- 1School of Chemistry and Physics, University of KwaZulu-Natal, Durban, South Africa
- 2KRISP, College of Health Sciences, University of KwaZulu-Natal, Durban, South Africa
- 3School of Chemical Engineering, University of KwaZulu-Natal, Durban, South Africa
- 4Department of Organic Chemistry, CIBER-BBN (Networking Centre on Bioengineering, Biomaterials and Nanomedicine), University of Barcelona, Barcelona, Spain
Linkers play major roles in conjugation chemistry toward the advancement of drug discovery. Two different series of fluorinated linkers were introduced to the backbone of a model peptide using solid phase peptide synthesis. These fluorinated linkers have the potential to conjugate two asymmetrical groups. This has not been done using other fluorinated linkers. This study deals with application of linkers with S, N, and O terminals and reports on the investigation of their chemoselectivity and activity for branching peptide backbones using a chosen model peptide. These fluorinated linkers have unique properties that will make it possible for a large diversity of bioconjugated chemicals for different bioapplications to be designed and synthesized.
Introduction
Conjugation of molecules is a chemical tool with increasing interest in several scientific areas, such as pharmaceuticals or materials. Several techniques have been reported in the literature, but many of them have drawbacks associated with chemoselectivity, tunability, and/or synthetic practicality (Schilling et al., 2011). On the other hand, the presence of fluorine in a molecule is key for fine-tuning its properties. The high electronegativity of fluorine has a number of obvious advantages leading to polarization and imparting a less covalent and more electrostatic character to the C–F bond. This leads to a relatively large dipole, which interacts with other dipoles in its vicinity, resulting in a conformational change (O'Hagan, 2008). Furthermore, the presence of fluorine in the backbone of an organic compound results in some changes such as omniphobicity/lipophilicity and electrostatic interactions that can dramatically influence its chemical reactivity (O'Hagan, 2008; Wang et al., 2014). Furthermore, one of the important effects of fluorination is the alteration of acidity and basicity of the parent compounds (Kobzev et al., 1989; van Niel et al., 1999; Rowley et al., 2001; Morgenthaler et al., 2007). In the pharmaceutical field, this can intensely influence binding affinity, pharmacokinetic properties, and bioavailability of a given drug candidate. More importantly, it must be emphasized that fluorine provides significant impact in pharmaceuticals in general, not only on fluorinated drugs but also in various health care products. Fluorine scanning is currently a routine approach for the development of novel fluorinated drug candidates. It is quite remarkable that three out of the five top-selling pharmaceuticals contain a fluorine atom in its structure (Wang et al., 2014).
Pentelute and co-workers have extensively studied the use of perfluororoaryl linkers for different applications in the peptide field, such as macrocyclization and/or peptide stapling as well as site-selective bioconjugation (Spokoyny et al., 2013; Zhang et al., 2013, 2014, 2016; Zou et al., 2014; Dai et al., 2016; Lautrette et al., 2016; Lühmann et al., 2016; Evans and Pentelute, 2018). Scheme 1A shows some examples of fluorine containing linkers such as perfluorobenzene (1), perfluorobiphenyl (2), and other fluorinated linkers that have been applied and studied in bioconjugation chemistry (Hynes and Peach, 1986; Spokoyny et al., 2013; Zhang et al., 2013, 2014; Zou et al., 2014; Dai et al., 2016; Lautrette et al., 2016; Kalhor-Monfared et al., 2017; Evans and Pentelute, 2018).
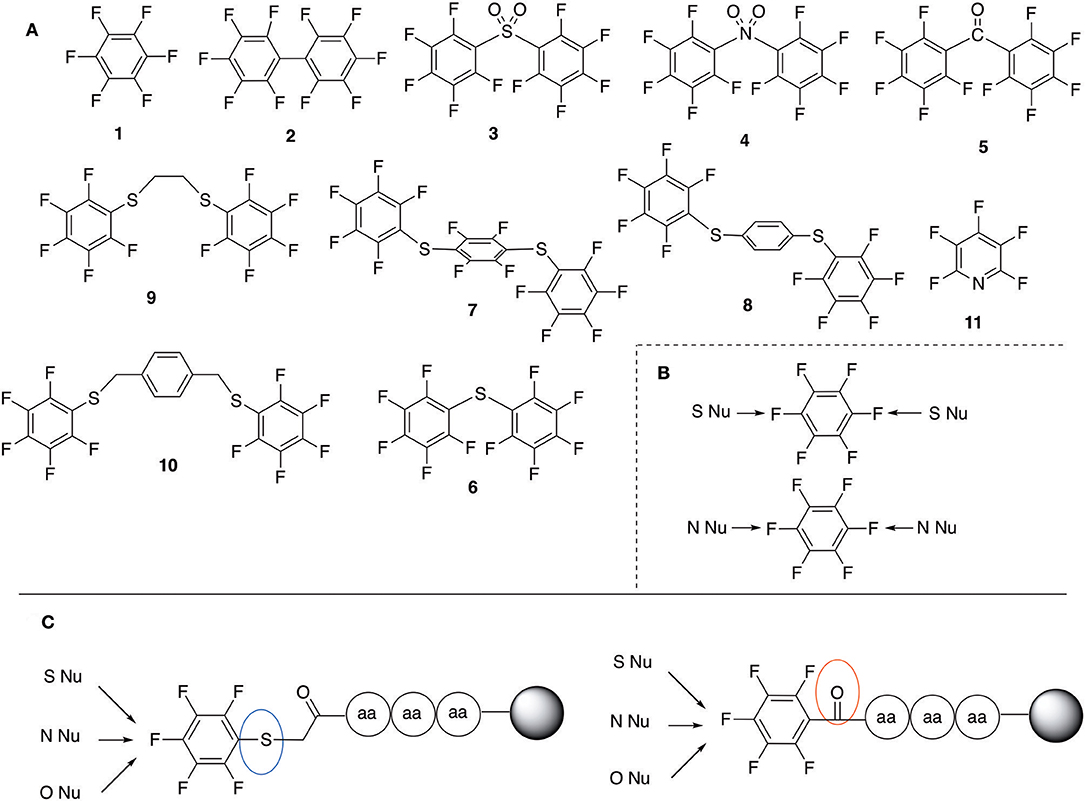
Scheme 1. (A) Perfluoro aromatic linkers that has been previously investigated (B) Types of SNAr nucleophilic reactions (C) Present work.
All of the linkers previously described are symmetric (Scheme 1B), which might limit their application to some extent. To the best of our knowledge, all of the reported fluorine-based linkers are connecting mainly through S and in much less extension N nucleophiles in their/two sides. Finally, all fluorine based conjugation has been carried out in solution, which could be less selective and require tedious purification of the final products (Spokoyny et al., 2013; Zhang et al., 2013, 2014; Zou et al., 2014; Dai et al., 2016; Lautrette et al., 2016; Kalhor-Monfared et al., 2017; Evans and Pentelute, 2018). Herein, we report on the investigation of unsymmetrical fluorine based linkers in solid phase that enable peptides to be coupled to N, O, and S containing substituents (Scheme 1C).
This study investigates the reactivity and site specificity of two new generation of perfluoroaromatic linkers, 2–6-pentafluoroacetic acid and 2–6-pentafluorothiophenol anchored to the model peptide H-Gly-Phe-Leu-NH-resin (Scheme 1C). Both linkers are commercially available, which could facilitate its use by the scientific community. The reactivity of these novel scaffolds/linkers under SNAr with three types of soft-hard nucleophiles such as amines, thiols and alcohols was studied, which represent the functionality found in peptides and other biomolecules. Overall, an unsymmetrical substitution in these fluorine containing linkers is illustrated.
Results and Discussion
In addition to the pentafluoro system, the first bidentate linker contains a carboxylic acid, while the second a thiol (Scheme 2), which results in diversity in the conjugation. In the first instance, the short peptide H-Gly-Phe-Leu-NH-Resin was built up using solid-phase peptide synthesis (SPPS) methodology. 2–6-Pentafluorobenzoic acid was safely coupled to the tripeptidyl resin using DIC and OxymaPure to form L1. On the other hand, 2–6-pentafluorothiophenol was incorporated to the iodoacetyl derivative of the tripeptidyl resin in the absence of any extra reagent to form L2 (Scheme 2). L1 contained a carboxamide which is an electron withdrawing group and L2 an alkylthio S which is an electron donating.
The reactivity of these two series of peptidyl resins in SNAr reactions with different nucleophiles were investigated. Both peptidyl resins were left to react with thiols, amines, phenols, and alcohols, which are the nucleophiles present in the biomolecules. Firstly, using butylamine, which has a moderate reactivity in comparison to S nucleophiles, solvent optimization was studied using N,N'-diisopropylethylamine (DIEA) as a base and L1 as substrate. The use of polar solvents such N,N-dimethylformamide (DMF) and N-methylpyrrolidone (NMP) results in a higher yield when compared to less polar solvents such as dichloromethane (DCM) and/or toluene (Table 1). This is possibly due to stabilization of the Meinsheimer complex formed during the SNAr (Alapour et al., 2015). The best yield obtained during solvent optimization was 35% in NMP (#2, Table 1). This can be considered mediocre. Poor conversion was also experienced when Tris was used as a base. However, use of the non-nucleophilic DBU, a stronger base than DIEA, resulted in yields of 99%. Thus, the optimized conditions were identified as using NMP as a solvent and DBU as a base with a relatively short reaction time of 1 h (#6, Table 1). In all cases, 19F NMR confirmed that the regioisomer para to the carboxamide group was the only product obtained (Table 2, Figure 1A).
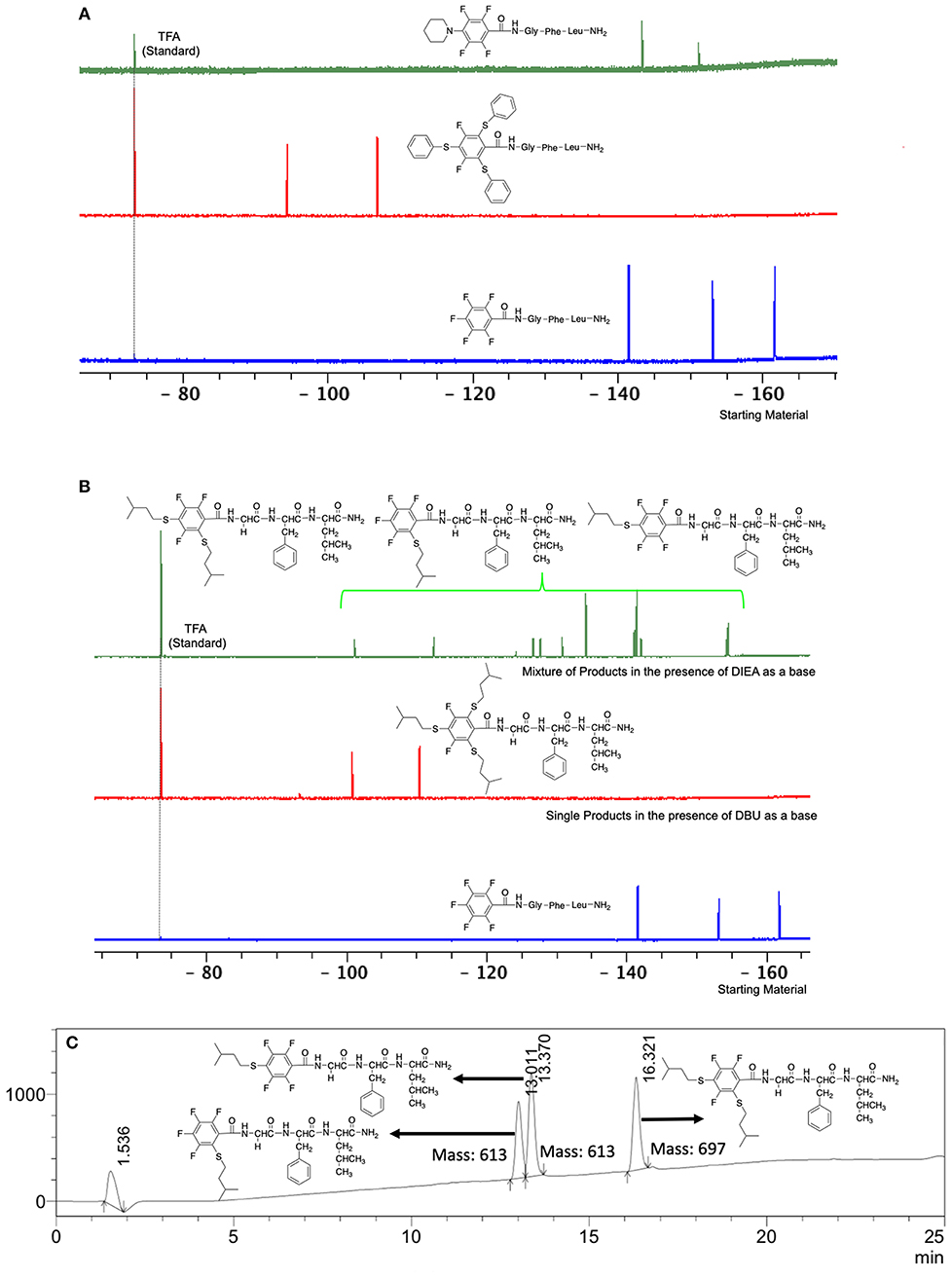
Figure 1. (A) Comparison of the 19F NMR of the product of the reaction between piperidine and benzenethiol as a reactant with the peptide linker L1 using DBU as a base and NMP as solvent (room temp., 1 h) and the starting material L1; (B). Comparison of 19F NMR of the product of the reaction of 3-methyl-1-butanethiol with starting material L1 in two different bases (DBU and DIEA) using NMP as a solvent (room temp., 1 h); (C). LC-MS of the reaction of methyl-1-butanethiol with the starting material L1 in NMP and DIEA as a base (acetonitrile:water step gradient from 30 to 95% in 15 min and held at 95% acetonitrile in water for 5 min, then flushed with 30% acetonitrile in water for 5 min).
The same conditions (NMP as a solvent and DBU as a base) were used for L2 with the same high yields (#2, Table 2). Piperidine and piperazine as nucleophiles also showed excellent conversion in all cases in just 1 h (Table 2, #1–6). Selectivity of the reaction toward para substitution was not affected when a secondary amine was used (Table 2, #3–6). Furthermore, when the bis-amine (piperazine) was used, cross-linking was not detected (Table 2, #5–6).
The reaction with phenol was slower as full conversion was only reached after 24 h. In both cases, only the substitution in para position was detected (Table 2, #7 and 8). On the other hand, no product was observed with aliphatic alcohols (ROH), even after 48 h (Table 2, #9 and 10).
Finally, both aliphatic and aromatic S containing nucleophiles resulted in triple substitutions in both ortho and para positions (Table 2, #11–14). Stabilization of the Meisenheimer complex through resonance of the negative charge at the sulfur on the para position of the thioether moiety enhanced reactivity of the linker-peptide complex to nucleophilic substitution (Birchall et al., 1967; Langille and Peach, 1972; Zou et al., 2014; Alapour et al., 2018). In this case, this reactivity was observed by substitution up to three positions in both groups of I and II.
A milder base, DIEA was also applied in the investigation of the reaction of 3-methylbutan-1-thiol and L1 for S substitution. However, a mixture of mono-ortho, mono-para and di-para and ortho products were obtained. This was confirmed via 19F NMR and LCMS and can be seen in Figure 1B and Figure 1C, respectively. Using a stronger base, DBU, only a single trisubstituted product at the ortho and para positions were observed (Figure 1B). However, the use of DIEA as a weak base for this fast SNAr allowed us to have a better understanding of the present sequence in this reaction. According to the HPLC profile, it seems that strong nucleophiles such as RSH starts with monosubstitution at either the para or ortho positions, and proceeds to disubstitution at the ortho and para positions as the reaction continues. The absence of a trisubstituted product indicates that DIEA is not strong enough to facilitate triple substitution. This reaction also monitored without using any base, however no product was detected in absence of base.
Based on the report by Pentalute et al. having a S group can activate the para position and increase the reactivity of the fluorolinker for further substitution (Lühmann et al., 2016). However, based on our experiments, the same reactivity was observed with both L1 and L2, indicating that electron withdrawing groups such as the carbonyl group or electron donating groups such as thiols do not affect the reactivity or selectivity of the reaction. According to the mechanism (Scheme 3), the Meisenheimer complex can be stabilized by the delocation of the negative charge on either S in L1 or oxygen of the carbonyl group in L2. This explains why both L1 and L2 show the same reactivity toward different nucleophiles.
Theoretical Calculations
To elucidate the electronic effect on perfluorobenzene (Group I) and perfluorobiphenyl (Group II) conjugated on the tripeptide during the different nucleophilic substitution reactions, a density functional theory (DFT) geometry optimization calculation performed in the Gas phase was carried out. DFT in the Gaussian09 program package (Frisch et al., 2009) employing the B3LYP (Becke three parameters Lee–Yang–Parr) exchange correlation functional, which combines the hybrid exchange functional of Becke (1988) with the gradient-correlation functional of Lee et al. (1988) and the 6-31G(d) basis set was used. The frequency calculations afforded no negative Eigen values indicating stability of the molecule. After optimization of the molecules, their atomic charges were calculated using natural bond orbital (NBO) analysis, another efficient tool for studying hyperconjugative interactions, intermolecular charge transfer, and electron density transfer (EDT), which are fundamentally linked for calculations of atomic charges (Drissi et al., 2015; Md Abdur Rauf et al., 2015; El-Faham et al., 2016; Kouakou Nobel et al., 2017).
In the present work, NBO analysis was performed on all derivatives including one nucleophilic substitution on Group I and II, and comparison was made on the basis of the charge carried by the fluorine atom (Supplementary Data). Table 3 shows the electronic charges present on fluorine in all molecules. As seen from Table 3, the fluorine atom in Group I and II carries a negative charge due to high electronegativity of the fluorine atom and are quite similar upon comparison of Group I and II, which indicates identical reactivity toward nucleophilic substitution at the para position (as confirmed by 19F NMR).
Due to the multiple substitution at the ortho and para positions in case of thiol, comparison of charge carried by fluorine after the first substitution was also studied (Table 3). To ease the explanation, the fluorine atom in the molecule was labeled as 1-F, 2-F, 3-F, 4-F, and 5-F. As explained by NMR studies, the substitution of 3-F by nucleophiles was observed which changed the atomic charges on remaining fluorine atoms in both cases. It was observed that after substitution by amines as nucleophile in the case of Group I and II, the charges carried by remaining fluorine atoms attain a more negative value i.e., ~-0.320 to −0.330 from that of −0.304 to −0.322, which may be the reason for making the next substitution difficult under the same reaction conditions leading to the formation of only mono-substituted product.
On the other hand, when phenol was used as a nucleophile, a similar increase of charge on fluorine was witnessed (as explained above), and hence explained the mono-substituted product. In the case of substitution by thiol at the para position, the charge carried by the rest of the fluorine atoms (# 6, 7, 13, and 14) is still comparable to that of Group I and II (# 1 and 8) especially for 1-F and 5-F, respectively, explaining the formation of the three substituted products (#6, 7, 13, and 14), irrespective of the nucleophile being aliphatic or aromatic as a similar conclusion was drawn in both cases.
Conclusion
Using a solid phase approach, the reactivity and chemoselectivity of fluorine containing linkers for peptide conjugation was studied. Using DBU as a base and NMP as solvent, primary and secondary amines and phenols render the mono substituted product in the para position in both linkers. On the other hand, when thiols are used as the nucleophile, the tri-substituted product (at the ortho and para positions) takes place. Using thiols in the presence of a weaker base such as DIEA, a mixture of the mono- (ortho or para) and di-substituted (ortho and para) were obtained. The experimental data was corroborated further with theoretical calculations. Natural atomic charges were calculated and were also found to be consistent in explaining the experimental results. These two linkers could be used for the conjugation of amines and phenols and for the preparation of dendrimers in the case of thiols.
Author Contributions
All experiments, data analysis, discussion and the manuscript preparation were done by SA. The computational analysis and the discussion of computational data are done by AS. BdlT, DR, NK, and FA assisted with experiment design, manuscript preparation, and discussion. All authors have approved the submitted manuscript.
Funding
The project was funded by Pelchem and the Fluorochemical Expansion Initiative of Department of Science and Technology-National Research Foundation (DST-NRF) South Africa. Furthermore, National Research Foundation (NRF) (Blue Sky's Research Programme # 110960) and the Generalitat de Catalunya (2017 SGR 1439) (Spain) contributed to the funding of this project.
Conflict of Interest Statement
The authors declare that the research was conducted in the absence of any commercial or financial relationships that could be construed as a potential conflict of interest.
Supplementary Material
The Supplementary Material for this article can be found online at: https://www.frontiersin.org/articles/10.3389/fchem.2018.00589/full#supplementary-material
References
Alapour, S., de la Torre, B. G., Ramjugernath, D., Koorbanally, N. A., and Albericio, F. (2018). Application of decafluorobiphenyl (DFBP) moiety as a linker in bioconjugation. Bioconjug. Chem. 29, 225–233. doi: 10.1021/acs.bioconjchem.7b00800
Alapour, S., Ramjugernath, D., and Koorbanally, N. A. (2015). Copper-catalysed cross-coupling affected by the Smiles rearrangement: a new chapter on diversifying the synthesis of chiral fluorinated 1,4-benzoxazine derivatives. RSC Adv. 5, 83576–83580. doi: 10.1039/C5RA18897K
Becke, A. D. (1988). Density-functional exchange-energy approximation with correct asymptotic behavior. Phys. Rev. A 38, 3098–3100. doi: 10.1103/PhysRevA.38.3098
Birchall, J. M., Green, M., Haszeldine, R. N., and Pitts, A. D. (1967). The mechanism of the nucleophilic substitution reactions of polyfluoroarenes. Chem. Commun. 338–339. doi: 10.1039/c19670000338
Dai, P., Zhang, C., Welborn, M., Shepherd, J. J., Zhu, T., Van Voorhis, T., et al. (2016). Salt effect accelerates site-selective cysteine bioconjugation. ACS Cent. Sci. 2, 637–646. doi: 10.1021/acscentsci.6b00180
Drissi, M., Benhalima, N., Megrouss, Y., Rachida, R., Chouaih, A., and Hamzaoui, F. (2015). Theoretical and experimental electrostatic potential around the m-nitrophenol molecule. Molecules 20, 4042–4054. doi: 10.3390/molecules20034042
El-Faham, A., Soliman, S. M., Ghabbour, H. A., Elnakady, Y. A., Mohaya, T. A., Siddiqui, M. R. H., et al. (2016). Ultrasonic promoted synthesis of novel s-triazine-Schiff base derivatives; molecular structure, spectroscopic studies and their preliminary anti-proliferative activities. J. Mol. Struct. 1125, 121–135. doi: 10.1016/j.molstruc.2016.06.061
Evans, E. D., and Pentelute, B. L. (2018). Discovery of a 29-amino-acid reactive abiotic peptide for selective cysteine arylation. ACS Chem. Biol. 13, 527–532. doi: 10.1021/acschembio.7b00520
Frisch, M. J., Trucks, G. W., Schlegel, H. B., Scuseria, G. E., Robb, M. A., Cheeseman, J. R., et al. (2009). Gaussian 09, Revision B.01. Wallingford, CT.
Hynes, R. C., and Peach, M. E. (1986). The thiolate anion as a nucleophile. Part XIII. Reactions of some tin(II) aromatic thiolates. J. Fluorine Chem. 31, 129–133. doi: 10.1016/S0022-1139(00)80528-X
Kalhor-Monfared, S., Jafari, M. R., Patterson, J. T., Kitov, P. I., Dwyer, J. J., Nuss, J. M., et al. (2017). Correction: rapid biocompatible macrocyclization of peptides with decafluoro-diphenylsulfone. Chem. Sci. 8:807. doi: 10.1039/C6SC90071B
Kobzev, S. P., Soloshonok, V. A., Galushko, S. V., Yagupolskii, Y. L., and Kukhar, V. P. (1989). Fluorine-containg amino-acids.6. Acis-Base properties of alpha-trifluoromethyl-alpha-amino acids. Zhurnal Obshchei Khimii 59, 909–912.
Kouakou Nobel, N. G., Bamba, K., Wawohinlin Patrice, O., and Ziao, N. (2017). NBO population analysis and electronic calculation of four azopyridine ruthenium complexes by DFT Method. Comput. Chem. 5, 51–64. doi: 10.4236/cc.2017.51005
Langille, K. R., and Peach, M. E. (1972). The thiolate anion as a nucleophile part I. Reactions with hexafluorobenzene, decafluorobiphenyl and hexachlorobenzene. J. Fluorine Chem. 1, 407–414. doi: 10.1016/S0022-1139(00)82961-9
Lautrette, G., Touti, F., Lee, H. G., Dai, P., and Pentelute, B. L. (2016). Nitrogen arylation for macrocyclization of unprotected peptides. J. Am. Chem. Soc. 138, 8340–8343. doi: 10.1021/jacs.6b03757
Lee, C., Yang, W., and Parr, R. G. (1988). Development of the Colle-Salvetti correlation-energy formula into a functional of the electron density. Phys. Rev. B 37, 785–789. doi: 10.1103/PhysRevB.37.785
Lühmann, T., Mong, S. K., Simon, M. D., Meinel, L., and Pentelute, B. L. (2016). A perfluoroaromatic abiotic analog of H2 relaxin enabled by rapid flow-based peptide synthesis. OBC 14, 3345–3349. doi: 10.1039/C6OB00208K
Md Abdur Rauf, S., Arvidsson, P. I., Albericio, F., Govender, T., Maguire, G. E., Kruger, H. G., et al. (2015). The effect of N-methylation of amino acids (Ac-X-OMe) on solubility and conformation: a DFT study. OBC 13, 9993–10006. doi: 10.1039/C5OB01565K
Morgenthaler, M., Schweizer, E., Hoffmann-Röder, A., Benini, F., Martin, R. E., Jaeschke, G., et al. (2007). Predicting properties and tuning physicochemical in lead optimization: amine basicities. ChemMedChem 2, 1100–1115. doi: 10.1002/cmdc.200700059
O'Hagan, D. (2008). Understanding organofluorine chemistry. An introduction to the C-F bond. Chem. Soc. Rev. 37, 308–319. doi: 10.1039/B711844A
Rowley, M., Hallett, D. J., Goodacre, S., Moyes, C., Crawforth, J., Sparey, T. J., et al. (2001). 3-(4-fluoropiperidin-3-yl)-2-phenylindoles as high affinity, selective, and orally bioavailable h5-HT2A receptor antagonist. J. Med. Chem. 44, 1603–1614. doi: 10.1021/jm0004998
Schilling, C. I., Jung, N., Biskup, M., Schepers, U., and Bräse, S. (2011). Bioconjugation via azide-Staudinger ligation: an overview. Chem. Soc. Rev. 40, 4840–4871. doi: 10.1039/c0cs00123f
Spokoyny, A. M., Zou, Y. K., Ling, J. J., Yu, H. T., Lin, Y. S., and Pentelute, B. L. (2013). A perfluoroaryl-cysteine SNAr chemistry approach to unprotected peptide stapling. J. Am. Chem. Soc. 135, 5946–5949. doi: 10.1021/ja400119t
van Niel, M. B., Collins, I., Beer, M. S., Broughton, H. B., Cheng, S. K., Goodacre, S. C., et al. (1999). Fluorination of 3-(3-(piperidin-1-yl)propyl)indoles and 3-(3-(piperazin-1-yl)propyl)indoles gives selective human 5-HT1D receptor ligands with improved pharmacokinetic profiles. J. Med. Chem. 42, 2087–2104. doi: 10.1021/jm981133m
Wang, J., Sánchez-Roselló, M., Aceña, J. L., Del Pozo, C., Sorochinsky, A. E., Fustero, S., et al. (2014). Fluorine in pharmaceutical industry: fluorine-containing drugs introduced to the market in the last decade (2001–2011). Chem. Rev. 114, 2432–2506. doi: 10.1021/cr4002879
Zhang, C., Dai, P., Spokoyny, A. M., and Pentelute, B. L. (2014). Enzyme-catalyzed macrocyclization of long unprotected peptides. Org. Lett. 16, 3652–3655. doi: 10.1021/ol501609y
Zhang, C., Spokoyny, A. M., Zou, Y., Simon, M. D., and Pentelute, B. L. (2013). Enzymatic “Click” ligation: selective cysteine modification in polypeptides enabled by promiscuous glutathione s-transferase. Angew. Chem., Int. Ed. 52, 14001–14005. doi: 10.1002/anie.201306430
Zhang, C., Welborn, M., Zhu, T., Yang, N. J., Santos, M. S., Van Voorhis, T., et al. (2016). π-Clamp-mediated cysteine conjugation. Nat. Chem. 8, 120–128. doi: 10.1038/nchem.2413
Keywords: fluorolinkers, conjugation, solid-phase peptide synthesis, peptide stapling, chimera
Citation: Alapour S, Sharma A, de la Torre BG, Ramjugernath D, Koorbanally NA and Albericio F (2018) Perfluorophenyl Derivatives as Unsymmetrical Linkers for Solid Phase Conjugation. Front. Chem. 6:589. doi: 10.3389/fchem.2018.00589
Received: 05 October 2018; Accepted: 13 November 2018;
Published: 28 November 2018.
Edited by:
Gwilherm Evano, Free University of Brussels, BelgiumReviewed by:
Benoit Crousse, Centre national de la recherche scientifique (CNRS), FranceBasem Moosa, KAUST Catalysis Center (KCC), Saudi Arabia
Copyright © 2018 Alapour, Sharma, de la Torre, Ramjugernath, Koorbanally and Albericio. This is an open-access article distributed under the terms of the Creative Commons Attribution License (CC BY). The use, distribution or reproduction in other forums is permitted, provided the original author(s) and the copyright owner(s) are credited and that the original publication in this journal is cited, in accordance with accepted academic practice. No use, distribution or reproduction is permitted which does not comply with these terms.
*Correspondence: Neil A. Koorbanally, a29vcmJhbmFsbHlAdWt6bi5hYy56YQ==
Fernando Albericio YWxiZXJpY2lvQHVrem4uYWMuemE=