl benzoheteroles, the sulfonation mechanism involved aliphatic nucleophilic substitution (Liu et al., 2014).
In summary, we have developed a synthesis of 5-(2-chloroquinolin-3-yl)oxazole via a van Leusen procedure from 2-chloroquinoline-3-carbaldehydes and TosMIC, which were efficiently subjected to Pd-catalyzed amidation with isocyanides to form 3-(oxazol-5-yl)quinoline-2-carboxamides. The synthesis of 5-(2-tosylquinolin-3-yl)oxazole via a Cs2CO3-mediated domino process starting from 2-chloroquinoline-3-carbaldehydes with TosMIC was also demonstrated.
ZM, ZT, and SF synthesized all of the compounds with the help of ZY. MS supervised this work and wrote the paper with the help of ZY.
The authors declare that the research was conducted in the absence of any commercial or financial relationships that could be construed as a potential conflict of interest.
We are thankful to Alzahra University and the Iran National Science Foundation (INSF) for the financial support.
The Supplementary Material for this article can be found online at: https://www.frontiersin.org/articles/10.3389/fchem.2019.00433/full#supplementary-material
Supplementary Data Sheet 1. Isocyanide reactions toward the synthesis of 3-(Oxazol-5-yl)quinoline-2-carboxamides and 5-(2-Tosylquinolin-3-yl)oxazole.
Åkerbladh, L., Schembri, L. S., Larhed, M., and Odell, L. R. (2017). Palladium(0)-catalyzed carbonylative one-pot synthesis of N-acylguanidines. J. Org. Chem 82, 12520–12529. doi: 10.1021/acs.joc.7b02294
Bode, J. W. (2006). Emerging methods in amide- and peptide-bond formation. Curr. Opin. Drug Discov. Devel. 9, 765–775. doi: 10.1002/chin.200721229
Bounar, H., Liu, Z., Zhang, L., Guan, X., Yang, Z., Liao, P., et al. (2015). Silver-catalyzed cascade reaction of tosylmethyl isocyanide (TosMIC) with propargylic alcohols to (E)-vinyl sulfones: dual roles of TosMIC. Org. Biomol. Chem. 13, 8723–8728. doi: 10.1039/C5OB01129A
Chaudhary, A., Sharma, P. P., Bhardwaj, G., Jain, V., Bharatam, P. V., Shrivastav, B., et al. (2013). Synthesis, biological evaluation, and molecular modeling studies of novel heterocyclic compounds as anti-proliferative agents. Med. Chem. Res. 22, 5654–5669. doi: 10.1007/s00044-013-0556-x
Davyt, D., and Serra, G. (2010). Thiazole and oxazole alkaloids: isolation and synthesis. Mar. Drugs 8, 2755–2780. doi: 10.3390/md8112755
Domling, A. (2006). Recent developments in isocyanide based multicomponent reactions in applied chemistry. Chem. Rev. 106, 17–89. doi: 10.1021/cr0505728
Domling, A., and Ugi, I. (2000). Multicomponent eeactions with isocyanides. Angew. Chem. Int. Ed 39, 3168–3210. doi: 10.1002/1521-3773(20000915)39:18<3168::AID-ANIE3168>3.0.CO;2-U
Fang, Y., Luo, Z., and Xu, X. (2016). Recent advances in the synthesis of vinyl sulfones. RSC Adv. 6, 59661–59676. doi: 10.1039/c6ra10731a
Guan, Z.-R., Liu, Z.-M., and Ding, M. W. (2018). New efficient synthesis of 1H-imidazo-[4,5-c]quinolines by a sequential Van Leusen/Staudinger/aza-Wittig/carbodiimide-mediated cyclization. Tetrahedron 74, 7186–7192. doi: 10.1016/j.tet.2018.10.052
Hranjec, M., Horak, E., Babic, D., Plavljanin, S., Srdovic, Z., Steinberg, I. M., et al. (2017). Fluorescent benzimidazo[1,2-a]quinolines: synthesis, spectroscopic and computational studies of protonation equilibria and metal ion sensitivity. N. J. Chem. 41, 358–371. doi: 10.1039/c6nj02268e
Ishida, K., Nakagawa, H., and Murakami, M. (2000). Microcyclamide, a cytotoxic cyclic hexapeptide from the Cyanobacterium microcystis aeruginosa. J. Nat. Prod 63, 1315–1317. doi: 10.1021/np000159p
Jiang, H., Liu, B., Li, Y., Wang, A., and Huang, H. (2011). Synthesis of Amides via palladium-catalyzed amidation of aryl halides. Org. Lett. 13, 1028–1031. doi: 10.1021/ol103081y
Kadari, L., Palakodety, R. K., and Yallapragada, L. P. (2017). Electrochemical decarboxylative sulfonylation of cinnamic acids with aromatic sulfonylhydrazides to vinyl sulfones. Org. Lett. 19, 2580–9661. doi: 10.1021/acs.joc.7b01741
Linington, R. G., González, J., Ureña, L., Romero, L. I., Ortega-Barría, E., and Gerwick, W. H. (2007). Venturamides, A and B: antimalarial constituents of the panamanian marine cyanobacterium Oscillatoria sp. J. Nat. Prod. 70, 397–401. doi: 10.1021/np0605790
Liu, J., Liu, Z., Liao, P., and Bi, X. (2014). Modular synthesis of sulfonyl benzoheteroles by silver-catalyzed heteroaromatization of propargylic alcohols with p-toluenesulfonylmethyl isocyanide (TosMIC): dual roles of TosMIC. Org. Lett. 16, 6204–6207. doi: 10.1021/ol5031316
Marco-Contelles, J., Perez-Mayoral, E., Samadi, A., Carreiras, M. D., and Soriano, E. (2009). Recent advances in the Friedländer reaction. Chem. Rev. 109, 2652–2671. doi: 10.1021/cr800482c
Metzner, P., and Thuillier, A. (1994). Sulfur Reagents in Organic Synthesis. Best synthetic methods. eds A. R. Katritzky, O. Meth-Cohn, and C. W. Rees (London: Academic Press).
Michael, J. P. (2002). Quinoline, quinazoline and acridone alkaloids. Nat. Prod. Rep. 19, 742–760. doi: 10.1039/B104971M
Montalbetti, C. A. G. N., and Falque, V. (2005). Amide bond formation and peptide coupling. Tetrahedron. 61, 10827–10852. doi: 10.1016/j.tet.2005.08.031
Nainwal, L. M., Tasneem, S., Akhtar, W., Verma, G., Khan, M. F., Parvez, S., et al. (2019). Green recipes to quinoline: a review. Eur. J. Med. Chem. 164, 121–170. doi: 10.1016/j.ejmech.2018.11.026
Pathare, R. S., Sharma, S., Elagandhula, S., Saini, V., Sawant, D. M., Yadav, M., et al. (2016). Application of isocyanides as amide surrogates in the synthesis of diverse isoindolin-1-one derivatives by a palladium-catalyzed tandem carboxamidation/hydroamidation reaction. Eur. J. Org. Chem. 2016, 5579–5587. doi: 10.1002/ejoc.201600999
Phanindrudu, M., Tiwari, D. K., Sridhar, B., Likhar, P. R., and Tiwari, D. K. (2016). Magnetically separable nano-copper catalyzed unprecedented stereoselective synthesis of E-vinyl sulfones from tosylmethyl isocyanide and alkynes: TosMIC as a source of the sulfonyl group. Org. Chem. Front. 3, 795–798. doi: 10.1039/C6QO00063K
Prajapati, S. M., Patel, K. D., Vekariya, R. H., Panchal, S. N., and Patel, H. D. (2014). Recent advances in the synthesis of quinolines: a review. Rsc Adv. 4, 24463–24476. doi: 10.1039/c4ra01814a
Raveh, A., Moshe, S., Evron, Z., Flescher, E., and Carmeli, S. (2010). Novel thiazole and oxazole containing cyclic hexapeptides from a waterbloom of the Cyanobacterium microcystis sp. Tetrahedron 66, 2705–2712. doi: 10.1016/j.tet.2010.02.008
Rönn, R., Lampa, A., Peterson, S. D., Gossas, T., Åkerblom, E., Danielson, U. H., et al. (2008). Hepatitis C virus NS3 protease inhibitors comprising a novel aromatic P1 moiety. Bioorg. Med. Chem. 16, 2955–2967. doi: 10.1016/j.bmc.2007.12.041
Salehi, P., and Shiri, M. (2019). Palladium-catalyzed regioselective synthesis of 3-(hetero)arylpropynamides from gem-dibromoalkenes and isocyanides. Adv. Synth. Catal. 361, 118–125. doi: 10.1002/adsc.201800963
Sharma, R., Kour, P., and Kumar, A. (2018). A review on transition-metal mediated synthesis of quinolines. J. Chem. Sci. 130:73. doi: 10.1007/s12039-018-1466-8
Shiri, M., Faghihi, Z., Oskouei, H. A., Heravi, M. M., Fazelzadeh, S., and Notash, B. (2016a). The synthesis of iminothiophenone-fused quinolines and evaluation of their serendipitous reactions. RSC Adv. 6:92235. doi: 10.1039/c6ra11469e
Shiri, M., Heydari, M., and Zadsirjan, V. (2017b). Efficient synthesis of novel functionalized pyrazolo-pyranoquinoline and tetrahydrodibenzo-[1,8]naphthyridinone derivatives. Tetrahedron 73,2116–2122. doi: 10.1016/j.tet.2017.02.064
Shiri, M., Pourabed, R., Zadsirjan, V., and Sodagar, E. (2016b). Highly selective organocatalytic three-component reaction of 2-chloroquinoline-3-carbaldehydes, 6-aminouracils, and cyclic methylene active compounds. Tetrahedron Lett. 57, 5435–5438. doi: 10.1016/j.tetlet.2016.10.057
Shiri, M., Ranjbar, M., Yasaei, Z., Zamanian, F., and Notash, B. (2017a). Palladium-catalyzed tandem reaction of 2-chloroquinoline-3-carbaldehydes and isocyanides. Org. Biomol. Chem. 15:10073. doi: 10.1039/c7ob02043k
Shiri, M., Zolfigol, M. A., Kruger, H. G., and Tanbakouchian, Z. (2011). Friedlander annulation in the synthesis of azaheterocyclic compounds. Adv. Heterocycl. Chem. 185, 139–227. doi: 10.1016/B978-0-12-385464-3.00002-9
Shiri, M., Zolfigol, M. A., Pirveysian, M., Ayazi-Nasrabadi, R., Kruger, H. G., Naicker, T., et al. (2012). A new and facile access to the 2-(indol-3-yl)-3-nitriloquinolines based on Friedlander annulations. Tetrahedron 68, 6059–6064. doi: 10.1016/j.tet.2012.05.006
Smith, J. D., Ansari, T. N., Andersson, M. P., Yadagiri, D., Ibrahim, F., Liang, S., et al. (2018). Micelle-enabled clean and selective sulfonylation of polyfluoroarenes in water under mild conditions. Green Chem. 20, 1784–1790. doi: 10.1039/C7GC03514D
Sun, Y., Abdukader, A., Lu, D., Zhang, H., and Liu, C. (2017). Synthesis of (E)-β-iodo vinylsulfones via iodine-promoted iodosulfonylation of alkynes with sodium sulfinates in an aqueous medium at room temperature. Green Chem. 19, 1255–1258. doi: 10.1039/C6GC03387C
Tocco, G., Begala, M., Esposito, F., Caboni, P., Cannas, V., and Tramontano, E. (2013). ZnO-mediated regioselective C-arylsulfonylation of indoles: a facile solvent-free synthesis of 2- and 3-sulfonylindoles and preliminary evaluation of their activity against drug-resistant mutant HIV-1 reverse transcriptases (RTs). Tetrahedron Lett. 54, 6237–6241. doi: 10.1016/j.tetlet.2013.09.017
Tyagi, V., Khan, S., Giri, A., Gauniyal, H. M., Sridhar, B., and Chauhan, P. M. S. (2012). A ligand-free Pd-catalyzed cascade reaction: an access to the highly diverse isoquinolin-1(2H)-one derivatives via isocyanide and Ugi-MCR synthesized amide precursors. Org. Lett. 14, 3126–3129. doi: 10.1021/ol301131s
Ulijn, R. V., Moore, B. D., Janssen, A. E. M., and Halling, P. J. (2002). A single aqueous reference equilibrium constant for amide synthesis–hydrolysis. J. Chem. Soc. Perkin Trans. 2 1024–1028. doi: 10.1039/B108041E
Vlaar, T., Ruijter, E., Znabet, A., Janssen, E., de Kanter, F. J. J., Maes, B. U. W., et al. (2011). Palladium-catalyzed synthesis of 4-aminophthalazin-1(2H)-ones by isocyanide insertion. Org. Lett. 13, 6496–6499. doi: 10.1021/ol202784d
Wang, L., Woods, K. W., Li, Q., Barr, K. J., McCroskey, R. W., Hannick, S. M., et al. (2002). Potent, orally active heterocycle-based combretastatin A-4 cnalogues: synthesis, structure–activity relationship, pharmacokinetics, and in vivo antitumor activity evaluation. J. Med. Chem. 45, 1697–1711. doi: 10.1021/jm010523x
Yavari, I., Ghazanfarpour-Darjani, M., and Bayat, M. J. (2014). Synthesis of amides via copper-catalyzed amidation of aryl halides using isocyanides. Tetrahedron Lett. 55, 4981–4982. doi: 10.1016/j.tetlet.2014.05.032
Yu, L.-L., Li, Z. -Y., Peng, C. -S., Li, Z. -Y., and Guo, Y. -W. (2009). Neobacillamide, A., a novel thiazole-containing alkaloid from the marine bacterium Bacillus vallismortis C89, associated with south China sea sponge Dysidea avara. Helv. Chim. Acta 92, 607–612. doi: 10.1002/hlca.200800349
Yuan, Y., Yu, Y., Qiao, J., Liu, P., Yu, B., Zhang, W., et al. (2018). Exogenous-oxidant-free electrochemical oxidative C–H sulfonylation of arenes/heteroarenes with hydrogen evolution. Chem. Commun. 54, 11471–11474. doi: 10.1039/C8CC06451B
Zhang, D., Cui, X., Zhang, Q., and Wu, Y. (2015). Pd-Catalyzed direct C–H bond sulfonylation of azobenzenes with arylsulfonyl chlorides. J. Org. Chem. 80, 1517–1522. doi: 10.1021/jo502451k
Keywords: palladium acetate, carboxamidation, isocynides, sulfonylation, TosMIC
Citation: Yasaei Z, Mohammadpour Z, Shiri M, Tanbakouchian Z and Fazelzadeh S (2019) Isocyanide Reactions Toward the Synthesis of 3-(Oxazol-5-yl)Quinoline-2-Carboxamides and 5-(2-Tosylquinolin-3-yl)Oxazole. Front. Chem. 7:433. doi: 10.3389/fchem.2019.00433
Received: 03 January 2019; Accepted: 28 May 2019;
Published: 14 June 2019.
Edited by:
Jonathan G. Rudick, Stony Brook University, United StatesReviewed by:
Steven Ballet, Vrije University Brussel, BelgiumCopyright © 2019 Yasaei, Mohammadpour, Shiri, Tanbakouchian and Fazelzadeh. This is an open-access article distributed under the terms of the Creative Commons Attribution License (CC BY). The use, distribution or reproduction in other forums is permitted, provided the original author(s) and the copyright owner(s) are credited and that the original publication in this journal is cited, in accordance with accepted academic practice. No use, distribution or reproduction is permitted which does not comply with these terms.
*Correspondence: Morteza Shiri, bXNoaXJpQGFsemFocmEuYWMuaXI=
Disclaimer: All claims expressed in this article are solely those of the authors and do not necessarily represent those of their affiliated organizations, or those of the publisher, the editors and the reviewers. Any product that may be evaluated in this article or claim that may be made by its manufacturer is not guaranteed or endorsed by the publisher.
Research integrity at Frontiers
Learn more about the work of our research integrity team to safeguard the quality of each article we publish.