- 1Normandie Univ, INSA Rouen, UNIROUEN, CNRS, COBRA (UMR 6014), Rouen, France
- 2Institut Universitaire de France, Paris, France
Fluorine-containing molecules are compounds of interest in materials as well as in pharmaceutical and agrochemical industries. Therefore, developments in that research field are tremendous and a special focus was dedicated to the design and the study of emergent fluorinated groups. In particular, the CF2Me residue is attractive as it could be used for example as a bioisostere of the methoxy group. Despite the clear asset that represents this fluorinated moiety and in complement to the traditional approaches used to construct the CF2Me residue from existing functional groups, the quest for direct methodologies for the 1,1-difluoroethylation reaction of molecules has triggered a strong interest from the scientific community. This Mini-review will focus on the recent advances toward the design of reagents and their applications for the direct 1,1-difluoroethylation of various classes of compounds.
Introduction
The design of tools and unprecedented synthetic pathways in organofluorine chemistry is of prime importance to extend the current tool box (Landelle et al., 2013; Liang et al., 2013; Besset et al., 2014, 2016; Egami and Sodeoka, 2014; Merino and Nevado, 2014; Belhomme et al., 2015; Champagne et al., 2015; Ni and Hu, 2016; Lemos et al., 2018; Song et al., 2018). With more than 40% of agrochemicals and 25% of pharmaceuticals having at least one fluorine atom, this research field is very active and has witnessed a strong interest from academic and industry laboratories (Purser et al., 2008; Fujiwara and O'Hagan, 2014; Ilardi et al., 2014; Wang et al., 2014; Gillis et al., 2015; Ni et al., 2015; Meanwell, 2018). Taking benefit from the unique features of the fluorine atom and fluorinated groups, the biological and physical properties of a molecule might be modulated at will (O'Hagan, 2008). Therefore, it appeared as crucial to further develop efficient transformations for the introduction of fluorinated moieties onto complex molecules and to design and study new fluorine-containing moieties. Among these emergent fluorinated groups, a special attention was paid to the 1,1-difluoroethyl group. Present in various compounds of interest, the CF2Me residue appears as a fluorinated bioisostere of the alkoxy ethers. Indeed, the replacement of the oxygen atom by a CF2 residue makes the molecules more metabolically stable and the presence of a CF2Me moiety impacts the spatial geometry (difference of conformational preference of an OMe vs. CF2Me group) although keeping similar electronic and steric properties (Zhou et al., 2013). In addition, the metabolic stability of the molecules might be modulated by replacing a benzylic methylene residue by a CF2 one as it was the case for a urea transporter B (UTB) inhibitor (II), used for edema (Anderson et al., 2012). In addition, these compounds bearing this fluorinated moiety already demonstrated interesting properties, like in the case of edema and malaria treatment, for instance (Coteron et al., 2011; Anderson et al., 2012). Consequently, over the last years, the landscape of this research field has been impacted by key contributions from several research groups, who have pioneered the synthesis and application of original reagents to construct C-CF2Me bonds. The synthesis of CF2Me-containing molecules mainly relied on the fluorination of (1) carbonyl derivatives (or analogs i.e., thiocarbonyl compounds), (2) benzylic positions, (3) alkynes, or (4) alkenes and was well-documented (for selected examples: Markovskij et al., 1973; Middleton, 1975; York et al., 1996; Lal et al., 1999; Reddy et al., 2005; Yamauchi et al., 2008; Umemoto and Singh, 2012; Xia et al., 2013; Ilchenko et al., 2014; Okoromoba et al., 2014; Xu et al., 2014; Ma et al., 2015; Koperniku et al., 2016; Hua et al., 2017; Li et al., 2017; Zhao et al., 2017; Iacono et al., 2018; Tomita et al., 2018). In sharp contrast, the design of new reagents or methodologies for the direct incorporation of this fluorinated moiety onto molecules is still underdeveloped. Recently Li, Dong et al. reviewed the synthesis of (1,1-difluoroethyl)arenes based on the construction of the CF2Me motif and few examples describing its direct incorporation onto arenes were reported (Li et al., 2018).
The aim of this mini-review is to showcase and discuss the recent advances made on novel synthetic strategies for the direct introduction of the emergent CF2Me group onto various classes of molecules (arenes, aliphatic, and carbonyl compounds). Therefore, in this review, we will highlight the new technological solutions based on the design of original reagents and methodologies to build up C-CF2Me bonds. Note that the approaches employed to construct the CF2Me group will not be discussed. Taking these considerations in mind, a first strategy to access to CF2Me-containing molecules relies on the introduction of the CF2Me group by using nucleophilic reagents, whereas a second approach deals with the construction of a C-CF2Me bond according to a radical pathway.
1,1-Difluoroethylation of Molecules Using a Nucleophilic CF2Me-Containing Reagent
Transition Metal-Free Reactions to Access CF2Me-Containing Molecules
In this section, key advances for the direct 1,1-difluoroethylation of carbonyl derivatives via a transition metal-free process will be depicted.
TESCF2Me and TMSCF2Me as CF2Me Sources
In their quest for new fluorine-containing reagents, Prakash et al. (Mogi et al., 2007) reported the synthesis of a 1,1-difluoroethylated reagent, the TESCF2Me (Scheme 1). The latter resulted from the reaction between TESCl and (1,1-difluoroethyl)phenylsulfone 1 in the presence of magnesium metal in 35% yield on a gram scale. The precursor 1 was itself prepared in three steps from thiophenol (Langlois, 1988; Mogi et al., 2007). Note that the nature of the solvents (THF/HMPA, 1:1) was crucial to ensure the full conversion of the sulfone 1 into the desired TESCF2Me reagent. When this reagent was reacted with aromatic aldehydes 2, the corresponding 1,1-difluoroethylated secondary alcohols 3 were obtained in moderate to good 19F NMR yields (50–77%). The reaction was tolerant to electron-donating groups (3b,c) and halogen (3d). The reaction was also sensitive to steric hindrance since when the reaction was conducted with a sterically hindered aldehyde or ketone, only traces of products were obtained. Finally, an enolizable aldehyde and ketone and an α,β-unsaturated aldehyde were not suitable substrates in that transformation and constituted the main limitations.
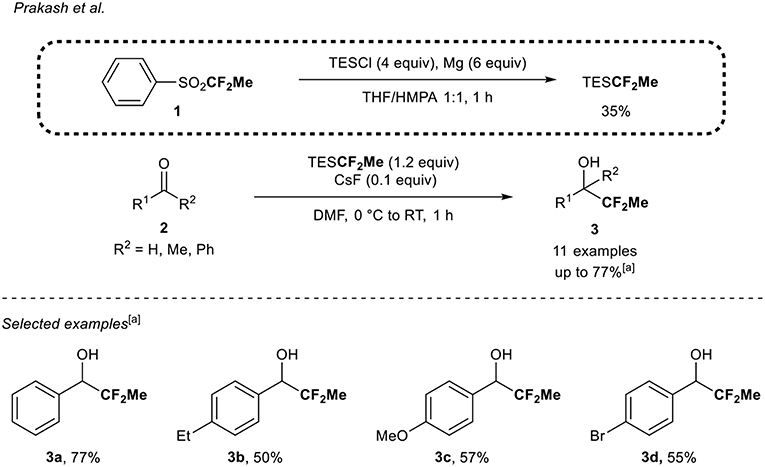
Scheme 1. 1,1-Difluoroethylation of carbonyl derivatives 2 with the TESCF2Me reagent. [a] 1H NMR yield of the crude reaction mixture.
In the course of their studies regarding the difluoromethylation of aldehydes, ketones, and N-tert-butylsulfinimines using the nucleophilic TMSCF2H source, Hu and co-workers (Zhao et al., 2011) reported the 1,1-difluoroethylation of carbonyl derivatives 4, 6, and 8 (three examples, up to 92% yield) with the corresponding (1,1-difluoroethyl)trimethylsilane (TMSCF2Me) under basic conditions (Scheme 2).
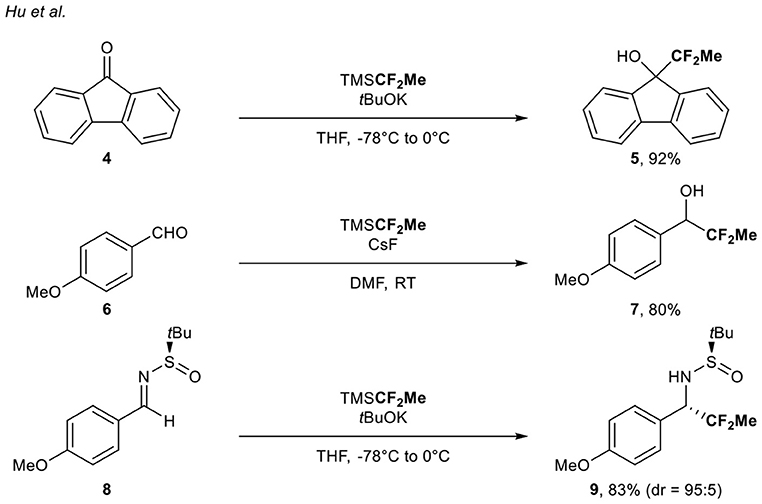
Scheme 2. (1,1-Difluoroethyl)trimethylsilane as CF2Me source for the functionalization of carbonyl derivatives.
Use of a CF2Me-Substituted Phosphonium Salt
About 10 years later, Xiao and co-workers reported a new methodology to access CF2Me-containing molecules by means of a nucleophilic source (Deng et al., 2016). As an alternative to the powerful TESCF2Me reagent, which has a low boiling point and which required a tedious multi-step synthesis [four steps from thiophenol (Mogi et al., 2007)], Lin and Xiao designed a nucleophilic reagent based on a phosphonium salt. The shelf-stable 1,1-difluoroethyl phosphonium salt was prepared in three-steps from the commercially available and inexpensive PPh3 and EtBr, in a 29% overall yield (Scheme 3). After the formation of the ethyl phosphonium salt, a subsequent two-step fluorination sequence with NFSI, allowed the formation of the desired reagent on a gram-scale.
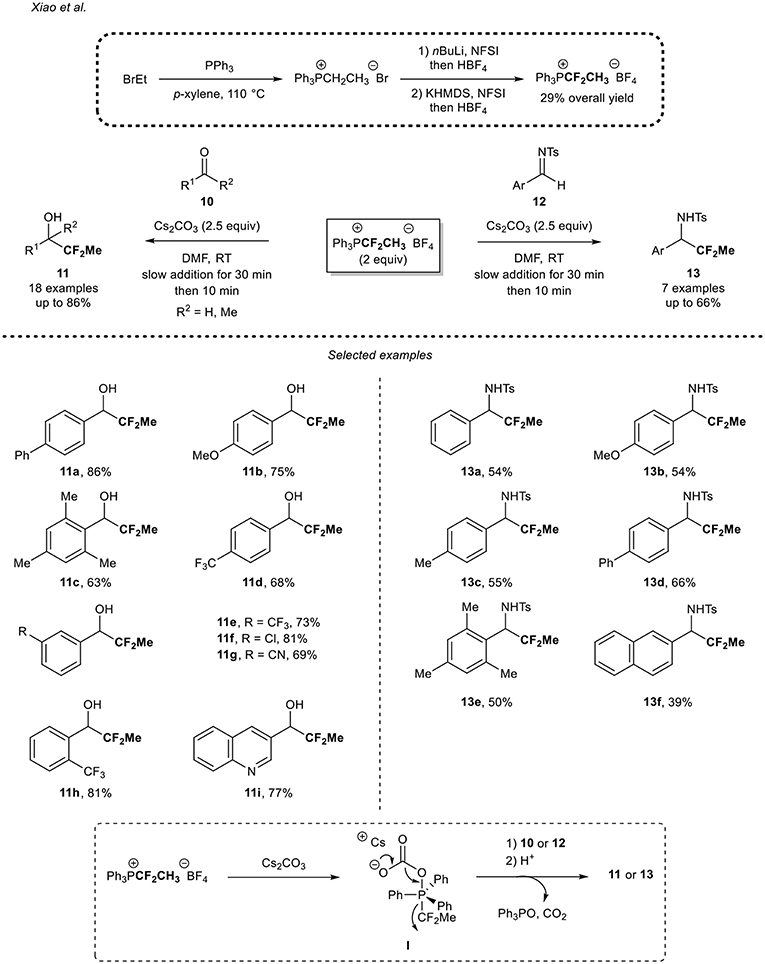
Scheme 3. Application of the 1,1-difluoroethyl phosphonium salt for the 1,1-difluoroethylation reaction of carbonyl derivatives.
This reagent was then successfully applied for the 1,1-difluoroethylation of (het)aromatic aldehydes and the 4-phenyl acetophenone 10. Electron-rich aryl aldehydes were smoothly converted into the desired secondary alcohols in moderate to high yields, as illustrated with the para-phenyl derivative 11a and the para-methoxy derivative 11b. A slight decrease of yield was observed when the reaction was performed from the sterically hindered electron-rich aldehyde 10c. Aryl aldehydes bearing an electron-withdrawing substituent (i.e., CF3, CN, halogens) were also suitable substrates 10d-h for the reaction. In addition, the substitution pattern had no impact on the reaction outcome since the para-, meta-, and ortho-CF3 substituted aryl alcohols 11d,e,h were obtained in good yields (68, 73, and 81%, respectively). Note that the 3-quinolinecarboxaldehyde 10i was functionalized in a good yield. However, only traces of the desired 1,1-difluoroethylated product were detected when starting from an aliphatic aldehyde and a lower 19F NMR yield (21%) was observed when the reaction was carried out with 4-phenyl acetophenone. To further demonstrate the potential of the methodology, the authors applied their methodology to the functionalization of the N-tosyl imines 12a-f and the corresponding products 13 were obtained in moderate to good yields (39–66%). It is worth mentioning that due to the lower reactivity of N-tosyl imines 12 compared to aldehydes 10, 1,1-difluoroethylated amines 13 were obtained in lower yields than 1,1-difluoroethylated alcohols 11. A plausible mechanism was suggested by the authors. After reaction of the Cs2CO3 promoter with the 1,1-difluoroethyl phosphonium salt, the intermediate I would be formed. This latter might then undergo a decarboxylation reaction to afford Ph3PO and release a nucleophilic CF2Me residue from the cleavage of the P–CF2 bond. Then, this species might react with 10 or 12 to afford the desired 1,1-difluoroethyated compound 11 or 13.
Transition Metal-Promoted Reactions to Access CF2Me-Containing Derivatives
As a complementary strategy, the development of transition metal promoted 1,1-difluoroethylation of molecules offered an efficient synthetic pathway to build up C-CF2Me bonds.
Access to 1,1-Difluoroethylated Derivatives From Organozinc Reagents
The group of Dilman (Zemtsov et al., 2014) depicted a two-step process for the synthesis of 1,1-difluoroethylated derivatives, thanks to the in situ formation of the MeCF2ZnX species. The reaction of the difluorocarbene with MeZnI formed the desired organozinc reagent. The latter was engaged in a copper-catalyzed allylation reaction and allowed the synthesis of the corresponding CF2-containing molecules. With this methodology, a single example of a CF2Me-containing molecule 14 was prepared in 66% yield (Scheme 4).
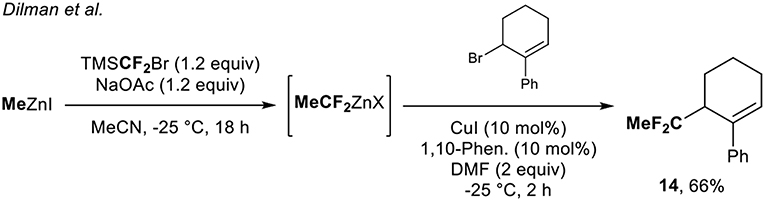
Scheme 4. Access to the CF2Me-containing molecule 14 via a two-step process. 1,10-Phen. = 1,10-Phenanthroline.
Copper-Mediated 1,1-Difluoroethylation Reaction Using TMSCF2Me as a Fluorinated Source
In 2016, Hu and co-workers (Li et al., 2016) described the copper-mediated 1,1-difluoroethylation reaction of diaryliodoniums triflate 15 using CuCF2Me (Scheme 5). The CuCF2Me reagent was in situ generated from the reaction of CuCl with TMSCF2Me (Mogi et al., 2007) in the presence of tBuOK. Then, the synthesis of (1,1-difluoroethyl)arenes 16 was carried out. In presence of 1.5 equivalents of the in-situ generated CuCF2Me and 0.5 equivalents of Et3N·3HF, as a crucial additive, a panel of electron-rich diaryliodoniums salts were smoothly converted into the desired CF2Me-containing arenes 16a,b,g in good yields. The reaction conditions were also tolerant toward diaryliodoniums salts bearing an electron-withdrawing group such as halogen, ketone, ester, aldehyde, and nitro groups to furnish the 1,1-difluoroethylated arenes 16c-f,h in moderate to high yields. Sterically hindered diaryliodonium salts 15i and 15j were also suitable substrates under these reaction conditions and furnished the desired products 16i-j in good yields. The potential of this strategy was further demonstrated by the 1,1-difluoroethylation of analogs of relevant compounds such as estrone 15k and the anti-inflammatory drug naproxen 15l. Concerning the mechanism, the authors ruled out a radical pathway and proposed the following mechanism pathway: formation of the intermediate Cu(III) species I, which would result from the oxidative addition of the CuCF2Me species with the diaryliodonium salt 15, followed by a final reductive elimination step to furnish the expected product 16.
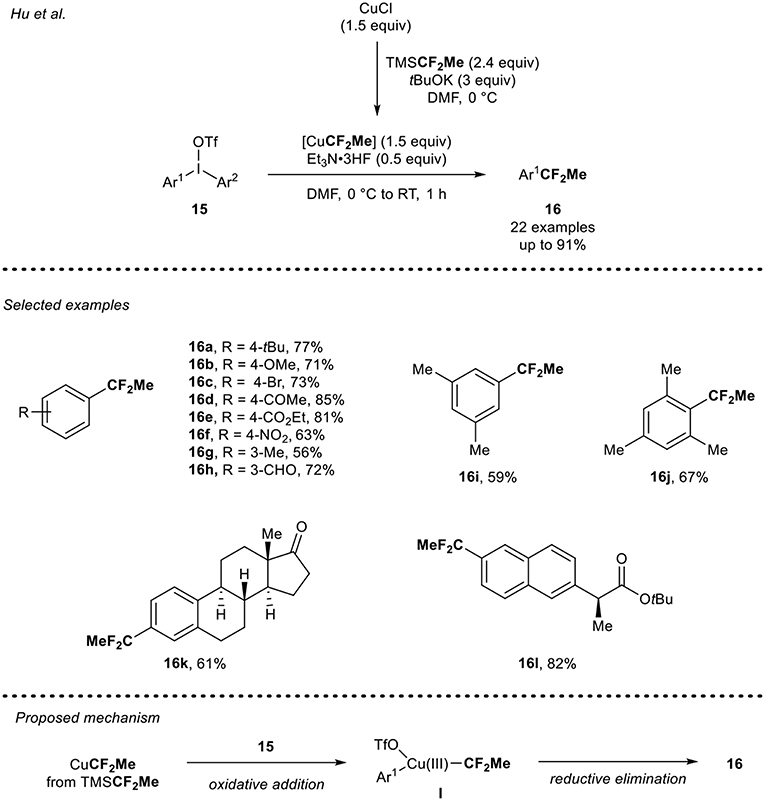
Scheme 5. 1,1-Difluoroethylation of diaryliodonium salts 15 using the in situ generated CuCF2Me reagent.
Cobalt-Catalyzed 1,1-Difluoroethylation Reaction of Aryl Grignard Reagent Using BrCF2Me
A complementary synthetic route toward the synthesis of 1,1-difluoroethylated arenes was reported by Yamakawa and Ohtsuka (Ohtsuka and Yamakawa, 2016). The cobalt-catalyzed 1,1-difluoroethylation of aryl Grignard derivatives 17a-g using BrCF2Me was developed (Scheme 6). Various aryl Grignard derivatives were functionalized using two sets of reaction conditions. Note that the nature of the ligand and the solvent played an important role in each catalytic system. Aryl Grignard reagents bearing electron-donating and electron-withdrawing groups at the para position were functionalized leading to the corresponding products 18 in low to good yields. It turned out that the substitution pattern had an impact on the reaction outcome since the derivative 18g bearing a methoxy group at the ortho-position was obtained in a lower yield compared to the compound 18b and 18f with both reaction conditions (A and B). Note that, except for product 18c, the isolated yields were rather lower than the ones determined by 19F NMR, presumably due to volatility issue of the fluorinated products.
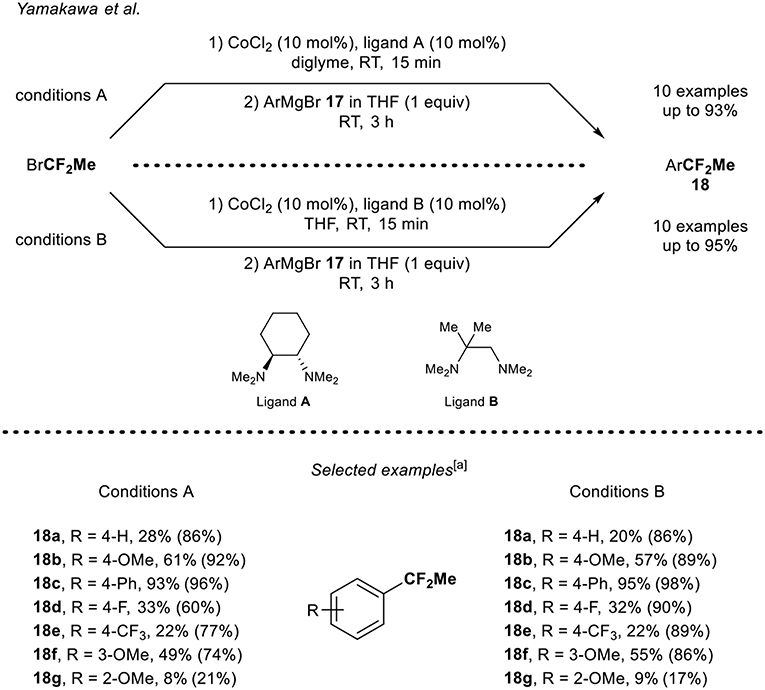
Scheme 6. Cobalt-catalyzed 1,1-difluoroethylation of ArMgBr 17 with BrCF2Me. [a] Yields shown in parenthesis were determined by 19F NMR.
Direct Introduction of the CF2Me Moiety via a Radical Process
In this section, the recent breakthroughs for the direct and selective incorporation of the CF2Me residue onto molecules via a radical process will be discussed. Note that this strategy was mainly used to access 1,1-difluoroethyl-containing heteroarenes.
DFES-Na as the 1,1-Difluoroethyl Source
In 2013, a pioneer work was reported by the group of Baran (Zhou et al., 2013). They designed the synthesis of the sodium difluoroethylsulfinate (DFES-Na) and investigated its application to the functionalization of various heterocycles. The DFES-Na reagent was obtained in two steps from the Hu's reagent and was prepared on a large scale (>100 g). Under oxidative and robust conditions (TBHP, water as co-solvent under air), various classes of heteroarenes 19 were functionalized (21 examples) in the presence of ZnCl2 and TsOH.H2O. The transformation turned out to be functional group tolerant and moderate to good selectivity was observed. In addition, the authors demonstrated the possible direct radical functionalization of Michael acceptors 21 and thiol derivatives 22 (Scheme 7).
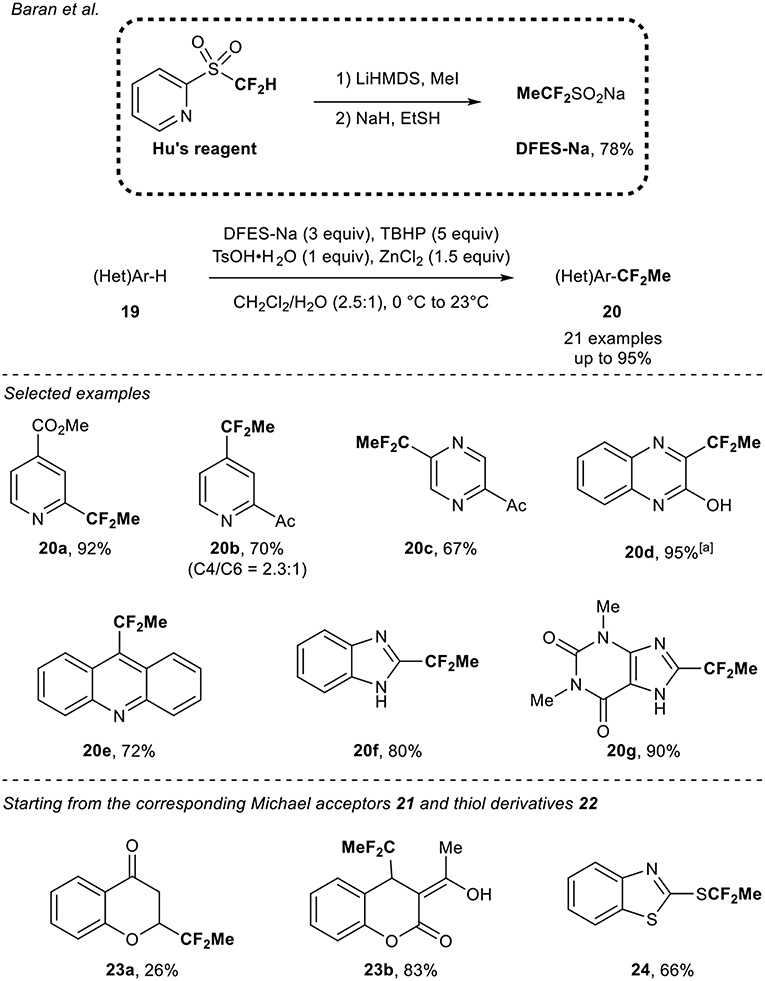
Scheme 7. 1,1-Difluoroethylation of heteroarenes and extension to other classes of compounds with the DFES-Na reagent. [a] After 10 h using DFES-Na (2 equiv) and ZnCl2 (1 equiv).
This reagent was then used by the group of Vincent (Ryzhakov et al., 2017) for the direct introduction of the CF2Me residue on protected indoles 25. The reaction led to the corresponding fluorinated spirocyclic indolines 26 under oxidative conditions (2 examples, Scheme 8).
CF2Me-Containing Sulfones as the 1,1-Difluoroethyl Source
In 2015, the group of Dolbier (Zhang et al., 2015) reported the synthesis of fluorinated phenanthridines 28 under visible light photoredox catalysis. Starting from biphenyl isocyanides 27, the 1,1-difluoroalkylation occurred using an Ir photocatalyst via a tandem addition/cyclization/oxidation sequence. Although the study mainly focused on the difluoromethylation reaction, the introduction of the 1,1-difluoroethyl radical was readily performed using the MeCF2SO2Cl reagent as precursor of the CF2Me radical (Scheme 9). Using this reaction manifold, four CF2Me-containing phenanthridines were synthesized in good yields (up to 83%). The following mechanism was proposed by the authors: first, the generation of the radical CF2Me from the reduction of the MeCF2SO2Cl reagent with the excited Ir catalyst followed by its addition on the isocyanides 27. Then a cyclization would lead to the corresponding radical A, which would be oxidized into the species B, regenerating the Ir-catalyst. A final deprotonation of B would yield to the expected products 28.
A complementary approach was reported by Hu and co-workers in 2016 (Rong et al., 2016). They developed a new class of fluoroalkylation reagents, which enabled the formation of Rf radical using visible light photoredox catalysis. Using fluoroalkylated heteroaryl sulfones, various isocyanides were functionalized with several fluorinated motifs (Rf = CH2F, CF2H, CF2Me, CF2Ph, CF3, and CF2COPh). Among them, the Ru-catalyzed 1,1-difluoroethylation of two biphenyl isocyanides 30 using the reagent 29 was reported (Scheme 10).
In 2017, the group of Dolbier (Zhang et al., 2017) developed a radical fluoroalkylation of unactivated alkenes under photoredox catalysis to build up fluorinated tetralin derivatives. In that context, a single example of the direct introduction of the CF2Me group to build up the corresponding carbocyclic compound under Ir catalysis was depicted. The expected product 33 was obtained in 64% yield using the MeCF2SO2Cl reagent (Scheme 11).
Summary and Outlook
In this Mini-review, we discussed the recent advances made for the direct and selective introduction of the valuable CF2Me group. As alternative to the traditional synthetic routes, these direct approaches represented efficient and new retrosynthetic pathways. In this context, technological solutions based on the design of new tools (original strategies and reagents) for the 1,1-difluoroethylation of several classes of compounds were designed. Two strategies were employed based either on the use of nucleophilic reagents or precursors of CF2Me radical sources. In the former case, transition metal free approaches as well as transition metal-mediated or -catalyzed transformations were depicted. The complementary synthetic pathway relied on the use of a radical process to access CF2Me-containing molecules using carefully designed reagents. Beyond these impressive achievements, further developments for the direct introduction of the CF2Me residue, especially for the late-stage functionalization of complex (bioactive) molecules will be of prime importance to enlarge the existing toolbox. Thanks to the pivotal importance of the organofluorine chemistry in various research fields, we strongly believe that this Mini-review will offer new perspectives to further study and apply this original fluorinated moiety.
Author Contributions
EC and TB collected the literature data related to this review article. EC, TP, PJ, and XP wrote sections of the manuscript. TB wrote the first draft of the manuscript. All authors contributed to the final version of the manuscript and approved the submitted version.
Funding
This work was partially supported by INSA Rouen, Rouen University, CNRS, EFRD, LabexSynOrg (ANR-11-LABX-0029), Région Normandie (Crunch Network) and the IUF (Institut Universitaire de France). EC thanks the Labex SynOrg (ANR-11-LABX-0029) for a doctoral fellowship. TP thanks the IUF for support. TB thanks the European Research Council (ERC) under the European Union's Horizon 2020 research and innovation programme (grant agreement no. 758710).
Conflict of Interest Statement
The authors declare that the research was conducted in the absence of any commercial or financial relationships that could be construed as a potential conflict of interest.
References
Anderson, M. O., Zhang, J., Liu, Y., Yao, C., Phuan, P.-W., and Verkman, A. S. (2012). Nanomolar Potency and Metabolically Stable Inhibitors of Kidney Urea Transporter UT-B. J. Med. Chem. 55, 5942–5950. doi: 10.1021/jm300491y
Belhomme, M.-C., Besset, T., Poisson, T., and Pannecoucke, X. (2015). Recent progress toward the introduction of functionalized difluoromethylated building blocks onto C(sp2) and C(sp) centers. Chem. Eur. J. 21, 12836–12865. doi: 10.1002/chem.201501475
Besset, T., Jubault, P., Pannecoucke, X., and Poisson, T. (2016). New entries toward the synthesis of OCF3-containing molecules. Org. Chem. Front. 3, 1004–1010. doi: 10.1039/C6QO00164E
Besset, T., Poisson, T., and Pannecoucke, X. (2014). Recent progress in direct introduction of fluorinated groups on alkenes and alkynes by means of C-H bond functionalization. Chem. Eur. J. 20, 16830–16845. doi: 10.1002/chem.201404537
Champagne, P. A., Desroches, J., Hamel, J.-D., Vandamme, M., and Paquin, J.-F. (2015). Monofluorination of organic compounds: 10 years of innovation. Chem. Rev. 115, 9073–9174. doi: 10.1021/cr500706a
Coteron, J. M., Marco, M., Esquivias, J., Deng, X., White, K. L., White, J., et al. (2011). Structure-Guided Lead Optimization of Triazolopyrimidine-Ring Substituents Identifies Potent Plasmodium falciparum Dihydroorotate Dehydrogenase Inhibitors with Clinical Candidate Potential. J. Med. Chem. 54, 5540–5561. doi: 10.1021/jm200592f
Deng, Z., Liu, C., Zeng, X.-L., Lin, J.-H., and Xiao, J.-C. (2016). Nucleophilic 1,1-Difluoroethylation with Fluorinated Phosphonium Salt. J. Org. Chem. 81, 12084–12090. doi: 10.1021/acs.joc.6b02723
Egami, H., and Sodeoka, M. (2014). Trifluoromethylation of alkenes with concomitant introduction of additional functional groups. Angew. Chem. Int. Ed. 53, 8294–8308. doi: 10.1002/anie.201309260
Fujiwara, T., and O'Hagan, D. (2014). Successful fluorine-containing herbicide agrochemicals. J. Fluorine Chem. 167, 16–29. doi: 10.1016/j.jfluchem.2014.06.014
Gillis, E. P., Eastman, K. J., Hill, M. D., Donnelly, D. J., and Meanwell, N. A. (2015). Applications of Fluorine in Medicinal Chemistry. J. Med. Chem. 58, 8315–8359. doi: 10.1021/acs.jmedchem.5b00258
Hua, A. M., Mai, D. N., Martinez, R., and Baxter, R. D. (2017). Radical C–H fluorination using unprotected amino acids as radical precursors. Org. Lett. 19, 2949–2952. doi: 10.1021/acs.orglett.7b01188
Iacono, C. E., Stephens, T. C., Rajan, T. S., and Pattison, G. (2018). A coupling approach for the generation of α,α-bis(enolate) equivalents: regioselective synthesis of gem-difunctionalized ketones. J. Am. Chem. Soc. 140, 2036–2040. doi: 10.1021/jacs.7b12941
Ilardi, E. A., Vitaku, E., and Njardarson, J. T. (2014). Data-Mining for Sulfur and Fluorine: An Evaluation of Pharmaceuticals To Reveal Opportunities for Drug Design and Discovery. J. Med. Chem. 57, 2832–2842. doi: 10.1021/jm401375q
Ilchenko, N. O., Tasch, B. O. A., and Szab,ó, K. J. (2014). Mild silver-mediated geminal difluorination of styrenes using an air and moisture-stable fluoroiodane reagent. Angew. Chem., Int. Ed. 53, 12897–12901. doi: 10.1002/anie.201408812
Koperniku, A., Liu, H., and Hurley, P. B. (2016). Mono- and Difluorination of Benzylic Carbon Atoms. Eur. J. Org. Chem., 871–886. doi: 10.1002/ejoc.201501329
Lal, G. S., Pez, G. P, Pesaresi, R. J., Prozonic, F. M., and Cheng, H. (1999). Bis(2-methoxyethyl)aminosulfur trifluoride: a new broad-spectrum deoxofluorinating agent with enhanced thermal stability. J. Org. Chem. 64, 7048–7054. doi: 10.1021/jo990566
Landelle, G., Panossian, A., Pazenok, S., Vors, J.-P., and Leroux, F. R. (2013). Recent advances in transition metal-catalyzed Csp2-monofluoro-, difluoro-, perfluoromethylation and trifluoromethylthiolation. Beilstein J. Org. Chem. 9, 2476–2536. doi: 10.3762/bjoc.9.287
Langlois, B. R. (1988). Improvement of the synthesis of aryl difluoromethyl ethers and thioethers by using a solid-liquid phase-transfer technique. J. Fluorine Chem. 41, 247–261. doi: 10.1016/S0022-1139(00)81548-1
Lemos, A., Lemaire, C., and Luxen, A. (2018). Progress in difluoroalkylation of organic substrates by visible light photoredox catalysis. Adv. Synth. Catal. doi: 10.1002/adsc.201801121
Li, X., Liu, J., Li, X., Liu, H., Liu, H., Li, Y., et al. (2018). Recent advance in the synthesis of (1,1-difluoroethyl)arenes. J. Fluorine Chem. 216, 102–106. doi: 10.1016/j.jfluchem.2018.10.011
Li, X., Zhao, J., Wang, Y., Rong, J., Hu, M., Chen, D., et al. (2016). Copper-Mediated Aromatic 1,1-Difluoroethylation with (1,1-Difluoroethyl)trimethylsilane (TMSCF2CH3). Chem. Asian J. 11, 1789–1792. doi: 10.1002/asia.201600577
Li, Y.-L., Li, J., and Deng, J. (2017). Practical access to difluoromethyl ketones via straightforward decarboxylative difluorination of β-ketoacids. Adv. Synth. Catal. 359, 1407–1412. doi: 10.1002/adsc.201601315
Liang, T., Neumann, C. N., and Ritter, T. (2013). Introduction of Fluorine and Fluorine-Containing Functional Groups. Angew. Chem. Int. Ed. 52, 8214–8264. doi: 10.1002/anie.201206566
Ma, J.-J., Yi, W.-B., Lu, G.-P., and Cai, C. (2015). Transition-metal-free C–H oxidative activation: persulfate-promoted selective benzylic mono- and difluorination. Org. Biomol. Chem. 13, 2890–2894. doi: 10.1039/C4OB02418D
Markovskij, L. N., Pashinnik, V. E., and Kirsanov, A. V. (1973). Application of Dialkylaminosulfur Trifluorides in the Synthesis of Fluoroorganic Compounds. Synthesis, 787–789. doi: 10.1055/s-1973-22302
Meanwell, N. A. (2018). Fluorine and fluorinated motifs in the design and application of bioisosteres for drug design. J. Med. Chem. 61, 5822–5880. doi: 10.1021/acs.jmedchem.7b01788
Merino, E., and Nevado, C. (2014). Addition of CF3 across unsaturated moieties: a powerful functionalization tool. Chem. Soc. Rev. 43, 6598–6608. doi: 10.1039/C4CS00025K
Middleton, W. J. (1975). New fluorinating reagents. Dialkylaminosulfur fluorides. J. Org. Chem. 40, 574–578. doi: 10.1021/jo00893a007
Mogi, R., Morisaki, K., Hu, J., Surya Prakash, G. K., and Olah, G. A. (2007). Synthesis of 1,1-difluoroethylsilanes and their application for the introduction of the 1,1-difluoroethyl group. J. Fluorine Chem. 128, 1098–1103. doi: 10.1016/j.jfluchem.2007.03.013
Ni, C., and Hu, J. (2016). The unique fluorine effects in organic reactions: recent facts and insights into fluoroalkylations. Chem. Soc. Rev. 45, 5441–5454. doi: 10.1039/C6CS00351F
Ni, C., Hu, M., and Hu, J. (2015). Good partnership between sulfur and fluorine: sulfur-based fluorination and fluoroalkylation reagents for organic synthesis. Chem. Rev. 115, 765–825. doi: 10.1021/cr5002386
O'Hagan, D. (2008). Understanding organofluorine chemistry. An introduction to the C–F bond. Chem. Soc. Rev. 37, 308–319. doi: 10.1039/B711844A
Ohtsuka, Y., and Yamakawa, T. (2016). Cobalt/diamine-catalyzed 1,1-difluoroethylation and 2,2,2-trifluoroethylation of aryl Grignard reagents with corresponding fluoroalkyl halides. J. Fluorine Chem. 185, 96–102. doi: 10.1016/j.jfluchem.2016.03.007
Okoromoba, O. E., Han, J., Hammond, G. B., and Xu, B. (2014). Designer HF-based fluorination reagent: highly regioselective synthesis of fluoroalkenes and gem-difluoromethylene compounds from alkynes. J. Am. Chem. Soc. 136, 14381–14384. doi: 10.1021/ja508369z
Purser, S., Moore, P. R., Swallow, S., and Gouverneur, V. (2008). Fluorine in medicinal chemistry. Chem. Soc. Rev., 2008, 37, 320–330. doi: 10.1039/B610213C
Reddy, V. P., Alleti, R., Perambuduru, M. K., Welz-Biermann, U., Buchholz, H., and Prakash, G. K. S. (2005). gem-Difluorination of 2,2-diaryl-1,3-dithiolanes by Selectfluor® and pyridinium polyhydrogen fluoride. Chem. Commun. 654–656. doi: 10.1039/B414254C
Rong, J., Deng, L., Tan, P., Ni, C., Gu, Y., and Hu, J. (2016). Radical fluoroalkylation of isocyanides with fluorinated sulfones by visible-light photoredox catalysis. Angew. Chem. Int. Ed. 55, 2743–2747. doi: 10.1002/anie.201510533
Ryzhakov, D., Jarret, M., Guillot, R., Kouklovsky, C., and Vincent, G. (2017). Radical-mediated dearomatization of indoles with sulfinate reagents for the synthesis of fluorinated spirocyclic indolines. Org. Lett. 19, 6336–6339. doi: 10.1021/acs.orglett.7b03155
Song, H.-X., Han, Q.-Y., Zhao, C.-L., and Zhang, C.-P. (2018). Fluoroalkylation reactions in aqueous media: a review. Green Chem. 20, 1662–1731. doi: 10.1039/C8GC00078F
Tomita, R., Al-Maharik, N., Rodil, A., Bühl, M., and O'Hagan, D. (2018). Synthesis of aryl α,α-difluoroethyl thioethers a novel structure motif in organic chemistry, and extending to aryl α,α-difluoro oxyethers. Org. Biomol. Chem. 16, 1113–1117. doi: 10.1039/C7OB02987J
Umemoto, T., and Singh, R. P. (2012). Arylsulfur chlorotetrafluorides as useful fluorinating agents: Deoxo- and dethioxo-fluorinations. J. Fluorine Chem. 140, 17–27. doi: 10.1016/j.jfluchem.2012.03.008
Wang, J., Sánchez-Rosell,ó, M., Aceña, J. L., del Pozo, C., Sorochinsky, A. E., Fustero, S., et al. (2014). Fluorine in Pharmaceutical Industry: Fluorine-Containing Drugs Introduced to the Market in the Last Decade (2001–2011). Chem. Rev. 114, 2432–2506. doi: 10.1021/cr4002879
Xia, J.-B., Zhu, C., and Chen, C. (2013). Visible Light-Promoted Metal-Free C–H Activation: Diarylketone-Catalyzed Selective Benzylic Mono- and Difluorination. J. Am. Chem. Soc. 135, 17494–17500. doi: 10.1021/ja410815u
Xu, P., Guo, S., Wang, L., and Tang, P. (2014). Silver-Catalyzed Oxidative Activation of Benzylic C-H Bonds for the Synthesis of Difluoromethylated Arenes. Angew. Chem., Int. Ed. 53, 5955–5958. doi: 10.1002/anie.201400225
Yamauchi, Y., Fukuhara, T., Hara, S., and Senkobu, H. (2008). Electrochemical carboxylation of α,α-difluorotoluene derivatives and its application to the synthesis of α-fluorinated nonsteroidal anti-inflammatory drugs. Synlett 438–442. doi: 10.1055/s-2008-1032069
York, C., Surya Prakash, G. K., and Olah, G. A. (1996). Desulfurative fluorination using nitrosonium tetrafluoroborate and pyridinium poly(hydrogen fluoride). Tetrahedron 52, 9–14. doi: 10.1016/0040-4020(95)00864-5
Zemtsov, A. A., Kondratyev, N. S., Levin, V. V., Struchkova, M. I., and Dilman, A. D. (2014). Copper-Catalyzed Allylation of α,α-Difluoro-Substituted Organozinc Reagents. J. Org. Chem. 79, 818–822. doi: 10.1021/jo4024705
Zhang, Z., Martinez, H., and Dolbier, W. R. (2017). Photoredox catalyzed intramolecular fluoroalkylarylation of unactivated alkenes. J. Org. Chem. 82, 2589–2598. doi: 10.1021/acs.joc.6b03012
Zhang, Z., Tang, X., and Dolbier, W. R. (2015). Photoredox-catalyzed tandem insertion/cyclization reactions of difluoromethyl and 1,1-difluoroalkyl radicals with biphenyl isocyanides. Org. Lett. 17, 4401–4403. doi: 10.1021/acs.orglett.5b02061
Zhao, Y., Huang, W., Zheng, J., and Hu, J. (2011). Efficient and direct nucleophilic difluoromethylation of carbonyl compounds and imines with Me3SiCF2H at ambient or low temperature. Org. Lett. 13, 5342–5345. doi: 10.1021/ol202208b
Zhao, Z., Racicot, L., and Murphy, G. K. (2017). Fluorinative rearrangements of substituted phenylallenes mediated by (difluoroiodo)toluene: synthesis of α-(difluoromethyl)styrenes. Angew. Chem., Int. Ed. 56, 11620–11623. doi: 10.1002/anie.201706798
Keywords: organofluorine chemistry, synthetic methodology, emergent fluorinated groups, CF2Me-containing reagent, C-CF2Me bond formation
Citation: Carbonnel E, Poisson T, Jubault P, Pannecoucke X and Besset T (2019) Recent Advances for the Direct Introduction of the CF2Me Moiety. Front. Chem. 7:111. doi: 10.3389/fchem.2019.00111
Received: 21 January 2019; Accepted: 12 February 2019;
Published: 04 March 2019.
Edited by:
Gwilherm Evano, Free University of Brussels, BelgiumReviewed by:
Anis Tlili, UMR5246 Institut de Chimie et Biochimie Moléculaires et Supramoléculaires (ICBMS), FranceGavin Chit Tsui, The Chinese University of Hong Kong, China
Nicolas Blanchard, UMR7042 Laboratoire d'Innovation Moléculaire et Applications (LIMA), France
Copyright © 2019 Carbonnel, Poisson, Jubault, Pannecoucke and Besset. This is an open-access article distributed under the terms of the Creative Commons Attribution License (CC BY). The use, distribution or reproduction in other forums is permitted, provided the original author(s) and the copyright owner(s) are credited and that the original publication in this journal is cited, in accordance with accepted academic practice. No use, distribution or reproduction is permitted which does not comply with these terms.
*Correspondence: Tatiana Besset, tatiana.besset@insa-rouen.fr