- 1Department of Organic and Bioorganic Chemistry, State Scientific Institution “Institute for Single Crystals”, National Academy of Sciences of Ukraine (NAS), Kharkiv, Ukraine
- 2Chemistry Faculty, Karazin Kharkiv National University, Kharkiv, Ukraine
The comprehensive review contains the analysis of literature data concerning reactions of heterocyclization of aminoazoles and demonstrates the application of these types of transformations in diversity-oriented synthesis. The review is oriented to wide range of chemists working in the field of organic synthesis and both experimental and theoretical studies of nitrogen-containing heterocycles.
Introduction
Heterocyclic compounds are backbone of drug design–about 80% of the known small molecule drugs belong to this type of substances and among them 60% relates to nitrogen containing heterocycles (Kombarov et al., 2010; Vitaku et al., 2014; Taylor et al., 2016). On the other hand, heterocyclic compounds play important role in other branches of science and are the base of all living organisms. Therefore, study of the appropriate field of organic chemistry is a very important challenge that has been attracting attention of numerous scientific groups for last decades and stimulating for detailed study of the topic including the search for novel and development of known synthetic methods.
One of the important pathways to nitrogen containing heterocycles is reactions of aminoazoles (two-component, one-pot, multicomponent, etc.) being efficient mono-, bi- and polynucleophiles with different electrophiles. The presence of several alternative reaction centers in aminoazoles often makes them useful reagents in controlled multidirectional interactions providing the possibility to synthesize diverse chemotypes of final products (see some examples in Figure 1). Such approach is widely used in the modern heterocyclic chemistry and some books and reviews have been already published in this field (Desenko, 1995; Chebanov and Desenko, 2006, 2012, 2014; Chebanov et al., 2008a, 2010; Moderhack, 2011; Sedash et al., 2012; Tkachenko and Chebanov, 2016; Aggarwal and Kumar, 2018), however, many of them deal with particular problems of aminoazole chemistry and actually during long period no comprehensive analysis of the problem has been made.
Thus, the present review is devoted to diversity-oriented reactions of heterocyclization involving aminoazoles as a key reagent. It presents analysis of literature mainly from 2010 till present and three main types of such reactions are discussed: multicomponent reactions including application of condition-based divergence strategy for the control of their directions; two-component heterocyclizations and one-pot cascade processes; “click”-chemistry concerning azoles and aminoazoles.
Main Part
Multicomponent Reactions of Aminoazoles Involving Cyclic CH-Acids
Multicomponent reactions (MCRs) involving aminoazoles and aldehydes with cyclic CH-acids (different ketones, 1,3-diketones, Meldrum's acid etc.) are similar to the classic Hantzsch or Biginelly condensations. In early publications they had often resulted in the formation of mixtures of positional and regioisomers, therefore, some efficient methods for tuning chemo- and regioselectivity of such multicomponent heterocyclizations, including Condition-based divergence strategy to switch their directions by simple variation of the reaction conditions (solvent, temperature, method of activation–microwave irradiation (MW) and ultrasonication (US), catalyst, etc), were found and developed (Desenko, 1995; Chebanov and Desenko, 2006, 2012; Chebanov et al., 2008a, 2010; Ruijter et al., 2011).
Varying temperature and catalyst allowed authors (Chebanov et al., 2007b, 2008b) to switch the heterocyclization of aromatic aldehydes 1, 1,3-cyclohexanedione (2a) or dimedone (2b) with 5-amino-3-arylpyrazoles 3 between two directions with the formation of pyrazoloquinolinenones 6 (EtOH-Et3N, MW, 150°C, 15 min) and pyrazoloquinazolinones 7 (EtOH, US, r.t., 30 min) being the products of thermodynamically and kinetically controlled reactions, respectively. Non-classical activation methods led to the reduction in time; moreover, applying microwave activation allowed to carry out the transformations at higher temperatures in comparison with standard heating, thus, additionally favoring reaction regioselectivity in case of thermodynamically controlled pathway (Figure 2).
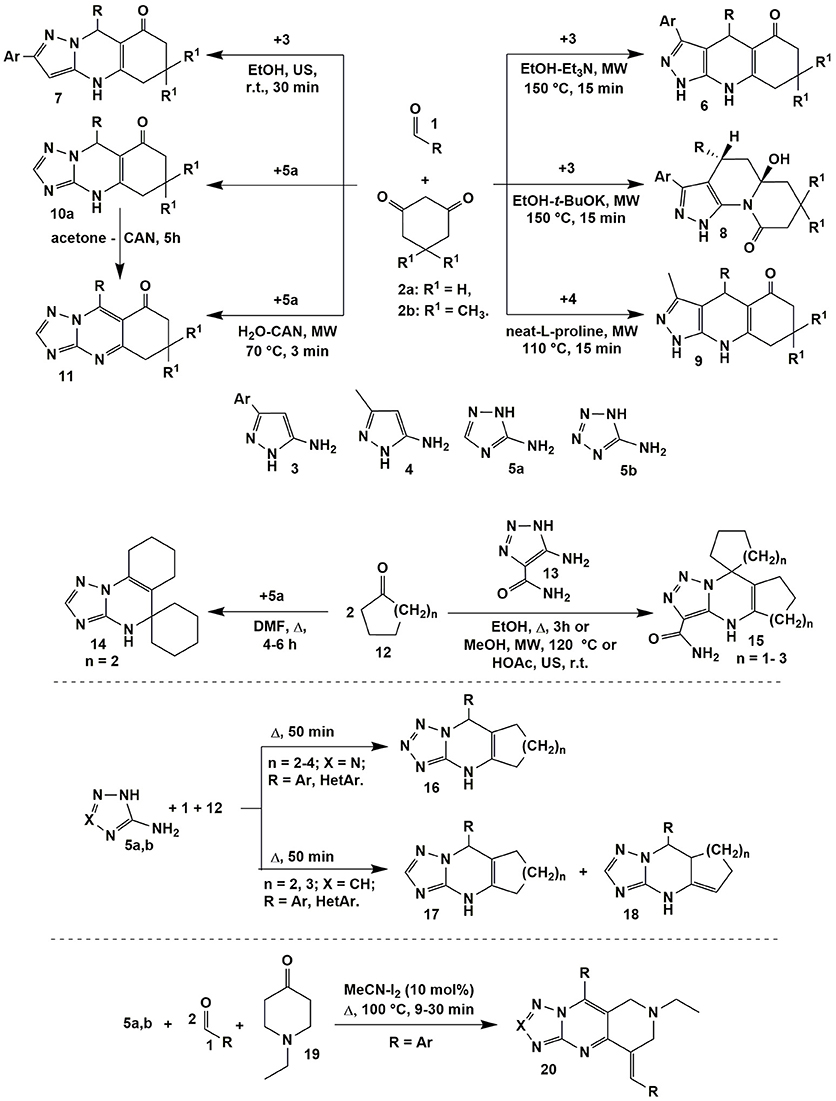
Figure 2. Examples of the condensations involving α-aminoazoles, aldehydes and cyclic carbonyl compounds.
In the process of optimization, the new multicomponent reaction was found: t-BuOK being a stronger nucleophile then Et3N attacked the carbonyl group of cyclic 1,3-diketone moiety in the intermediate which resulted in the ring opening and recyclization with the formation of quinolizinones 8. Later on the greener methodology of obtaining pyrazoloquinolinenones 6 was elaborated using microwave synthesis in water (170°C, 10 min; Andriushchenko et al., 2011). Similar to compounds 6 pyrazoloquinolinenones 9 were synthesized even without solvent using L-proline as a catalyst (MW, 110°C, 15 min; Bhattacharjee et al., 2016).
The analogous to heterocycles 7 linear quinazolinones 10 were obtained on the basis of 3-amino-1,2,4-triazole (5a) applying the great variety of conditions (only the endocyclic aminogroup in the position 2 took part in the condensation; Puligoundla et al., 2013; Petrov and Kasatochkin, 2014; Sompalle et al., 2016; Vibhute et al., 2017a,b). It should be noted, that in all cases tetrahydroderivatives 6–10 were formed. However, Petrov and Kasatochkin (2014) oxidized partially hydrogenated pyrimidine ring of 10 to obtain compounds 11 using ceric ammonium nitrate (CAN) in acetone. Later on the compounds 11 were synthesized in the three-component reaction of 1, 2a and 5a in water under microwave irradiation also with application of CAN (Figure 2; Sompalle and Roopan, 2016).
Linear tetrahydroquinazolinones of type 10 had been also formed in condensations involving 5-amino-4-aryl-1,2,3-triazole and 5-amino-N-aryl-1,2,3-triazole-4-carboxamide (Gladkov et al., 2010), 5-aminotetrazole (Shen et al., 2013; Gein et al., 2015; Kour et al., 2017), 2-aminobenzimidazole (Puligoundla et al., 2013; Maleki et al., 2015), 2-aminoindazole moiety (Palaniraja and Roopan, 2015; Shinde and Jeong, 2016), methyl 5-amino-pyrazole-4-carboxylate (Lipson et al., 2010) and 5-amino-pyrazole-4-carbonitrile (Lipson et al., 2010), 4-aryl-5-aminopyrazole (Petrov and Kasatochkin, 2014). It should be noted, that N-unsubstituted 5-amino-1,2,3-triazole-4-carboxamide showed the same behavior and the products of reaction involving carboxamide aminogroup were not separated (Gladkov et al., 2012, 2013).
One of the first angular-structured heterocycles 14 was formed in the ABB′ type multicomponent reaction of 3-amino-1,2,4-triazole (5a) (X = CH) and two equivalents of cyclohexanone 12 (n = 2) and described by Desenko et al. (1990; Figure 2). When 5-amino-1,2,3-triazole-4-carboxamide (13) was introduced into the same condensation linear compounds 15 with other positional orientation of ketone moieties were obtained. The same heterocycles were synthesized in the reaction with cyclopentanone 12 (n = 1; Gladkov et al., 2012). However, cycloheptanone (12) (n = 3) did not react in a multicomponent procedure, therefore, corresponding spiroheterocycles of type 15 were got by the stepwise protocol through the synthesis of cycloheptalidenecycloheptanone [using two equivalents of ketone 12 (n = 3)] and further cyclization with 5-amino-1,2,3-triazole-4-carboxamide (13). It's worth noting that in case of other ketones 12 (n = 0, 1, 2) compounds of type 15 were formed both by the stepwise and by the multicomponent protocols (Figure 2; Gladkov et al., 2012).
ABC type multicomponent cyclization of 5-aminotetrazole (5b) (X = N), different aromatic and heteroaromatic aldehydes 1 and ketones 12 (n = 2–4) under heating without solvent afforded only one linear isomer 16 (Matveeva et al., 2013), while the same reaction involving 3-amino-1,2,4-triazole (5a) (X = CH) resulted in formation of the mixture of isomeric cycloalkatriazolopyrimidines 17 and 18 (Figure 2; Matveeva et al., 2015). The analogous to compounds 16 linear tetrahydrobenzo[h]tetrazoloquinazolines were yielded in the condensation of the reagents 1, 5b with α-tetralone acting as CH-acid (Kantin and Krasavin, 2016).
When 1-ethyl-4-piperidinone (19) was used as a CH-acid in the condensation with two equivalents of aromatic aldehyde 1 and 3-amino-1,2,4-triazole (5a) or 5-aminotetrazole (5b) or (2-aminobenzimidazole) upon heating (MeCN-I2, Δ, 100°C) 1,2,3,4-tetrahydro-pyrido[4,3-d]tetrazolo[1,5-a]pyrimidines 20 bearing in situ oxidized triazolopyrimidine system were formed (Figure 2; Farghaly et al., 2015).
The condensations involving 5-amino-3-methyl-1-phenylpyrazole (21) afforded fused heteroaromatic azolopyridines. Thus, the variation of acid-base properties of the reaction medium led to the change in a sequence of elementary stages in multicomponent reaction involving 5-amino-3-methyl-1-phenylpyrazole (21), cyclopentanone (12a) and aromatic aldehydes 1 that allowed to switch the reaction between two alternative directions and selectively got positional isomers–angular pyrazolopyridines 23 (n = 1; Wang et al., 2011) and linear heterocycles 24. Another authors (Jiang et al., 2011; Chen et al., 2015) described fused pyrazolopyridines 23 with n = 2–4, 8 (Figure 3).
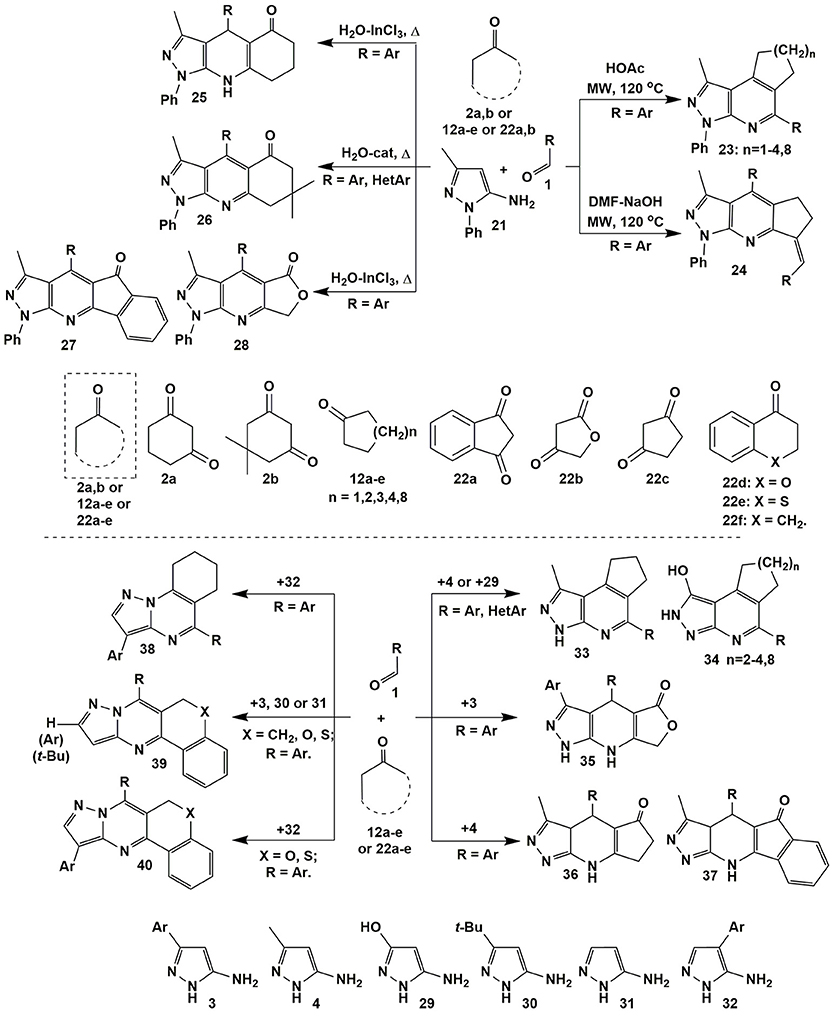
Figure 3. Examples of heterocyclization reactions involving 5-aminopyrazoles, aldehydes and cyclic active methylene compounds.
Several publications deal with condensations of the reagents 1 and 21 with 1,3-diketones [dimedone (2b) (Karnakar et al., 2012; Wang and Shi, 2012), indane-1,3-dione (22a) (Quiroga et al., 2008; Shi et al., 2009) and furane-2,4-dione (22b) (Shi et al., 2009)] resulting in the formation of heteroaromatic derivatives 26–28. It's interesting that under the same conditions (H2O-InCl3, Δ) Khurana et al. (2012) obtained dihydropyrazolopyridines 25 only from 1,3-cyclohexanedione (2a), whereas in case of indane-1,3-dione (22a) and furane-2,4-dione (22b) heteroaromatic compounds 27, 28 were formed (Figure 3).
Similar to heterocycles 23 angular products 33 (DMF-MeOH, Δ; Lipson et al., 2015) and 34 (HOAc-TFA, MW, 140°C; Jiang et al., 2011) were also got in the condensation with 5-amino-3-methylpyrazole (4) and 5-amino-3-hydroxypyrazole (29), while the transformations involving 5-amino-4-arylpyrazoles 32 afforded pyrazolopyrimidines 38 (HOAc, Δ; Figure 3; Petrov and Kasatochkin, 2013).
An exhaustive review on the properties of 5-aminopyrazoles as precursors in design and synthesis of fused pyrazoloazines being published yet (Aggarwal and Kumar, 2018) describes the reaction of 5-amino-3-methyl-1-phenylpyrazole (21) and aromatic aldehydes 1 with 4-hydroxycoumarin, where 3 types of possible products (4,7-dihydropyrazolo[3,4-b]pyridine-, aromatized pyrazolo[3,4-b]pyridine derivatives and the product of C–O bond cleavage from cyclic ester) were formed depending on the type of solvent and temperature. MCRs of 1-aryl-3-indolyl-5-aminopyrazoles, cyclic β-diketones (dimedone, cyclopentanedione, indane-1,3-dione) and aromatic aldehydes also gave dihydro- and aromatized pyrazolo[3,4-b]pyridine derivatives, what is more, dihydropyrazolo[3,4-b]pyridines formed could be transformed into their heteroaromatized analogs by prolonged heating in acetonitrile with DDQ (2,3-dichloro-5,6-dicyanobenzoquinone).
It should be noted, that reactions with chroman-4-one (22d), thiochroman-4-one (22e) or 3,4-dihydronaphthalen-1(2H)-one (22f) (EtOH-t-BuOK, Δ) despite of the origin or position of the substituent in pyrazole afforded only heteroaromatized “classical” Biginelly-type pyrazolopyrimidines 39 and 40 (Saikia et al., 2014). Condensations of 1,3-diketones with 3-substituted 5-aminopyrazoles 3 and 4 also led exclusively to linear dihydropyrazolopyridinones 35 (Hatti et al., 2015) and 36, 37 (Lipson et al., 2015), correspondingly (Figure 3).
Using 5-aminopyrazoles 41 bearing carboxamide fragment in the fourth position in condensations with 1,3-cyclohexanediones 2a,b and aromatic aldehydes 1 led to widening the scope of target compounds whereas varying reaction parameters and applying non-classical methods of activation (ultrasonication and microwave irradiation) allowed to switch cyclizations between several directions (Figure 4; Chebanov et al., 2012b).
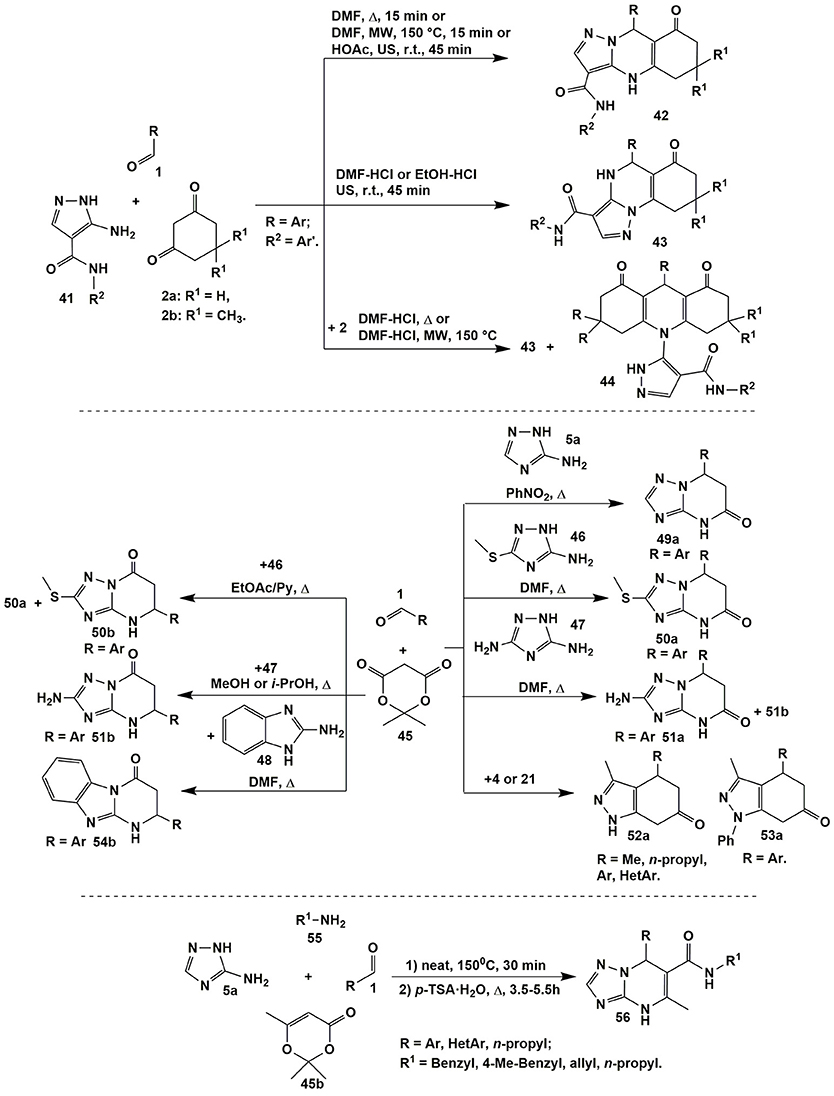
Figure 4. Examples of azoloazines synthesis via reactions involving 5-aminopyrazole-4-carboxamides of 3-amino-1,2,4-triazoles derivatives.
Thus, condensation of starting reagents 1, 2a,b, and 41 upon heating or MW irradiating in DMF or ultrasonication in HOAc at room temperature always afforded tricyclic dihydropyrimidines 42. Addition of catalytic amounts of hydrochloric acid resulted in switching the reaction to another direction and yielding positionally isomeric angular compounds 43. Implementation of the third route with the formation of acrydindiones 44 occurred upon increasing the temperature and introducing the double excess of diketone 2 (Chebanov et al., 2012b).
Meldrum's acid is also widely used as a building block for the synthesis of azoloazine systems. A significant contribution to the study of the condensations involving aminoazoles and aldehydes with Meldrum's acid was made by Lipson's group (Figure 4; Lipson and Gorobets, 2009). It was established, that in some cases these multicomponent reactions afforded positional isomers. For example, condensations involving 3-amino-5-methylthio-1,2,4-triazole (46) gave 5-pyrimidinones 50a or 7-pyrimidinones 50b with impurities of 50a depending on solvent and catalyst (Lipson and Gorobets, 2009).
The opposite situation was observed in case of 3,5-diamino-1,2,4-triazole (47): 5-pyrimidinones 51a were obtained only in mixture with isomers 51b. At the same time the latter compounds 51b were isolated in a pure state after changing DMF to methanol or isopropanol (Lipson and Gorobets, 2009). When 3-amino-1,2,4-triazole 5a (Lipson and Gorobets, 2009) took part in the condensation with compounds 1 and 45 only 5-pyrimidinones 49a were isolated while the reaction involving 2-aminobenzimidazole 48 (Sheibani et al., 2012) afforded only 7-pyrimidinones 54b (Figure 4).
Condensations of 5-amino-3-methylpyrazole (4) (Lipson and Gorobets, 2009; Zhong et al., 2013) or 5-amino-3-methyl-N-phenylpyrazole (21) (Shi et al., 2011) with aldehydes 1 or arylglyoxals (Petrova et al., 2014) and Meldrum's acid 45 under various conditions gave 5-pyrimidinones 52a and 53a, correspondingly (Figure 4); similar heterocycles were obtained from 2-aminobenzothiazole (Arya et al., 2012).
An interesting one-pot four-component reaction was described by Shaabani et al. (2015): [1,2,4]triazolo[1,5-a]pyrimidine-6-carboxamide derivatives 56 were synthesized via reaction of primary aliphatic or aromatic amines 55 and 2,2,6-trimethyl-4H-1,3-dioxin-4-one (45b) (heating under solvent-free conditions, 150°C, 30 min) followed by the subsequent condensation with 3-amino-1,2,4-triazole (5a) and aliphatic or aromatic aldehydes 1 (H2O-p-TSA, Δ, 3.5–4.5 h; Figure 4).
The presence of four non-equivalent reaction centers in 1,2-diamino-4-phenylimidazole (57) makes possible new alternative reaction routes with electrophilic reagents. Due to the lower nucleophilicity of exocyclic amino groups in comparison with endocyclic CH-group, 1,2-diaminoimidazoles in the reactions with α,β-unsaturated ketones, their mono- and dibromo derivatives, with aroylacrylic acids, and in the three-component reactions with aldehydes and Meldrum's acid formed not triazepine fragments but pyridazine and pyrimidine systems fused with azole cycle (Lipson et al., 2012). This fact was also confirmed in the multicomponent reaction involving 1,3-cyclohexanediones 2a,b upon boiling in DMF (1 h) or methanol (2 h) which resulted in the formation of dihydroimidazo[1,5-b]cinnolinones 58. Only in case of 4-nitrobenzaldehyde 1 the short-term boiling the compounds 1, 2 and 57 in DMF led to the formation of heteroaromatic derivatives 59 (Figure 5; Lipson et al., 2012).
N-Unsubstituted 2-aminoimidazole exhibited similar properties (lower nucleophilicity of exocyclic aminogroup than endocyclic reaction centers) in the reactions with aromatic aldehydes and different CH-acids (dimedone, barbituric acid), but instead of cyclic products the treatments yielded Michael adducts (with participation of CH-center in the position 3; Andriushchenko et al., 2013). On the other hand, the formation of Mannich bases in the similar reactions involving 2-aminothiazole indicates on the higher reactivity of its exocyclic NH2-group in comparison with the endocyclic nucleophilic centers (Ghatole et al., 2015).
There is contradictory data in the literature about three-component reactions involving 5-amino-3-methyl(aryl)isoxazole 60 and aromatic aldehydes 1 with various 1,3-diketones. Thus, Tu et al. (2009) carried out that condensations under microwave irradiation at 120°C in water and synthetized isoxazolo[5,4-b]pyridines 61–63. Surprisingly, heterocyclization involving 1,3-cyclohexanedione (2a) under the same conditions led to dihydroderivatives 64 (Figure 5).
Later on Muravyova et al. (2013) carried out wide screening of the reaction conditions and found that in all cases including those ones described in the work of Tu et al. (2009) [in the model reactions with 1,3-cyclohexanedione (2a) and dimedone (2b)] dihydroisoxazole[5,4-b]pyridines 64 and 67, respectively, had been formed. Annulated with furane-2,4-dione moiety dihydroisoxazolo[5,4-b]pyridines 65 were yielded upon boiling the starting materials in EtOH (Kamal et al., 2011). Hamama et al. (2012) managed to synthesize angular heterocycles 66 on the basis of indane-1,3-dione (22a) [EtOH-HOAc (15:1), Δ], however, we suggest that there is not enough data proving the structure of compounds 66 (Figure 5).
When Meldrum's acid was used, different compounds−4,7-dihydroisoxazolo[5,4-b]pyridine-6(5H)-ones 68 (6–9 min) or spiroheterocycles 69 (9–13 min) were got under almost the same conditions by Tu et al. in two consecutive publications (Tu et al., 2009; Ma et al., 2010). Later on Morozova et al. (2017) reproduced the synthesis of compounds 68 and 69 under the same conditions, however, all the attempts gave only the mixtures of compounds 68 and 69 or heterocycles 69 were isolated in the lower yields than in the previous work (Tu et al., 2009). Therefore, Morozova et al. (2017) studied in details the reactions of 5-amino-3-methylisoxazole (60a) and aromatic aldehydes 1 with Meldrum's acid (45) and developed the preparative methodologies for selective synthesis of the products 68 (boiling in DMF or n-BuOH) and 69 (ultrasonication in EtOH).
The detailed study of the reactions of 5-aminopyrazoles (3a, 4, 21, and 70) with aromatic aldehydes 1 and barbituric acids 71 (a: X = O, b: X = S) showed that varying temperature and type of R3-substituent in 5-aminopyrazole were the main factors of switching the direction to produce different final compounds (Muravyova et al., 2009). Thus, in case of pyrazoles 3a and 4 (R3 = H) boiling the reagents in DMF led to dihydropyrazolopyridopyrimidines 72 (Muravyova et al., 2009) whereas pyrazoles 70 and 21 bearing methyl or aryl R3-substituents (both of electron donor and acceptor origin) afforded heteroaromatized derivatives 75 (Muravyova et al., 2009). It's interesting to note that Satasia et al. (2014) got dihydropyrazolopyridopyrimidines 73 in case of all the pyrazoles (R3 = H, Ph) under refluxing the starting materials in ethanol with adding cellulose supported ionic liquid (Cell-IL) (Figure 5).
Similar to heterocycles 75 other heteroaromatic compounds were synthesized earlier by the group of Shi (Shi et al., 2008) in the reaction of 5-amino-3-methyl-1-phenylpyrazole (21), aromatic aldehydes 1 and barbituric acids 71a (R1 = H, CH3; H2O-p-TSA, MW, 140°C) whereas the condensation involving thiobarbituric acids 71b (R1 = H; neat-p-TSA, MW, 100°C) afforded corresponding dihydropyrazolopyridopyrimidines of type 73 (El-Emary and El-Mohsen, 2012). Later on dihydropyridopyrimidines 74 were synthesized starting from 3-amino-1,2,4-triazole (5a) (Karami et al., 2015a) and 2-aminobenzimidazole (Kaur G. et al., 2015).
Ultrasonication at room temperature of compounds 1 and 71 with 5-aminopyrazoles 3a, 4 or 21 afforded new spiroheterocyclic systems 76 (Muravyova et al., 2009) that had not been formed in the previously described reactions with 1,3-diketones. Analogous reactions involving 5-amino-3-methylisoxazole were studied in several works. After wide screening the reaction conditions similar to compounds 76 spiroheterocycles were the only product obtained in the condensation of 5-amino-3-methylisoxazole (60a) with aromatic aldehydes 1 and barbituric acids 71 under MW irradiation in water (9–13 min; Jiang et al., 2012) or by ultrasonication in ethanol (r.t., 2 h; Morozova et al., 2017).
Replacing 5-amino-3-methylisoxazole (60a) with isomeric 3-amino-5-methylisoxazole did not contribute to the formation of new heterocyclic fragments: it didn't react with aldehydes and barbituric acids or Meldrum's acid (arylidene derivatives were yielded); heating 3-amino-5-methylisoxazole with aldehydes and Meldrum's acid in DMF or ethanol afforded only its acylated derivative (Morozova et al., 2017). Such a reactivity of 3-amino-5-methylisoxazole is consistent with other literature data, which describes its chemical behavior in the synthesis of pyrrolones (Ryabukhin et al., 2012), in the Hantzch (Rajanarendar et al., 2011b) and Betti (Shafiee et al., 2012) reactions as well as in four-component condensation with formation of imidazole moiety (Rajanarendar et al., 2011a).
When glyoxales and arylglyoxales were used instead of aldehydes in multicomponent reactions, some additional reaction pathways could be implemented. Thus, Petrova et al. (2013b) studied the condensations of wide spectrum of aminoazoles (3a, 3b, 4, 5a, 5b, 46, 77a, and 77b) with glyoxales 78 and 1,3-diketones 2a,b or 79 upon refluxing in ethanol (for 30–40 min in case of 5-aminopyrazoles; 2.5–3 h in case of 1,2,4-triazoles and for 10 h in case of 5-aminotetrazole) and obtained the novel heterocyclic system–indolo[1,2-c]polycyclic compounds 80 (Figure 6) instead of the expected 4,5,6,7,8,9-hexahydro-8-oxoazolo[5,1-b]quinazoline-9-carbaldehyde derivatives (similar to compounds 7 or 10, see Figure 2).
In case of 2-aminobenzimidazole 48 its condensation with glyoxal 78 and 1,3-diketones 2a,b under the same conditions led to the formation of benzo[d]imidazo[1,2-a]benzimidazoles 81 containing only one cyclohexanedione fragment in their structure (Figure 6; Petrova et al., 2013b).
When arylglyoxals 83 had been introduced into condensation with 5-aminopyrazoles 82 having arylcarboxamide group in the fourth position, angular pyrazoloquinazolinones 84 (similar to compounds 43, Figure 4) were formed under refluxing in EtOH (Figure 6; Petrova et al., 2013a). Other 1- and 3-substituted 5-aminopyrazoles 21 and 70 (R4 = H) in the condensation with compounds 2a,b and 83 under the same conditions gave expected pyrazoloquinolinenones 85. Applying microwave irradiation in DMSO-Et2NH (120°C, 20 min) to the mixture of diketones 2a,b, pyrazoles 21 or 70 and two equivalents of arylglyoxals 83 afforded an elegant four-component domino reaction leading to polycyclic compounds 86 (Wang et al., 2015b; Figure 6).
A multicomponent reaction involving 2-aminobenzimidazole (48), cyclohexanedione (2a) and arylglyoxales 83 was thoroughly studied by Petrova et al. (2015b); all the compounds including intermediates were isolated in individual form, characterized and their structures were proven with the help of X-Ray analysis (Figure 7).
It was established that the reaction of the reagents 2a, 48, and 83 in ethanol at room temperature for 5–10 min yielded Michael adduct 87 that remained stable upon latter refluxing in primary alcohols. However, after adding the second equivalent of cyclohexanedione (2a) and further prolonged refluxing the reaction mixture in ethanol salts 90 were isolated. All the attempts to convert them into the products of condensation with 2-aminobenzimidazole (48) upon refluxing in DMF or acetic acid or fusion under neat conditions were unsuccessful. In all cases the mixtures of xanthenediones 91 and salts 92 were obtained.
The authors (Petrova et al., 2015b) established that Michael adduct 87 had been also formed after heating the starting reagents in acetic acid at 100°C; after longer heating, they turned into condensed quinazolinones 88. Microwave irradiation of the starting reagents 2a, 48, and 83 in DMF (150°C) afforded to get heterocycles 89 as the main products (Figure 7).
Linear pyrazolopyridinones 93 (Petrova et al., 2015a) were synthesized by the condensation involving 5-amino-3-methyl-pyrazole (4) and arylglyoxales 83 with indane-1,3-dione (22a) upon short-term heating in ethanol. Further prolonged treatment of compounds 93 at room temperature in isopropanol with the addition of KOH led to their transformation into heteroaromatic derivatives 94. When the authors (Petrova et al., 2015a) applied 5-aminopyrazole 21 containing an aryl substituent in the first position, in the reaction with compounds 83 and 22a in ethanol (60–65°C, 15 min) the Michael adduct 95 was initially isolated. Further prolonged boiling the compound 95 in isopropanol led directly to heteroaromatic derivatives 96 whereas dihydroindeno[1,2-b]pyrazolo[4,3-e]pyridinones of type 93 failed to be found (Figure 7).
Multicomponent Reactions of Aminoazoles Involving Non-cyclic CH-Acids
Multicomponent reactions of aminoazoles and carbonyl compounds often involve such CH-acids as enolizable ketones, 1,3-dicarbonyl compounds (acetoacetic acid and its derivatives, 1,3-diketones, ketosulfones), 1,2-dicarbonyl compounds (pyruvic acid and its derivatives), malonic acid and its derivatives, cyanoacetamide etc.
Recently published review by Sedash et al. (2012) clearly illustrates the diversity and complexity of MCRs of aminoazoles, carbonyl compounds and non-cyclic CH-acids on the example of the Biginelly-type transformations involving 3-amino-1,2,4-triazoles as 1,3-binucleophiles. It was shown that the stepwise character of the MCRs themselves and polyfunctional character of that 1,3-binucleophile could lead to at least eight possible products A–H from one set of the starting reagents usually depending on the reaction conditions (and sometimes specific structure of the starting reagents themselves). The pairs A-B, C-D, E-F, and G-F could be considered as positional isomers whereas the pairs A-C, B-D, E-G, and F-H–as regioisomers. As a consequence of such diversity of the possible reaction products their structural elucidation often becomes problematic (Figure 8).
The authors (Sedash et al., 2012) explored the literature dealing with this type of reactions and concluded that the existing data about the structure of the reaction products A–H was not always comprehensive and found that most of the literature sources concerning Biginelli-like MCRs involving 3-amino-1,2,4-triazoles had described structure A as the most usual product under quite harsh conditions and different reagents (acetophenone, ethyl and methyl esters of acetoacetic acid and its fluorinated derivatives, substituted amides of acetoacetic acids, pyruvic acid, 1-(methylsulfonyl)propan-2-one and 2-(methylsulfonyl)-1-phenylethanone, aliphatic ketones). The structure C was sometimes reported as a side product accompanying the formation of the main product A (in the reactions with pyruvic acid or methyl acetoacetate). Only the condensation involving acetylpyrazole derivative afforded compound of structure C as the only product (Ali et al., 2016). Tetrahydroderivatives of type F were described in the reactions under mild conditions (with phenylpyruvic acid or ethyl acetoacetate). Products of E-type could be obtained on the basis of fluorinated esters of acetoacetic acid and further converted into A-type heterocycles thus being the products of kinetically and thermodynamically controlled reactions. The products of structure B could be formed when two molecules of acetophenone (or cyclohexanone, see compound 14, Figure 2) reacted with 3-amino-1,2,4-triazole derivatives. The products D were not obtained by Biginelli-like MCRs, they could be synthesized using other approaches whereas products of structure G and H were not described at all (Figure 8; Sedash et al., 2012).
The conclusions of Sedash et al. about preferential formation of compounds with the structure of type A in the multicomponent reactions involving 3-amino-1,2,4-triazole were confirmed by the subsequent publications of other authors: 4,7-dihydroazolo[1,5-a]pyrimidines were synthesized from 3-amino-1,2,4-triazole or 5-aminotetrazole, aromatic aldehydes and acetone, α-acetyl-butyrolactone, acetylacetone or acetoacetic acid derivatives (Ryabukhin et al., 2011; Gein et al., 2012, 2014; Kumari et al., 2012; Li et al., 2012; Liu et al., 2012b; Rajua et al., 2012; Ghorbani-Vaghei et al., 2013; El Rady, 2014; Haleel et al., 2014; Bhatt et al., 2015; Adrom et al., 2016; Komykhov et al., 2016, 2017; Gümüş et al., 2017b; Kour et al., 2017; Maleki et al., 2017).
An example illustrating the dependence of the reaction direction on the conditions is given in work of Muravyova et al. (2011) Varying temperature and using ultrasonic activation allowed to switch MCR involving acetoacetamides 98b (R1 = NHAr, R2 = CH3), aromatic aldehydes 1 and substituted 3-amino-1,2,4-triazole 97 (R3 = SAlk, SCH2Ar) between kinetically- and thermodynamically-controlled directions and selectively obtain tetrahydro- or dihydroderivatives 99 or 100, correspondingly. Later on Wang et al. (2015a) expanded the list of dihydropyrimidines 100 by using other substituted triazoles 97 (R3 = H, NH2, CO2Alk, SCH2CONH2, SCH2CO2C2H5, SCH2CH2NHCO2CH2Ph) and acetoacetic acid derivatives 98 (R1 = OAlk, NH2, NHAr, NHHetAr, R2 = CH3; Figure 8).
It is worth noting that the analogous behavior of 5-aminopyrazoles substituted in the fourth position with electron-withdrawing groups [CN, CO2CH3, CONH2 (Muravyova et al., 2011)] in the condensations being similar to the described above and leading to tetrahydro- and dihydropyrimidines of types 99 and 100 as well.
Dihydropyrimidine systems 102a–e (Figure 8) were also formed in condensation of acetoacetic acid and its derivatives (or acetylacetone) 98 with 2-aminobenzimidazole (101a) [under neat conditions-ionic liquid catalyzed, US, 50°C (Reddy et al., 2016) etc. (Ryabukhin et al., 2011; Shaterian et al., 2014; Kaur N. et al., 2015; Abedini et al., 2016; Dam et al., 2016)], 2-aminoimidazoles 101b [DMF-(CH3)3SiCl, US, r.t. (Ryabukhin et al., 2011)], 2-aminobenzothiazole (101c) (MeOH-HCl, Δ (Chikhale et al., 2015) etc. (Zhao et al., 2013; Atar et al., 2014; Shaterian et al., 2014; Moradi et al., 2015), 2-aminothiazole 101d [HOAc, MW, 80°C (Zhao et al., 2013) etc. (Batool et al., 2016; Dam et al., 2016; Tan et al., 2016)] and 2-amino-1,3,4-thiadiazole 101e (HOAc, MW, 65°C; Zhao et al., 2014).
It should be noted, that Thorat et al. (2013) obtained imidazopyrimidines (EtOH-ionic liquid catalyzed, r.t.) with another positional orientation of substituents than in compounds 102b. However, there was not enough data (2D NMR experiments or X-Ray analysis) proving that structure while the structure of azolopyrimidines 102 was proven with the help of X-Ray analysis in cases of heterocycles 102d (Zhao et al., 2013) and 102e (Zhao et al., 2014).
Formation of pyrimidines 105a and 106d,e was also observed in the reactions with the CH-acids 103: malononitrile (103a) (X = CN) or ethyl 2-cyanoacetate (103b) (X = COOC2H5). The condensation of aldehydes 1, malononitrile (103a) and 2-aminobenzimidazole (101a) under the variety of conditions [neat, poly(vinylpyrrolidonium) perchlorate catalyzed, 100°C (Abedini et al., 2016); neat-p-TSA(10%), 80°C (Reddy et al., 2014b); EtOH-Fe3O4@IM, Δ (Hemmati et al., 2016); PEG-H2O (4:1), Δ (Survase et al., 2017)] afforded dihydrobenzo[4,5]imidazo[1,2-a]pyrimidines 105a. When 2-aminothiazoles 101d and 2-amino-1,3,4-thiadiazoles 101e reacted with aldehydes 1 and malononitrile (103a) or ethyl 2-cyanoacetate (103b), [EtOH-H2O, MW, 100°C (Sahi and Paul, 2016)] pyrimidines 106d,e were isolated (Figure 9).
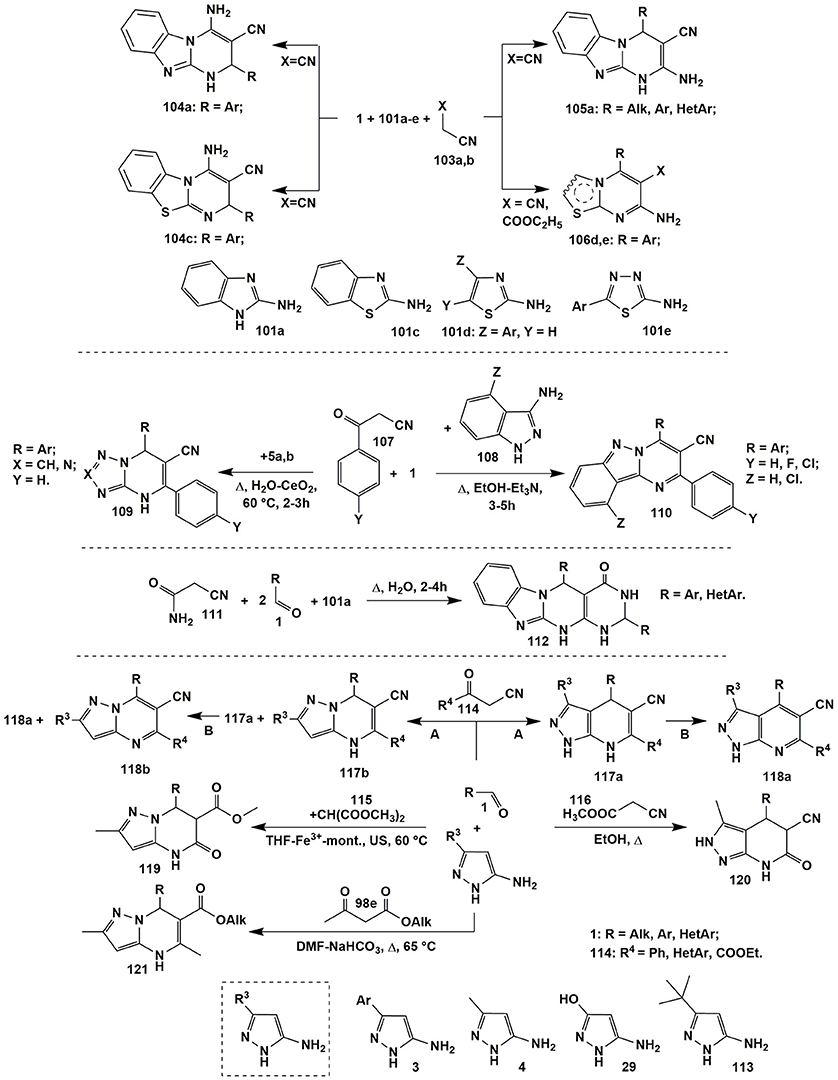
Figure 9. Examples of cyclizations involving α-aminoazoles, aldehydes and non-cyclic carbonyl compounds.
On the other hand, a lot of earlier publications stated that the MCR of malononitrile (103a) or ethyl 2-cyanoacetate (103b) and aromatic aldehydes 1 with 2-aminobenzimidazole (101a) or 2-aminobenzothiazole (101c) afforded the products 104a (EtOH-alum, 70°C (Karimi and Bayat, 2011); H2O, Δ (Risley et al., 2014); neat with alkyl disulfamic acid functionalized magnetic nanoparticles, 90°C (Bodaghifard et al., 2016) and 104c [MeCN-MgO, Δ (Sheibani and Babaie, 2013)] with the other positional orientation than in heterocycles 105a and 106d,e. Since a lot of authors provided no sufficient experiment to prove the stated structures the data described above was contradictory. For example, some publications operate with 2D NMR studies: Hemmati et al. (2016) observed NOE between the signals of pyrimidine CH and aromatic benzimidazole protons and indicated on the structure 105a, whereas Karimi and Bayat (2011) didn't observe such NOE correlation and suggested the formation of isomeric structure 104a. Some authors on the basis of references for similar compounds having the results of the X-Ray analysis indicated on the formation of isomer 104a as well.
Similar to compounds 106 heteroaromatized pyrazolopyridines were obtained in the condensations of malononitrile (103a) and arylglyoxals 83 both with 5-amino-3-methyl(aryl)-1-phenylpyrazole and N-unsubstituted 5-amino-3-methylpyrazole (Petrova et al., 2016).
A facile and efficient cascade reaction of 3-oxo-3-arylpropanenitrile 107 and aromatic aldehydes 1 with substituted 1H-indazol-3-amines 108 upon refluxing in EtOH-Et3N medium (3–5 h) under metal-free conditions afforded pyrimido[1,2-b]indazole-3-carbonitrile derivatives 110 (Li et al., 2017) while their dihydro analogs 109 (Suresh et al., 2016) were synthesized in the condensation of the starting reagents 1 and 107 with 3-amino-1,2,4-triazole (5a) (X = CH) or 5-aminotetrazole (5b) (X = N) under heating in water with adding of nano CeO2 catalyst (60°C, 2–3 h). Tetracyclic derivatives 112 were formed in the reaction involving 2-cyanoacetamide (111) with 2-aminobenzimidazole (101a) and aldehydes 1 followed by the further cyclization with the second equivalent of aldehyde 1 by heating in water for 2–4 h (Liu et al., 2012a) or at room temperature in polyethylene glycol for 1 h (Figure 9; Reddy et al., 2014a).
The introduction of 1- and 4-unsubstituted 5-aminopyrazoles (3, 4, 29 or 113) to the reactions with aldehydes and CH-acids enables the formation of the regioisomers. Thus, 4,7-dihydropyrazolopyridines 117a were the products of condensation of 5-aminopyrazoles 3, 4 or 29 and aldehydes 1 with substituted 3-oxopropanenitriles 114 (A: DMF-Et3N, Δ). The volatile substances were removed from the reaction mixture and the residue was oxidized with sodium nitrite in acetic acid (B), which resulted in isolation of pyrazolopyridines 118a (Hill, 2016). Regioisomeric pyrazolopyrimidines 117b were formed under the same conditions when 5-aminopyrazoles contained a sufficiently large substituent R3 at position 3 (for example, tert-butyl in compound 113) which complicated the electrophilic aromatic substitution with the participation of the C4 nucleophilic center in the aminopyrazole 113 and led to cyclization into compounds 117b. The authors (Hill, 2016) also discovered the steric influence of an aldehyde component 1 on the ratio of products 118a and 118b in the mixture (Figure 9). Analogous to heterocycles 117a dihydropyrazolopyridines were isolated as a result of heating the compounds 1, 4 and 114 in ethanol with adding Fe(III)-montmorillonite (Mamaghani et al., 2013).
When methyl cyanoacetate 116 was introduced into the reaction with compounds 1 and 4 under refluxing in ethanol (Mahdavinia and Rahmati, 2015) or ethanol with p-TSA (Rahmati, 2010) 6-oxo-4,5,6,7-tetrahydro-2H-pyrazolo[3,4-b]pyridines 120 were obtained. Isomeric pyrazolopyrimidinones 119 (Hossein Nia et al., 2014) were isolated in the condensation involving dimethyl malonate 115 under ultrasonication in THF with adding of Fe3+-montmorillonite (Figure 9). Cyclizations involving acetoacetic acid derivative 98e proceeded involving endocyclic amino group of 5-aminopyrazole 4 with the formation of dihydropyrimidines 121 (Finlay et al., 2012, 2013).
Steric and electronic effects of a substituent R in aldehyde 1 significantly influenced the ability to oxidation of dihydropyrimidine cycle in the condensations involving 5-amino-3-methylisoxazole (60a) and aromatic aldehydes 1 with N-arylacetoacetamides 98c. Thus, under identical conditions (n-BuOH, Δ, oxygen of air) dihydropyridines 122 were isolated only in case of para-halogeno- and ortho-substituted aldehydes 1. The authors (Tkachenko et al., 2014b) associate this with the electronic influence of the halogenaryl moiety or the steric effect of ortho-substituents, which complicates the oxidation of heterocycles 122 to 123. In case of other aldehydes such conditions led to the formation of heteroaromatized systems 123. Only carrying out the reaction in the argon atmosphere afforded isolation of dihydropyridines 122 (except hydroxy-substituted ones). However, blowing oxygen through their ethanol solutions led to the transformation of compounds 122 to 123 (Figure 10).
The influence of substituents on the direction of a reaction involving asymmetric 1,3-diketones 125, aromatic aldehydes 1 and 5-amino-1-aryl-3-methylpyrazoles 124 was also significant. The regioselectivity of the formation of aromatic pyrazolopyridines 126 was caused by a greater electrophilicity of COCF3 than COAr-carbonyl group. However, for some combinations of substituents in 5-aminopyrazole 124 and aldehyde 1 dihydropyrazolopyridines 127 without trifluoroacetyl moiety were formed (Figure 10; Gunasekaran et al., 2013).
When 5-amino-3-methyl-1-phenylpyrazole (21) reacted with aldehydes 1 and other CH-acids (acetoacetic acid derivatives), e.g., 98e,f (Fan et al., 2016), 3-oxo-3-phenylpropanenitrile 107 (Huang et al., 2011; Rahmati and Khalesi, 2012) heteroaromatic pyrazolopyridines 128 (similar to compounds 126) and 129 (similar to compounds 118a, Figure 8) were formed (Figure 10).
When acetophenones 130 were used as CH-acids in condensations with aldehydes 1 and different aminoazoles [3-amino-1,2,4-triazole (5a), 5-aminotetrazole (5b), 2-aminobenzimidazole (101a), 5-aminopyrazole and 3-aminoindazole] two types of products were formed–azolopyrimidines of types 132 (Palaniraja et al., 2016a) and 134 (Palaniraja et al., 2016a) or their dihydroanalogues 131 (Ghorbani-Vaghei et al., 2013; Hassaneen and Farghaly, 2015; Kour et al., 2017) and 133 (Hassaneen and Farghaly, 2015; Figure 10).
Conditions for the obtaining thiazolidin-4-ones 138 from aldehydes 1, different aminoazoles and thioglycolic acid (137) were dependent on the origin of aminoazole. Thus, for 3-amino-1,2,4-triazole 5a (Ebrahimi, 2016) the cyclization was performed under solvent-free conditions with addition of ammonium persulfate as a catalyst (Δ, 90°C, 1 h); for 2-aminobenzimidazole (101a) (Kumar et al., 2013) and 2-aminobenzothiazole (101c) (Kumar et al., 2013)–in toluene with addition of HClO4-SiO2 catalyst (Δ, 100°C, 3–6 h); for 2-aminothiazole (101d) (Wu et al., 2014)–in toluene (Δ, 140°C) and for 3-amino-5-methylisoxazole (135) (Murugesan et al., 2014)–in toluene (Δ, 140°C, ~24 h). Application of 1- and 3-substituted 5-aminopyrazoles 136 (Abonia, 2014) with the reagents 1 and 137 under solvent-free conditions and self-catalysis with thioglycolic acid (137) afforded cyclic pyrazolo[3,4-e][1,4]thiazepin-7(4H)-ones 139 to be isolated (Figure 10).
A special attention should be also paid to the multicomponent reactions of aminoazoles and aromatic aldehydes with pyruvic acid and its derivatives, especially because of the ambiguity in the realization of directions of such processes. Chebanov's group (Chebanov et al., 2005, 2007a, 2012a; Sakhno et al., 2008, 2010, 2011, 2015) contributed a lot to studying both stepwise and MCR reactions involving pyruvic acid and aminoazoles and showed that their chemo- and regioselectivity, positional orientation of the substituents in the final products significantly depend on the reaction parameters and structure of the starting reagents.
Thus, in the heterocyclizations involving 3-amino-1,2,4-triazole (5a) and aromatic aldehydes 1 with pyruvic acid (140a) (R1 = H) dihydrotriazolopyrimidines 144 (Chebanov et al., 2005) with the same positional orientation as for acetoacetic acid reaction (heterocycle 100, Figure 8) were formed (HOAc, Δ, 4 h). When compounds 1, 5a, and 140a were refluxed in DMF dihydrotriazolopyrimidine 144 was obtained in a mixture with regioisomer 145 (which was impossible to isolate in a pure state). Later on it was found that prolonged heating of compounds 1, 5a, and 140a (HOAc, Δ, 65°C, 48 h) also afforded tetrahydroderivatives 143 (Murlykina et al., 2015) that could be converted into dihydropyrimidines 144 after refluxing in HOAc for 4 h (Figure 11).
Temperature regime was also crucial in the condensations involving arylpyruvic acid 140b (R1 = Ar): (Sakhno et al., 2008, 2010; Murlykina et al., 2015), tetrahydropyrimidines 141 were yielded under the room temperature conditions (HOAc, US, r.t., 30 min) while pyrrolones 142 were obtained at elevated temperatures (HOAc, MW, 170°C, 20 min). Authors also carried out the transformation of compounds 141 into 142 (HOAc, MW, 170°C, 40 min) and proved the formation of compounds 141 and 142 under kinetic or thermodynamic control, respectively (Figure 11).
Heterocyclizations involving 5-aminopyrazolecarboxamides (Chebanov et al., 2007a) under the same conditions afforded products of types 141, 142 and 144 that again indicated on the similar behavior of substituted in the position 4 pyrazoles and 3-amino-1,2,4-triazole that had been already mentioned for the condensations with acetoacetic acid derivatives (see Figure 8).
In the contrary, the behavior of 5-aminotetrazole (5b) (X = N, R2 = H) and 1-(4-chlorophenyl)-3,5-diamino-1,2,4-triazole (5c) (X = CNH2, R2 = 4-ClC6H4) was somewhat different. Application of the same conditions (HOAc or DMF, Δ) or (EtOAc-I2, Δ) (Zeng et al., 2011) for the condensation of aldehydes 1, pyruvic acid (140a) and 5-aminotetrazole (5b) gave the analogous to compounds 144 dihydrotetrazolopyrimidines 147 (Chebanov et al., 2005) while in case of 1-(4-chlorophenyl)-3,5-diamino-1,2,4-triazole (5c)–furanones 148 (Sakhno et al., 2011). Pyrrolones 146 (Sakhno et al., 2008) were the high-temperature products of the condensations involving both aminoazoles 5b and 5c with arylpyruvic acids [as in case of 3-amino-1,2,4-triazole (5a)]. All the attempts to isolate dihydropyrimidine acids of type 147 on the basis of 3,5-diaminotriazole 5c were unsuccessful that was explained (Sakhno et al., 2011) by the loss of aromaticity in azole cycle during the formation of the fused fragment (Figure 11).
A large number of pyrrolones was also synthesized by Ryabukhin et al. (2012) in the reactions of ethyl 2,4-dioxo-4-arylbutanoates with aldehydes and 2-aminobenzothiazole, 2-aminothiazole, 2-amino-1,3,4-thiadiazole, 5-amino-1,2,4-thiadiazole, 3-amino-5-methylisoxazole, 3-amino-4-methyl-1,2,5-oxadiazole.
Condensation of pyruvic acid (140a) or its esters 149 and aldehydes 1 with 5-amino-3-aryl(alkyl)pyrazoles 150 afforded heteroaromatic pyrazolopyridine acids 152 (Cowen et al., 2016) and 154 (Chebanov et al., 2007a) (HOAc, Δ), their esters 151 [HOAc, Δ (Chebanov et al., 2007a) or EtOH-HCl, Δ (Dias et al., 2007)], correspondingly. It's interesting to note, that in case of 5-amino-1-phenyl-3-(pyridine-3-yl)-1H-pyrazole El-Borai et al. (2012) obtained pyrazolopyridines 153 (HOAc, MW, 160°C, 20 min) with different positional orientation of substituents than in the products 151, 152, 154 (Figure 11).
Introduction of arylpyruvic acid 140b into the same high-temperature reaction yielded no pyrrolone of type 142 but led to the tarring the reaction mixture. Only reducing temperature together with applying ultrasonic activation allowed to synthesize tetrahydropyrazolopyrimidines 156 and 157 (Murlykina et al., 2013; similar to compounds 141) in cases of 4-ethyl-5-amino-3-(4-fluorophenyl)pyrazole 155 and 5-amino-3-aryl-pyrazole 3 (HOAc, US, r.t., 60 min; Figure 11).
The analogous tetrahydropyrazolopyrimidines 160 (Sakhno et al., 2018) were synthesized in the reaction of 3-aryl-4-alkyl-substituted 5-aminopyrazoles 158 and aromatic aldehydes 1 with alkyl pyruvates 149 in acetic acid at room temperature. At the same time refluxing compounds 1, 149 and 158 in acetic acid for 7 h led to the formation of a pyrimidine ring followed by an oxidative heteroaromatization process which gave 6-hydroxy-substituted alkyl pyrazolopyrimidine-5-carboxylates 159 (Sakhno et al., 2018). That was explained by disproportionation process; it was confirmed by carrying out this reaction in the inert atmosphere (where neither dihydropyrazolopyrimidine nor the compound without a hydroxyl group was observed). As it was expected, heterocycles 160 were transformed into heteroaromatic derivatives 159 upon boiling in acetic acid for 9 h (Figure 11).
Post-cyclizations can serve as an additional source of molecular diversity in the MCRs. They occur, for example, when salicylaldehyde is used in the MCRs. Thus, Gorobets et al. (2010) by varying temperature in the MCR of salicylaldehyde 162 with acetone (161) and 3-amino-1,2,4-triazole (5a) yielded both bridged benzoxadiazocines 164 (MeOH-HCl, MW, 150°C, 30 min) and tetrahydroderivatives 165 (CH3OH-HCl, Δ, 40°C). Later on several groups (Kondratiuk et al., 2016; Gümüş et al., 2017a; Komykhov et al., 2017; Aydemir et al., 2018) studied the aspects of these transformations in details. It's interesting, that condensation involving 5-amino-3-arylpyrazoles 3, salicylaldehydes 162 and pyruvic acid (140a), unlike the MCR with 3-amino-1,2,4-triazole (5a), afforded bridged benzoxazocines 163 at room temperature (HOAc, US, 90 min) whereas microwave heating at 150°C led to heteroaromatized pyrazolopyridines of type 152 (Figure 12).
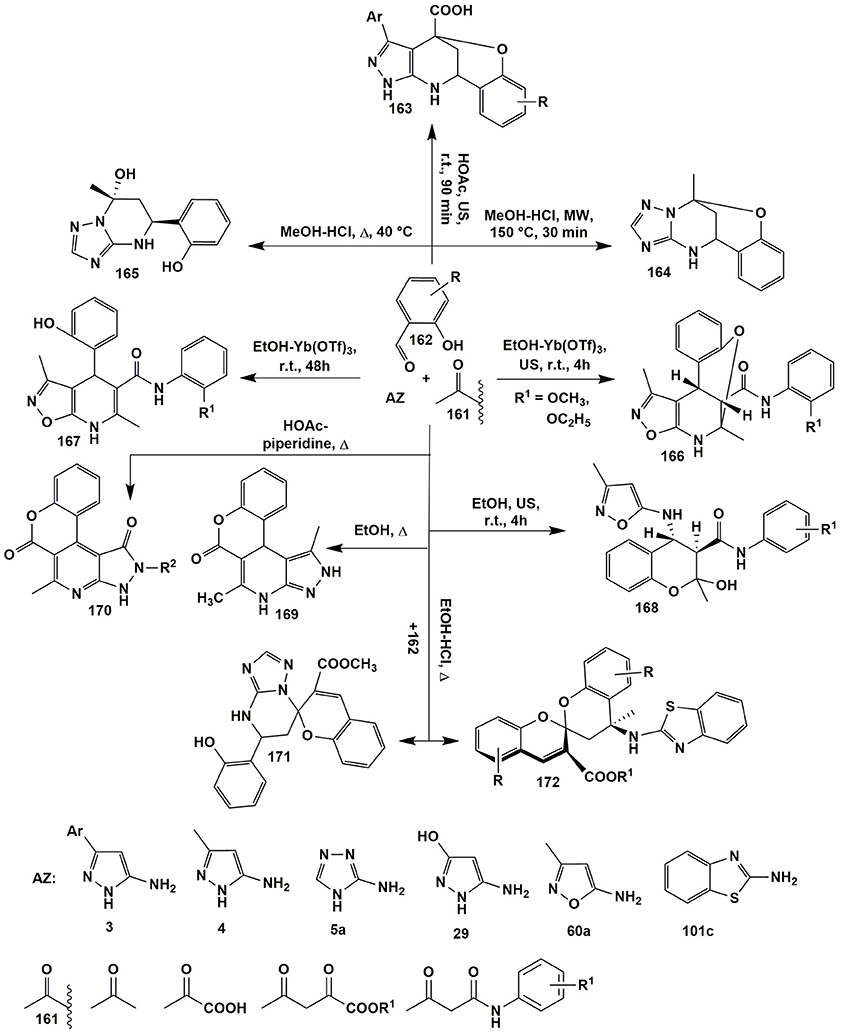
Figure 12. Some post-cyclizations serving as an additional source of molecular diversity in MCRs involving salicylic aldehydes, aminoazoles and active methylene compounds.
Varying the conditions of the reaction and structures of the starting reagents afforded to synthesize three different classes of compounds 166–168 from the same reagents (Tkachenko et al., 2014a). Ultrasonication of 5-amino-3-methylisoxazole (60), N-aryl-3-oxobutanamide (161) and salicylaldehyde (162) afforded N-aryl-4-(3-methylisoxazole-5-ylamino)chromane-3-carboxamides 168. Stirring N-aryl-3-oxobutanamides 161 with R1 = 2-CH3O- or 2-C2H5O- and compounds 162 and 60 in the presence of Yt(OTf)3 redirected the condensation toward the formation of dihydroisoxazolopyridines 167, whereas ultrasonication led to benzoxazocines 166. This was almost an exceptional case when the replacement of the usual stirring by ultrasonic activation under other identical conditions led to the formation of different compounds (Figure 12; Tkachenko et al., 2014a).
It's worth to note, that in case of other substituted N-aryl-3-oxobutanamides 161 (R1 = 2-OH, 2-CH3, 2-Cl, 3-Cl) only heterocycles 167 were isolated both with the help of mechanical stirring and under ultrasonication. The authors (Tkachenko et al., 2014a) suppose that the direction leading to benzoxazocines 166 is favored by the formation of 3-coordinated complex of Yt(OTf)3 with NH- and CH3O(C2H5O)-groups of carboxamide fragment and OH-group of intermediate tetrahydroisoxazolopyridine. In turn, ultrasound supplies to the reaction system a sufficient amount of energy that is needed for nucleophilic substitution and bridged moiety formation (Figure 12).
o-Hydroxyl group of aldehyde 162 can also participate in the formation of lactones of types 169 (Svetlik et al., 2010) and 170 (Frolova et al., 2011), that were synthesized in the condensation of salicylaldehyde (162), 5-aminopyrazoles 4 or 29 and acetoacetic acid esters 161. Heterocyclization of compound 161 with a double excess of salicylaldehyde (162) and 3-amino-1,2,4-triazole (5a) or 2-aminobenzothiazole (101c) gave spiroheterocycles 171 (Světlík and Kettmann, 2011) and 172 (Svetlík et al., 2014); 2-aminobenzimidazole reacted with an exocyclic amino-group as a mononucleophile (Figure 12).
In the multicomponent reaction of 5-amino-3-methylisoxazole (60a) and salicylaldehydes 162 with 1,3-cyclohexanediones 2 Muravyova et al. (2013) synthesized several heterocycles 173–175 depending on the conditions. In case of 2-aminobenzimidazole (101a) condensation with the reagents 2 and 162 under the different conditions (heating in toluene with addition of K2CO3 or heating in chloroform with addition of sulfamic acid) resulted exclusively in xanthene-1-ones 176. Only the stepwise transformation involving the preliminary synthetized imine 177 with dimedone (2b) afforded tetrahydrobenzo[4,5]imidazo[2,1-b]chromeno[4,3,2-de]quinazolines 178 (Saeedi et al., 2011; Figure 13).
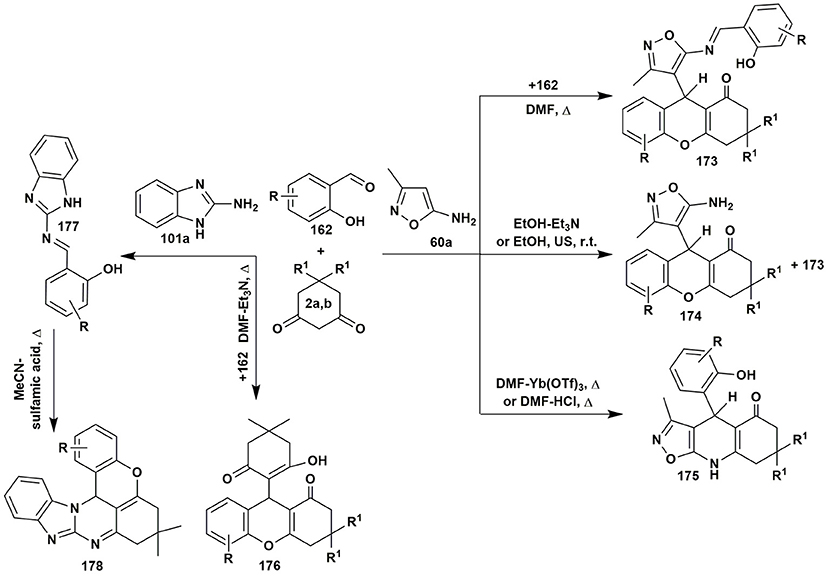
Figure 13. Condition-based divergence strategy in MCRs of aminoazoles with salicylic aldehydes and cyclic active methylene compounds.
Other Multicomponent Reactions of Aminoazoles
Isocyanide-based reactions may be separated into an individual large group and certainly should be described in special reviews, a lot of brilliant examples of which have already been published (Dömling and Ugi, 2000; Banfi et al., 2010; Ruijter et al., 2011; Dömling et al., 2012; Cioc et al., 2014; Koopmanschap et al., 2014; Devi et al., 2015; Zarganes-Tzitzikas et al., 2015; Shaaban and Abdel-Wahab, 2016). As it's recognized the classical components of the Ugi four-component reaction (Ugi-4CR) are aliphatic or aromatic amines and aldehydes, carboxylic acids and substituted isocyanides, that are generally well responsive to the formation of Ugi products at room or slightly elevated temperatures (Dömling and Ugi, 2000; Dömling, 2006). Groebke-Blackburn-Bienaymé three-component reaction (GBB-3CR) usually undergoes with participation of 2-aminoazines or 2-aminoazoles, aromatic or aliphatic aldehydes and substituted isocyanides. Brønsted or Lewis acids are often used in GBB-3CR (sometimes in Ugi-4CR) for activation of intermediate imine. Almost all the types of solvents (including water and ionic liquids) and catalysts, different temperature regimes (conventional or microwave heating) were studied in GBB-3CR (Figure 14; Devi et al., 2015).
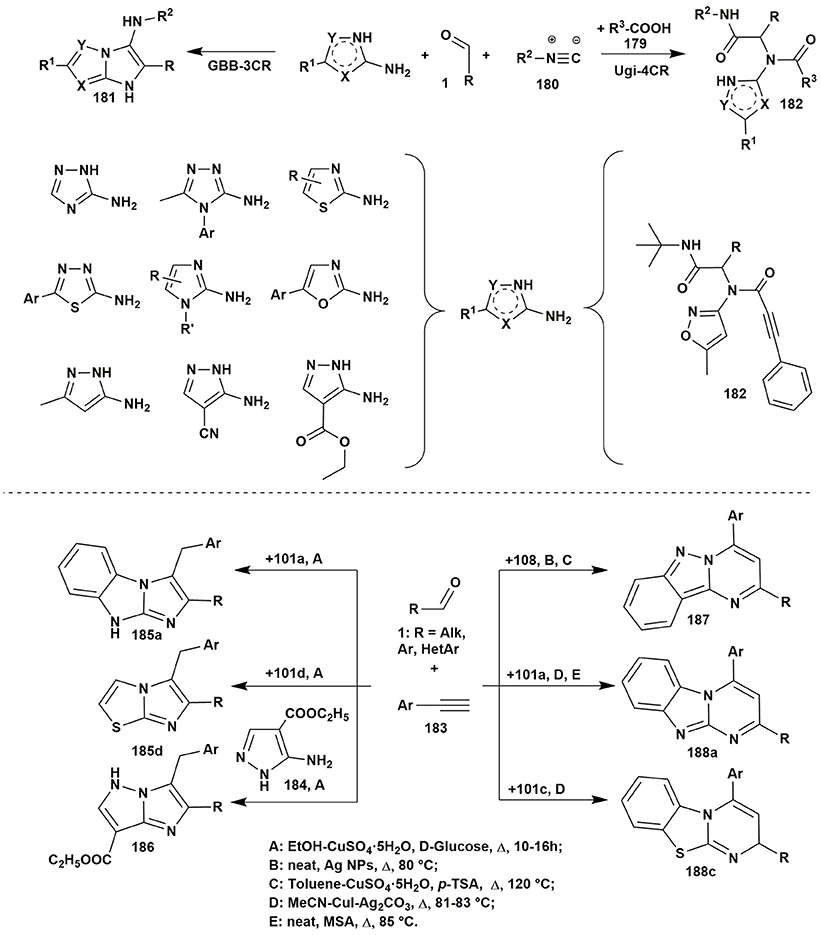
Figure 14. Examples of the application of aminoazoles in GBB-3CR, Ugi-4CR and A3 coupling reactions.
There are a lot of examples of using aminoazoles as an amine component in GBB-3CR resulting in the formation of heterocycles like 181. The most studied ones are the processes involving 3-amino-1,2,4-triazoles (Bienaymé and Bouzid, 1998; Tyagi et al., 2012; Urich et al., 2013; Aouali et al., 2015), 2-amino(benzo)thiazoles (Bienaymé and Bouzid, 1998; Guchhait and Madaan, 2009, 2010; Guchhait et al., 2009; Al-Tel et al., 2010; Akritopoulou-Zanze et al., 2011; Baviskar et al., 2011; Burchak et al., 2011; Hieke et al., 2012; Tyagi et al., 2012; Vidyacharan et al., 2014; Martinez-Ariza et al., 2015; Ansari et al., 2016; Shaabani and Hooshmand, 2016; Shao et al., 2017), 2-amino-1,3,4-thiadiazoles (Krasavin et al., 2008; Guchhait and Madaan, 2009; Guchhait et al., 2009; Wadhwa et al., 2015), 2-amino(benz)imidazoles (Lee et al., 2013; Pereshivko et al., 2013). GBB-3CR involving 2-aminooxazoles (Bienaymé and Bouzid, 1998) led to the formation of imidazoazoles while involving 1,2,5-oxadiazole-3,4-diamine (Kysil et al., 2010) gave oxadiazolopyrazines. Groebke condensations of 5-aminopyrazoles (5-amino-3-methylpyrazole, 5-aminopyrazole-4-carbonitrile, ethyl 5-aminopyrazole-4-carboxylate) are described in the following publications (Bienaymé and Bouzid, 1998; Guchhait and Madaan, 2009; Guchhait et al., 2009; Baviskar et al., 2011; Rahmati and Kouzehrash, 2011; Rahmati et al., 2013; Demjén et al., 2014; Murlykina et al., 2017).
Although there are a lot of variations and modifications known for the Ugi-4CR there is only one example of using aminoazole as an amine component in this reaction, which includes the treatment of 3-amino-5-methylisoxazole, aromatic aldehydes, phenylpropiolic acid and tert-butylisocyanide with formation of Ugi-product 182 (Figure 14; Murlykina et al., 2017).
Several publications deal with A3 coupling reactions between aromatic aldehydes 1, aryl acetylenes 183 and aminoazoles resulting in the formation of two types of products–via multicomponent assembly reaction through 6-endo-dig (heterocycles 187, 188) or through 5-exo-dig cyclization (heterocycles 185, 186). Thus, imidazoazoles 185a,d and 186 were synthesized via method A [EtOH-CuSO4·5H2O, D-glucose, Δ, 10–16 h (Guchhait et al., 2012)] on the basis of 2-aminobenzimidazole (101a), 2-aminothiazole (101d) and ethyl 5-aminopyrazole-4-carboxylate (184) via A3-coupling reaction followed with 5-exo-dig cycloisomerization and prototropic shift (Figure 14).
At the same time, coupling involving 2-aminobenzimidazole (101a) (or 2-aminobenzothiazole 101c) under other conditions [MeCN-CuI-Ag2CO3, Δ, 81–83°C (Kumar et al., 2014)] or [neat, MSA (molybdate sulfuric acid), Δ, 85°C (Shinde and Jeong, 2015)] led to formation of the benzimidazolopyrimidines 188a (or benzothiazolopyrimidines 188c). Carrying out the reaction involving 1H-indazol-3-amine (108) under neat conditions [Ag NPs (nanoparticles), Δ, 80°C (Balwe et al., 2017)] or in toluene [CuSO4·5H2O, p-TSA Δ, 120°C (Palaniraja et al., 2016b)] resulted in multicomponent assembly reaction through 6-endo-dig cyclization and formation of pyrimido[1,2-b]indazoles 187 (Figure 15).
A four-component strategy for the selective synthesis of fused azepino[5,4,3-cd]indoles 189 and pyrazolo[3,4-b]pyridines 190 was elaborated by Jiang et al. (2014). The direction of multicomponent reaction involving 1,3-substituted 5-aminopyrazoles 150 and amines 55 with two equivalents of arylglyoxals 83 (DMF-p-TSA, MW, 115°C) was dependent on the electronic effects of arylglyoxals and aromatic amines (Figure 15).
Reddy et al. (2017) developed a highly active and stable heterogeneous POEGMA-g-TEGBDIM (polyethylene glycol methacrylate-grafted tetraethyleneglycol-bridged dicationic imidazolium-based IL) catalyst for the synthesis of substituted benzo[4,5]imidazo[1,2-a]pyrimidine heterocycles 192 upon solvent-free conditions (80°C) from 2-aminobenzimidazole (101c), aromatic aldehydes 1 and (E)-N-methyl-1-(methylthio)-2-nitroethenamine (191) (Figure 15).
A synthetic pathway to access fused pyrazolo[3,4-d]pyrimidine-6(7H)-thiones 194 by the three-component reaction of 5-amino-3-methylpyrazole (4), aldehydes 1 and arylisothiocyanates 193 in an ionic liquid in the presence of catalytic amounts of p-TSA was elaborated by Safaei et al. (2012; Figure 15).
Application of heterogeneous solid base, silica sodium carbonate (SSC) as a catalyst allowed isolation of dimethyl 4,5-dihydrotriazolopyrimidine-6,7-dicarboxylates 196 in the MCR of dimethyl acetylenedicarboxylate (195) with 1 and 5a. The authors (Karami et al., 2015b) suggested that the base favors the formation of an intermediate product of condensation between nucleophilic NH in the position 2 of 3-amino-1,2,4-triazole (5a) and electrophilic CH-center of dimethyl acetylenedicarboxylate (195) followed by the attack of aldehyde 1, cyclization and dehydration (Figure 15).
In some cases, synthesis of starting materials for MCRs is also a difficult task. For example, the formation of acetoacetamide building-block by synthetic methods is an expensive and difficult procedure. Therefore, to avoid laborious stage of acetoacetamide synthesis, as a continuation of the work of Shaabani et al. (2009) four-component procedure for obtaining N,7-disubstituted-5-methyl-4,7-dihydrotetrazolo[1,5-a]pyrimidine-6-carboxamides 199 (Zeng et al., 2012) was elaborated. It consisted of the reaction of primary amines 198, diketene 197, 5-aminotetrazole (5b) and aldehydes 1 (EtOAc-I2, Δ, 78°C, 4 h). In this MCR acetoacetamide was formed in situ by the addition of amine to diketene molecule (Figure 15).
Two-Component Condensations of Aminoazoles
To the best of our knowledge the condensations of aminoazoles with α,β-unsaturated carbonyl compounds 200 could be performed as one of the simplest and effective ways to the diverse azoloazine systems, such as 203, 204, since this type of starting materials usually contains alternative nucleophilic and electrophilic reaction centers. The most utilized α,β-unsaturated carbonyl compounds in such reactions are chalcones or cinnamic acid derivatives. The condensations of the enones with aminoazoles could be performed in various solvents within wide range of the temperatures and with application of different types of catalysis (Kolos et al., 2011; Yoshida et al., 2011; Orlov and Sidorenko, 2012; Figure 16).
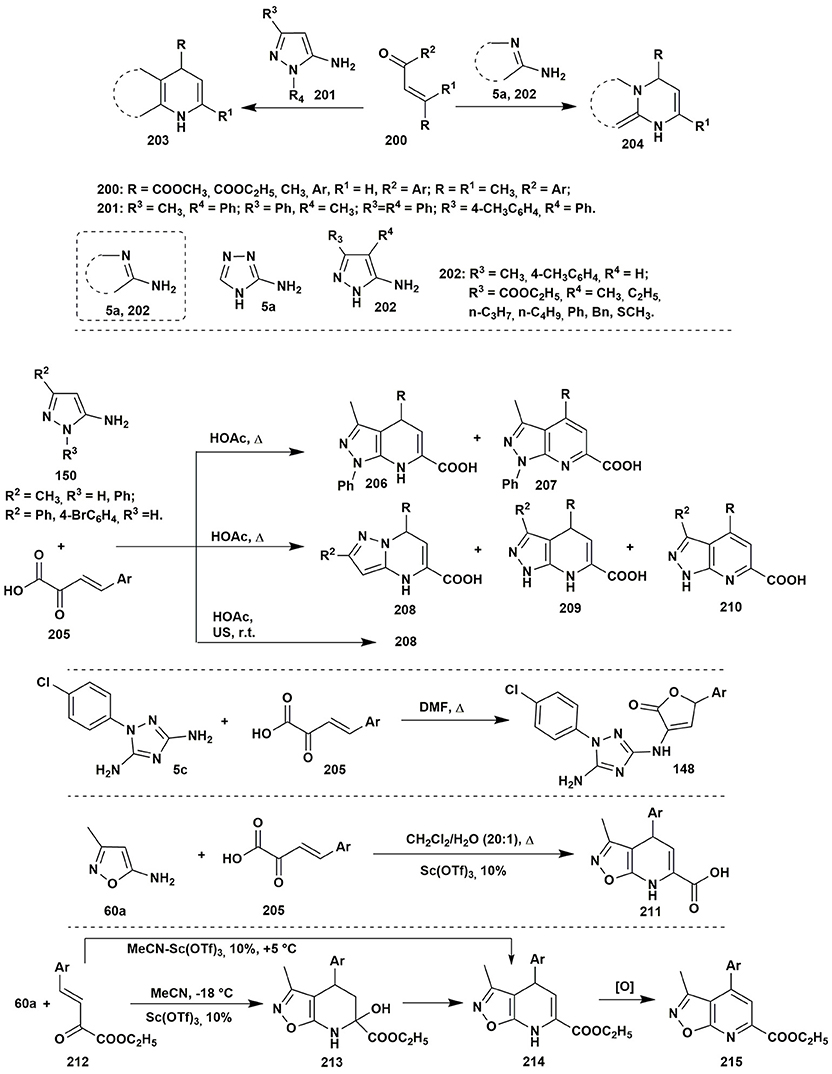
Figure 16. Examples of two-component heterocyclizations of the aminoazoles with α,β-unsaturated carbonyl compounds.
Two-component heterocyclizations of the aminoazoles could be considered as convergent procedures concerning the corresponding multicomponent synthesis, or as independent transformations. Thus, in the previous section of the review it was shown that multicomponent heterocyclizations of the pyruvic acid derivatives with α-aminoazoles and carbonyl compounds could be applied for the synthesis of diverse heterocyclic systems. However, preliminary condensation of the pyruvic acid with aromatic aldehyde gives arylidenepyruvic acids 205 and their further reaction with 5-aminopyrazoles 150 in comparison to the multicomponent procedure allows to obtain different regioisomers 208, 209 (Chebanov et al., 2007a, 2012a). At the same time, in the article (Sakhno et al., 2011) it was shown that the two-component condensation of arylidenepyruvic acid 205 and 1-(4-chlorophenyl)-3,5-diamino-1,2,4-triazole (5c) in DMF resulted in the formation of the same furanones 148 as in the corresponding MCR, however, in smaller yields (Figure 16).
The opposite pattern was observed in case of the 5-amino-3-methylisoxazole (60a) (Morozova et al., 2016). Multicomponent condensation of this aminoazole, pyruvic acid and aromatic aldehyde resulted in the decomposition of the initial amine due to the low stability of the isoxazole moiety in the acidic media. Application of the two-component procedure, via preliminary synthesis of unsaturated acids 205, under Sc(OTf)3 catalysis in CH2Cl2:H2O (20:1) allowed to isolate compound 211 in low yields. An unexpected result was obtained when the unsaturated acid was replaced by the corresponding ethyl ester 212: the condensation of the starting reagents in MeCN containing Sc(OTf)3 at −18°C resulted in the formation of tetrahydroisoxazolopyridine system 213. Typically, such compounds cannot be isolated due to the fast water elimination with the formation of dihydropyridine rings. Indeed, the condensation at higher temperatures led to the formation of dihydropyridine 214, which was further spontaneously oxidized (Figure 16).
The condensation of ethyl arylidenepyruvate 212 with 5-aminopyrazoles 158 in acetic acid without additional catalyst (Sakhno et al., 2018) had a different character and allowed to isolate both pyrazolopyrimidines 159 (under heating) and dihydropyrazolopyrimidines 216 (at room temperature) having OH-group in position 6 (Figure 17). The yields of the product 159 for this two-component condensation were better in comparison to the multicomponent procedure (Figure 11).
3-Methoxalylchromone (218) containing hidden α,β-unsaturated fragment exhibited properties being similar to the derivatives of arylidenepyruvic acid: its condensation with different α-aminoazoles 21, 217a–c resulted in the formation of azoloazines 219 under refluxing in acetic acid or heating in DMF-TMSCl at 80–100°C (Mkrtchyan et al., 2010). Another group studied the condensation of ethyl 5,5,5-trichloro-3-[(dimethylamino)methylene]-2,4-dioxopentanoate (220) with 2-aminothiazole or 2-aminobenzimidazole 101a,d. The starting β-enaminodiketone has two nonequivalent carbonyl groups; however, the condensation with aminoazole is selective through the influence of the trichloromethyl group adjacent to the reaction's site. Nucleophilic attack of the carbonyl carbon atom by the lone electron pair of the endocyclic nitrogen resulted in the elimination of the trichloromethyl group and in the formation of corresponding thiazolo[3,2-a]pyrimidinone and pyrimido[1,2-a]benzimidazole 221 (Campos et al., 2017; Figure 17).
The application of 1,3-dielectrophiles in the azoloazine synthesis is not limited to the enones. β-Dicarbonyl compounds, for example, derivatives of acetylacetone 98 and acetoacetate 225 (Marjani et al., 2015) are used for the formation of the pyrimidine ring with substituents in positions 4 and 6. The asymmetric β-dicarbonyl compounds can produce positional isomers, but often the reactions give only one compound. The aminoazoles with pyrrole N-atom in the α-position to the NH2-group are most often used as 1,3-binucleophiles (Gujjar et al., 2011; Ivachtchenko et al., 2011; Gege et al., 2012; Patnaik et al., 2012; Figure 17).
Among reactions involving β-diketones there is rather interesting condensation of 5-aminopyrazole 158 and dehydroacetic acid 227 (Aggarwal et al., 2014). It was found that the reaction did not stop on the formation of 228: the presence of the reactive toward 5-aminopyrazole acetylacetone fragment induced further condensation with the second molecule of the amine 158 that gave bis(pyrazolo[1,5-a]pyrimidinyl)-7-ones 229 (Figure 18).
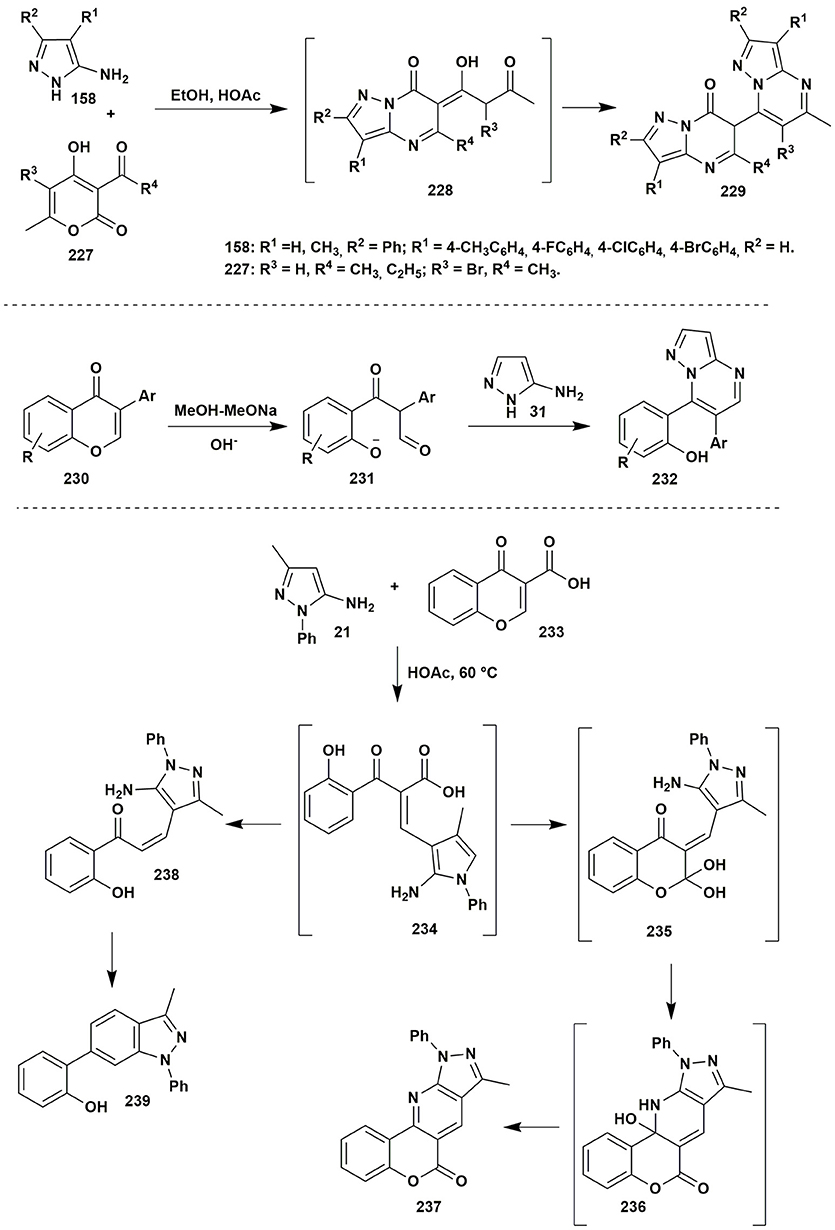
Figure 18. Two-component condensations of aminoazoles with carbonyl compounds containing pyrone moiety.
Despite the fact that compounds containing 4H-chromen-4-one moiety don't have real 1,3-dicarbonyl fragment in the presence of alkali in the reaction mixture the ring-opening process with the generation of the corresponding 1,3-dicarbonyl compound takes place (Zhang et al., 2011). In such way 7-diphenylpyrazolo[1,5-a]pyrimidine derivatives 232 were synthesized by the condensation of isoflavone 230 and 3-aminopyrazole (31) in MeOH-MeONa in moderate to good yields (Figure 18).
Resembling condensation of chromone-3-carboxylic acid (233) and aminopyrazole 21 in acetic acid gave chromeno[4,3-b]pyrazolo[4,3-e]pyridin-6(10H)-one 237 as the major product of the condensation. However, side decarboxylation of the intermediate 234 following with further condensation resulted in the formation of traces of pyrazolo[3,4-b]pyridine 239 (Miliutina et al., 2017). The intermediate 238 was isolated in low yields when the reaction was stopped after 1 h at 60°C (Figure 18).
Quite interesting heterocyclizations of 5-aminotetrazole were reported by Goryaeva et al. (2015): heterocyclization of 5-aminotetrazole (5b) and 2-ethoxymethylidene-3-oxo esters 240, depending on the ester type and/or the condition, could give 2-azidopyrimidines 241 or tetrazolo[1,5-a]pyrimidines 242. The starting materials under refluxing in EtOH or 1,4-dioxane didn't react completely even after long duration of the treatment and resulted in the formation of inseparable mixtures. Carrying out the reaction in the 2,2,2-trifluoroethanol (TFE) gave 2-azidopyrimidines 241 due to the opening of the tetrazole ring. At the same time, 4-methyl-2-azidopyrimidine was not stable and converted into the 242 even while standing as solid on air. The synthesis of the substance 242 could be carried out in EtOH at r.t. from 5-aminotetrazole (5b) and ester 240, the presence of the 241 was indicated by TLC in the reaction mixture, which allows to assume that the reaction could pass through the formation of azide (Figure 19).
Despite the fact that the condensation of 5-aminotetrazole (5b) with the fluorinated reagents in 1,4-dioxane resulted in the mixtures of compounds, the presence of the catalytic amounts of sodium acetate led to the formation of azide 241 with further elimination of the nitrogen and nitrenes that gave ethyl 2-amino-4-(polyfluoroalkyl)pyrimidine-5-carboxylates 243. In case of the CF3-substituted ester the formation of the side product 244 was observed as well. On the other hand, 2-benzoyl-3-ethoxyprop-2-enoate in the condensation with 5-aminotetrazole in TFE under reflux yielded the mixture of compounds 245 and 246 due to the decomposition of the initial ester and the formation of the reacting ethyl benzoylacetate. Application of 2-ethoxymethylidene malonate 240 under refluxing in EtOH allowed to isolate ethyl-7-hydroxytetrazolo[1,5-a]pyrimidine-6-carboxylate 247. Thus, depending on the substituent in 2-ethoxymethylidene-3-oxo esters different tetrazolopyrimidines or pyrimidines were obtained (Figure 19).
Acetoacetic esters may be easily replaced by malonic ester or sodium nitromalonaldehyde monohydrate (Ren et al., 2012). The malonic esters 248 were used as efficient starting materials for the synthesis of the azoloazines 249 substituted in the position 5 (Saito et al., 2011; Figure 20).
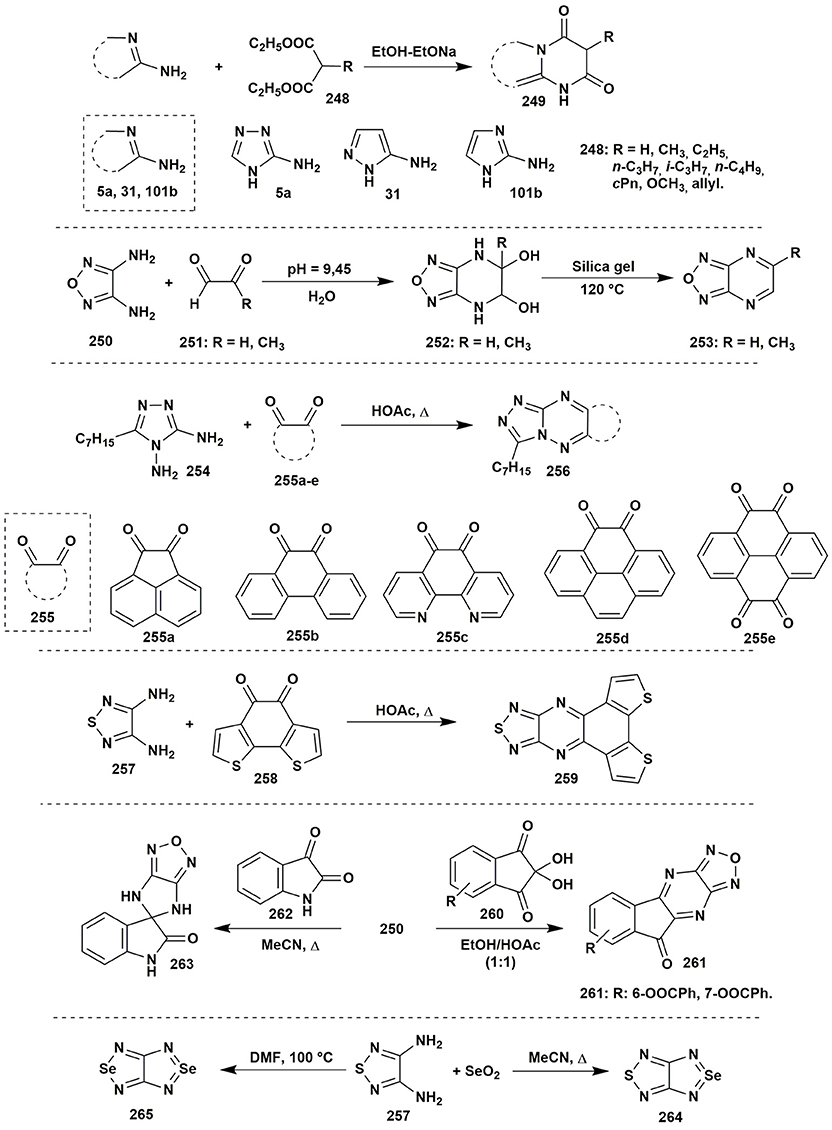
Figure 20. Some two-component transformations of aminoazoles with malonic esters, glyoxals derivatives, hem-diols, isatin, or selenium dioxide.
The condensations of α-dicarbonyl compounds could be carried out with α-diamines as well. An interesting publication was presented by Willer et al. (2012) dealing with short synthetic route to [1,2,5]oxadiazolo[3,4-b]pyrazine moiety. The reaction of diamine 250 and glyoxal or pyruvic aldehyde 251 under mild conditions (45°C, 45 min) yielded 5,6-dihydroxy-4,5,6,7-tetrahydro[1,2,5]oxadiazolo[3,4-b]pyrazine 252 (R = H; conversion 96%) or its unstable analog 252 (R = CH3). Isolation of the pure compound 252 was made by lyophilization or by carrying out the reaction at 20°C. Further pyrolysis on the silica gel gave target [1,2,5]oxadiazolo[3,4-b]pyrazines 253 in low yields (10–33%; Figure 20).
Fusco et al. (2016) applied the condensation between α-diamines 254 and α-diketones 255a–e to obtain triazolo[4,3-b][1,2,4]triazines 256 in 25–95% yield (the lowest yield for a system with two diketone moieties). An analogous result was obtained for 1,2,5-thiadiazole-3,4-diamine 257 (Planells et al., 2014; Figure 20).
The article published by Lauro et al. (2017) presented the possibility to apply hem-diols instead of α-diketones. The condensation of amine 250 with the selected 2,2-dihydroxy-1H-indene-1,3(2H)-diones 260 gave compounds 261 in moderate yields (Figure 20).
Diaminoazoles can be applied not only for synthesis of six-membered heterocycles: the condensation of isatin (262) and 1,2,5-oxadiazole-3,4-diamine (250) in the boiling acetonitrile gave 4,6-dihydrospiro(imidazo[4,5-c][1,2,5]oxadiazol-5,3′-indol)-2′(1′H)-one 263 (Gurevich et al., 2011; Figure 20).
Very unusual application of selenium dioxide as a carbonyl compound in the condensation with 1,2,5-thiadiazole-3,4-diamine (257) giving [1,2,5]selenadiazolo[3,4-c][1,2,5]thiadiazole (264) was described (Konstantinova et al., 2015). As the side reaction an exchange of the sulfur of the thiadiazole was observed: that also allowed to isolate [1,2,5]selenadiazolo[3,4-c][1,2,5]selenadiazole (265). The formation of compound 265 as the major product of the heterocyclization could happen in DMF at 100°C (Figure 20).
The acylation of the vicinal diamines by the acetic anhydride under reflux could give the new azole ring. Thus, Centore et al. (2017) applied the reaction of 3,4-diamino-1,2,4-triazole (266) with acetic anhydride to obtain [1,2,4]triazolo[3,2-c][1,2,4]triazole (267) without isolation of non-cyclic products of acylation (Figure 21).
The condensations of aminoazoles with carbonyl compounds are not limited to the vicinal amines. The azoles with an amide group next to the amine one also can be used as the reagents for the synthesis of pyrimidinones, but the condensation should be promoted by catalysts. Mulakayala et al. (2012) showed that condensation between 4-amino-1H-pyrazole-5-carboxamide (268) and aromatic aldehydes 1 occured without Lewis acid neither at room temperature nor under refluxing, however, the presence of the catalytic amounts of InCl3 promoted the cyclocondensation. Among the studied solvents, the best result was observed in case of MeCN (10% mol of the catalyst) at room temperature. Variation of the solvents (MeOH, i-PrOH, EtOAc, CH2Cl2, CHCl3) or Lewis acids (AlCl3, TiCl4, BF3-OEt2, FeCl3, CuCl2) led to the yields decreasing. Later on another method for synthesis of the similar 1,6-dihydro-7H-pyrazolo[4,3-d]pyrimidin-7-one (270) was performed (Mohammed et al., 2015). Metal-free condensation of aromatic ketones 269 with azole 268 was induced by the molecular iodine (10% mol) with oxygen in DMSO at 110°C and resulted in the formation of the Schiff bases but not the oxidation of the acetophenone to the 2-oxo-2-arylacetaldehyde that was observed in case of 110% I2 excess. Continuous heating promoted further intermolecular condensation with the formation of 1,6-dihydro-7H-pyrazolo[4,3-d]pyrimidin-7-one 270. Attempts to expand the range of the substrates have shown that aliphatic ketones couldn't be used as the reagents; N-substituted amides also did not undergo the condensation under such conditions. Application of the K2S2O8 in acetonitrile–water mixture (1:1) at the room temperature (Hudwekar et al., 2017) allowed to apply the procedure not only for carbonyl compounds, but also for benzylamines or benzyl alcohol via their in situ oxidation. The formation of 1,5-dihydro-4H-pyrazolo[3,4-d]pyrimidin-4-ones 273 was also observed in the reaction of 5-amino-1H-pyrazole-5-carboxamide 271 and methyl phenylacetate (272) in EtOH-EtONa (Tintori et al., 2015; Figure 21).
The construction of the azoloazine ring may be performed by using other types of reactive groups in the β-position to the amino group of aminoazole. For example, 5-amino-1H-pyrazole-4-carbonitrile (274) reacted with formamide (275) under microwave irradiation at 200°C with the formation of 1H-pyrazolo[3,4-d]pyrimidin-4-amine (276) (Todorovic et al., 2011). The condensation of the benzamidine 278 proceeded in a similar way (Makarov et al., 2015; Figure 21).
An interesting result was obtained in the condensation of 1-phenyl-5-(1H-pyrrol-1-yl)-1H-pyrazol-4-amine (280) with CDI in 1,4-dioxane or with CS2 in pyridine under refluxing that gave pyrazolo[4,3-e]pyrrolo[1,2-a]pyrazine systems 281, 282 (Farghaly and El-Kashef, 2011; Figure 22).
Two component heterocyclization of aminoazoles 101f–h and phenacyl bromide 283 or its aliphatic analogs was reported in numerous publications as a simple way to synthesize fused imidazoles 284: imidazolo[2,1-b]benzothiazole (Chandak et al., 2013), imidazo[2,1-b][1,3,4]thiadiazole (Copin et al., 2016) were obtained under refluxing in EtOH, CCl4, MeCN. It should be noted, that the formation of uncyclized reaction products is often observed due to the protonation of the exocyclic amino group to form hydrobromic acid salts (Kamal et al., 2010; Figure 22).
2-Chloroacetyl chloride (286) in glacial acetic acid and 2-chloroacetonitrile (288) in DMF-KOH also could be applied in such type of heterocyclizations with the formation of 3,5-dihydro-4H-imidazol-4-ones 287 and 4H-imidazol-5-amines 289 (Rateb, 2014; Soliman et al., 2014; Figure 22).
Reactions of aminoazoles and β-halogen containing carbonyl compounds were reported as a way for the synthesis of angular fused heterocyclic systems. The copper catalyzed synthesis of the pyrazolo[1,5-a]quinazolines 293 was published by Gao et al. The most promising results were obtained in the system DMF-CuI-K2CO3 while other types of alkali media gave the worth results. It was found, that the application of the CuI, additionally stabilized by ethylenediamine was the most effective (Gao et al., 2014; Figure 23).
On the other hand, Nue et al. showed that 2-F and 2-NO2-derivatives 294 may be also applied in such reactions. Simple heating of the starting reagents in dry DMF-Cs2CO3 yielded angular heterocycles 295. Unlike previous authors, application of the K2CO3 instead of Cs2CO3 gave worth results. The absence of the molecular sieves decreased the yield of the target [1,2,4]triazolo[1,5-a]quinazoline 295 from 84 to 74%. Monitoring the reaction mixture by HRMS showed the presence of the Schiff base, that could be one of the intermediates of the heterocyclization (Fang et al., 2014; Niu et al., 2014; Figure 23).
Hedidi et al. reported the copper catalyzed synthesis of pyrido[2,3-e]pyrimidines 297 (Hedidi et al., 2017). The attempts to obtain the target compounds via simple heating of the reagents 5a, 31, 101a, and 296 with Cs2CO3 in DMF, as it had been reported in Niu et al. (2014), were unsuccessful while application of the procedure reported by Gao et al. (2014) allowed to fix their traces. The best results were observed in the system DMSO-CuI-K3PO4 without any ligand (Figure 23).
3-Amino-5-methylisoxazole was sometimes considered as a 1,3-binucleophile reacting with the preservation of the isoxazole moiety. However, in some cases establishing structures of the compounds synthesized without X-Ray data was not sufficient (Rajanarendar et al., 2016; Diyanatizadeh and Yavari, 2017). Sometimes the structures of final compounds were assigned similarly with the pyrazole-containing compounds, which in our opinion may be incorrect due to the possibility of isoxazole ring opening. For instance, the condensations of 3-amino-5-methylisoxazole (298) with arylisothiocyanate 193 with further Boulton—Katritzky rearrangement result in the formation of the 1,2,4-thiadiazoles 300 (Pokhodylo and Shyyka, 2014; Proshin et al., 2014; Figure 24).

Figure 24. Boulton–Katritzky rearrangement in the reactions of 3-amino-5-methylisoxazole with arylisothiocyanates.
Thus, two component reactions involving aminoazoles and substrates of various origins allow forming diverse azoloazine, azinoazine and other heterocyclic systems. The substrates for condensations are not limited to 1,3-dielectrophiles or carbonyl compounds although they constitute the overwhelming majority of typical reagents.
Click Chemistry Concerning Azoles and Aminoazoles
Click chemistry, by B. Sharpless definition (Kolb et al., 2001), describes reactions that are wide in scope, suitable for most substrates, stereospecific, have high yields and low amount of side products, the latter can be removed without application of chromatography methods. The process itself needs to be conducted in mild conditions, the reactants–to be readily available, the solvent–to be easily removed or absent, and the product–to be effortlessly separated from the reaction mixture. The concept of click chemistry perfectly goes along with the principles of green chemistry and with diversity oriented synthesis due to the possibility to build different types of molecular skeleton and may be used for synthesis and further modification of aminoazoles as well.
Talking about click chemistry, azide-alkyne cycloaddition is always the first thought, but the authors of the term (Kolb et al., 2001) also include to the massive of click reactions the following:
• [3 + 2], [4 + 2] and [4 + 1] cycloadditions, Diels-Alder reaction, in particular;
• Nucleophilic addition, oxirane and aziridine ring opening;
• Some heterocyclization reactions
• Reactions of carbonyl compounds: azomethine derivatives formation, epoxidation, Michael reaction.
The most obvious reason for the development of this field of study is the minimization of efforts for obtaining the final product by means of resource economy. The advantages of click chemistry are useful for the purposes of pharmacology and medicinal chemistry (Choi et al., 2006; He et al., 2016). Wastelessness and bioorthogonality of this reaction type promoted its implementation into medicinal chemistry and caused, for example, development of new molecules for contrast identification of cancer cells (Lee et al., 2014), RNA and DNA molecules, proteins (Shieh et al., 2015), etc.
Although, as it was mentioned, the most popular first thought about click-chemistry is the Cu(I)-assisted synthesis of 1,2,3-triazoles, in this part of the review we will focus on the procedures with different starting reactants rather than publications discussing new catalysts for the reaction of azide and alkyne.
The pre-click triazole-forming cycloaddition reactions were well-known in the nineteenth century, but were very inconvenient as they required long-term heating in closed vessels, thus, could be in no way characterized as “click” reactions. As an example, one of those methods (Michael, 1893) included addition of 2-phenyl-2H-triazirine (301) to dimethyl acetylenedicarboxylate (195) in molten form and the product 302 was formed in low yields (Figure 25).
The first azide-alkyne reaction involved transformation of hydrogen azide (303) and acetylene (304) in ethanol-acetone mixture in a closed vessel for 70 h (Dimroth and Fester, 1910). Such unfriendly reaction conditions closed the door to 1,2,3-triazole (305) synthesis and research of the properties of these heterocycles for decades (Figure 25). It should be noted, that the original paper (Dimroth and Fester, 1910) can be hardly found in the journal, though there exists a plenty of references in many papers and theses.
Unarguably, the simplest method to obtain 1,2,3-triazole fragment is copper-catalyzed 1,3-dipolar azide-alkyne cycloaddition (CuAAC), firstly described by Meldal (Tornøe et al., 2002) and Sharpless (Rostovtsev et al., 2002) groups. Its mechanism was studied and published by Worrell et al. (2013), and mechanistic data was thoroughly reviewed by Berg and Straub (2013). Needless to say, CuAAC is highly progressing and, thus, popular object of research, described in a great number of papers and reviews (Hein and Fokin, 2010; Berg and Straub, 2013), and a plenty of other publications are devoted to this reaction in different subtopics, including solid-phase (Castro et al., 2016), green (Shirame and Bhosale, 2018), solvent-free (Tireli et al., 2017) syntheses.
Cu(I) only shows its great catalytic activity with terminal alkynes as reactants (Liang and Astruc, 2011). For non-terminal alkynes catalysts on the basis of platinum metals are known, such as ruthenium (Boren et al., 2008; Johansson et al., 2016) and palladium (Kamijo et al., 2002). Metal-free azide-alkyne cycloadditions and photoclick reactions are also known and intensively studied (Rodríguez-Rodríguez et al., 2015; Singh et al., 2016; Jalani et al., 2017).
Regardless of the novelty of CuAAC method it has quickly become the most popular procedure for the synthesis of 1,2,3-triazole derivatives. Variations were also invented for preparation of these heterocycles from alkenes. For instance, Janreddy et al. (2013) report successful cycloaddition of organic azides 308 to α,β-unsaturated ketones 200, such as vinyl ketones and chalcones, in an oxidative atmosphere of pure oxygen. As an oxidant CuO can also be employed, as a more convenient reagent than gaseous oxygen (Figure 26). In another paper, the triazole ring was also arylated by aryl halides without separation to obtain triazoles 307 (Zhang et al., 2012).
1,2,3-Triazole fragments were also obtained by multicomponent ways. For example, Wu et al. (2005) reported reaction of organic azide 308 and terminal alkyne 183 in the presence of Cu(I) salts and following cleavage of C-Cu bond in an Ullmann-like reaction by an alkylating agent 309, which resulted in 1,4,5-substituted triazole 310 (Figure 26).
Another multicomponent approach (Figure 26) included consecutive reaction of terminal alkyne 183 with acyl halide 311, and then with sodium azide with application of zinc bromide, which served as a catalyst for both steps of the process (Keivanloo et al., 2013).
Another type of click reactions–syntheses of tetrazoles–were carried out even more than a century ago (Pinner, 1894; Dimroth and Merzbacher, 1910), but those tries took a lot of time and energy, requiring 40 h-long refluxing in ethanol, heating in a sealed vial under high pressure etc. Such methods were reviewed by Benson (1947).
Only the second half of XX century contributed easier and faster procedure of tetrazole fragment formation. An end-of-the-century review was published in 1994 (Wittenberger, 1994) and included latest advances in synthesis, functionalization and applications of these heterocycles.
Three-component reactions of primary amines 55 of various origin, including aminoazoles, orthoformic ester 313 and sodium azide leading to 1-substituted tetrazoles 315 (Figure 27) was thoroughly studied by Gaponik group and their followers (Gaponik et al., 1985, 1990; Voitekhovich et al., 2005, 2013). One of the latest and most complete reviews was presented in Grigoriev et al. (2017).
Isonitriles 180 were shown to react with trimethylsilylazide in the presence of hydrochloric acid (Jin et al., 2004), forming 1-substituted tetrazoles 315. Arylisothiocyanates 193 in the reaction with sodium azide form 5-thiotetrazoles 314, which could be oxidized to 1-aryltetrazoles 315 by hydrogen peroxide, chromium trioxide and other oxidizers (Joule and Mills, 2010; Figure 27).
Synthesis of C-substituted tetrazoles 317 is more widely studied, and the number of methods for their preparation is larger. A Lewis acid-assisted reaction of organic nitriles 316 and sodium azide is probably the most popular (Figure 28). Yields were reported to be as high as 90% for benzonitriles in the presence of ZrOCl2, but using zinc salts became a classic procedure (Galante and Somerville, 1996).
Nitriles 316, reactive toward [3 + 2]-cycloaddition reactions with azide, can be formed in situ from primary alcohols 318 or aldehydes 1 by oxidation with iodine in aqueous ammonia under microwave irradiation (Shie and Fang, 2007; Figure 28). Tetrazoles 317 were reported to be separated in high yields in such procedure.
Diphenylphosphorazidate (DPPA) served as a reactant in conversion of aldoximes 319 to 5-substituted tetrazoles 317 (Figure 28), making the publication (Ishihara et al., 2018) different from other methods employing aldoximes as initial compounds by the relative safety of the procedure, which excludes explosive azide sources and heavy metals.
5-Aryl-1-substituted tetrazoles 321 can also be obtained via click reactions. The method (Kaim et al., 2011) suggests mixing of isonitriles 180 with bromine and sodium azide in acetonitrile; arylboric acid with Suzuki catalysts are introduced to the reaction mixture without separation of an intermediate product 320.
Other method of preparation of 1,5-disubstituted tetrazoles 323 can include one-pot transformation of an alkene 322, N-bromosuccinimide, nitriles and trimethylsilane azide, catalyzed by triflates and is reported by Hajra et al. (2007). Yields appeared to be higher when zinc triflate was used (Figure 29).
Methods of preparation of tetrazole 325 starting from carboxamides 324 are also known. In this case, amide group needs to be activated, for example, by trifluorosulfonic acid anhydride (Thomas, 1993) and then [3 + 2]-cycloaddition of azide proceeds (Figure 29).
Tetrazolopyridine 327 synthesis was described by Keith (2006). Pyridine N-oxides 326 were allowed to react with phosphorylazides in hot pyridine as a solvent. Various phosphorylazides were tested, but diphenylphosphorazidate (DPPA) proved to be the most convenient source of azide group (Figure 29).
1-Alkyl-5-aminotetrazoles 332 can be obtained by reaction of cyanazide 329 and primary amines, which form intermediate amidoylazide with subsequent cyclization in acetonitrile-water mixture (Joo and Shreeve, 2008; Figure 30).
5-Aminotetrazole (5b) (332 R = H) was synthesized by nitrosation of aminoguanidine (330) and following heterocyclization of the intermediate with high yields (Kurzer and Godfrey, 1963; Figure 30). 5-Molar nitric acid needs to be considered as a hazard in this synthesis.
1,5-Diaminotetrazole (332) (R = NH2) was formed by heating thiosemicarbazide (331) suspension in dry DMF with ammonium chloride, lead(II) oxide and sodium azide (Gaponik and Karavai, 1984). Only freshly obtained red modification of PbO proved to be useful in this reaction (Figure 30).
Conclusions
In summary, comprehensive analysis of the literature concerning the topic of the present review demonstrates that reactions involving aminoazoles as key reagents possess a high potential for diversity-oriented synthesis and open up effective and convenient pathways to numerous types of final heterocyclic compounds. Classical two-component and stage-by-stage procedures as well as multicomponent reactions of aminoazoles allow to synthesize diverse five-, six- and seven-membered heterocycles using a limited set of reagents the most common of which are α,β-unsaturated carbonyl and carboxyl compounds, cyclic and non-cyclic CH-acids, aldehydes, ketones and diketones of different origin. Additional benefits may be obtained by application of such innovative approaches as microwave- and ultrasonic-assisted organic synthesis, methods of click-chemistry, using special catalysts etc. The compounds synthesized from aminoazoles are useful as building-blocks for further construction of complex heterocyclic systems, as promising objects of medicinal-oriented chemistry to search for the novel drug-like substances and as components of functional materials.
Author Contributions
YS and SD collected most publications related to this review article and sorted them. MM analyzed the literature and wrote the chapter about multicomponent reactions. AM analyzed the literature and wrote the chapter about two-component reactions. IZ analyzed the literature and wrote the chapter about click-chemistry. VC developed the concept of the review, co-wrote and corrected the manuscript.
Funding
Authors thank National Academy of Sciences of Ukraine for financial support in the frame of the projects Development of methods of synthesis of novel chemotypes of drug-like nitrogen containing heterocyclic compounds (0116U001209) and Development of methodology of click-chemistry for the creation of components for novel chelating materials (0117U001280) and President of Ukraine for financial support in the frame of the project Multicomponent isocyanide-based reactions of functionalized starting reagents and post-transformations of the compounds synthesized (F-78/205-2018).
Conflict of Interest Statement
The authors declare that the research was conducted in the absence of any commercial or financial relationships that could be construed as a potential conflict of interest.
References
Abedini, M., Shirini, F., Mousapour, M., and Jolodar, O. G. (2016). Poly(vinylpyrrolidonium) perchlorate catalyzed one-pot synthesis of tricyclic dihydropyrimidine derivatives. Res. Chem. Intermed. 42, 6221–6229. doi: 10.1007/s11164-016-2456-4
Abonia, R. (2014). Solvent-free and self-catalyzed three-component synthesis of diversely substituted pyrazolo[1,4]thiazepinones of potential antitumor activity. Curr. Org. Synth. 11, 773–786. doi: 10.2174/1570179411666140327002045
Adrom, B., Hazeri, N., Lashkari, M., and Maghsoodlou, M. T. (2016). Multicomponent facile synthesis of highly substituted [1,2,4]triazolo[1,5-a] pyrimidines. J. Chem. Res. 40, 458–460. doi: 10.3184/174751916X14664307728623
Aggarwal, R., and Kumar, S. (2018). 5-Aminopyrazole as precursor in design and synthesis of fused pyrazoloazines. Beilstein J. Org. Chem. 14, 203–242. doi: 10.3762/bjoc.14.15
Aggarwal, R., Rani, C., Kumar, R., Garg, G., and Sharma, J. (2014). Synthesis of new bi(pyrazolo[1,5-a]pyrimidinyl)-7-one derivatives from dehydroacetic acid and its analogues as antibacterial agents. Arkivoc 2014, 120–134. doi: 10.3998/ark.5550190.p008.089
Akritopoulou-Zanze, I., Wakefield, B. D., Gasiecki, A., Kalvin, D., Johnson, E. F., Kovar, P., et al. (2011). Scaffold oriented synthesis. Part 4: Design, synthesis and biological evaluation of novel 5-substituted indazoles as potent and selective kinase inhibitors employing heterocycle forming and multicomponent reactions. Bioorganic Med. Chem. Lett. 21, 1480–1483. doi: 10.1016/j.bmcl.2011.01.001
Ali, K. A., Ragab, E. A., Abdelghafar, H. S., and Farag, A. M. (2016). Facile synthetic approaches for new series of pyrazole-4-carbonitrile derivatives. Res. Chem. Intermed. 42, 3553–3566. doi: 10.1007/s11164-015-2231-y
Al-Tel, T. H., Al-Qawasmeh, R. A., and Voelter, W. (2010). Rapid assembly of polyfunctional structures using a one-pot five- and six-component sequential Groebke-Blackburn/Ugi/Passerini process. Eur. J. Org. Chem. 5586–5593. doi: 10.1002/ejoc.201000808
Andriushchenko, A. Y., Desenko, S. M., Chernenko, V. N., and Chebanov, V. A. (2011). Green and efficient synthesis of pyrazolo[3,4-b]quinolin-5-ones derivatives by microwave-assisted multicomponent reaction in hot water medium. J. Heterocycl. Chem. 48, 365–367. doi: 10.1002/jhet.586
Andriushchenko, A. Y., Saraev, V. E., Shishkina, S. V., Shishkin, O. V., Musatov, V. I., Desenko, S. M., et al. (2013). Unusual direction of three-component reactions involving 2-amino-4-arylimidazoles and carbonyl compounds leading to Knoevenagel-Michael adducts. Arkivoc 2013, 61–80. doi: 10.3998/ark.5550190.0014.306
Ansari, A. J., Sharma, S., Pathare, R. S., Gopal, K., Sawant, D. M., and Pardasani, R. T. (2016). Solvent-free multicomponent synthesis of biologically-active fused-imidazo heterocycles catalyzed by reusable Yb(OTf)3 under microwave irradiation. ChemistrySelect 1, 1016–1021. doi: 10.1002/slct.201600241
Aouali, M., Mhalla, D., Allouche, F., El Kaim, L., Tounsi, S., Trigui, M., et al. (2015). Synthesis, antimicrobial and antioxidant activities of imidazotriazoles and new multicomponent reaction toward 5-amino-1-phenyl[1,2,4]triazole derivatives. Med. Chem. Res. 24, 2732–2741. doi: 10.1007/s00044-015-1322-z
Arya, A. K., Gupta, S. K., and Kumar, M. (2012). A domino protocol for the efficient synthesis of structurally diverse benzothiazolylquinoline-2,5-diones and their spiro analogues. Tetrahedron Lett. 53, 6035–6038. doi: 10.1016/j.tetlet.2012.08.099
Atar, A. B., Jeong, Y. S., and Jeong, Y. T. (2014). Iron fluoride: The most efficient catalyst for one-pot synthesis of 4H-pyrimido[2,1-b]benzothiazoles under solvent-free conditions. Tetrahedron 70, 5207–5213. doi: 10.1016/j.tet.2014.05.094
Aydemir, E., Kansiz, S., Gumus, M. K., Gorobets, N. Y., and Dege, N. (2018). Crystal structure and Hirshfeld surface analysis of 7-ethoxy-5-methyl-2-(pyridin-3-yl)-11,12-dihydro-5,11-methano[1,2,4]triazolo[1,5-c][1,3,5]benzoxadiazocine. Acta Crystallogr. Sect. E Crystallogr. Commun. 74, 367–370. doi: 10.1107/S2056989018002621
Balwe, S. G., Shinde, V. V., Rokade, A. A., Park, S. S., and Jeong, Y. T. (2017). Green synthesis and characterization of silver nanoparticles (Ag NPs) from extract of plant Radix Puerariae: an efficient and recyclable catalyst for the construction of pyrimido[1,2-b]indazole derivatives under solvent-free conditions. Catal. Commun. 99, 121–126. doi: 10.1016/j.catcom.2017.06.006
Banfi, L., Basso, A., and Riva, R. (2010). “Synthesis of heterocycles through classical Ugi and Passerini reactions followed by secondary transformations involving one or two additional functional groups,” in Synthesis of Heterocycles via Multicomponent Reactions I, eds R. V. A. Orru, and E. Ruijter (Berlin; Heidelberg: Springer-Verlag), 1–39.
Batool, I., Saeed, A., Qureshi, I. Z., Kalsoom, S., and Razzaq, A. (2016). Synthesis, molecular docking and biological evaluation of new thiazolopyrimidine carboxylates as potential antidiabetic and antibacterial agents. Res. Chem. Intermed. 42, 1139–1163. doi: 10.1007/s11164-015-2078-2
Baviskar, A. T., Madaan, C., Preet, R., Mohapatra, P., Jain, V., Agarwal, A., et al. (2011). N-fused imidazoles as novel anticancer agents that inhibit catalytic activity of topoisomerase IIα and induce apoptosis in G1/S phase. J. Med. Chem. 54, 5013–5030. doi: 10.1021/jm200235u
Benson, F. R. (1947). The chemistry of the tetrazoles. Chem. Rev. 41, 1–61. doi: 10.1021/cr60128a001
Berg, R., and Straub, B. F. (2013). Advancements in the mechanistic understanding of the copper-catalyzed azide–alkyne cycloaddition. Beilstein J. Org. Chem. 9, 2715–2750. doi: 10.3762/bjoc.9.308
Bhatt, J. D., Chudasama, C. J., and Patel, K. D. (2015). Pyrazole clubbed triazolo[1,5-a]pyrimidine hybrids as an anti-tubercular agents: synthesis, in vitro screening and molecular docking study. Bioorganic Med. Chem. 23, 7711–7716. doi: 10.1016/j.bmc.2015.11.018
Bhattacharjee, D., Kshiar, B., and Myrboh, B. (2016). L-Proline as an efficient enantioinduction organo-catalyst in the solvent-free synthesis of pyrazolo[3,4-b]quinoline derivatives via one-pot multi-component reaction. RSC Adv. 6, 95944–95950. doi: 10.1039/C6RA22429F
Bienaymé, H., and Bouzid, K. (1998). A new heterocyclic multicomponent reaction for the combinatorial synthesis of fused 3-aminoimidazoles. Angew. Chem. Int. Ed. Engl. 37, 2234–2237. doi: 10.1002/(SICI)1521-3773(19980904)37:16<2234::AID-ANIE2234>3.0.CO;2-R
Bodaghifard, M. A., Faraki, Z., and Karimi, A. R. (2016). Mild synthesis of mono-, bis- and tris 1,2-dihydrobenzo[4,5]imidazo[1,2-a]pyrimidine derivatives using alkyl disulfamic acid functionalized magnetic nanoparticles. Curr. Org. Chem. 20, 1648–1654. doi: 10.2174/1385272820666160218233729
Boren, B. C., Narayan, S., Rasmussen, L. K., Zhang, L., Zhao, H., Lin, Z., et al. (2008). Ruthenium-catalyzed azide–alkyne cycloaddition: scope and mechanism. J. Am. Chem. Soc. 130, 8923–8930. doi: 10.1021/ja0749993
Burchak, O. N., Mugherli, L., Ostuni, M., Lacapère, J. J., and Balakirev, M. Y. (2011). Combinatorial discovery of fluorescent pharmacophores by multicomponent reactions in droplet arrays. J. Am. Chem. Soc. 133, 10058–10061. doi: 10.1021/ja204016e
Campos, P. T., Rodrigues, L. V., Belladona, A. L., Bender, C. R., Bitencurt, J. S., Rosa, F. A., et al. (2017). Regiochemistry of cyclocondensation reactions in the synthesis of polyazaheterocycles. Beilstein J. Org. Chem. 13, 257–266. doi: 10.3762/bjoc.13.29
Castro, V., Rodríguez, H., and Albericio, F. (2016). CuAAC: an efficient click chemistry reaction on solid phase. ACS Comb. Sci.18, 1–14. doi: 10.1021/acscombsci.5b00087
Centore, R., Manfredi, C., Capobianco, A., Volino, S., Ferrara, M. V., Carella, A., et al. (2017). Solid state separation and isolation of tautomers of fused-ring triazolotriazoles. J. Org. Chem. 82, 5155–5161. doi: 10.1021/acs.joc.7b00380
Chandak, N., Bhardwaj, J. K., Sharma, R. K., and Sharma, P. K. (2013). Inhibitors of apoptosis in testicular germ cells: Synthesis and biological evaluation of some novel IBTs bearing sulfonamide moiety. Eur. J. Med. Chem. 59, 203–208. doi: 10.1016/j.ejmech.2012.11.015
Chebanov, V. A., and Desenko, S. M. (2006). Dihydroazines based on α,β-unsaturated ketones reactions. Curr. Org. Chem. 10, 297–317. doi: 10.2174/138527206775473904
Chebanov, V. A., and Desenko, S. M. (2012). Multicomponent heterocyclization reactions with controlled selectivity (review). Chem. Heterocycl. Compd. 48, 566–583. doi: 10.1007/s10593-012-1030-2
Chebanov, V. A., and Desenko, S. M. (2014). Switchable multicomponent heterocyclizations for diversity oriented synthesis. Divers. Oriented Synth. 1, 43–63. doi: 10.2478/dos-2014-0003
Chebanov, V. A., Desenko, S. M., and Gurley, T. W. (2008a). Azaheterocycles Based on α,β-Unsaturated Carbonyls. Berlin; Heidelberg: Springer-Verlag.
Chebanov, V. A., Gura, K. A., and Desenko, S. M. (2010). “Aminoazoles as key reagents in multicomponent heterocyclizations,” in Synthesis of Heterocycles via Multicomponent Reactions I, eds R. V. A. Orru, and E. Ruijter (Berlin; Heidelberg: Springer-Verlag), 41–84.
Chebanov, V. A., Sakhno, Y. I., and Desenko, S. M. (2012a). High regioselective ultrasonic-assisted synthesis of 2,7-diaryl-4,7-dihydropyrazolo[1,5-a]pyrimidine-5-carboxylic acids. Ultrason. Sonochem. 19, 707–709. doi: 10.1016/j.ultsonch.2011.08.003
Chebanov, V. A., Sakhno, Y. I., Desenko, S. M., Chernenko, V. N., Musatov, V. I., Shishkina, S. V., et al. (2007a). Cyclocondensation reactions of 5-aminopyrazoles, pyruvic acids and aldehydes. Multicomponent approaches to pyrazolopyridines and related products. Tetrahedron 63, 1229–1242. doi: 10.1016/j.tet.2006.11.048
Chebanov, V. A., Sakhno, Y. I., Desenko, S. M., Shishkina, S. V., Musatov, V. I., Shishkin, O. V., et al. (2005). Three-component procedure for the synthesis of 5-aryl-5,8-dihydroazolo[1,5-a]pyrimidine-7-carboxylic acids. Synthesis, 2597–2601. doi: 10.1055/s-2005-872073
Chebanov, V. A., Saraev, V. E., Desenko, S. M., Chernenko, V. N., Knyazeva, I. V., Groth, U., et al. (2008b). Tuning of chemo- and regioselectivities in multicomponent condensations of 5-aminopyrazoles, dimedone, and aldehydes. J. Org. Chem. 73, 5110–5118. doi: 10.1021/jo800825c
Chebanov, V. A., Saraev, V. E., Desenko, S. M., Chernenko, V. N., Shishkina, S. V., Shishkin, O. V., et al. (2007b). One-pot, multicomponent route to pyrazoloquinolizinones. Org. Lett. 9, 1691–1694. doi: 10.1021/ol070411l
Chebanov, V. A., Saraev, V. E., Shishkina, S. V., Shishkin, O. V., Musatov, V. I., and Desenko, S. M. (2012b). Controlled switching of multicomponent heterocyclizations of 5-amino-N-arylpyrazole-4-carboxamides, 1,3-cyclohexanediones, and aldehydes. Eur. J. Org. Chem. 2012, 5515–5524. doi: 10.1002/ejoc.201200669
Chen, Z., Shi, Y., Shen, Q., Xu, H., and Zhang, F. (2015). Facile and efficient synthesis of pyrazoloisoquinoline and pyrazolopyridine derivatives using recoverable carbonaceous material as solid acid catalyst. Tetrahedron Lett. 56, 4749–4752. doi: 10.1016/j.tetlet.2015.06.044
Chikhale, R., Thorat, S., Pant, A., Jadhav, A., Thatipamula, K. C., Bansode, R., et al. (2015). Design, synthesis and pharmacological evaluation of pyrimidobenzothiazole-3-carboxylate derivatives as selective L-type calcium channel blockers. Bioorganic Med. Chem. 23, 6689–6713. doi: 10.1016/j.bmc.2015.09.009
Choi, W. J., Shi, Z. D., Worthy, K. M., Bindu, L., Karki, R. G., Nicklaus, M. C., et al. (2006). Application of azide–alkyne cycloaddition ‘click chemistry’ for the synthesis of Grb2 SH2 domain-binding macrocycles. Bioorganic Med. Chem. Lett. 16, 5265–5269. doi: 10.1016/j.bmcl.2006.08.004
Cioc, R. C., Ruijter, E., and Orru, R. V. A. (2014). Multicomponent reactions: advanced tools for sustainable organic synthesis. Green Chem. 16, 2958–2975. doi: 10.1039/c4gc00013g
Copin, C., Buron, F., and Routier, S. (2016). Palladium-catalyzed amination of C-5 bromoimidazo[2,1-b]-[1,3,4]-thiadiazoles. Eur. J. Org. Chem. 2016, 1958–1962. doi: 10.1002/ejoc.201600145
Cowen, S. D., Russell, D., Dakin, L. A., Chen, H., Larsen, N. A., Godin, R., et al. (2016). Design, synthesis, and biological activity of substrate competitive SMYD2 inhibitors. J. Med. Chem. 59, 11079–11097. doi: 10.1021/acs.jmedchem.6b01303
Dam, B., Pal, A. K., and Gupta, A. (2016). Nano-Fe3O4@silica sulfuric acid as a reusable and magnetically separable potent solid acid catalyst in Biginelli-type reaction for the one-pot multicomponent synthesis of fused dihydropyrimidine derivatives: a greener NOSE and SFRC approach. Synth. Commun. 46, 275–286. doi: 10.1080/00397911.2015.1135955
Demjén, A., Gyuris, M., Wölfling, J., Puskás, L. G., and Kanizsai, I. (2014). Facile synthesis of 1H-imidazo[1,2-b]pyrazoles via a sequential one-pot synthetic approach. Beilstein J. Org. Chem. 10, 2338–2344. doi: 10.3762/bjoc.10.243
Desenko, S. M. (1995). Dihydroazolopyrimidines with a nodal nitrogen atom: Synthesis, reactions, tatutomerism (review). Chem. Heterocycl. Compd. 31, 125–136. doi: 10.1007/BF01169665
Desenko, S. M., Orlov, V. D., and Estrada, K. (1990). Formation of derivatives of 1,2,4-triazoloquinazolines in the reactions of 3-amino-1,2,4-triazoles with cyclohexanone. Chem. Heterocycl. Compd. 26, 839–840. doi: 10.1007/BF00509729
Devi, N., Rawal, R. K., and Singh, V. (2015). Diversity-oriented synthesis of fused-imidazole derivatives via Groebke-Blackburn-Bienayme reaction: a review. Tetrahedron 71, 183–232. doi: 10.1016/j.tet.2014.10.032
Dias, L. R. S., Santos, M. B., De Albuquerque, S., Castro, H. C., De Souza, A. M. T., Freitas, A. C. C., et al. (2007). Synthesis, in vitro evaluation, and SAR studies of a potential antichagasic 1H-pyrazolo[3,4-b]pyridine series. Bioorganic Med. Chem. 15, 211–219. doi: 10.1016/j.bmc.2006.09.067
Dimroth, O., and Fester, G. (1910). Triazole and tetrazole from hydrazoic acid (Engl. Transl.). Berichte Dtsch. Chem. Gesellschaft 43, 2219–2223.
Dimroth, O., and Merzbacher, S. (1910). Synthese von Tetrazolen aus Arylaziden. Berichte Dtsch. Chem. Gesellschaft 43, 2899–2904. doi: 10.1002/cber.19100430350
Diyanatizadeh, M. H., and Yavari, I. (2017). Synthesis of spiro heterocyclic systems from 2-(3-oxoisobenzofuran-1(3H)-ylidene)malononitrile and binucleophiles. J. Chem. Res. 41, 330–332. doi: 10.3184/174751917X14944355549159
Dömling, A. (2006). Recent developments in isocyanide-based multicomponent reactions in applied chemistry. Chem. Rev. 106, 17–89. doi: 10.1021/cr0505728
Dömling, A., and Ugi, I. (2000). Multicomponent reactions with isocyanides. Angew. Chem. Int. Ed. Engl. 39, 3168–3210. doi: 10.1002/1521-3773(20000915)39:18<3168::AID-ANIE3168>3.0.CO;2-U
Dömling, A., Wang, W., and Wang, K. (2012). Chemistry and biology of multicomponent reactions. Chem. Rev. 112, 3083–3135. doi: 10.1021/cr100233r
Ebrahimi, S. (2016). One-pot synthesis of 1,3-thiazolidin-4-one using ammonium persulfate as catalyst. J. Sulfur Chem. 5993, 1–6. doi: 10.1080/17415993.2016.1223298
El Rady, E. A. (2014). Three-component uncatalyzed eco-friendly reactions for one-pot synthesis of 4,7-dihydro[1,2,4]triazolo[1,5-a]pyrimidine derivatives. J. Heterocycl. Chem. 51, 869–875. doi: 10.1002/jhet.1771
El-Borai, M. A., Rizk, H. F., Abd-Aal, M. F., and El-Deeb, I. Y. (2012). Synthesis of pyrazolo[3,4-b]pyridines under microwave irradiation in multi-component reactions and their antitumor and antimicrobial activities–part 1. Eur. J. Med. Chem. 48, 92–96. doi: 10.1016/j.ejmech.2011.11.038
El-Emary, T. I., and El-Mohsen, S. A. (2012). Multi-component one-pot synthesis and antimicrobial activities of 3-methyl-1,4-diphenyl-7-thioxo-4,6,8,9-tetrahydro-pyrazolo[5,4-b]pyrimidino[5,4-e]pyridine-5-one and related derivatives. Molecules 17, 14464–14483. doi: 10.3390/molecules171214464
Fan, L., Yao, C., and Shu, M. (2016). Three-component synthesis of new o-hydroxyphenyl-substituted pyrazolo[3,4-b]pyridines promoted by FeCl3. Heterocycl. Commun. 22, 63–67. doi: 10.1515/hc-2015-0234
Fang, S., Niu, X., Yang, B., Li, Y., Si, X., Feng, L., et al. (2014). One-Pot synthesis of benzo[4,5]imidazo[1,2-a]quinazoline derivatives via facile transition-metal-free tandem process. ACS Comb. Sci. 16, 328–332. doi: 10.1021/co500001u
Farghaly, A.-R., and El-Kashef, H. (2011). Pyrazoles and pyrazolo[4,3-e]pyrrolo[1,2-a]pyrazines II. J. Heterocycl. Chem. 48, 678–683. doi: 10.1002/jhet.581
Farghaly, T. S., Shawali, A. M. H., Abbas, E. A., and Abdel-hafez, N. (2015). A facile synthesis of new polyazaheterocycles via one-pot three-components condensation reaction and study of their reactions with nitrilimines. Curr. Org. Synth. 12, 95–101. doi: 10.2174/1570179411666140806005524
Finlay, H. J., Jiang, J., Caringal, Y., Kover, A., Conder, M. L., Xing, D., et al. (2013). Triazolo and imidazo dihydropyrazolopyrimidine potassium channel antagonists. Bioorganic Med. Chem. Lett. 23, 1743–1747. doi: 10.1016/j.bmcl.2013.01.064
Finlay, H. J., Lloyd, J., Vaccaro, W., Kover, A., Yan, L., Bhave, G., et al. (2012). Discovery of ((S)-5-(methoxymethyl)-7-(1-methyl-1H-indol-2-yl)-2-(trifluoromethyl)-4,7-dihydro pyrazolo[1,5-a]pyrimidin-6-yl)((S)-2-(3-methylisoxazol-5-yl)pyrrolidin-1-yl)methanone as a potent and selective IKur inhibitor. J. Med. Chem. 55, 3036–3048. doi: 10.1021/jm201386u
Frolova, L. V., Malik, I., Uglinskii, P. Y., Rogelj, S., Kornienko, A., and Magedov, I. V. (2011). Multicomponent synthesis of 2,3-dihydrochromeno[4,3-d]pyrazolo[3,4-b]pyridine-1,6-diones: a novel heterocyclic scaffold with antibacterial activity. Tetrahedron Lett. 52, 6643–6645. doi: 10.1016/j.tetlet.2011.10.012
Fusco, S., Maglione, C., Velardo, A., Piccialli, V., Liguori, R., Peluso, A., et al. (2016). N-rich fused heterocyclic systems: synthesis, structure, optical and electrochemical characterization. Eur. J. Org. Chem. 2016, 1772–1780. doi: 10.1002/ejoc.201501283
Galante, R. J., and Somerville, N. J. (1996). Method of Synthesizing Sterically Hindered 5-Substituted-1H-Tetrazoles From Nitriles Using a Lewis Acid and an Azide. U.S. Patent No 5502191. Madison, NJ: U.S. American Cyanamid Company.
Gao, L., Song, Y., Zhang, X., Guo, S., and Fan, X. (2014). Copper-catalyzed tandem reactions of 2-bromobenzaldehydes/ketones with aminopyrazoles toward the synthesis of pyrazolo[1,5-a]quinazolines. Tetrahedron Lett. 55, 4997–5002. doi: 10.1016/j.tetlet.2014.07.028
Gaponik, P. N., and Karavai, V. P. (1984). Synthesis and properties of 1,5-diaminotetrazole. Chem. Heterocycl. Compd. 20, 1388–1391. doi: 10.1007/BF00505966
Gaponik, P. N., Karavai, V. P., Davshko, I. E., Degtyarik, M. M., and Bogatikov, A. N. (1990). Synthesis and properties of phenylenebis-1H-tetrazoles. Chem. Heterocycl. Compd. 26, 1274–1278. doi: 10.1007/BF00476984
Gaponik, P. N., Karavai, V. P., and Grigor'ev, Y. V. (1985). Synthesis of 1-substituted tetrazoles by heterocyclization of primary amines, orthoformic ester, and sodium azide. Chem. Heterocycl. Compd. 21, 1255–1258. doi: 10.1007/BF00515224
Gege, C., Bao, B., Bluhm, H., Boer, J., Gallagher, B. M., Korniski, B., et al. (2012). Discovery and evaluation of a non-Zn chelating, selective matrix metalloproteinase 13 (MMP-13) inhibitor for potential intra-articular treatment of osteoarthritis. J. Med. Chem. 55, 709–716. doi: 10.1021/jm201152u
Gein, V. L., Kazantseva, M. I., Zamaraeva, T. M., Gein, L. F., and Slepukhin, P. A. (2015). Synthesis of 9-aryl-5,6,7,9-tetrahydrotetrazolo[5,1-b]quinazolin-8(4H)-ones. Russ. J. Gen. Chem. 85, 1984–1986. doi: 10.1134/S1070363215080332
Gein, V. L., Zamaraeva, T. M., Nosova, N. V., Vakhrin, M. I., and Slepukhin, P. A. (2012). Synthesis of N-substituted 7-aryl-5-methyl-4,7-dihydrotetrazolo[1,5-a]pyrimidine-6-carboxamides. Russ. J. Org. Chem. 48, 419–422. doi: 10.1134/S107042801203013X
Gein, V. L., Zamaraeva, T. M., and Vakhrin, M. I. (2014). Synthesis of N,7-diaryl-5-methyl-4,7-dihydro-1,2,4-triazolo[1,5-α]pyrimidine-6-carboxamides. Russ. J. Gen. Chem. 84, 82–85. doi: 10.1134/S1070363214010125
Ghatole, A. M., Lanjewar, K. R., Gaidhane, M. K., and Hatzade, K. M. (2015). Evaluation of substituted methyl cyclohexanone hybrids for anti-tubercular, anti-bacterial and anti-fungal activity: facile syntheses under catalysis by ionic liquids. Spectrochim. Acta Part A Mol. Biomol. Spectrosc. 151, 515–524. doi: 10.1016/j.saa.2015.06.035
Ghorbani-Vaghei, R., Toghraei-Semiromi, Z., Amiri, M., and Karimi-Nami, R. (2013). One-pot synthesis of tetrazolo[1,5-a]pyrimidines under solvent-free conditions. Mol. Divers. 17, 307–318. doi: 10.1007/s11030-013-9435-0
Gladkov, E. S., Desenko, S. M., Konovalova, I. S., Groth, U., Shishkin, O. V., Vashchenko, E. V., et al. (2013). Microwave-assisted and ultrasonic-assisted three-component heterocyclization of 4-amino-5-carboxamido-1,2,3-triazole, thiopyran-3-one-1,1-dioxide, and aromatic aldehydes. J. Heterocycl. Chem. 50, E189–E192. doi: 10.1002/jhet.1503
Gladkov, E. S., Gura, K. A., Sirko, S. M., Desenko, S. M., Groth, U., and Chebanov, V. A. (2012). Features of the behavior of 4-amino-5-carboxamido-1,2,3-triazole in multicomponent heterocyclizations with carbonyl compounds. Beilstein J. Org. Chem. 8, 2100–2105. doi: 10.3762/bjoc.8.236
Gladkov, E. S., Sirko, S. N., Shishkina, S. V., Shishkin, O. V., Knyazeva, I. V., Desenko, S. M., et al. (2010). Efficient multicomponent synthesis of highly substituted [1,2,3]triazolo[1,5-a]pyrimidines. Monatshefte Chemie Chem. Mon. 141, 773–779. doi: 10.1007/s00706-010-0326-0
Gorobets, N. Y., Sedash, Y. V., Ostras, K. S., Zaremba, O. V., Shishkina, S. V., Baumer, V. N., et al. (2010). Unexpected alternative direction of a Biginelli-like multicomponent reaction with 3-amino-1,2,4-triazole as the urea component. Tetrahedron Lett. 51, 2095–2098. doi: 10.1016/j.tetlet.2010.02.045
Goryaeva, M. V., Burgart, Y. V., Ezhikova, M. A., Kodess, M. I., and Saloutin, V. I. (2015). The reactions of 2-ethoxymethylidene-3-oxo esters and their analogues with 5-aminotetrazole as a way to novel azaheterocycles. Beilstein J. Org. Chem. 11, 385–391. doi: 10.3762/bjoc.11.44
Grigoriev, Y. V., Voitekhovich, S. V., Karavai, V. P., and Ivashkevich, O. A. (2017). Synthesis of tetrazole and its derivatives by heterocyclization reaction involving primary amines, orthoesters, and azides. Chem. Heterocycl. Compd. 53, 670–681. doi: 10.1007/s10593-017-2108-7
Guchhait, S. K., Chandgude, A. L., and Priyadarshani, G. (2012). CuSO4 – glucose for in situ generation of controlled Cu(I)–Cu(II) bicatalysts: multicomponent reaction of heterocyclic azine and aldehyde with alkyne, and cycloisomerization toward synthesis of N-fused Imidazoles. J. Org. Chem. 77, 4438–4444. doi: 10.1021/jo3003024
Guchhait, S. K., and Madaan, C. (2009). An efficient, regioselective, versatile synthesis of N-fused 2- and 3-aminoimidazoles via Ugi-type multicomponent reaction mediated by zirconium(IV) chloride in polyethylene glycol-400. Synlett 2009, 628–632. doi: 10.1055/s-0028-1087915
Guchhait, S. K., and Madaan, C. (2010). Towards molecular diversity: dealkylation of tert-butyl amine in Ugi-type multicomponent reaction product establishes tert-butyl isocyanide as a useful convertible isonitrile. Org. Biomol. Chem. 8, 3631–3634. doi: 10.1039/c0ob00022a
Guchhait, S. K., Madaan, C., and Thakkar, B. S. (2009). A highly flexible and efficient Ugi-type multicomponent synthesis of versatile N-fused aminoimidazoles. Synthesis 2009, 3293–3300. doi: 10.1055/s-0029-1216916
Gujjar, R., El Mazouni, F., White, K. L., White, J., Creason, S., Shackleford, D. M., et al. (2011). Lead optimization of aryl and aralkyl amine-based triazolopyrimidine inhibitors of plasmodium falciparum dihydroorotate dehydrogenase with antimalarial activity in mice. J. Med. Chem. 54, 3935–3949. doi: 10.1021/jm200265b
Gümüş, M. K., Gorobets, N. Y., Sedash, Y. V., Chebanov, V. A., and Desenko, S. M. (2017a). A modified Biginelli reaction toward oxygen-bridged tetrahydropyrimidines fused with substituted 1,2,4-triazole ring. Chem. Heterocycl. Compd. 53, 1261–1267. doi: 10.1007/s10593-018-2204-3
Gümüş, M. K., Gorobets, N. Y., Sedash, Y. V., Shishkina, S. V., and Desenko, S. M. (2017b). Rapid formation of chemical complexity via a modified Biginelli reaction leading to dihydrofuran-2(3H)-one spiro-derivatives of triazolo[1,5-a]pyrimidine. Tetrahedron Lett. 58, 3446–3448. doi: 10.1016/j.tetlet.2017.07.071
Gunasekaran, P., Indumathi, S., and Perumal, S. (2013). L-Proline-catalyzed three-component domino reactions in the regioselective synthesis of novel densely functionalized pyrazolo[3,4-b]pyridines. RSC Adv. 3, 8318–8325. doi: 10.1039/c3ra00136a
Gurevich, P. A., Sattarova, L. F., Petrovskiy, A. S., Frolova, N. A., Strunin, B. P., and Musin, R. Z. (2011). Interaction of spiro-heterocyclic oxindole system with sodium diformylimide. Chem. Heterocycl. Compd. 46, 1527–1530. doi: 10.1007/s10593-011-0703-6
Hajra, S., Sinha, D., and Bhowmick, M. (2007). Metal triflate catalyzed reactions of alkenes, NBS, nitriles, and TMSN3: synthesis of 1,5-disubstituted tetrazoles. J. Org. Chem. 72, 1852–1855. doi: 10.1021/jo062432j
Haleel, A., Arthi, P., Reddy, N. D., Veena, V., Sakthivel, N., Arun, Y., et al. (2014). DNA binding, molecular docking and apoptotic inducing activity of nickel(II), copper(II) and zinc(II) complexes of pyridine-based tetrazolo[1,5-a]pyrimidine ligands. RSC Adv. 4, 60816–60830. doi: 10.1039/C4RA11197D
Hamama, W. S., Ibrahim, M. E., and Zoorob, H. H. (2012). Efficient regioselective synthesis and potential antitumor evaluation of isoxazolo[5,4-b]pyridines and related annulated compounds. Arch. Pharm. 345, 468–475. doi: 10.1002/ardp.201100258
Hassaneen, H. M. E., and Farghaly, T. A. (2015). A simple, convenient, one-pot synthesis of dihydro-azolopyrimidines, DFT calculation, and NMR determination by using H-ferrierite zeolite as catalyst. J. Heterocycl. Chem. 52, 1154–1161. doi: 10.1002/jhet.2152
Hatti, I., Sreenivasulu, R., Jadav, S. S., Jayaprakash, V., Kumar, C. G., and Raju, R. R. (2015). Synthesis, cytotoxic activity and docking studies of new 4-aza-podophyllotoxin derivatives. Med. Chem. Res. 24, 3305–3313. doi: 10.1007/s00044-015-1375-z
He, X. P., Zeng, Y. L., Zang, Y., Li, J., Field, R. A., and Chen, G. R. (2016). Carbohydrate CuAAC click chemistry for therapy and diagnosis. Carbohydr. Res. 429, 1–22. doi: 10.1016/J.CARRES.2016.03.022
Hedidi, M., Maillard, J., Erb, W., Lassagne, F., Halauko, Y. S., Ivashkevich, O. A., et al. (2017). Fused systems based on 2-aminopyrimidines: synthesis combining deprotolithiation-in situ zincation with N-arylation reactions and biological properties. Eur. J. Org. Chem. 2017, 5903–5915. doi: 10.1002/ejoc.201701004
Hein, J. E., and Fokin, V. V. (2010). Copper-catalyzed azide-alkyne cycloaddition (CuAAC) and beyond: new reactivity of copper(I) acetylides. Chem. Soc. Rev. 39, 1302–1315. doi: 10.1039/b904091a
Hemmati, B., Javanshir, S., and Dolatkhah, Z. (2016). Hybrid magnetic Irish moss/Fe3O4 as a nano-biocatalyst for synthesis of imidazopyrimidine derivatives. RSC Adv. 6, 50431–50436. doi: 10.1039/C6RA08504K
Hieke, M., Rödl, C. B., Wisniewska, J. M., La Buscató, E., Stark, H., Schubert-Zsilavecz, M., et al. (2012). SAR-study on a new class of imidazo[1,2-a]pyridine-based inhibitors of 5-lipoxygenase. Bioorganic Med. Chem. Lett. 22, 1969–1975. doi: 10.1016/j.bmcl.2012.01.038
Hill, M. D. (2016). A multicomponent approach to highly substituted 1H-pyrazolo[3,4-b]pyridines. Synthesis 48, 2201–2204. doi: 10.1055/s-0035-1562230
Hossein Nia, R., Mamaghani, M., Shirini, F., and Tabatabaeian, K. (2014). A convenient one-pot three-component approach for regioselective synthesis of novel substituted pyrazolo[1,5-a]pyrimidines using Fe+3-montmorillonite as efficient catalyst. J. Heterocycl. Chem. 51, 363–367. doi: 10.1002/jhet.1576
Huang, Z., Hu, Y., Zhou, Y., and Shi, D. (2011). Efficient one-pot three-component synthesis of fused pyridine derivatives in ionic liquid. ACS Comb. Sci. 13, 45–49. doi: 10.1021/co1000162
Hudwekar, A. D., Reddy, G. L., Verma, P. K., Gupta, S., Vishwakarma, R. A., and Sawant, S. D. (2017). Transition metal-free single step approach for arylated pyrazolopyrimidinones and quinazolinones using benzylamines/benzylalcohols/benzaldehydes. ChemistrySelect 2, 4963–4968. doi: 10.1002/slct.201700896
Ishihara, K., Kawashima, M., Matsumoto, T., Shioiri, T., and Matsugi, M. (2018). A practical synthesis of 5-substituted 1H-tetrazoles from aldoximes employing the azide anion from diphenyl phosphorazidate. Synthesis 50, 1293–1300. doi: 10.1055/s-0036-1591851
Ivachtchenko, A. V., Golovina, E. S., Kadieva, M. G., Koryakova, A. G., Mitkin, O. D., Tkachenko, S. E., et al. (2011). 2-Substituted 5,6-dimethyl-3-phenylsulfonyl-pyrazolo[1,5-a]pyrimidines: New series of highly potent and specific serotonin 5-HT6 receptor antagonists. Eur. J. Med. Chem. 46, 1189–1197. doi: 10.1016/j.ejmech.2011.01.038
Jalani, H. B., Karagöz, A. Ç., and Tsogoeva, S. B. (2017). Synthesis of substituted 1,2,3-triazoles via metal-free click cycloaddition reactions and alternative cyclization methods. Synthesis 49, 29–41. doi: 10.1055/s-0036-1588904
Janreddy, D., Kavala, V., Kuo, C. W., Chen, W. C., Ramesh, C., Kotipalli, T., et al. (2013). Copper(I)-catalyzed aerobic oxidative azide-alkene cycloaddition: an efficient synthesis of substituted 1,2,3-triazoles. Adv. Synth. Catal. 355, 2918–2927. doi: 10.1002/adsc.201300344
Jiang, B., Liu, Y.-P., and Tu, S.-J. (2011). Facile three-component synthesis of macrocyclane-fused pyrazolo[3,4-b]pyridine derivatives. Eur. J. Org. Chem. 2011, 3026–3035. doi: 10.1002/ejoc.201100127
Jiang, B., Ma, N., Wang, X. H., Tu, S. J., and Li, G. (2012). Microwave-assisted multicomponent reaction in water: highly stereoselective synthesis of pyrimidinespiroisoxazolo[5,4-b]pyridine derivatives. Heterocycles 84, 765–774. doi: 10.3987/COM-11-S(P)53
Jiang, B., Ye, Q., Fan, W., Wang, S. L., Tu, S. J., and Li, G. (2014). Four-component strategy for selective synthesis of azepino[5,4,3-cd]indoles and pyrazolo[3,4-b]pyridines. Chem. Commun. 50, 6108–6111. doi: 10.1039/C4CC00740A
Jin, T., Kamijo, S., and Yamamoto, Y. (2004). Synthesis of 1-substituted tetrazoles via the acid-catalyzed [3 + 2] cycloaddition between isocyanides and trimethylsilyl azide. Tetrahedron Lett. 45, 9435–9437. doi: 10.1016/j.tetlet.2004.10.103
Johansson, J. R., Beke-Somfai, T., Said Stålsmeden, A., and Kann, N. (2016). Ruthenium-catalyzed azide alkyne cycloaddition reaction: scope, mechanism, and applications. Chem. Rev. 116, 14726–14768. doi: 10.1021/acs.chemrev.6b00466
Joo, Y. H., and Shreeve, J. M. (2008). 1-Substituted 5-aminotetrazoles: syntheses from CNN3 with primary amines. Org. Lett. 10, 4665–4667. doi: 10.1021/ol8019742
Joule, J. A., and Mills, K. (2010). Heterocyclic Chemistry. 5th Edn. Chichester, UK: Wiley-Blackwell.
Kaim, L., El Grimaud, L., and Patil, P. (2011). Three-component strategy toward 5-membered heterocycles from isocyanide dibromides. Org. Lett. 13, 1261–1263. doi: 10.1021/ol200003u
Kamal, A., Dastagiri, D., Ramaiah, M. J., Reddy, J. S., Bharathi, E. V., Srinivas, C., et al. (2010). Synthesis of imidazothiazole-chalcone derivatives as anticancer and apoptosis inducing agents. ChemMedChem 5, 1937–1947. doi: 10.1002/cmdc.201000346
Kamal, A., Suresh, P., Mallareddy, A., Kumar, B. A., Reddy, P. V., Raju, P., et al. (2011). Synthesis of a new 4-aza-2,3-didehydropodophyllotoxin analogues as potent cytotoxic and antimitotic agents. Bioorganic Med. Chem. 19, 2349–2358. doi: 10.1016/j.bmc.2011.02.020
Kamijo, S., Jin, T., Huo, Z., and Yamamoto, Y. (2002). Regiospecific synthesis of 2-allyl-1,2,3-triazoles by palladium-catalyzed 1,3-dipolar cycloaddition. Tetrahedron Lett. 43, 9707–9710. doi: 10.1016/S0040-4039(02)02206-2
Kantin, G. P., and Krasavin, M. (2016). Reaction of α-tetralone, 1H-tetrazol-5-amine, and aromatic aldehydes upon microwave irradiation – a convenient method for the synthesis of 5,6,7,12-tetrahydrobenzo[h]tetrazolo[5,1-b]quinazolines. Chem. Heterocycl. Compd. 52, 918–922. doi: 10.1007/s10593-017-1985-0
Karami, B., Farahi, M., and Banaki, Z. (2015a). A new protocol for catalyst-free regioselective synthesis of 5,9-dihydropyrimido[5,4-e][1,2,4]triazolo[1,5-a]pyrimidine-6,8(4H,7H)-diones. Synlett 26, 741–744. doi: 10.1055/s-0034-1379953
Karami, B., Farahi, M., and Banaki, Z. (2015b). A novel one-pot method for highly regioselective synthesis of triazoloapyrimidinedicarboxylates using silica sodium carbonate. Synlett 26, 1804–1807. doi: 10.1055/s-0034-1380677
Karimi, A. R., and Bayat, F. (2011). Mono- and bis-pyrimido[1,2-a]benzimidazoles: alum catalyzed regioselective three- or pseudo five-component reaction of 2-aminobenzimidazole with aldehyde and malononitrile. Lett. Org. Chem. 8, 631–636. doi: 10.2174/157017811799304368
Karnakar, K., Narayana Murthy, S., Ramesh, K., Satish, G., Nanubolu, J. B., and Nageswar, Y. V. D. (2012). Polyethylene glycol (PEG-400): An efficient and recyclable reaction medium for the synthesis of pyrazolo[3,4-b]quinoline derivatives. Tetrahedron Lett. 53, 2897–2903. doi: 10.1016/j.tetlet.2012.03.135
Kaur, G., Raj, T., Kaur, N., and Singh, N. (2015). Pyrimidine-based functional fluorescent organic nanoparticle probe for detection of Pseudomonas aeruginosa. Org. Biomol. Chem. 13, 4673–4679. doi: 10.1039/C5OB00206K
Kaur, N., Kaur, K., Raj, T., Kaur, G., Singh, A., Aree, T., et al. (2015). One-pot synthesis of tricyclic dihydropyrimidine derivatives and their biological evaluation. Tetrahedron 71, 332–337. doi: 10.1016/j.tet.2014.11.039
Keith, J. M. (2006). One-step conversion of pyridine N-oxides to tetrazolo[1,5-a]pyridines. J. Org. Chem. 71, 9540–9543. doi: 10.1021/jo061819j
Keivanloo, A., Bakherad, M., Taheri, S. A. N., and Samangooei, S. (2013). One-pot synthesis of 4,5-disubstituted 1,2,3-(NH)-triazoles by silica supported-zinc bromide in the aerobic condition. Comptes Rendus Chim. 16, 239–243. doi: 10.1016/j.crci.2012.11.007
Khurana, J. M., Chaudhary, A., Nand, B., and Lumb, A. (2012). Aqua mediated indium(III) chloride catalyzed synthesis of fused pyrimidines and pyrazoles. Tetrahedron Lett. 53, 3018–3022. doi: 10.1016/j.tetlet.2012.04.001
Kolb, H. C., Finn, M. G., and Sharpless, K. B. (2001). Click chemistry: diverse chemical function from a few good reactions. Angew. Chemie Int. Ed. 40, 2004–2021. doi: 10.1002/1521-3773(20010601)40:11<2004::AID-ANIE2004>3.0.CO;2-5
Kolos, N. N., Kovalenko, L. U., and Borovskoy, V. A. (2011). Reactions of 3-aroylacrylates with α-aminoazoles. Chem. Heterocycl. Compd. 47, 983–988. doi: 10.1007/s10593-011-0864-3
Kombarov, R., Altieri, A., Genis, D., Kirpichenok, M., Kochubey, V., Rakitina, N., et al. (2010). BioCores: identification of a drug/natural product-based privileged structural motif for small-molecule lead discovery. Mol. Divers. 14, 193–200. doi: 10.1007/s11030-009-9157-5
Komykhov, S. A., Bondarenko, A. A., Musatov, V. I., Diachkov, M. V., Gorobets, N. Y., and Desenko, S. M. (2017). (5S,7R)-5-aryl-7-methyl-4,5,6,7-tetrahydro-[1,2,4]triazolo[1,5-a]pyrimidin-7-ols as products of three-component condensation. Chem. Heterocycl. Compd. 53, 378–380. doi: 10.1007/s10593-017-2059-z
Komykhov, S. A., Tkachenko, I. G., Musatov, V. I., Diachkov, M. V., Chebanov, V. A., and Desenko, S. M. (2016). Multicomponent synthesis in water of 7-unsubstituted 4,7-dihydro- 1,2,4-triazolo[1,5-a]pyrimidines and their antimicrobial and antifungal activity. Arkivoc 2016, 277–287. doi: 10.3998/ark.5550190.p009.610
Kondratiuk, M., Gorobets, N., Sedash, Y., Gümüş, M., and Desenko, S. (2016). 5-(5-Bromo-2-hydroxy-3-methoxyphenyl)-7-methyl-4,5,6,7-tetrahydro[1,2,4]triazolo[1,5-a]pyrimidin-7-ol. Molbank 2016:M898. doi: 10.3390/M898
Konstantinova, L. S., Knyazeva, E. A., Nefyodov, A. A., Camacho, P. S., Ashbrook, S. E. M., Woollins, J. D., et al. (2015). Direct synthesis of fused 1,2,5-selenadiazoles from 1,2,5-thiadiazoles. Tetrahedron Lett. 56, 1107–1110. doi: 10.1016/j.tetlet.2015.01.106
Koopmanschap, G., Ruijter, E., and Orru, R. V. (2014). Isocyanide-based multicomponent reactions towards cyclic constrained peptidomimetics. Beilstein J. Org. Chem. 10, 544–598. doi: 10.3762/bjoc.10.50
Kour, P., Singh, V. P., Khajuria, B., Singh, T., and Kumar, A. (2017). Al(III) chloride catalyzed multi-component domino strategy: Synthesis of library of dihydrotetrazolo[1,5-a]pyrimidines and tetrahydrotetrazolo[1,5-a]quinazolinones. Tetrahedron Lett. 58, 4179–4185. doi: 10.1016/j.tetlet.2017.09.052
Krasavin, M., Tsirulnikov, S., Nikulnikov, M., Kysil, V., and Ivachtchenko, A. (2008). Poorly reactive 5-piperazin-1-yl-1,3,4-thiadiazol-2-amines rendered as valid substrates for Groebke-Blackburn type multi-component reaction with aldehydes and isocyanides using TMSCl as a promoter. Tetrahedron Lett. 49, 5241–5243. doi: 10.1016/j.tetlet.2008.06.113
Kumar, A., Kumar, M., Maurya, S., and Khanna, R. S. (2014). Regioselective synthesis of fused imidazo[1,2-a]pyrimidines via intramolecular C–N bond formation/6-endo-dig cycloisomerization. J. Org. Chem. 79, 6905–6912. doi: 10.1021/jo5007762
Kumar, D., Sonawane, M., Pujala, B., Jain, V. K., Bhagat, S., and Chakraborti, A. K. (2013). Supported protic acid-catalyzed synthesis of 2,3-disubstituted thiazolidin-4-ones: enhancement of the catalytic potential of protic acid by adsorption on solid supports. Green Chem. 15, 2872–2884. doi: 10.1039/c3gc41218k
Kumari, K., Raghuvanshi, D. S., and Singh, K. N. (2012). An expeditious synthesis of tetrahydro-1,2,4-triazolo[5,1-b]quinazolin-8(4H)-ones and dihydro-1,2,4-triazolo[1,5-a]pyrimidines. Org. Prep. Proced. Int. 44, 460–466. doi: 10.1080/00304948.2012.715062
Kurzer, F., and Godfrey, L. E. A. (1963). Syntheses of heterocyclic compounds from aminoguanidine. Angew. Chemie Int. Ed. 2, 459–476. doi: 10.1002/anie.196304591
Kysil, V., Khvat, A., Tsirulnikov, S., Tkachenko, S., Williams, C., Churakova, M., et al. (2010). General multicomponent strategy for the synthesis of 2-amino-1,4-diazaheterocycles: scope, limitations, and utility. Eur. J. Org. Chem. 2010, 1525–1543. doi: 10.1002/ejoc.200901360
Lauro, G., Manfra, M., Pedatella, S., Fischer, K., Cantone, V., Terracciano, S., et al. (2017). Identification of novel microsomal prostaglandin E2 synthase-1 (mPGES-1) lead inhibitors from fragment virtual screening. Eur. J. Med. Chem. 125, 278–287. doi: 10.1016/j.ejmech.2016.09.042
Lee, C.-H., Hsu, W.-S., Chen, C.-H., and Sun, C.-M. (2013). A telescoping synthesis of chimeric polyheterocycles through a piperidine- mediated multicomponent reaction. Eur. J. Org. Chem. 2013, 2201–2208. doi: 10.1002/ejoc.201201645
Lee, S., Koo, H., Na, J. H., Han, S. J., Min, H. S., Lee, S. J., et al. (2014). Chemical tumor-targeting of nanoparticles based on metabolic glycoengineering and click chemistry. ACS Nano 8, 2048–2063. doi: 10.1021/nn406584y
Li, L., Xu, H., Dai, L., Xi, J., Gao, L., and Rong, L. (2017). An efficient metal-free cascade process for the synthesis of 4-arylpyrimido[1,2-b]indazole-3-carbonitrile derivatives. Tetrahedron 73, 5358–5365. doi: 10.1016/j.tet.2017.07.035
Li, T., Yao, C., Yu, C., Wang, X., and Tu, S.-J. (2012). Ionic liquid-mediated one-pot synthesis of 5-(trifluoromethyl)-4,7-dihydrotetrazolo[1,5-a]pyrimidine derivatives. Synth. Commun. 42, 2728–2738. doi: 10.1080/00397911.2011.566460
Liang, L., and Astruc, D. (2011). The copper(I)-catalyzed alkyne-azide cycloaddition (CuAAC) “click” reaction and its applications. An overview. Coord. Chem. Rev. 255, 2933–2945. doi: 10.1016/j.ccr.2011.06.028
Lipson, V. V., Borodina, V. V., Zemlyanaya, N. I., Shirobokova, M. G., Musatov, V. I., Shishkina, S. V., et al. (2015). Domino reactions of 3-methyl-5-aminopyrazole with aryl(hetaryl)aldehydes, cyclopentanone, cyclopentan-1,3-dione, and 1,3-indanedione. Russ. J. Org. Chem. 51, 697–704. doi: 10.1134/S1070428015050206
Lipson, V. V., and Gorobets, N. Y. (2009). One hundred years of Meldrum's acid: advances in the synthesis of pyridine and pyrimidine derivatives. Mol. Divers. 13, 399–419. doi: 10.1007/s11030-009-9136-x
Lipson, V. V., Svetlichnaya, N. V., Borodina, V. V., Shirobokova, M. G., Shishkina, S. V., Shishkin, O. V., et al. (2010). Cascade cyclization of 3(5)-aminopyrazoles with aromatic aldehydes and cyclohexanediones. Russ. J. Org. Chem. 46, 1388–1298. doi: 10.1134/S1070428010090216
Lipson, V. V., Svetlichnaya, N. V., Shirobokova, M. G., Musatov, V. I., Shishkin, O. V., and Shishkina, S. V. (2012). Cascade cyclization of 1,2-diamino-4-phenylimidazole with aromatic aldehydes and cyclohexanediones. Russ. J. Org. Chem. 48, 273–277. doi: 10.1134/S1070428012020182
Liu, J., Lei, M., and Hu, L. (2012a). A catalyst-free reaction in water: synthesis of benzo[4,5]imidazo[1,2-a]pyrimido[4,5-d]pyrimidin-4(1H)-one derivatives. Green Chem. 14, 2534–2539. doi: 10.1039/C2GC35745C
Liu, J., Lei, M., and Hu, L. (2012b). Thiamine hydrochloride (VB1): as an efficient promoter for the one-pot synthesis of benzo[4,5]imidazo[1,2-a]pyrimidine and [1,2,4]triazolo[1,5-a] pyrimidine derivatives in water medium. Green Chem. 14, 840–846. doi: 10.1039/C2GC16499J
Ma, N., Jiang, B., Zhang, G., Tu, S., Wever, W., and Li, G. (2010). New multicomponent domino reactions (MDRs) in water: highly chemo-, regio- and stereoselective synthesis of spiro{[1,3]dioxanopyridine}-4,6-diones and pyrazolo[3,4-b]pyridines. Green Chem. 12, 1357–1361. doi: 10.1039/c0gc00073f
Mahdavinia, G. H., and Rahmati, A. (2015). Silica sulfuric acid as a solid acid catalyzed synthesis of 4,5,6,7-tetrahydro-2H-pyrazolo[3,4-b]pyridine-5-carbonitriles and 1,4-diaryl-4,5-dihydro-3-methyl-1H-pyrazolo[3,4-b]pyridine-6(7H)-ones. Rev. Roum. Chim. 60, 1025–1032.
Makarov, V. A., Braun, H., Richter, M., Riabova, O. B., Kirchmair, J., Kazakova, E. S., et al. (2015). Pyrazolopyrimidines: potent inhibitors targeting the capsid of rhino- and enteroviruses. ChemMedChem 10, 1629–1634. doi: 10.1002/cmdc.201500304
Maleki, A., Aghaei, M., and Ghamari, N. (2015). Synthesis of benzimidazolo[2,3-b]quinazolinone derivatives via a one-pot multicomponent reaction promoted by a chitosan-based composite magnetic nanocatalyst. Chem. Lett. 44, 259–261. doi: 10.1246/cl.141074
Maleki, A., Ravaghi, P., Aghaei, M., and Movahed, H. (2017). A novel magnetically recyclable silver-loaded cellulose-based bionanocomposite catalyst for green synthesis of tetrazolo[1,5-a]pyrimidines. Res. Chem. Intermed. 43, 5485–5494. doi: 10.1007/s11164-017-2941-4
Mamaghani, M., Tabatabaeian, K., Bayat, M., Nia, R. H., and Rassa, M. (2013). Regioselective synthesis and antibacterial evaluation of a new class of substituted pyrazolo[3,4-b]pyridines. J. Chem. Res. 37, 494–498. doi: 10.3184/174751913X13735444714766
Marjani, A. P., Khalafy, J., Salami, F., and Ezzati, M. (2015). The synthesis of new pyrazolo[1,5-a]pyrimidine derivatives. Arkivoc 2015, 277–286. doi: 10.3998/ark.5550190.p009.062
Martinez-Ariza, G., Nunez-Rios, J., Lee, Y.-S., and Hulme, C. (2015). Acetyl cyanide as a cyanide source in a tandem catalyst-free modified Groebke–Blackburn–Bienaymé [4 + 1]-cycloaddition-Strecker cascade. Tetrahedron Lett. 56, 1038–1040. doi: 10.1016/j.tetlet.2014.12.127
Matveeva, A. A., Matikenova, A. A., Anis'kov, A. A., and Kriven'ko, A. P. (2015). One-pot synthesis of isomeric cycloalkatriazolopyrimidines. Russ. J. Org. Chem. 51, 378–381. doi: 10.1134/S107042801503015X
Matveeva, A. A., Poplevina, N. V., Borisova, N. O., and Kriven'ko, A. P. (2013). Three-component synthesis of tetrazolopyrimidines annelated by C6 to C8 carbocycles. Chem. Heterocycl. Compd. 48, 1877–1879. doi: 10.1007/s10593-013-1224-2
Michael, A. (1893). Ueber die Einwirkung von Diazobenzolimid auf Acetylendicarbonsäuremethylester. J. Prakt. Chem. 48, 94–95. doi: 10.1002/prac.18930480114
Miliutina, M., Janke, J., Chirkina, E., Hassan, S., Ejaz, S. A., Khan, S. U., et al. (2017). Domino reactions of chromone-3-carboxylic acids with aminoheterocycles: synthesis of heteroannulated pyrido[2,3-c]coumarins and their optical and biological activity. Eur. J. Org. Chem. 2017, 7148–7159. doi: 10.1002/ejoc.201701276
Mkrtchyan, S., Iaroshenko, V. O., Dudkin, S., Gevorgyan, A., Vilches-Herrera, M., Ghazaryan, G., et al. (2010). 3-Methoxalylchromone — a novel versatile reagent for the regioselective purine isostere synthesis. Org. Biomol. Chem. 8, 5280–5284. doi: 10.1039/c0ob00379d
Moderhack, D. (2011). N-hydroxy- and N-aminotetrazoles and their derivatives – synthesis and reactions. Heterocycles 83, 1435–1487. doi: 10.3987/REV-11-693
Mohammed, S., Vishwakarma, R. A., and Bharate, S. B. (2015). Iodine catalyzed oxidative synthesis of quinazolin-4(3H)-ones and pyrazolo[4,3-d]pyrimidin-7(6H)-ones via amination of sp3 C–H bond. J. Org. Chem. 80, 6915–6921. doi: 10.1021/acs.joc.5b00989
Moradi, A., Heydari, R., and Maghsoodlou, M. T. (2015). Agar: a novel, efficient, and biodegradable catalyst for the one-pot three-component and green synthesis of 2,3-dihydroquinazolin-4(1H)-one, 4H-pyrimidobenzothiazole and 2-aminobenzothiazolomethylnaphthol derivatives. Res. Chem. Intermed. 41, 7377–7391. doi: 10.1007/s11164-014-1818-z
Morozova, A. D., Muravyova, E. A., Desenko, S. M., Musatov, V. I., Yedamenko, D. V., and Chebanov, V. A. (2016). Heterocyclization reactions of 3-methylisoxazol-5-amine with pyruvic acid derivatives using classical and non-classical methods of activation. Chem. Heterocycl. Compd. 52, 934–942. doi: 10.1007/s10593-017-1989-9
Morozova, A. D., Muravyova, E. A., Shishkina, S. V., Vashchenko, E. V., Sen'ko, Y. V., and Chebanov, V. A. (2017). Diversity-oriented multicomponent heterocyclizations involving derivatives of 3(5)-aminoisoxazole, aldehydes and meldrum's or N,N′-dimethylbarbituric acid. J. Heterocycl. Chem. 54, 932–943. doi: 10.1002/jhet.2656
Mulakayala, N., Kandagatla, B., Ismail Rapolu, R. K., Rao, P., Mulakayala, C., et al. (2012). InCl3-catalysed synthesis of 2-aryl quinazolin-4(3H)-ones and 5-aryl pyrazolo[4,3-d]pyrimidin-7(6H)-ones and their evaluation as potential anticancer agents. Bioorganic Med. Chem. Lett. 22, 5063–5066. doi: 10.1016/j.bmcl.2012.06.003
Muravyova, E. A., Desenko, S. M., Rudenko, R. V., Shishkina, S. V., Shishkin, O. V., Sen'ko, Y. V., et al. (2011). Switchable selectivity in multicomponent heterocyclizations of acetoacetamides, aldehydes, and 3-amino-1,2,4-triazoles/5-aminopyrazoles. Tetrahedron 67, 9389–9400. doi: 10.1016/j.tet.2011.09.138
Muravyova, E. A., Shishkina, S. V., Musatov, V. I., Knyazeva, I. V., Shishkin, O. V., Desenko, S. M., et al. (2009). Chemoselectivity of multicomponent condensations of barbituric acids, 5-aminopyrazoles, and aldehydes. Synthesis 2009, 1375–1385. doi: 10.1055/s-0028-1088024
Muravyova, E. A., Tkachenko, V. V., Desenko, S. M., Sen'ko, Y. V., Müller, T. J. J., Vashchenko, E. V., et al. (2013). Behavior of 5-amino-3-methylisoxazole in multicomponent heterocyclizations with carbonyl compounds under thermal heating and non-classical conditions. Arkivoc 2013, 338–371. doi: 10.3998/ark.5550190.p008.093
Murlykina, M. V., Kornet, M. N., Desenko, S. M., Shishkina, S. V., Shishkin, O. V., Brazhko, A. A., et al. (2017). New tricks of well-known aminoazoles in isocyanide-based multicomponent reactions and antibacterial activity of the compounds synthesized. Beilstein J. Org. Chem. 13, 1050–1063. doi: 10.3762/bjoc.13.104
Murlykina, M. V., Sakhno, Y. I., Desenko, S. M., Konovalova, I. S., Shishkin, O. V., Sysoiev, D. A., et al. (2013). Features of switchable multicomponent heterocyclizations of salicylic aldehydes and 5-aminopyrazoles with pyruvic acids and antimicrobial activity of the reaction products. Tetrahedron 69, 9261–9269. doi: 10.1016/j.tet.2013.08.055
Murlykina, M. V., Sakhno, Y. I., Desenko, S. M., Shishkina, S. V., Shishkin, O. V., Sysoiev, D. O., et al. (2015). Study of the chemoselectivity of multicomponent heterocyclizations involving 3-amino-1,2,4-triazole and pyruvic acids as key reagents, and biological activity of the reaction products. Eur. J. Org. Chem. 2015, 4481–4492. doi: 10.1002/ejoc.201500469
Murugesan, V., Makwana, N., Suryawanshi, R., Saxena, R., Tripathi, R., Paranjape, R., et al. (2014). Rational design and synthesis of novel thiazolidin-4-ones as non-nucleoside HIV-1 reverse transcriptase inhibitors. Bioorganic Med. Chem. 22, 3159–3170. doi: 10.1016/j.bmc.2014.04.018
Niu, X., Yang, B., Fang, S., Li, Y., Zhang, Z., Jia, J., et al. (2014). An efficient one-pot synthesis of 1,2,4-triazoloquinoxalines. Tetrahedron 70, 4657–4660. doi: 10.1016/j.tet.2014.05.029
Orlov, V. D., and Sidorenko, D. Y. (2012). Carbo[3 + 3] cyclocondensation reactions. A new method for the synthesis of tetrahydropyrazolo[1,5-b]quinazolines and tetrahydropyrazolo[4,5-b]quinolines. Chem. Heterocycl. Compd. 48, 650–657. doi: 10.1007/s10593-012-1039-6
Palaniraja, J., Mohana Roopan, S., Mokesh Rayalu, G., Abdullah Al-Dhabi, N., and Valan Arasu, M. (2016a). A metal-free regioselective multicomponent approach for the synthesis of free radical scavenging pyrimido-fused indazoles and their fluorescence studies. Molecules 21:1571. doi: 10.3390/molecules21111571
Palaniraja, J., and Roopan, S. M. (2015). Iodine-mediated synthesis of indazolo-quinazolinones via a multi-component reaction. RSC Adv. 5, 8640–8646. doi: 10.1039/C4RA13779E
Palaniraja, J., Roopan, S. M., and Rayalu, G. M. (2016b). One-pot synthesis of highly functionalized pyrimido[1,2-b]indazoles via 6-endo-dig cyclization. RSC Adv. 6, 24610–24616. doi: 10.1039/C6RA02596J
Patnaik, S., Zheng, W., Choi, J. H., Motabar, O., Southall, N., Westbroek, W., et al. (2012). Discovery, structure–activity relationship, and biological evaluation of noninhibitory small molecule chaperones of glucocerebrosidase. J. Med. Chem. 55, 5734–5748. doi: 10.1021/jm300063b
Pereshivko, O. P., Peshkov, V. A., Ermolat'ev, D. S., and Van Der Eycken, E. V. (2013). Fast Assembly of 1H-Imidazo[1,2-a]imidazol-5-amines via Groebke–Blackburn–Bienaymé reaction with 2-aminoimidazoles. Synlett 24, 351–354. doi: 10.1055/s-0032-1317986
Petrov, A. A., and Kasatochkin, A. N. (2013). Synthesis of 6,7,8,9-Tetrahydropyrazolo[1,5-a]quinazolines containing a 5-phenylamine fragment. Russ. J. Org. Chem. 49, 1250–1252. doi: 10.1134/S1070428013080307
Petrov, A. A., and Kasatochkin, A. N. (2014). Convenient synthesis of 6,7-dihydroazolo[5,1-b]quinazolin-8(5H)-one derivatives. Russ. J. Org. Chem. 50, 1485–1495. doi: 10.1134/S1070428014100145
Petrova, O. N., Lipson, V. V., Zamigailo, L. L., Shirobokova, M. G., Musatov, V. I., Baumer, V. N., et al. (2015a). Synthesis and chemical properties of 4-aroyl-3-methyl-4,10-dihydroindeno[1,2-b]pyrazolo-[4,3-e]pyridin-5-ones. Russ. J. Org. Chem. 51, 1597–1605. doi: 10.1134/S1070428015110147
Petrova, O. N., Lipson, V. V., Zamigajlo, L. L., Shirobokova, M. G., Musatov, V. I., and Dmitrienko, D. A. (2016). Domino reactions of pyrazol-5-amines with arylglyoxals and malononitrile. Russ. J. Org. Chem. 52, 1168–1172. doi: 10.1134/S1070428016080121
Petrova, O. N., Zamigailo, L. L., Shirobokova, M. G., Shishkina, S. V., Shishkin, O. V., Musatov, V. I., et al. (2013a). Cyclocondensation of 3(5)-aminopyrazoles with arylglyoxals and cyclohexane-1,3-diones. Chem. Heterocycl. Compd. 49, 955–967. doi: 10.1007/s10593-013-1332-z
Petrova, O. N., Zamigajlo, L. L., Gella, I. M., Musatov, V. I., Shishkina, S. V., Shishkin, O. V., et al. (2014). Three-component synthesis of 4-aroyl-2(1),4,5,7-tetrahydropyrazolo[3,4-b]pyridin-6-ones and their properties. Chem. Heterocycl. Compd. 50, 514–527. doi: 10.1007/s10593-014-1502-7
Petrova, O. N., Zamigajlo, L. L., Ostras, K. S., Shishkina, S. V., Shishkin, O. V., Borisov, A. V., et al. (2015b). Multicomponent reaction of 2-aminobenzimidazole, arylglyoxals, and 1,3-cyclohexanedione. Chem. Heterocycl. Compd. 51, 310–319. doi: 10.1007/s10593-015-1700-y
Petrova, O. N., Zamigajlo, L. L., Shishkina, S. V., Shishkin, O. V., Musatov, V. I., Borisov, A. V., et al. (2013b). A facile one-pot highly chemo- and regioselective synthesis of the novel heterocyclic system indolo[1,2-c]azolo[1,5-a]quinazoline-8,10-dione. Tetrahedron 69, 11185–11190. doi: 10.1016/j.tet.2013.10.073
Pinner, A. (1894). Ueber die Einwirkung von Hydrazin auf Imidoäther. Berichte Dtsch. Chem. Gesellsch. 27, 984–1009. doi: 10.1002/cber.189402701207
Planells, M., Nikolka, M., Hurhangee, M., Tuladhar, P. S., White, A. J. P., Durrant, J. R., et al. (2014). The effect of thiadiazole out-backbone displacement in indacenodithiophene semiconductor polymers. J. Mater. Chem. C 2, 8789–8795. doi: 10.1039/C4TC01500B
Pokhodylo, N. T., and Shyyka, O. Y. (2014). 1-(5-(R-Amino)-1,2,4-thiadiazol-3-yl)propan-2-ones: convenient ketomethylenic reagents for the Gewald and Dimroth reactions. J. Heterocycl. Chem. 51, 1487–1490. doi: 10.1002/jhet.1719
Proshin, A. N., Serkov, I. V., Petrova, L. N., and Bachurin, S. O. (2014). 5-Amino-3-(2-aminopropyl)-1,2,4-thiadiazoles as the basis of hybrid multifunctional compounds. Russ. Chem. Bull. 63, 1148–1152. doi: 10.1007/s11172-014-0563-1
Puligoundla, R. G., Karnakanti, S., Bantu, R., Kommu, N., Kondra, S. B., and Nagarapu, L. (2013). A simple, convenient one-pot synthesis of [1,2,4]triazolo/benzimidazolo quinazolinone derivatives by using molecular iodine. Tetrahedron Lett. 54, 2480–2483. doi: 10.1016/j.tetlet.2013.02.099
Quiroga, J., Cobo, D., Insuasty, B., Abonía, R., Cruz, S., Nogueras, M., et al. (2008). Regioselective three-component synthesis of novel indeno[1,2-b]pyrazolo[4,3-e]pyridines-fused derivatives of 4-azafluorenone alkaloid. J. Heterocycl. Chem. 45, 155–159. doi: 10.1002/jhet.5570450116
Rahmati, A. (2010). Synthesis of 4-aryl-3-methyl-6-oxo-4,5,6,7-tetrahydro-2H-pyrazolo[3,4-b]pyridine-5-carbonitrile via a one-pot, three-component reaction. Tetrahedron Lett. 51, 2967–2970. doi: 10.1016/j.tetlet.2010.03.109
Rahmati, A., Eskandari-Vashareh, M., and Alizadeh-Kouzehrash, M. (2013). Synthesis of 3-(benzylideneamino)-2-phenyl-5H-imidazo[1,2-b]pyrazole-7-carbonitriles via a four-component condensation reaction. Tetrahedron 69, 4199–4204. doi: 10.1016/j.tet.2013.03.103
Rahmati, A., and Khalesi, Z. (2012). Catalyst free synthesis of fused pyrido[2,3-d]pyrimidines and pyrazolo[3,4-b]pyridines in water. Chinese Chem. Lett. 23, 1149–1152. doi: 10.1016/j.cclet.2012.08.009
Rahmati, A., and Kouzehrash, M. (2011). Synthesis of N-alkyl-2-aryl-5H-imidazo[1,2-b]pyrazol-3-amines by a three-component condensation reaction. Synthesis 2011, 2913–2920. doi: 10.1055/s-0030-1260154
Rajanarendar, E., Murthy, K. R., and Reddy, M. N. (2011a). A mild and efficient four component one-pot synthesis of 2,4,5-triphenyl-(1H-1-imidazolyl)isoxazoles catalyzed by ceric ammonium nitrate. Indian J. Chem. 50B, 926–930.
Rajanarendar, E., Reddy, M. N., and Raju, S. (2011b). An efficient one-pot synthesis of isoxazolyl polyhydroquinolines via Hantzsch condensation using L-proline as catalyst. Indian J. Chem. 50B, 751–755.
Rajanarendar, E., Thirupathaiah, K., Ramakrishna, S., and Nagaraju, D. (2016). A convenient and facile Hantzsch synthesis of aryl imidazo[1,2-b]Isoxazolyl-N-aryl thiazol amines. J. Heterocycl. Chem. 53, 1983–1989. doi: 10.1002/jhet.2518
Rajua, C., Kalaipriyab, M., Umaa, R., Sridhar, R., and Ramakrishna, S. (2012). Pyridinium trifluoro acetate mediated synthesis of 3,4-dihydropyrimidin-2(1H)-ones and tetrazolo[1,5-a]pyrimidine-6-carboxylates. Curr. Chem. Lett. 1, 27–34. doi: 10.5267/j.ccl.2011.12.004
Rateb, N. M. (2014). Synthesis of pyrido[2′,3′:3,4]pyrazolo[1,5-a]pyrimidine, pyrido[2′,3′:3,4]pyrazolo[5,1-c][1,2,4]triazine, and pyrazolyl oxadiazolylthieno[2,3-b]pyridine derivatives. J. Heterocycl. Chem. 51, 1349–1356. doi: 10.1002/jhet.1799
Reddy, M. V., Byeon, K. R., Park, S. H., and Kim, D. W. (2017). Polyethylene glycol methacrylate-grafted dicationic imidazolium-based ionic liquid: heterogeneous catalyst for the synthesis of aryl-benzo[4,5]imidazo[1,2-a]pyrimidine amines under solvent-free conditions. Tetrahedron 73, 5289–5296. doi: 10.1016/j.tet.2017.07.025
Reddy, M. V., Kim, J. S., Lim, K. T., and Jeong, Y. T. (2014a). Polyethylene glycol (PEG-400): an efficient green reaction medium for the synthesis of benzo[4,5]imidazo[1,2-a]pyrimido[4,5-d]pyrimidin-4(1H)-ones under catalyst-free conditions. Tetrahedron Lett. 55, 6459–6462. doi: 10.1016/j.tetlet.2014.09.135
Reddy, M. V., Oh, J., and Jeong, Y. T. (2014b). p-Toluenesulfonic acid-catalyzed one-pot synthesis of 2-amino-4-substituted-1,4-dihydrobenzo[4,5]imidazolo[1,2-a]pyrimidine-3-carbonitriles under neat conditions. Comptes Rendus Chim. 17, 484–489. doi: 10.1016/j.crci.2013.08.007
Reddy, M. V., Reddy, A. V. S., and Jeong, Y. T. (2016). Di-n-butyl ammonium chlorosulfonate ionic liquids as an efficient and recyclable catalyst for the synthesis of 1,4-dihydrobenzo[4,5]imidazo[1,2-a]pyrimidine-3-carboxylates under solvent-free ultrasound irradiation. Res. Chem. Intermed. 42, 4893–4906. doi: 10.1007/s11164-015-2328-3
Ren, L., Laird, E. R., Buckmelter, A. J., Dinkel, V., Gloor, S. L., Grina, J., et al. (2012). Potent and selective pyrazolo[1,5-a]pyrimidine based inhibitors of B-RafV600E kinase with favorable physicochemical and pharmacokinetic properties. Bioorganic Med. Chem. Lett. 22, 1165–1168. doi: 10.1016/j.bmcl.2011.11.092
Risley, V. A., Henry, S., Kosyrikhina, M. V., Manzanares, M. R., Payan, I., Downer, C. D., et al. (2014). 4-Amino-2-aryl-3-cyano-1,2-dihydropyrimido-[1,2-a]benzimidazoles and their pyrimidine analogs as new anticancer agents. Chem. Heterocycl. Compd. 50, 185–194. doi: 10.1007/s10593-014-1460-0
Rodríguez-Rodríguez, M., Gras, E., Pericàs, M. A., and Gómez, M. (2015). Metal-free intermolecular azide-alkyne cycloaddition promoted by glycerol. Chem. A Eur. J. 21, 18706–18710. doi: 10.1002/chem.201503858
Rostovtsev, V. V., Green, L. G., Fokin, V. V., and Sharpless, K. B. (2002). A stepwise Huisgen cycloaddition process: copper(I)-catalyzed regioselective “ligation” of azides and terminal alkynes. Angew. Chemie Int. Ed. 41, 2596–2599. doi: 10.1002/1521-3773(20020715)41:14<2596::AID-ANIE2596>3.0.CO;2-4
Ruijter, E., Scheffelaar, R., and Orru, R. V. A. (2011). Multicomponent reaction design in the quest for molecular complexity and diversity. Angew. Chemie Int. Ed. 50, 6234–6246. doi: 10.1002/anie.201006515
Ryabukhin, S. V., Panov, D. M., Plaskon, A. S., and Grygorenko, O. O. (2012). Approach to the library of 3-hydroxy-1,5-dihydro-2H-pyrrol-2-ones through a three-component condensation. ACS Comb. Sci. 14, 631–635. doi: 10.1021/co300082t
Ryabukhin, S. V., Plaskon, A. S., Boron, S. Y., Volochnyuk, D. M., and Tolmachev, A. A. (2011). Aminoheterocycles as synthons for combinatorial Biginelli reactions. Mol. Divers. 15, 189–195. doi: 10.1007/s11030-010-9253-6
Saeedi, M., Beheshtiha, Y. S., Heravi, M. M., and Oskooie, H. A. (2011). Synthesis of novel tetrahydro[4,5]imidazo[2,1-b]chromeno[4,3,2-de] quinazoline and benzothiazol-2-ylaminoxanthenone derivatives. Heterocycles 83, 1831–1841. doi: 10.3987/COM-11-12201
Safaei, S., Mohammadpoor-Baltork, I., Khosropour, A. R., Moghadam, M., Tangestaninejad, S., and Mirkhani, V. (2012). Regioselective multi-component synthesis of 1H-pyrazolo[3,4-d]pyrimidine-6(7H)-thiones. Mol. Divers. 16, 591–600. doi: 10.1007/s11030-012-9391-0
Sahi, S., and Paul, S. (2016). Synthesis and biological evaluation of quinolines, thiazolo[3,2-a]pyrimidines, thiadiazolo[3,2-a]pyrimidines and triazolo[3,4-b][1,3,4]thiadiazepines as antimicrobial agents. Med. Chem. Res. 25, 951–969. doi: 10.1007/s00044-016-1540-z
Saikia, P., Kaishap, P. P., Prakash, R., Shekarrao, K., Gogoi, S., and Boruah, R. C. (2014). A facile one-pot synthesis of 7-substituted pyrazolo[1,5-a]pyrimidines by base induced three-component reaction. Tetrahedron Lett. 55, 3896–3900. doi: 10.1016/j.tetlet.2014.05.021
Saito, T., Obitsu, T., Minamoto, C., Sugiura, T., Matsumura, N., Ueno, S., et al. (2011). Pyrazolo[1,5-a]pyrimidines, triazolo[1,5-a]pyrimidines and their tricyclic derivatives as corticotropin-releasing factor 1 (CRF1) receptor antagonists. Bioorganic Med. Chem. 19, 5955–5966. doi: 10.1016/j.bmc.2011.08.055
Sakhno, Y. I., Desenko, S. M., Shishkina, S. V., Shishkin, O. V., Musatov, V. I., and Chebanov, V. A. (2011). Unusual direction of cyclocondensation of 1-(4-chlorophenyl)-3,5-diamino-1,2,4-triazole, pyruvic acid, and aldehydes. Synthesis 1120–1124. doi: 10.1055/s-0030-1258468
Sakhno, Y. I., Desenko, S. M., Shishkina, S. V., Shishkin, O. V., Sysoyev, D. O., Groth, U., et al. (2008). Multicomponent cyclocondensation reactions of aminoazoles, arylpyruvic acids and aldehydes with controlled chemoselectivity. Tetrahedron 64, 11041–11049. doi: 10.1016/j.tet.2008.09.089
Sakhno, Y. I., Kozyryev, A. V., Desenko, S. M., Shishkina, S. V., Musatov, V. I., Sysoiev, D. O., et al. (2018). Features of two- and multicomponent heterocyclization reactions involving 3,4-disubstituted 5-aminopyrazoles and alkyl pyruvates. Tetrahedron 74, 564–571. doi: 10.1016/j.tet.2017.12.031
Sakhno, Y. I., Murlykina, M. V., Morozova, A. D., Kozyryev, A. V., and Chebanov, V. A. (2015). Heterocyclization reactions of pyruvic acids and aminoazoles with controlled chemoselectivity. French Ukrainian J. Chem. 3, 1–20. doi: 10.17721/fujcV3I2P1-20
Sakhno, Y. I., Shishkina, S. V., Shishkin, O. V., Musatov, V. I., Vashchenko, E. V., Desenko, S. M., et al. (2010). Diversity oriented heterocyclizations of pyruvic acids, aldehydes and 5-amino-N-aryl-1H-pyrazole-4-carboxamides: catalytic and temperature control of chemoselectivity. Mol. Divers. 14, 523–531. doi: 10.1007/s11030-010-9226-9
Satasia, S. P., Kalaria, P. N., and Raval, D. K. (2014). Catalytic regioselective synthesis of pyrazole based pyrido[2,3-d] pyrimidine-diones and their biological evaluation. Org. Biomol. Chem. 12, 1751–1758. doi: 10.1039/b000000x
Sedash, Y. V., Gorobets, N. Y., Chebanov, V. A., Konovalova, I. S., Shishkin, O. V., and Desenko, S. M. (2012). Dotting the i's in three-component Biginelli-like condensations using 3-amino-1,2,4-triazole as a 1,3-binucleophile. RSC Adv. 2, 6719–6728. doi: 10.1039/c2ra20195j
Shaaban, S., and Abdel-Wahab, B. F. (2016). Groebke–Blackburn–Bienaymé multicomponent reaction: emerging chemistry for drug discovery. Mol. Divers. 20, 233–254. doi: 10.1007/s11030-015-9602-6
Shaabani, A., and Hooshmand, S. E. (2016). Choline chloride/urea as a deep eutectic solvent/organocatalyst promoted three-component synthesis of 3-aminoimidazo-fused heterocycles via Groebke–Blackburn–Bienayme process. Tetrahedron Lett. 57, 310–313. doi: 10.1016/j.tetlet.2015.12.014
Shaabani, A., Seyyedhamzeh, M., Ganji, N., Hamidzad Sangachin, M., and Armaghan, M. (2015). One-pot four-component synthesis of highly substituted [1,2,4]triazolo[1,5-a]pyrimidines. Mol. Divers. 19, 709–715. doi: 10.1007/s11030-015-9604-4
Shaabani, A., Seyyedhamzeh, M., Maleki, A., Behnam, M., and Rezazadeh, F. (2009). Synthesis of fully substituted pyrazolo [3,4-b]pyridine-5-carboxamide derivatives via a one-pot four-component reaction. Tetrahedron Lett. 50, 2911–2913. doi: 10.1016/j.tetlet.2009.03.200
Shafiee, M., Khosropour, A. R., Mohammadpoor-Baltork, I., Moghadam, M., Tangestaninejad, S., and Mirkhani, V. (2012). An efficient, expeditious, and diastereoselective one-pot pseudo-five-component reaction for the synthesis of new bis-Betti bases under catalyst-free conditions. Tetrahedron Lett. 53, 3086–3090. doi: 10.1016/j.tetlet.2012.04.037
Shao, T., Gong, Z., Su, T., Hao, W., and Che, C. (2017). A practical and efficient approach to imidazo[1,2-a]pyridine-fused isoquinolines through the post-GBB transformation strategy. Beilstein J. Org. Chem. 13, 817–824. doi: 10.3762/bjoc.13.82
Shaterian, H. R., Fahimi, N., and Azizi, K. (2014). New applications of phosphoric acid supported on alumina (H3PO4-Al2O3) as a reusable heterogeneous catalyst for preparation of 2,3-dihydroquinazoline-4(1H)-ones, 2H-indazolo[2,1-b]phthalazinetriones, and benzo[4,5]imidazo[1,2-a]pyrimidines. Res. Chem. Intermed. 40, 1879–1898. doi: 10.1007/s11164-013-1087-2
Sheibani, H., and Babaie, M. (2013). Three-component synthesis of 4-amino-2-aryl-2H-pyrimido-[1,2-b][1,3]benzazole-3-carbonitriles and 4H-pyrimido-[2,1-b][1,3]benzazoles in the presence of magnesium oxide and 12-tungstophosphoric acid as catalysts. Russ. Chem. Bull. 62, 2202–2208. doi: 10.1007/s11172-013-0319-3
Sheibani, H., Saidi, K., and Lakaei, M. (2012). Three-component one-pot synthesis of 4-aryl-2,3-dihydropyrimido[1,2-a]benzimidazol-2-ones catalyzed by L-proline. J. Heterocycl. Chem. 49, 1386–1390. doi: 10.1002/jhet
Shen, S., Zhang, H., Yu, C., Yao, C., Li, T., Qin, B., et al. (2013). Solvent-free combinatorial synthesis of tetrazolo[1,5-a]thiopyrano[3,4-d]pyrimidine derivatives. Res. Chem. Intermed. 39, 1799–1806. doi: 10.1007/s11164-012-0714-7
Shi, C.-L., Chen, H., and Shi, D.-Q. (2011). An efficient one-pot synthesis of pyrazolo[3,4-b]pyridinone derivatives catalyzed by L-proline. J. Heterocycl. Chem. 48, 351–354. doi: 10.1002/jhet.573
Shi, D.-Q., Yang, F., and Ni, S.-N. (2009). A facile synthesis of furo[3,4-e]pyrazolo[3,4-b]pyridine-5(7H)-one derivatives via three-component reaction in ionic liquid without any catalyst. J. Heterocycl. Chem. 46, 469–476. doi: 10.1002/jhet.103
Shi, F., Zhou, D., Tu, S., Li, C., Cao, L., and Shao, Q. (2008). Pot, atom and step economic synthesis of fused three heterocyclic ring compounds under microwave irradiation in water. J. Heterocycl. Chem. 45, 1305–1310. doi: 10.1002/jhet.5570450508
Shie, J.-J., and Fang, J.-M. (2007). Microwave-assisted one-pot tandem reactions for direct conversion of primary alcohols and aldehydes to triazines and tetrazoles in aqueous media. J. Org. Chem. 72, 3141–3144. doi: 10.1021/jo0625352
Shieh, P., Dien, V. T., Beahm, B. J., Castellano, J. M., Wyss-Coray, T., and Bertozzi, C. R. (2015). CalFluors: a universal motif for fluorogenic azide probes across the visible spectrum. J. Am. Chem. Soc. 137, 7145–7151. doi: 10.1021/jacs.5b02383
Shinde, V. V., and Jeong, Y. T. (2015). Molybdate sulfuric acid (MSA): an efficient solid acid catalyst for the synthesis of diversely functionalized fused imidazo[1,2-a]pyrimidines under solvent-free conditions. New J. Chem. 39, 4977–4986. doi: 10.1039/C5NJ00516G
Shinde, V. V., and Jeong, Y. T. (2016). Sonochemical FeF3 catalyzed three-component synthesis of densely functionalized tetrahydroindazolo[3,2-b]quinazoline under solvent-free conditions. Tetrahedron Lett. 57, 3795–3799. doi: 10.1016/j.tetlet.2016.07.031
Shirame, S. P., and Bhosale, R. B. (2018). “Green approach in click chemistry,” in Green Chemistry, eds H. E. M. Saleh and M. Kolle (London: InTech).
Singh, M. S., Chowdhury, S., and Koley, S. (2016). Advances of azide-alkyne cycloaddition-click chemistry over the recent decade. Tetrahedron 72, 5257–5283. doi: 10.1016/J.TET.2016.07.044
Soliman, A. M. M., El-Remaily, M. A. E.-A. A. A., Sultan, A. A., and Abdel-Ghany, H. (2014). Synthesis of some novel imidazopyrazole and pyrazolopyrimidine derivatives. J. Heterocycl. Chem. 51, 1476–1481. doi: 10.1002/jhet.1701
Sompalle, R., Arunachalam, P., and Roopan, S. M. (2016). Conventional spectroscopic identification of N-alkylated triazolo-quinazolinones and its antioxidant, solvatochromism studies. J. Mol. Liq. 224, 1348–1357. doi: 10.1016/j.molliq.2016.10.124
Sompalle, R., and Roopan, S. M. (2016). Microwave assisted synthesis of ring junction heterocyclic antioxidants. Res. Chem. Intermed. 42, 5353–5366. doi: 10.1007/s11164-015-2371-0
Suresh, L., Kumar, P. S. V., Vinodkumar, T., and Chandramouli, G. V. P. (2016). Heterogeneous recyclable nano-CeO2 catalyst: efficient and eco-friendly synthesis of novel fused triazolo and tetrazolo pyrimidine derivatives in aqueous medium. RSC Adv. 6, 68788–68797. doi: 10.1039/C6RA16307F
Survase, D., Bandgar, B., and Helavi, V. (2017). Polyethylene glycol-promoted synthesis of pyrimido[1,2-a]benzimidazole and pyrano[2,3-c]pyrazole derivatives in water. Synth. Commun. 47, 680–687. doi: 10.1080/00397911.2017.1278774
Světlík, J., and Kettmann, V. (2011). The chameleon-like behaviour of 3-amino-1,2,4-triazole in the Biginelli reaction: unexpected formation of a novel spiroheterocyclic system. Tetrahedron 52, 1062–1066. doi: 10.1016/j.tetlet.2010.12.051
Svetlík, J., Pronayova, N., Svor, L., and Frecer, V. (2014). A complicated path of salicylaldehyde through the Biginelli reaction: a case of unexpected spiroketalization. Tetrahedron 70, 8354–8360. doi: 10.1016/j.tet.2014.09.003
Svetlik, J., Veizerová, L., Mayer, T. U., and Catarinella, M. (2010). Monastrol analogs: A synthesis of pyrazolopyridine, benzopyranopyrazolopyridine, and oxygen-bridged azolopyrimidine derivatives and their biological screening. Bioorganic Med. Chem. Lett. 20, 4073–4076. doi: 10.1016/j.bmcl.2010.05.085
Tan, S. H., Chuah, T. S., and Chia, P. W. (2016). An improved protocol on the synthesis of thiazolo [3,2-a]pyrimidine using ultrasonic probe irradiation. J. Korean Chem. Soc. 60, 245–250. doi: 10.5012/jkcs.2016.60.4.245
Taylor, A. P., Robinson, R. P., Fobian, Y. M., Blakemore, D. C., Jones, L. H., and Fadeyi, O. (2016). Modern advances in heterocyclic chemistry in drug discovery. Org. Biomol. Chem. 14, 6611–6637. doi: 10.1039/C6OB00936K
Thomas, E. W. (1993). The conversion of secondary amides to tetrazoles with trifluoromethanesulfonic anhydride and sodium azide. Synthesis 1993, 767–768. doi: 10.1055/s-1993-25934
Thorat, V. V., Dake, A. S., Deshmukh, U. S., Rasokkiyam, E., Farees Uddin, M., and Pawar, R. P. (2013). Ionic liquid mediated synthesis of novel tetrahydroimidazo[1,2-a]pyrimidine-6-carboxylate derivatives. Lett. Org. Chem. 10, 178–184. doi: 10.2174/1570178611310030006
Tintori, C., La Sala, G., Vignaroli, G., Botta, L., Fallacara, A. L., Falchi, F., et al. (2015). Studies on the ATP binding site of Fyn kinase for the identification of new inhibitors and their evaluation as potential agents against tauopathies and tumors. J. Med. Chem. 58, 4590–4609. doi: 10.1021/acs.jmedchem.5b00140
Tireli, M., Maračić, S., Lukin, S., Kulcsár, M. J., Žilić, D., Cetina, M., et al. (2017). Solvent-free copper-catalyzed click chemistry for the synthesis of N-heterocyclic hybrids based on quinoline and 1,2,3-triazole. Beilstein J. Org. Chem. 13, 2352–2363. doi: 10.3762/bjoc.13.232
Tkachenko, V. V., and Chebanov, V. A. (2016). Reactions of 3(5)-aminoisoxazoles using classical methods of activation, microwave irradiation, and ultrasonication. Chem. Heterocycl. Compd. 52, 866–886. doi: 10.1007/s10593-017-1980-5
Tkachenko, V. V., Muravyova, E. A., Desenko, S. M., Shishkin, O. V., Shishkina, S. V., Sysoiev, D. O., et al. (2014a). The unexpected influence of aryl substituents in n-aryl-3-oxobutanamides on the behavior of their multicomponent reactions with 5-amino-3-methylisoxazole and salicylaldehyde. Beilstein J. Org. Chem. 10, 3019–3030. doi: 10.3762/bjoc.10.320
Tkachenko, V. V., Muravyova, E. A., Shishkina, S. V., Shishkin, O. V., Desenko, S. M., and Chebanov, V. A. (2014b). Study of three-component reactions between 5-amino-3-methylisoxazole, n-arylamides of acetoacetic acid, and aromatic aldehydes. Chem. Heterocycl. Compd. 50, 1166–1176. doi: 10.1007/s10593-014-1578-0
Todorovic, N., Awuah, E., Shakya, T., Wright, G. D., and Capretta, A. (2011). Microwave-assisted synthesis of N1- and C3-substituted pyrazolo[3,4-d]pyrimidine libraries. Tetrahedron Lett. 52, 5761–5763. doi: 10.1016/j.tetlet.2011.08.103
Tornøe, C. W., Christensen, C., and Meldal, M. (2002). Peptidotriazoles on solid phase: [1,2,3]-triazoles by regiospecific copper(I)-catalyzed 1,3-dipolar cycloadditions of terminal alkynes to azides. J. Org. Chem. 67, 3057–3064. doi: 10.1021/jo011148j
Tu, S. J., Zhang, X. H., Han, Z. G., Cao, X. D., Wu, S. S., Yan, S., et al. (2009). Synthesis of isoxazolo[5,4-b]pyridines by microwave-assisted multi-component reactions in water. J. Comb. Chem. 11, 428–432. doi: 10.1021/cc800212v
Tyagi, V., Khan, S., Bajpai, V., Gauniyal, H. M., Kumar, B., and Chauhan, P. M. S. (2012). Skeletal diverse synthesis of N-fused polycyclic heterocycles via the sequence of ugi-type MCR and CuI-catalyzed coupling/tandem Pictet–Spengler reaction. J. Org. Chem. 77, 1414–1421. doi: 10.1021/jo202255v
Urich, R., Wishart, G., Kiczun, M., Richters, A., Tidten-Luksch, N., Rauh, D., et al. (2013). De novo design of protein kinase inhibitors by in silico identification of hinge region-binding fragments. ACS Chem. Biol. 8, 1044–1052. doi: 10.1021/cb300729y
Vibhute, S., Jamale, D., Undare, S., Valekar, N., Kolekar, G., and Anbhule, P. (2017a). An efficient, one-pot three components synthesis of [1,2,4] triazoloquinazolinone derivatives using anthranilic acid as green catalyst. Res. Chem. Intermed. 43, 4561–4574. doi: 10.1007/s11164-017-2896-5
Vibhute, S., Jamale, D., Undare, S., Valekar, N., Patil, K., Kolekar, G., et al. (2017b). A bio-oriented anthranilic acid catalyzed synthesis of quinazolin-8 (4H)-one derivatives: evaluation by green chemistry metrics. Synth. Commun. 47, 1747–1757. doi: 10.1080/00397911.2017.1348526
Vidyacharan, S., Shinde, A. H., Satpathi, B., and Sharada, D. S. (2014). A facile protocol for the synthesis of 3-aminoimidazo-fused heterocycles via the Groebke–Blackburn–Bienayme reaction under catalyst-free and solvent-free conditions. Green Chem. 16, 1168–1175. doi: 10.1039/c3gc42130a
Vitaku, E., Smith, D. T., and Njardarson, J. T. (2014). Analysis of the structural diversity, substitution patterns, and frequency of nitrogen heterocycles among U.S. FDA Approved Pharmaceuticals. J. Med. Chem. 57, 10257–10274. doi: 10.1021/jm501100b
Voitekhovich, S. V., Ivashkevich, O. A., and Gaponik, P. N. (2013). Synthesis, properties, and structure of tetrazoles: certain achievements and prospects. Russ. J. Org. Chem. 49, 635–654. doi: 10.1134/S1070428013050011
Voitekhovich, S. V., Vorob'ev, A. N., Gaponik, P. N., and Ivashkevich, O. A. (2005). Synthesis of new functionally substituted 1-R-tetrazoles and their 5-amino derivatives. Chem. Heterocycl. Compd. 41, 999–1004. doi: 10.1007/s10593-005-0267-4
Wadhwa, P., Kaur, T., and Sharma, A. (2015). The first catalyst and solvent-free synthesis of 2-arylimidazo[2,1-b][1,3,4]thiadiazoles: a comparative assessment of greenness. RSC Adv. 5, 44353–44360. doi: 10.1039/C5RA06747B
Wang, H., Lee, M., Peng, Z., Blázquez, B., Lastochkin, E., Kumarasiri, M., et al. (2015a). Synthesis and evaluation of 1,2,4-triazolo[1,5-a]pyrimidines as antibacterial agents against Enterococcus faecium. J. Med. Chem. 58, 4194–4203. doi: 10.1021/jm501831g
Wang, H.-Y., and Shi, D.-Q. (2012). Three-component one-pot synthesis of pyrazolo[3,4-b]quinolin-5(6H)-one derivatives in aqueous media. J. Heterocycl. Chem. 49, 212–216. doi: 10.1002/jhet.781
Wang, J. J., Feng, X., Xun, Z., Shi, D. Q., and Huang, Z. B. (2015b). Multicomponent strategy to pyrazolo[3,4-e]indolizine derivatives under microwave irradiation. J. Org. Chem. 80, 8435–8442. doi: 10.1021/acs.joc.5b01314
Wang, S. L., Liu, Y. P., Xu, B. H., Wang, X. H., Jiang, B., and Tu, S. J. (2011). Microwave-assisted chemoselective reaction: a divergent synthesis of pyrazolopyridine derivatives with different substituted patterns. Tetrahedron 67, 9417–9425. doi: 10.1016/j.tet.2011.09.081
Willer, R. L., Storey, R. F., Campbell, C. G., Bunte, S. W., and Parrish, D. (2012). A re-examination of the reaction of 3,4-diamino[1,2,5]oxadiazole with glyoxal. J. Heterocycl. Chem. 49, 919–925. doi: 10.1002/jhet.1054
Wittenberger, S. J. (1994). Recent developments in tetrazole chemistry. A review. Org. Prep. Proced. Int. 26, 499–531. doi: 10.1080/00304949409458050
Worrell, B. T., Malik, J. A., and Fokin, V. V. (2013). Direct evidence of a dinuclear copper intermediate in Cu(I)-catalyzed azide-alkyne cycloadditions. Science 340, 457–460. doi: 10.1126/science.1229506
Wu, J., Yu, L., Yang, F., Li, J., Wang, P., Zhou, W., et al. (2014). Optimization of 2-(3-(arylalkyl amino carbonyl) phenyl)-3-(2-methoxyphenyl)-4-thiazolidinone derivatives as potent antitumor growth and metastasis agents. Eur. J. Med. Chem. 80, 340–351. doi: 10.1016/j.ejmech.2014.04.068
Wu, Y. M., Deng, J., Li, Y., and Chen, Q. Y. (2005). Regiospecific synthesis of 1,4,5-trisubstituted-1,2,3-triazole via one-pot reaction promoted by copper(I) salt. Synthesis, 1314–1318. doi: 10.1055/s-2005-861860
Yoshida, M., Mori, A., Morimoto, S., Kotani, E., Oka, M., Notoya, K., et al. (2011). Novel and potent calcium-sensing receptor antagonists: discovery of (5R)-N-[1-ethyl-1-(4-ethylphenyl)propyl]-2,7,7-trimethyl-5-phenyl-4,5,6,7-tetrahydropyrazolo[1,5-a]pyrimidine-3-carboxamide monotosylate (TAK-075) as an orally active bone anabolic agent. Bioorganic Med. Chem. 19, 1881–1894. doi: 10.1016/j.bmc.2011.02.001
Zarganes-Tzitzikas, T., Chandgude, A. L., and Dömling, A. (2015). Multicomponent reactions, union of MCRs and beyond. Chem. Rec. 15, 981–996. doi: 10.1002/tcr.201500201
Zeng, L.-Y., Ji, F., and Cai, C. (2012). Four-component tandem reaction to synthesize dihydrotetrazolopyrimidinyl carbamides. J. Heterocycl. Chem. 49, 237–241. doi: 10.1002/jhet.698
Zeng, L.-Y., Ren, Y.-M., and Cai, C. (2011). Iodine-catalyzed, multicomponent, one-pot synthesis of 5-aryl-5,8-dihydrotetrazolo[1,5-a]pyrimidine-7-carboxylic acids. Synth. Commun. 41, 3635–3643. doi: 10.1080/00397911.2010.519842
Zhang, Y., Li, X., Li, J., Chen, J., Meng, X., Zhao, M., et al. (2012). CuO-promoted construction of N-2-aryl-substituted-1,2,3-triazoles via azide-chalcone oxidative cycloaddition and post-triazole arylation. Org. Lett. 14, 26–29. doi: 10.1021/ol202718d
Zhang, Z.-T., Ma, Y.-Q., Liang, Y., Xue, D., and He, Q. (2011). An efficient one-pot synthesis of diarylpyrazolo[1,5-a]pyrimidine from isoflavones. J. Heterocycl. Chem. 48, 279–285. doi: 10.1002/jhet.546
Zhao, B., Jiang, L.-L., Liu, Z., Deng, Q.-G., Wang, L.-Y., Song, B., et al. (2013). A microwave assisted synthesis of highly substituted 7-methyl-5H-thiazolo[3,2-a]pyrimidine-6-carboxylate derivatives via one-pot reaction of aminothiazole, aldehyde and ethyl acetoacetate. Heterocycles 87, 2093–2102. doi: 10.3987/COM-13-12797
Zhao, B., Xu, Y., Deng, Q. G., Liu, Z., Wang, L. Y., and Gao, Y. (2014). One-pot, three component synthesis of novel 5H-[1,3,4]thiadiazolo[3,2-a]pyrimidine-6-carboxylate derivatives by microwave irradiation. Tetrahedron Lett. 55, 4521–4524. doi: 10.1016/j.tetlet.2014.06.073
Keywords: aminoazole, diversity-oriented synthesis, heterocycle, heterocyclization reaction, multicomponent reaction, microwave-assisted organic synthesis, ultrasonication, click-chemistry
Citation: Murlykina MV, Morozova AD, Zviagin IM, Sakhno YI, Desenko SM and Chebanov VA (2018) Aminoazole-Based Diversity-Oriented Synthesis of Heterocycles. Front. Chem. 6:527. doi: 10.3389/fchem.2018.00527
Received: 28 June 2018; Accepted: 11 October 2018;
Published: 13 November 2018.
Edited by:
Andrea Basso, Università di Genova, ItalyReviewed by:
Thomas J. J. Müller, Heinrich-Heine-Universität Düsseldorf, GermanyTatiana Besset, UMR6014 Chimie Organique, Bioorganique Réactivité et Analyse (COBRA), France
Copyright © 2018 Murlykina, Morozova, Zviagin, Sakhno, Desenko and Chebanov. This is an open-access article distributed under the terms of the Creative Commons Attribution License (CC BY). The use, distribution or reproduction in other forums is permitted, provided the original author(s) and the copyright owner(s) are credited and that the original publication in this journal is cited, in accordance with accepted academic practice. No use, distribution or reproduction is permitted which does not comply with these terms.
*Correspondence: Valentyn A. Chebanov, Y2hlYmFub3ZAaXNjLmtoLnVh