- School of Environmental and Resource Sciences, Zhejiang A & F University, Hangzhou, China
In this study, La and Ce doped PbO2 electrodes were prepared and the characteristic of the electrodes were discussed with the help of structure analysis. The catalytic effects of the doped electrodes were explored through the degradation of para-aminophenol wastewater. The results showed that the para-aminophenol removal was 96.96%, 89.34%, and 77.55% after 180 min treatment with Ce-PbO2, La-PbO2, and PbO2, respectively. The para-aminophenol enhanced degradation mechanism was discussed with rare earth element doping electrodes and a kinetic model was established based on radical reactions mechanism with genetic algorithm (GA) calculation. The reaction constants of these electrodes were calculated and the results showed that the reaction constant of Ce-PbO2 electrode was the highest, which indicated that Ce-PbO2 electrode could have a better treatment effect. The EE/O was used as the index of energy consumption efficiency and the results were calculated and compared. This paper could provide basic data and technique reference of the prediction the oxidation reaction process of different electrodes for the electrochemical oxidation application in wastewater treatment.
Introduction
Para-aminophenol (PAP) is the primary material of paracetamol industry, which was widely used in a number of countries (Bloomfield, 2002; Bahrami and Salehabadi, 2014). Due to the characteristic of the pharmacy production process, the PAP would be contained in the wastewater. If this kind wastewater was discharged directly into water environment, there would affect human's health (Hallman and Tarloff, 2000; Li et al., 2004; Harmon et al., 2005; Khodaveisi et al., 2015). Therefore, the degradation and mineralization of PAP wastewater and relative pharmaceutical wastewater has received a great concern in the field of environment.
As one of advanced oxidation processes (AOPs), electrochemical oxidation could remove bio-refractory organic pollutants in wastewater and has the advantages of relatively high treatment efficiency with easy operation (Velegraki et al., 2010; Chen et al., 2014; Xia et al., 2015a). Electrode materials, such as boron-doped diamond, platinum, IrO2, RuO2, and PbO2 and carbon relative materials (Arapoglou et al., 2003; Zhuo et al., 2011; Li et al., 2014; Wang et al., 2019), play an important role in electrochemical reaction. The β-PbO2 electrode and rare earth element doped β-PbO2 electrode were widely investigated due to the easy preparation technology and excellent degradation effect (Aquino et al., 2014; Mukimin et al., 2015; Xia et al., 2015b; Dai et al., 2016). However, the study of the reactions kinetic model on the doped and undoped PbO2 electrodes is still limited.
In this study, PAP was selected as the model pollutant and the degradation of PAP wastewater was studied with the electrodes of PbO2, La doped PbO2 (La-PbO2), and Ce doped PbO2 (Ce-PbO2). A kinetic model was established based on genetic algorithm (GA) calculation and used to quantify the performance of electrode materials. This paper could provide basic data and theoretical support for PAP wastewater treatment by electrochemical oxidation.
Experimental
Chemicals
PAP was selected as the model pollutant and obtained from Shanghai J&K Chemical Reagent (China) Co., Ltd. (purity 99.5%, wt%). The structure formula and general characteristics of PAP were given in Table 1. Other chemicals were purchased from Aladdin Reagent (China) Co., Ltd. All the other reagents were of analytical grad.
Electrode Preparation
The detail process for the preparation and characteristic of the electrodes has been fully described in previous literatures (Weng et al., 2013; Dai et al., 2014; Weng and Pei, 2016). Briefly, the electrode included three layers: the Ti substrate inner layer, thermally deposited Sn-SbOx intermediate layer, and the electrodeposited α-PbO2 and β-PbO2 outer layer. When La-PbO2 and Ce-PbO2 electrodes were prepared, certain amounts of La or Ce nitrate were added to the acid solution for the electro-deposition of β-PbO2. Compared with undoped PbO2 electrode, the surface and structure analysis results of doped PbO2 showed that the electrodes after doped with rare earth could effectively improve the crystallinity of PbO2 crystal on electrode surface. The tinier grain crystals on the doped electrodes could promote the conductivity, stability, and catalytic activity of electrode, which would be potential widely applied in dye wastewater pollution control in application filed.
Analysis Methods
The PAP concentration was analyzed by high performance liquid chromatography (Agilent 1200, USA) with C18 column and UV detector. The UV detector wavelength was set at 220 nm. The injection volume was 10 μl. The mobile phase was methanol and H3PO4 solution (1‰) and the ratio of methanol with H3PO4 solution was 22:78 (v/v). The flow rate was 0.4 ml min−1. The crystal structure was investigated by X-ray diffraction (XRD) instrument with CuKα radiating (Rigaku D/MAX 2500PC, Japan) under condition of 40 kV voltage and 300 mA tube current. The continuous scan mode was used and the scan speed was 4°·min−1 with the range of 10°~90°.
The average current efficiency (ACE) was calculated by chemical oxygen demand (COD) and current data at time intervals (Comninellis and Pulgarin, 1991).
Where: COD0 and CODt are the CODs (in O, g·L−1) of samples at different time (s), respectively. F is the faraday constant (96487 C·mol−1), V is the volume of the electrolyte (L), I is the electrolysis current (A), and t is the reaction time (s).
Experimental Procedure
PAP wastewater degradation experiment was performed in a 250 ml customize reactor. During PAP degradation, samples were taken at presumed intervals to determine the concentration of PAP, COD, and total organic carbon (TOC). All tests were run three times. The results for each experimental condition were the means of the three experiments and the relative standard deviations (RSD) were <5%.
Modeling Approach
To establish model, the rate constants were calculated with the degradation data of PAP concentration. The minimum value of residual between experimental data and modeling data as the objective function was calculated by GA calculation (Goldberg, 1989; Ndiritu and Daniell, 2001).
The objective function (OF) was listed as follows:
Where: OF = objective function, dimensionless, n = number of data points, unitless
Cdata,i = measured concentration of data point i, mmol L−1,
Cmodel,i = predicted concentration of data point i, mmol L−1
All the reaction constants (k) were calculated by the GA method.
Results and Discussion
PAP Degradation With Different Electrodes
PbO2 electrode, La-PbO2 electrode and Ce-PbO2 electrode were applied for the degradation of PAP wastewater on the condition of electrolyte concentration Na2SO4 0.1 mol·L−1, PAP concentration 500 mg·L−1 and current density 70 mA·cm−2. The degradation results were shown in Figure 1.
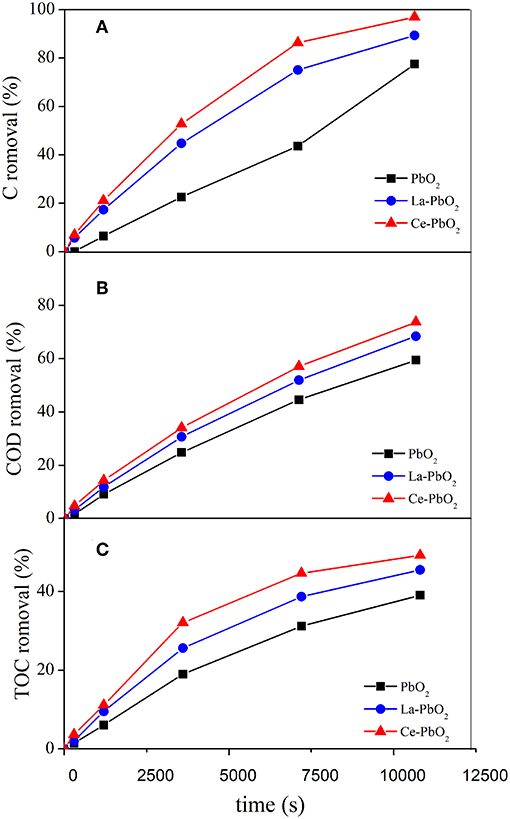
Figure 1. Effect of different electrodes on PAP degradation: (A) Concentration Removal, (B) COD Removal, (C) TOC Removal.
The PAP removal with Ce-PbO2 electrode was 96.96%, which is higher than the removal with La-PbO2 (89.34%) and PbO2 electrodes (77.55%) after 3 h treatment. The COD and TOC removal after 3 h for these three electrodes was 73.79, 68.49, 59.49%, and 49.23, 45.47, 39.08%, respectively. And these results were showed in Figures 1A–C, respectively. Under the same conditions, the ACE of the La-PbO2 and Ce-PbO2 electrode were higher 8.35 and 11.69% than that of undoped electrode (42.05%). The results demonstrated that the catalytic activity of La-PbO2 and Ce-PbO2 were improved degradation of PAP effectively. Also, the Ce-PbO2 electrode displayed a higher removal efficiency and faster mineralization than La-PbO2 and PbO2 electrodes for PAP degradation.
Materials Characterization
Figure 2 showed the XRD patterns of different electrodes. Compared with PbO2, La and Ce doping would cause the change of crystal orientation of β-PbO2. After doped, the lattice plane of (110) was enhanced, which would influence the catalytic activity for organics degradation. The SEM results showed that after doped, the electrode surface had a tinier crystal structure and higher degree of crystallinity (Weng et al., 2013), which means that the addition of Ce could effectively improve the crystallinity of PbO2 crystal on electrode surface, and thus could increase the efficiency of PAP degradation.
Kinetics Model Study
The Key Reaction in Electrochemical Oxidation System
A generalized scheme of the electrochemical degradation of PAP with PbO2 electrode was shown in Figure 3. Firstly (No. 1, Table 2), H2O was decomposed on the electrode surface to yield adsorbed hydroxyl radical PbO2 (•OH). Secondly (No. 2, Table 2), hydroxyl radical on the oxide electrode could both react with PAP and form the lattice oxide of PbO2 (•O). Thus, there are two kinds of “active oxygen” could be existed on the PbO2 electrode surface: physisorption “active oxygen” PbO2 (•OH) and chemisorbed “active oxygen” PbO2 (•O) (Comminellis, 1994).
Due to the instability, the chemisorbed “active oxygen” PbO2(•O) would decompose according to the equation (No. 3, Table 2). The PbO2 (•O) would participate in the reaction of oxidation PAP, as was listed in the equation (No. 4, Table 2), while PbO2(•OH) complete fast reaction of oxidation PAP and mineralization them completely, according to No. 5 in Table 2 (Buxton et al., 1988; Simond et al., 1996). The hydroxyl radicals also might interact and the reaction constant of k4 means the interaction of hydroxyl radicals (No. 6, Table 2). The ionization balances and carbonate quenching equation were considered in our system list in Table 2.
From the No. 4 and No. 5 in Table 2, the ordinary differential equation (ODE) of PAP degradation rate with the time was deduced as follows, which included PAP reaction with PbO2 (•OH) and PbO2 (•O):
From the No. 1, 2, 5, 6, 7, and 8 in Table 2, the ODE of PbO2 (•OH) reaction rate variation with the time was studied, which included both yielding and decaying of PbO2(•OH):
From the No. 2, No. 3 and No. 4 in Table 2, the ODE of PbO2 (•O) reaction rate variation with the time was deduced, which included yielding and decaying of PbO2 (•O):
The ODE of HCO3− and CO32− termination the reaction with the time was deduced, including the key reaction No. 7 and No. 8 in Table 2:
These ODEs were calculated, as were listed in Table 3. The reaction constants of k1, k2, and k3 represent the speed of PbO2(•OH), PbO2(•O) formation and PbO2(•O) decomposition, which were determined by the properties of electrode materials on a certain external condition. The larger value of k1 means that the production of HO• and degradation PAP efficient would be enhanced. The larger of k2 means that the larger probability of physisorbed •OH could transfer to PbO2(•O), which would react with PAP relatively slowly and competed with oxygen evolution from PbO2(•O) (represented by k3). As the concentration of hydroxyl radicals is very little, so the interaction of hydroxyl radicals (k4) could be ignored. The kO and kHO represent the speed of PAP reaction with “active oxygen.” These reaction constants were calculated by least square method and GA.
Rate Constants Calculated and Modeling
The model assumption as follows:
(1) Solution in reaction system mixed completely, where species concentration uniform.
(2) Reaction temperature was constant.
(3) The O2 evolution of physisorbed “active oxygen” PbO2 (•OH) was ignored; The O2 evolution of chemisorbed “active oxygen” PbO2(•O) were considered. The PbO2 (•OH) and PbO2 (•O) were the main radical reaction with PAP in the solution.
Figure 4 showed the comparison between the predicted and experimental decomposition of PAP under condition of PAP initial concentration 500 mg▪L−1, Na2SO4 0.1 mol▪L−1 and current density 70 mA▪cm−2. The kinetic model was established by least square method and GA. The pseudo reaction constants k▪O was much less than k▪OH, which indicated that the activity of physisorbed “active oxygen” is much stronger than chemisorbed “active oxygen.”
Kinetics and Mechanism Analysis
Compared with PbO2 electrode (a), the La doped PbO2 electrode (b) and Ce doped PbO2 electrode(c) would lead to increase active points on crystal plane (Feng et al., 2008), which would lead to the increase of k1 and decrease of k2 and k3. After comparing k1 of undoped and doped PbO2 electrodes, it could be found that Ce-PbO2 had a greater k1 value, which indicated PbO2(•OH) was easier produced from electrode material of Ce-PbO2. Comparing k2 and k3 of doped PbO2 electrodes, it could be found that Ce-PbO2 had a smaller k2 and k3 value, which indicated the speed of physisorbed “active oxygen” transfer to crystals and lattice oxygen releasing of superoxide was weaker. Therefore, the catalytic efficiency of the three electrodes is Ce-PbO2 > La-PbO2 > PbO2.
The intermediates during the PAP degradation were analyzed. Kinds of intermediates were detected at time intervals, including maleic acid, acetic acid, formic acid, NO2− and NO3−. The results showed that the degradation of PAP would open loop into small molecule acid, such as maleic acid, acetic acid, formic acid on the condition of PbO2 (•OH) effective. Then kinds of small molecule acids would be degraded into to CO2 and H2O in the process of electrochemical oxidation.
EE/O Calculation
The electrical efficiency per log order (EE/O) is one of the best conceptions to describe energy utilization, which could be calculated by the established model. The EE/O calculation using equation (Crittenden et al., 2012; Butcher, 2016):
Where: EE/O = the electrical efficiency per log order reduction (kWh m−3);
P = the average power output (kW), t is the electrolysis time (h);
V = the wastewater volume (m3);
C0 = the initial PAP concentration (mg L−1);
Ct = the PAP concentration at different time (mg L−1);
The results of EE/O at time intervals were showed in Figure 5. The final EE/O of PbO2, La-PbO2, and Ce-PbO2 were 51.79 kWh m−3, 34.56 kWh m−3, 22.22 kWh m−3. The EE/O of Ce-PbO2 was <34% of La-PbO2 and 55% of PbO2.
Conclusions
The Ce-PbO2 electrode was successful prepared and used for PAP wastewater treatment. It was found that the novel Ce doped electrode is much more energy saving than La-PbO2 and PbO2. The EE/O of Ce-PbO2 was 34% less than that of La-PbO2 and 55% of PbO2. A kinetic model was established base on GA calculation, which could help us evaluate the catalytic efficiency of doped PbO2 electrode and predicted the reaction process on the surface of the electrode. The main advantage of this paper is to give a method for the energy consumption analysis based on pseudo element reaction calculation. This paper could provide basic data and technique reference of the prediction the oxidation reaction process of different electrodes for the electrochemical oxidation application in wastewater treatment.
Author Contributions
MW designed and co-carried the experiment, performed the analysis and wrote the manuscript. XY co-carried the experiment.
Funding
The authors are grateful for the financial support provided by the Research Development Fund of Zhejiang Agriculture and Forestry University (2012FR088).
Conflict of Interest Statement
The authors declare that the research was conducted in the absence of any commercial or financial relationships that could be construed as a potential conflict of interest.
References
Aquino, J. M., Rocha-Filho, R. C. L., Ruotolo, A. M., Bocchi, N., and Biaggio, S. R. (2014). Electrochemical degradation of a real textile wastewater using β-PbO2 and DSA® anodes. Chem. Eng. J. 251, 138–145. doi: 10.1016/j.cej.2014.04.032
Arapoglou, D., Vlyssides, A., Israilides, C., Zorpas, A., and Karlis, P. (2003). Detoxification of methyl-parathion pesticide in aqueous solutions by electrochemical oxidation. J. Hazard. Mater. 98, 191–199. doi: 10.1016/S0304-3894(02)00318-7
Bahrami, H., and Salehabadi, H. (2014). Experimental and theoretical investigation of para-aminophenol ionization in corona discharge. J. Mol. Struct. 1038, 330–335. doi: 10.1016/j.molstruc.2014.12.012
Bloomfield, M. S. (2002). A sensitive and rapid assay for 4-aminophenol in paracetamol drug and tablet formulation, by flow injection analysis with spectrophotometric detection. Talanta 580, 1301–1310. doi: 10.1016/S0039-9140(02)00421-6
Butcher, J. C. (2016). Numerical Methods for Ordinary Differential Equations, 3rd Edn. New Jersey, NJ: John Wiley & Sons.
Buxton, G. V., Greenstock, C. L., Helman, W. P., and Ross, A. B. (1988). Critical review of rate constants for reactions of hydrated electrons, hydrogen atoms and hydroxyl radicals in aqueous solution. J. Phys. Chem. 17, 513–886. doi: 10.1063/1.555805
Chen, Y., Li, H. Y., Lu, W. J., Tu, Y., Zhang, Y. H., Han, W. Q., et al. (2014). Electrochemical degradation of nitrobenzene by anodic oxidation on the constructed TiO2-NTs/SnO2-Sb/PbO2 electrode. Chemosphere 113, 48–55. doi: 10.1016/j.chemosphere.2014.03.122
Comminellis, C. (1994). ElectroCatalysis in the electrochemical conversion/combustion organic pollutants for water treatment. Electrochim. Acta 39, 1857–1862. doi: 10.1016/0013-4686(94)85175-1
Comninellis, C., and Pulgarin, C. (1991). Anodic oxidation of phenol for waste water treatment. J. Appl. Electrochem. 21, 703–708. doi: 10.1007/BF01034049
Crittenden, J. C., Trussell, R. R., Hand, D. W., Howe, K. J., and Tchobanoglous, G. (2012). MWH'S Water Treatment: Principles and Design, 3rd Edn. New Jersey, NJ: John Wiley & Sons.
Dai, Q. Z., Xia, Y. J., Sun, C., Weng, M. L., Chen, J., Wang, J. D., et al. (2014). Electrochemical degradation of levodopa with modified PbO2 electrode: Parameter optimization and degradation mechanism. Chem. Eng. J. 245, 359–366. doi: 10.1016/j.cej.2013.08.036
Dai, Q. Z., Zhou, J. Z., Meng, X. Y. L., Feng, D., Wu, C. Q., and Chen, J. M. (2016). Electrochemical oxidation of cinnamic acid with Mo modified PbO2 lectrode: Electrode characterization, kinetics and degradation pathway. Chem. Eng. J. 289, 239–246. doi: 10.1016/j.cej.2015.12.054
Feng, Y. J., Gui, Y. H., and Logan, B. (2008). Performance of Gd doped Ti-base Sb-SnO2 anodes for electrochemical destruction of phenol. Chemosphere 70, 1629–1636. doi: 10.1016/j.chemosphere.2007.07.083
D. E. Goldberg (ed.). (1989). Genetic Algorithms in Search, Optimization and Machine Learning. Boston, MA: Addison-Wesley Longman Publishing Co. Inc.
Hallman, M. A, Tchao, R., and Tarloff, J. B. (2000). Effect of antioxidants on para-aminophenol-induced toxicity in LLC-PK1 cells. Toxicol. Appl. Pharm. 156, 37–45. doi: 10.1016/S0300-483X(00)00326-7
Harmon, M. R. C., Terneus, K. V., Kiningham, K., and Monica, V. (2005). Time-dependent effect of p-Aminophenol (PAP) toxicity in renal slices and development of oxidative. Toxicol. Appl. Pharm. 209, 86–94. doi: 10.1016/j.taap.2005.03.011
Khodaveisi, J., Dadfarnia, S. A., Shabani, M. H., Moghadam, M. R., and Hormozi-Nezhad, M. R. (2015). Artificial neural network assisted kinetic spectrophotometric technique for simultaneous determination of paracetamol and p-aminophenol in pharmaceutical samples using localized surface plasmon resonance band of silver nanoparticles. Spectrochim. Acta. A 138, 474–480. doi: 10.1016/j.saa.2014.11.094
Li, H., Yu, Q. N., Yang, B., Li, Z. J., and Lei, L. C. (2014). Electrochemical treatment of artificial humidity condensate by large-scale boron doped diamond electrode. Sep.Purif. Technol. 138, 13–20. doi: 10.1016/j.seppur.2014.10.004
Li, Y., Bentzley, C. M., and Tarloffa, J. B. (2004). Comparison of para-aminophenol cyto toxicity in rat renal epithelial cells and hepatocytes. Toxicology 209, 69–76. doi: 10.1016/j.tox.2004.12.008
Mukimin, A., Vistanty, H., and Zen, N. (2015). Oxidation of textile wastewater using cylinder Ti/β-PbO2 electrode in electrocatalytic tube reactor. Chem. Eng. J. 259, 430–437. doi: 10.1016/j.cej.2014.08.020
Ndiritu, J. G., and Daniell, T. M. (2001). An improved genetic algorithm for rainfall-runoff model calibration and function optimization. Math. Comp. Model. Dyn. 33, 695–706. doi: 10.1016/S0895-7177(00)00273-9
Simond, O., Schaller, V., and Comminellis, C. (1996). Theoretical model for the anodic oxidation of oganic on metal oxide electrodes. Electrochim. Acta 42, 13–14. doi: 10.1016/S0013-4686(97)85475-8
Velegraki, T., Balayiannis, G., Diamadopoulos, E., Katsaounis, A., and Mantzavinos, D. (2010). Electrochemical oxidation of benzoic acid in water over boron-doped diamond electrodes: statistical analysis of key operating parameters, kinetic modeling, reaction by-products and ecotoxicity. Chem. Eng. J. 160, 538–548. doi: 10.1016/j.cej.2010.03.065
Wang, L., Cao, J., Cheng, X., Lei, C., Dai, Q., Yang, B., et al. (2019). ZIF-derived carbon nanoarchitecture as a Bi-functional pH-universal electrocatalyst for energy-efficient hydrogen evolution. ACS Sustain. Chem. Eng. 7, 10044–10051. doi: 10.1021/acssuschemeng.9b01315
Weng, M. L., and Pei, J. C. (2016). Electrochemical oxidation of reverse osmosis concentrate using a novel electrode: parameter optimization and kinetics study. Desalination 399, 21–28. doi: 10.1016/j.desal.2016.08.002
Weng, M. L., Zhou, Z. J., and Zhang, Q. (2013). Electrochemical degradation of typical dyeing wastewater in aqueous solution: performance and mechanism. Int. J. Electrochem. Sci. 8, 290–296.
Xia, Y. J., Dai, Q. Z., and Chen, J. M. (2015a). Electrochemical degradation of aspirin using a Ni doped PbO2 electrode. J. Electroanal. Chem. 744, 117–125. doi: 10.1016/j.jelechem.2015.01.021
Xia, Y. J., Dai, Q. Z., Weng, M. L., Peng, Y., Luo, J. M., Meng, X. Y., et al. (2015b). Fabrication and electrochemical treatment application of a novel Al-doped PbO2 electrode with high oxidation capability, oxygen evolution potential and reusability. J. Electrochem. Soc. 162, E258–E262. doi: 10.1149/2.0351508jes
Keywords: electrochemical oxidation, rare earth, doped lead dioxide electrodes, kinetics modeling, mechanism
Citation: Weng M and Yu X (2019) Electrochemical Oxidation of Para-Aminophenol With Rare Earth Doped Lead Dioxide Electrodes: Kinetics Modeling and Mechanism. Front. Chem. 7:382. doi: 10.3389/fchem.2019.00382
Received: 30 August 2018; Accepted: 13 May 2019;
Published: 11 June 2019.
Edited by:
Lifen Liu, Dalian University of Technology (DUT), ChinaReviewed by:
Yuqing Miao, University of Shanghai for Science and Technology, ChinaLihua Qian, Huazhong University of Science and Technology, China
Copyright © 2019 Weng and Yu. This is an open-access article distributed under the terms of the Creative Commons Attribution License (CC BY). The use, distribution or reproduction in other forums is permitted, provided the original author(s) and the copyright owner(s) are credited and that the original publication in this journal is cited, in accordance with accepted academic practice. No use, distribution or reproduction is permitted which does not comply with these terms.
*Correspondence: Mili Weng, wengml@163.com