- Department of Chemistry, CRI Center for Chemical Proteomics, Seoul National University, Seoul, South Korea
In the interdisciplinary research field of chemical biology and drug discovery, diversity-oriented synthesis (DOS) has become indispensable in the construction of novel small-molecule libraries rich in skeletal and stereochemical diversity. DOS aims to populate the unexplored chemical space with new potential bioactive molecules via forward synthetic analysis. Since the introduction of this concept by Schreiber, DOS has evolved along with many significant breakthroughs. It is therefore important to understand the key DOS strategies to build molecular diversity with maximized biological relevancy. Due to the length limitations of this mini review, we briefly discuss the recent DOS plans using build/couple/pair (B/C/P) and ring-distortion strategies for the synthesis of major biologically relevant target molecules like natural products and their related compounds, macrocycles, and privileged structures.
Introduction
Small molecules play an indispensable role in the fields of drug discovery and chemical biology due to their unique features compared to biologics, polymers, and nanoparticles (Samanen, 2013). However, while the knowledge of biological systems has grown in the post-genomic era, the discovery of novel small molecular therapeutics or bioprobes has become more complicated. This can be attributed to advances in chemical biology and drug discovery disclosing novel targets beyond conventional druggable proteins, such as DNA (Hurley, 2002), RNA (Lieberman, 2018; Warner et al., 2018), protein–protein interactions (PPIs) (Scott et al., 2016), and protein–RNA interactions (PRIs) (Hentze et al., 2018), among others. Furthermore, a lack of information regarding the structures and modes of action of these novel targets renders rational drug discovery challenging.
The development of high-throughput screening (HTS) and high-content screening (HCS) enabled rapid and efficient investigation of biological activities to yield existing drug-like compound libraries constructed by combinatorial synthesis (Schreiber, 2000; Tan, 2005; Basso, 2012). However, contrary to expectations, extensive screening exercises against huge compound libraries delivered a relatively small number of new chemical entities, particularly in the case of bioassays for novel undruggable targets or unbiased phenotypic screenings, where rational ligand design is challenging (Burke and Schreiber, 2004; Galloway et al., 2010; Garcia-Castro et al., 2016). This may be due to the limited diversity of conventional drug-like compound libraries, especially in terms of skeletal and stereochemical diversity. Indeed, it should be noted that skeletal diversity is essential for specific binding events with diverse biopolymers bearing three-dimensional (3D) unique binding sites and structural diversity (Kim et al., 2014). In fact, it is not the size of a chemical library that is most important, but the skeletal and stereochemical diversity of its core structures. Thus, there is a huge demand for high-quality compound collections through the efficient construction of drug-like small molecule libraries enriched with molecular diversity, and particularly, skeletal and stereochemical diversity (Spring, 2003).
To meet such demands in molecular diversity, Schreiber et al. introduced the concept of diversity-oriented synthesis (DOS) (Schreiber, 2000). The aim of DOS as a synthetic strategy is to occupy the unexplored parts of chemical space via the efficient synthesis of unique compound collections bearing diversity and complexity in their scaffolds. DOS involves “forward synthetic analysis,” where the products of each step become the branching substrates for subsequent steps (Tan, 2005). Hence, the DOS approach leads to an exponential increase in the molecular diversity of chemical libraries through multiple systematic branching sequences. Indeed, the DOS strategy has attested its capacity and value through the development of various novel therapeutic agents and biological modulators and through advancing biological understandings (Kuruvilla et al., 2002; Kuo et al., 2015; Schreiber et al., 2015; Hideshima et al., 2016; Kato et al., 2016; Plouffe et al., 2016; Wellington et al., 2017; Gerry and Schreiber, 2018). For example, Schreiber et al. discovered a novel multistage antimalarial inhibitor, BRD7929, through the extensive screening of their compound library constructed by DOS strategy (Kato et al., 2016), while Park et al. reported a novel leucyl-tRNA synthetase/RagD PPI inhibitor discovered from DOS library (Kim et al., 2016).
Since the DOS concept was introduced, many synthetic pathways have been developed by various research groups to construct efficient chemical libraries (Nielsen and Schreiber, 2008). Among them, the build/couple/pair (B/C/P) strategy is the most widely followed and commonly applicable synthetic strategy. This strategy involves 3 synthetic phases, namely a build phase, a couple phase, and a pair phase (Figure 1A; Burke and Schreiber, 2004). More specifically, the build phase involves the synthesis of a single or multiple key building blocks embedded with suitable functional groups for later-stage coupling reactions. In the couple phase, a variety of intermolecular coupling reactions can be employed to generate a dense array of reactive sites and functional groups on the key building blocks installed during the build phase. Finally, in the pair phase, the intermediates constructed through the build and couple phases take part in intramolecular pairing reactions to yield an array of final products with the desired skeletal and stereochemical diversity (Nielsen and Schreiber, 2008; Kim et al., 2016).
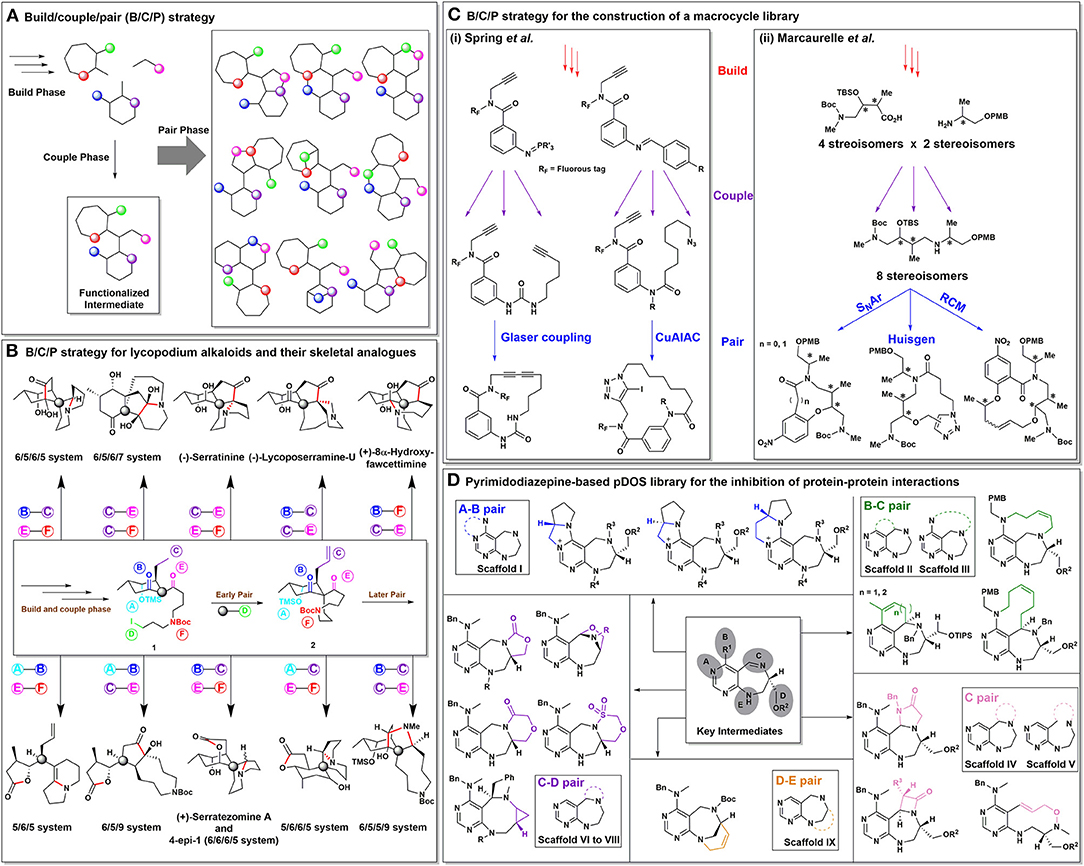
Figure 1. (A) A schematic representation of the B/C/P strategy. (B) Outline of lycopodium alkaloids and their unnatural scaffolds with their respective pairing patterns. (C) Outline of the B/C/P strategy for the construction of libraries consisting of macrocycles and medium-sized rings. (D) A pDOS library established via the B/C/P strategy for the inhibition of protein–protein interactions.
Recently, the ring-distortion strategy has also been developed as a distinctive DOS strategy for the systematic construction of novel small-molecule collections with high structural diversity and complexity. In contrast to the B/C/P strategy, the ring-distortion strategy is distinct in that it pursues molecular diversity via distortion of the existing ring systems through ring-cleavage, ring-expansion, ring-contraction, ring-fusion, ring-rearrangement, ring-aromatization, and combinations of the above (Figure 2A; Huigens et al., 2013).
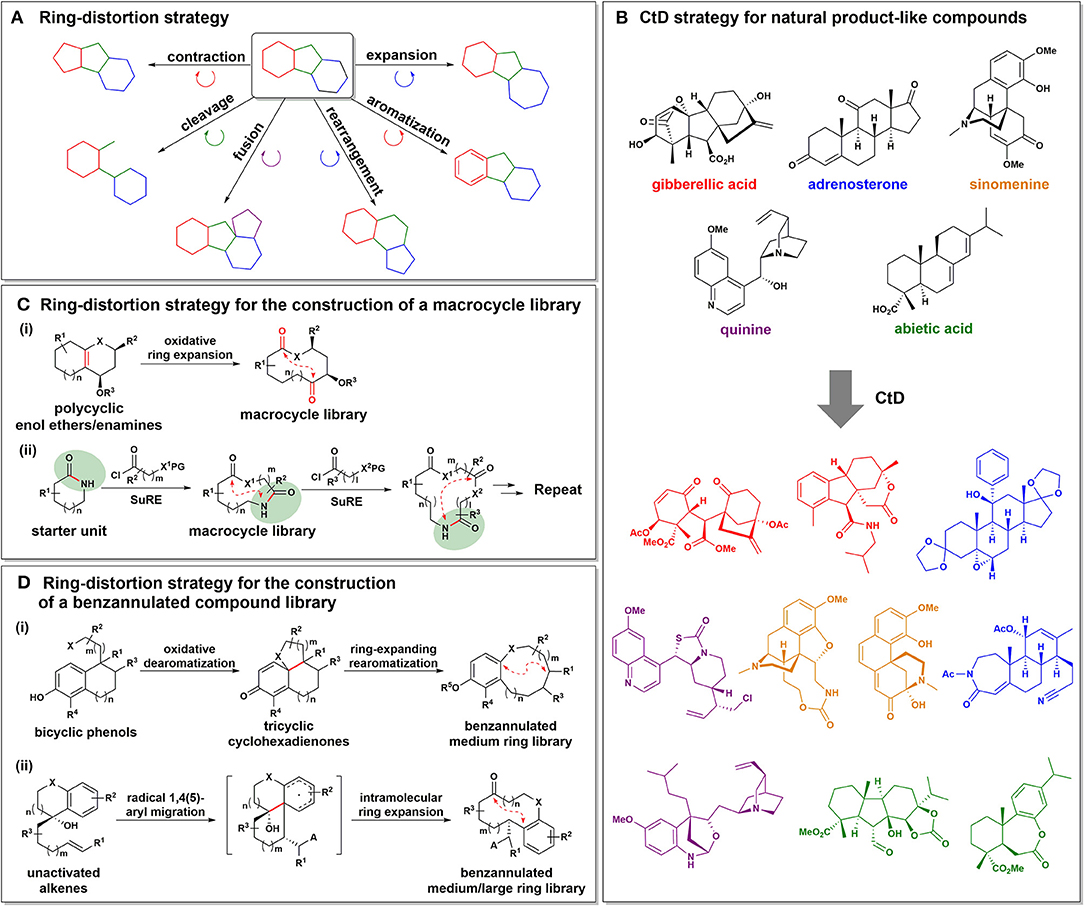
Figure 2. (A) Schematic representation of the ring-distortion strategy. (B) The complexity-to-diversity (CtD) strategy for the construction of diverse and complex compounds starting from readily available natural products. (C) The ring-distortion strategy for the construction of macrocyclic lactone and lactam libraries. (D) The ring-distortion strategy for the construction of biologically relevant benzannulated small molecules.
However, in any DOS strategy, the common structural features of existing bioactive molecules have been widely investigated to grant sufficient biological relevancy to the resulting compounds (Kim et al., 2014). As such, natural products are commonly investigated, and their structural features are considered to be potent sources of information in drug discovery. In addition, macrocycles have received a significant amount of attention in the field of drug discovery due to distinguishable structural features compared to other small molecules. Furthermore, privileged structures, which are common structural motifs in a vast number of bioactive natural products and therapeutic agents, contain novel structural features that secure a high biological relevancy (Evans et al., 1988).
Thus, in this mini review, we present recent advancements in the B/C/P and ring-distortion DOS approaches in the context of natural products, natural product-like compounds, macrocycles, and privileged structures.
The Build/Couple/Pair (B/C/P) Strategy
Synthesis of Natural Products and Natural Product-Like Compounds via the B/C/P Strategy
Natural products play a pivotal role in the search of novel therapeutics. Bioactive natural products tend to have complex 3D polycyclic structures rich in sp3 carbons and stereogenic centers, and their inherent bioactivities may provide clues for the design of novel core skeletons with high biological relevancy (Wipf, 2012; Shimokawa, 2014; Chen et al., 2015). Therefore, the efficient construction of natural product libraries and their unnatural analogs can be considered an important DOS strategy.
A team led by Lei proposed that complex molecules such as bioactive natural products can be synthesized via the B/C/P strategy through the pairing of various functional groups present in their structures (Zhang et al., 2014). Selecting lycopodium alkaloids as a model system, they reported the total syntheses of four lycopodium alkaloids and six related unnatural scaffolds. In contrast to other total synthetic approaches, the reported DOS approach allowed the parallel synthesis of unnatural scaffolds, thereby increasing the population of “lycopodium-like” natural products in the unexplored chemical space (Harayama et al., 1974; Ma and Gang, 2004; Chandra et al., 2009). As shown in Figure 1B, chiral intermediate 1 was formed through the build and couple phases, while intermediate 2 was prepared by means of the early pairing phase, which was crucial to the overall synthetic protocol. By encompassing double pairing processes in a sequential manner, the “later pairing phase” allowed the efficient construction of unique core skeletons. To illustrate the power of this B/C/P strategy, stepwise double pairing procedures, such as B–C pairing and E–F pairing in Figure 1B led to the total synthesis of (+)-serratezomine A (6/6/6/5 system) and an unnatural skeletal analog (6/5/6/5 system) of (–)-serratinine. In addition, the double pairing pattern involving A–B pairing followed by C–E or E–F pairing led to the formation of tricyclic compounds (6/5/9 or 5/6/5 systems, respectively). Other pairing patterns leading to the total syntheses of (–)-serratinine, (+)-8α-hydroxyfawcettimine, (–)-lycoposerramine-U, and three tetracyclic unnatural scaffolds were also examined. Overall, this work demonstrated a unique and efficient route to the synthesis of complex natural product-like molecules using the B/C/P strategy.
Synthesis of Macrocycles via the B/C/P Strategy
Although various macrocycle-based natural products are known to exhibit therapeutic potential, as a sole structural unit, macrocycles have not been traditionally considered as suitable small molecules for drug discovery screening processes (Schreiber, 2000). However, recent reports have claimed that macrocyclic structures can pre-organize their conformations, which allows improved interactions with extended protein surfaces and subsequent high biological activities (Driggers et al., 2008; Villar et al., 2014). As such, numerous DOS strategies have been pursued to construct structurally and functionally diverse macrocycles (Madsen and Clausen, 2011; Collins et al., 2016).
For the efficient construction of libraries containing a diverse array of macrocycles, Spring et al. developed advanced B/C/P approaches (Beckmann et al., 2013; Nie et al., 2016). These B/C/P approaches not only allowed diversification in the multi-dimensional pattern, but also resulted in the judicious modification of the chemical structures following the pairing phase (Figure 1Ci). Using an advanced B/C/P approach, they also reported the synthesis of a library containing 73 macrocycles having 59 different scaffolds (Beckmann et al., 2013). In this case, the build phase involved the synthesis of fluorous-tagged azido compounds, which were converted in situ into the corresponding pluripotent aza-ylides. These aza-ylides were then coupled with suitable appendages to facilitate the subsequent pairing reactions. Similarly, in 2016, they reported the synthesis of 45 diverse macrocyclic compounds of various sizes, ranging from 15- to 33-membered rings (Nie et al., 2016). In this case, the imine moieties branching out from the aza-ylides served as second-line building blocks for diversification of the macrocycle library. The introduction and subsequent modification of the fluorous tag and other reactive sites in these macrocycles could therefore improve the efficiency as well as skeletal diversity of the library synthesis.
Moreover, Marcaurelle et al. utilized an aldol-based B/C/P strategy to construct a library containing in excess of 30,000 compounds, which were based on a variety of skeletons ranging from 8- to 14-membered rings, of which 14,400 compounds were macrolactams aimed at the discovery of novel histone deacetylase inhibitors (Figure 1Cii; Marcaurelle et al., 2010). Notably, this study presented an excellent example of the DOS strategy to demonstrate its power and efficiency for the highly systematic construction of small-molecule libraries with maximized architectural complexity.
The B/C/P Strategy in the pDOS Pathway
A clear definition of privileged structures was made in a seminal article on drug discovery methods reported by Evans et al. (1988). More specifically, they stated that “privileged structures are capable of providing useful ligands for more than one receptor and that judicious modification of such structures could be a viable alternative in the search for new receptor agonists and antagonists.” Based on the concept of modification around privileged structures, a number of groups have reported various bio-relevant compounds, with many successfully delivering clinical candidates as well as FDA-approved drugs (Mason et al., 1999; Nicolaou et al., 2000a,b,c; Brohm et al., 2002; Kissau et al., 2003; Newman, 2008). For example, Nicolaou et al. published a series of articles on the combinatorial library syntheses of natural product-like compounds in which the benzopyran skeleton was employed as a privileged structure (Nicolaou et al., 2000a,b,c). In this context, the construction of a DOS library derived from privileged structures can be considered crucial to accessing highly biologically relevant molecular diversity (Kim et al., 2014).
We envisioned that incorporating these privileged structures into polyheterocycles enhances the biological relevancy of the resulting compounds with pre-defined conformations, which may be beneficial for specific binding with biopolymers due to the prepaid entropic penalty (Oh and Park, 2011; Kim et al., 2014; Lenci et al., 2016). Hence, within the theme of DOS, our group introduced a novel design strategy, namely “privileged substructure-based diversity-oriented synthesis” (pDOS), which aims to populate the chemical space with privileged substructure-embedded polyheterocycles (An et al., 2008; Oh and Park, 2011; Zhu et al., 2012; Kim et al., 2013, 2014). In particular, the systematic construction of diverse sp3-rich 3D polyheterocycles containing privileged substructures has been emphasized since their rigid and diverse frameworks can selectively bind with biopolymers to induce conformational changes and subsequent functional modulation. Thus, a small-molecule library constructed by the pDOS strategy could be considered an excellent resource for the discovery of specific modulators of protein–protein and protein–DNA/RNA interactions.
In addition, we recently reported a pDOS library in which pyrimidodiazepines were employed as the privileged substructure (Kim et al., 2016). We found that the 6/7-bicyclic pyrimidodiazepine system demonstrated a significantly higher conformational flexibility with more reactive sites compared to those of pyrimidine-embedded 6/6 or 6/5 systems. In this case, the build and couple phases produced key pyrimidodiazepine-based intermediates containing five orthogonal reactive sites. In the pair phase, each reactive site was paired to produce 16 different polyheterocycles containing the pyrimidodiazepine substructure and with a high degree of 3D skeletal complexity in nine distinct scaffolds. As shown in Figure 1D, A–B pairing and B–C pairing led to the synthesis of tetracyclic and tricyclic compounds, respectively (scaffolds I–III). Due to the dual (i.e., electrophilic and nucleophilic) nature of the imine moiety, the C pairing allowed the synthesis of scaffolds IV and V. Using the C–D and D–E pairing combinations, scaffolds VI–IX were also constructed. Based on our HTS screening endeavors against this pDOS library, we identified aziridine-containing pyrimidodiazepines from scaffold VIII (constructed through C–D pairing) as a novel small-molecule inhibitor of the leucine tRNA synthetase (LRS)–RagD protein–protein interaction.
The Ring-Distortion Strategy
Synthesis of Natural Product-Like Compounds via the Ring-Distortion Strategy
For the construction of natural product-like compound collections, Hergenrother et al. developed a novel approach starting from natural products, known as the complexity-to-diversity (CtD) strategy (Huigens et al., 2013; Rafferty et al., 2014; Garcia et al., 2016). In this approach, the molecular frameworks of readily available natural products were converted into structurally complex and diverse core skeletons through various chemoselective ring-distortion reactions (Figure 2B). As natural products exhibit an inherent structural complexity with defined stereochemistry (Clardy and Walsh, 2004), the resulting core skeletons derived from natural products tend to be structurally and stereochemically more complex and distinct compared to existing compound collections. In their initial report on the CtD strategy, gibberellic acid, quinine, and adrenosterone were employed as synthetic starting points, and were transformed into 19, 12, and 18 different scaffolds, respectively, through various ring-distortion reactions (3 reaction steps on average; Huigens et al., 2013). The subsequent application of traditional diversification strategies to final scaffolds therefore allowed the construction of a 119-membered highly complex compound library. They also applied the CtD strategy to other readily available natural products such as abietic acid and sinomenine, which afforded 84 and 65 complex compounds, respectively (Rafferty et al., 2014; Garcia et al., 2016). Chemoinformatic analysis of the resulting compound collections obtained using the CtD strategy demonstrated a higher skeletal complexity compared to conventional compound collections in terms of higher fractions of sp3-hybridized carbon atoms (Fsp3), lower clogP values, and greater numbers of stereocenters.
Synthesis of Macrocycles via the Ring-Distortion Strategy
For the systematic construction of diverse macrocycles, several DOS approaches utilizing ring-distortion reactions (and in particular, ring-expansion reactions) have been pursued (Kopp et al., 2012; Kitsiou et al., 2015; Stephens et al., 2017, 2018). For example, Tan et al. reported an efficient oxidative ring-expansion strategy for the construction of diverse macrocyclic small molecule collections (Figure 2Ci; Kopp et al., 2012). Interestingly, easily accessible polycyclic enol ethers or enamines containing bridging double bonds were found to smoothly undergo oxidative cleavage to generate various macrolactones and macrolactams, regardless of substrate effects, such as ring size, substituents, and stereochemistry. Subsequent transformations using functional handles in the macrocyclic scaffolds afforded additional structural diversity. In addition, the chemoinformatic analysis of 32 unprecedented macrocyclic compounds using principal component analysis (PCA) and principal moments of inertia (PMI) analysis illustrated the possibilities of the resulting macrocycles to modulate novel biological targets through occupying unique chemical space distinct from the current synthetic drugs.
Moreover, the successive ring-expansion (SuRE) strategy described by Unsworth et al. led to the generation of structurally diverse macrocyclic lactams and lactones in a sequential manner (Kitsiou et al., 2015; Stephens et al., 2017, 2018). As shown in Figure 2Cii, the amide functionality present in the cyclic starter unit enabled coupling with the linear fragment via an acylation reaction, and subsequent deprotection and ring-opening along with chain incorporation yielded the ring-expanded product. The key strength of the SuRE method is that the same coupling and ring-expansion sequence can be repeated as the reactive amide functionality is regenerated in the product. Using this simple SuRE strategy, a functionalized macrocycle library was successfully constructed.
Synthesis of Biologically Relevant Benzannulated Compounds via the Ring-Distortion Strategy
Benzannulated medium/macro- or bridged rings are common structural moieties in a number of bioactive natural products and pharmacologically significant synthetic compounds such as penicillide, zeranol, and rifampin (Salituro et al., 1993; Fürstner et al., 1999; Yu and Sun, 2013; Hussain et al., 2014). In this context, Tan et al. developed an efficient biomimetic ring-expansion approach to construct diverse benzannulated medium-sized rings via an oxidative dearomatization and ring-expanding rearomatization sequence (Figure 2Di; Bauer et al., 2013). This strategy involves the oxidative dearomatization of bicyclic phenol precursors to provide polycyclic cyclohexadienones and a subsequent ring-expansion driven by rearomatization of the phenol ring to afford benzannulated medium-sized rings. The structural and physicochemical similarities between the resulting 47 scaffolds and benzannulated medium ring-based natural products were confirmed by PCA analysis.
Furthermore, Liu et al. reported a radical-based diversity-oriented synthetic approach for the fabrication of 37 discrete benzannulated medium/macro- or bridged-rings in a stereoselective manner (Figure 2Dii; Li et al., 2016). In this strategy, the radical 1,4- or 1,5-aryl migration of unactivated alkenes and subsequent intramolecular ring-expansion provided benzannulated medium or large rings. Additional ring-distortion reactions of the resulting core skeletons afforded novel medium-sized and medium-bridged rings with high regio- and stereoselectivities. PCA analysis and preliminary biological studies confirmed the significant biological relevance of this compound collection.
Conclusion
In this mini review, we briefly emphasized the important roles of diversity-oriented synthesis (DOS) in the field of drug discovery and chemical biology, and introduced the most common DOS strategies for the construction of novel small molecule libraries with maximized molecular diversity. We also discussed two key diversity-oriented synthetic approaches (i.e., the build/couple/pair (B/C/P) strategy and the ring-distortion strategy) and visualized how each strategy allows design of the resulting scaffolds with high biological relevancy via the incorporation of key structural elements such as bioactive natural products, macrocycles, and privileged structures. We concluded that both the B/C/P strategy and the ring-distortion strategy are powerful approaches for the creation of a number of diverse and complex scaffolds in an efficient manner. The combination of DOS-based molecular diversity and unbiased phenotypic screening may shed light on the unraveled signaling pathways and other intricate biological processes by allowing the sustainable supply of new drug candidates and chemical probes.
Author Contributions
SY, BV, and YC contributed equally to this manuscript. SY, BV, and YC collected the related references and prepared the manuscript. SP directed the preparation of this manuscript. All authors critically reviewed the text and figures prior to submission.
Funding
This work was supported by the Creative Research Initiative Grant (2014R1A3A2030423), Bio & Medical Technology Development Program (2012M3A9C4048780), and A3 Foresight International Cooperation Program (2016K2A9A2A10005504) through the National Research Foundation of Korea (NRF) funded by the Korean Government (Ministry of Science & ICT).
Conflict of Interest Statement
The authors declare that the research was conducted in the absence of any commercial or financial relationships that could be construed as a potential conflict of interest.
Acknowledgments
We appreciate Mr. Chanwoo Kim and Miss Jaeyoung Koo of our laboratory at Seoul National University for their helpful discussion about this manuscript. SY is grateful for the NRF-2017-Fostering Core Leaders of the Future Basic Science Program/Global Ph.D. Fellowship Program (2017H1A2A1045200).
References
An, H., Eum, S.-J., Koh, M., Lee, S. K., and Park, S. B. (2008). Diversity-oriented synthesis of privileged benzopyranyl heterocycles from s-cis-enones. J. Org. Chem. 73, 1752–1761. doi: 10.1021/jo702196f
Basso, A. (2012). Diversity oriented synthesis: how and why? Diversity Oriented Synthesis. Nat. Commun. 1, 1–5. doi: 10.2478/dos-2012-0001
Bauer, R. A., Wenderski, T. A., and Tan, D. S. (2013). Biomimetic diversity-oriented synthesis of benzannulated medium rings via ring expansion. Nat. Chem. Biol. 9, 21–29. doi: 10.1038/nchembio.1130
Beckmann, H. S. G., Nie, F., Hagerman, C. E., Johansson, H., Tan, Y. S., Wilcke, D., et al. (2013). A strategy for the diversity-oriented synthesis of macrocyclic scaffolds using multidimensional coupling. Nat. Chem. 5, 861–867. doi: 10.1038/nchem.1729
Brohm, D., Philippe, N., Metzger, S., Bhargavba, A., Müller, O., Lieb, F., et al. (2002). Solid-phase synthesis of dysidiolide-derived protein phosphatase inhibitors. J. Am. Chem. Soc. 124, 13171–13178. doi: 10.1021/ja027609f
Burke, M. D., and Schreiber, S. L. (2004). A planning strategy for diversity-oriented synthesis. Angew. Chem. Int. Ed. 43, 46–58. doi: 10.1002/anie.200300626
Chandra, A., Pigza, J. A., Han, J.-S., Mutnick, D., and Johnston, J. N. (2009). Total synthesis of the Lycopodium alkaloid (+)-serratezomine A. J. Am. Chem. Soc. 131, 3470–3471. doi: 10.1021/ja900536d
Chen, Q.-F., Wang, F.-P., and Liu, X.-Y. (2015). Generating skeletal diversity from the C19-diterpenoid alkaloid deltaline: a ring-distortion approach. Chem. Eur. J. 21, 8946–8950. doi: 10.1002/chem.201500839
Clardy, J., and Walsh, C. (2004). Lessons from natural molecules. Nature 432, 829–837. doi: 10.1038/nature03194
Collins, S., Bartlett, S., Nie, F., Sore, H. F., and Spring, D. R. (2016). Diversity-oriented synthesis of macrocycle libraries for drug discovery and chemical biology. Synthesis 48, 1457–1473. doi: 10.1055/s-0035-1561414
Driggers, E. M., Hale, S. P., Lee, J., and Terrett, N. K. (2008). The exploration of macrocycles for drug discovery–an underexploited structural class. Nat. Rev. Drug Discov. 7, 608–624. doi: 10.1038/nrd2590
Evans, B. E., Rittle, K. E., Bock, M. G., Dipardo, R. M., Freidinger, R. M., Whitter, W. L., et al. (1988). Methods for drug discovery: development of potent, selective, orally effective cholecystokinin antagonists. J. Med. Chem. 31, 2235–2246. doi: 10.1021/jm00120a002
Fürstner, A., Seidel, G., and Kindler, N. (1999). Macrocycles by ring-closing-metathesis, XI: syntheses of (R)-(+)-lasiodiplodin, zeranol, and truncated salicylihalamides. Tetrahedron 55, 8215–8230. doi: 10.1016/S0040-4020(99)00302-6
Galloway, W. R. J. D., Isidro-Llobet, A., and Spring, D. R. (2010). Diversity-oriented synthesis as a tool for the discovery of novel biologically active small molecules. Nat. Commun. 1, 80–92. doi: 10.1038/ncomms1081
Garcia, A., Drown, B. S., and Hergenrother, P. J. (2016). Access to a structurally complex compound collection via ring distortion of the alkaloid sinomenine. Org. Lett. 18, 4852–4855. doi: 10.1021/acs.orglett.6b02333
Garcia-Castro, M., Zimmermann, S., Sankar, M. G., and Kumar, K. (2016). Scaffold diversity synthesis and its application in probe and drug discovery. Angew. Chem. Int. Ed. 55, 7586–7605. doi: 10.1002/anie.201508818
Gerry, C. J., and Schreiber, S. L. (2018). Chemical probes and drug leads from advances in synthetic planning and methodology. Nat. Rev. Drug Discov. 17, 333–352. doi: 10.1038/nrd.2018.53
Harayama, T., Ohtani, M., Oki, M., and Inubushi, Y. (1974). Total synthesis of the lycopodium alkaloid (±)-serratinine. J. Chem. Soc., Chem. Commun. 827–828.
Hentze, M. W., Castello, A., Schwarzl, T., and Preiss, T. (2018). A brave new world of RNA-binding proteins. Nat. Rev. Mol. Cell Biol. 19, 327–341. doi: 10.1038/nrm.2017.130
Hideshima, T., Qi, J., Paranal, R. M., Tang, W., Greenberg, E., West, N., et al. (2016). Discovery of selective small-molecule HDAC6 inhibitor for overcoming proteasome inhibitor resistance in multiple myeloma. Proc. Natl. Acad. Sci. U.S.A.113, 13162–13167. doi: 10.1073/pnas.1608067113
Huigens, R. W. I. I. I., Morrison, K. C., Hicklin, R. W., Flood, T. A. Jr., Richter, M. F., and Hergenrother, P. J. (2013). A ring-distortion strategy to construct stereochemically complex and structurally diverse compounds from natural products. Nat. Chem. 5, 195–202. doi: 10.1038/nchem.1549
Hurley, L. H. (2002). DNA and its associated processes as targets for cancer therapy. Nat. Rev. Cancer 2, 188–200. doi: 10.1038/nrc749
Hussain, A., Yousuf, S. K., and Mukherjee, D. (2014). Importance and synthesis of benzannulated medium-sized and macrocyclic rings (BMRs). RSC Adv. 4, 43241–43257. doi: 10.1039/C4RA07434C
Kato, N., Comer, E., Sakata-Kato, T., Sharma, A., Sharma, M., Maetani, M., et al. (2016). Diversity-oriented synthesis yields novel multistage antimalarial inhibitors. Nature 538, 344–349. doi: 10.1038/nature19804
Kim, H., Tung, T. T., and Park, S. B. (2013). Privileged substructure-based diversity-oriented synthesis pathway for diverse pyrimidine-embedded polyheterocycles. Org. Lett. 15, 5814–5817. doi: 10.1021/ol402872b
Kim, J., Jung, J., Koo, J., Cho, W., Lee, W. S., Kim, C., et al. (2016). Diversity-oriented synthetic strategy for developing a chemical modulator of protein–protein interaction. Nat. Commun. 7, 13196–13205. doi: 10.1038/ncomms13196
Kim, J., Kim, H., and Park, S. B. (2014). Privileged structures: efficient chemical “navigators” toward unexplored biologically relevant chemical spaces. J. Am. Chem. Soc. 136, 14629–14638. doi: 10.1021/ja508343a
Kissau, L., Stahl, P., Mazitschek, R., Giannis, A., and Waldmann, H. (2003). Development of natural product-derived receptor tyrosine kinase inhibitors based on conservation of protein domain fold. J. Med. Chem. 46, 2917–2931. doi: 10.1021/jm0307943
Kitsiou, C., Hindes, J. J., I'Anson, P., Jackson, P., Wilson, T. C., Daly, E. K., et al. (2015). The synthesis of structurally diverse macrocycles by successive ring expansion. Angew. Chem. Int. Ed. 54, 15794–15798. doi: 10.1002/anie.201509153
Kopp, F., Stratton, C. F., Akella, L. B., and Tan, D. S. (2012). A diversity-oriented synthesis approach to macrocycles via oxidative ring expansion. Nat. Chem. Biol. 8, 358–365. doi: 10.1038/nchembio.911
Kuo, S.-Y., Castoreno, A. B., Aldrich, L. N., Lassen, K. G., Goel, G., Dantik, V., et al. (2015). Small-molecule enhancers of autophagy modulate cellular disease phenotypes suggested by human genetics. Proc. Natl. Acad. Sci. U.S.A.112, E4281–E4287. doi: 10.1073/pnas.1512289112
Kuruvilla, F. G., Shamji, A. F., Sternson, S. M., Hergenrother, P. J., and Schreiber, S. L. (2002). Dissecting glucose signaling with diversity-oriented synthesis and small-molecule microarrays. Nature 416, 653–657. doi: 10.1038/416653a
Lenci, E., Menchi, G., and Trabocchi, A. (2016). Carbohydrates in diversity-oriented synthesis: challenges and opportunities. Org. Biomol. Chem. 14, 808–825. doi: 10.1039/C5OB02253C
Li, L., Li, Z.-L., Wang, F.-L., Guo, Z., Cheng, Y.-F., Wang, N., et al. (2016). Radical aryl migration enables diversity-oriented synthesis of structurally diverse medium/macro- or bridged-rings. Nat. Commun. 7, 13852–13862. doi: 10.1038/ncomms13852
Lieberman, J. (2018). Tapping the RNA world for therapeutics. Nat. Struct. Mol. Biol. 25, 357–364. doi: 10.1038/s41594-018-0054-4
Ma, X, and Gang, D. R. (2004). The Lycopodium alkaloids. Nat. Prod. Rep. 21, 752–772. doi: 10.1039/b409720n
Madsen, C. M., and Clausen, M. H. (2011). Biologically active macrocyclic compounds – from natural products to diversity-oriented synthesis. Eur. J. Org. Chem. 3107–3115. doi: 10.1002/ejoc.201001715
Marcaurelle, L. A., Comer, E., Dandapani, S., Duvall, J. R., Gerard, B., Kesavan, S., et al. (2010). An aldol-based build/couple/pair strategy for the synthesis of medium- and large-sized rings: discovery of macrocyclic histone deacetylase inhibitors. J. Am. Chem. Soc. 132, 16962–16976. doi: 10.1021/ja105119r
Mason, J. S., Morize, I., Menard, P. R., Cheney, D. L., Hulme, C., and Labaudiniere, R. F. (1999). New 4-point pharmacophore method for molecular similarity and diversity applications: overview of the method and applications, including a novel approach to the design of combinatorial libraries containing privileged substructures. J. Med. Chem. 42, 3251–3264. doi: 10.1021/jm9806998
Newman, D. J. (2008). Natural products as leads to potential drugs: an old process or the new hope for drug discovery? J. Med. Chem. 51, 2589–2599. doi: 10.1021/jm0704090
Nicolaou, K. C., Pfefferkorn, J. A., Barluenga, S., Mitchell, H. J., Roecker, A. J., and Cao, G.-Q. (2000c). Natural product-like combinatorial libraries based on privileged structures. 3. The “libraries from libraries” principle for diversity enhancement of benzopyran libraries. J. Am. Chem. Soc. 122, 9968–9976. doi: 10.1021/ja0020355
Nicolaou, K. C., Pfefferkorn, J. A., Mitchell, H. J., Roecker, A. J., Barluenga, S., Cao, G.-Q., et al. (2000b). Natural product-like combinatorial libraries based on privileged structures. 2. Construction of a 10,000-membered benzopyran library by directed split-and-pool chemistry using nanokans and optical encoding. J. Am. Chem. Soc. 122, 9954–9967. doi: 10.1021/ja002034c
Nicolaou, K. C., Pfefferkorn, J. A., Roecker, A. J., Cao, G.-Q., Barluenga, S., and Mitchell, H. J. (2000a). Natural product-like combinatorial libraries based on privileged structures. 1. General principles and solid-phase synthesis of benzopyrans. J. Am. Chem. Soc. 122, 9939–9953. doi: 10.1021/ja002033k
Nie, F., Kunciw, D. L., Wilcke, D., Stokes, J. E., Galloway, W. R. J. D., Bartlett, S., et al. (2016). A multidimensional diversity-oriented synthesis strategy for structurally diverse and complex macrocycles. Angew. Chem. Int. Ed. 55, 11139–11143. doi: 10.1002/anie.201605460
Nielsen, T. E., and Schreiber, S. L. (2008). Towards the optimal screening collection: a synthesis strategy. Angew. Chem. Int. Ed. 47, 48–56. doi: 10.1002/anie.200703073
Oh, S., and Park, S. B. (2011). A design strategy for drug-like polyheterocycles with privileged substructures for discovery of specific small-molecule modulators. Chem. Commun. 47, 12754–12761. doi: 10.1039/c1cc14042f
Plouffe, D. M., Wree, M., Du, A. Y., Meister, S., Li, F., Patra, K., et al. (2016). High-throughput assay and discovery of small molecules that interrupt malaria transmission. Cell Host Microbe 19, 114–126. doi: 10.1016/j.chom.2015.12.001
Rafferty, R. J., Hicklin, R. W., Maloof, K. A., and Hergenrother, P. J. (2014). Synthesis of complex and diverse compounds through ring distortion of abietic acid. Angew. Chem. Int. Ed. 53, 220–224. doi: 10.1002/anie.201308743
Salituro, G. M., Pettibone, D. J., Clineschmidt, B. V., Williamson, J. M., and Zink, D. L. (1993). Potent, non-peptidic oxytocin receptor antagonists from a natural source. Bioorg. Med. Chem. Lett. 3, 337–340. doi: 10.1016/S0960-894X(01)80905-7
Samanen, J. (2013). “How do SMDs differ from biomolecular drugs?” in: Introduction to Biological and Small Molecule Drug Research and Development: Theory and Case Studies, eds R. Jefferis, S. Roberts, and R. Ganellin (Waltham, MA: Elsevier), 169–176.
Schreiber, S. L. (2000). Target-oriented and diversity-oriented organic synthesis in drug discovery. Science 287, 1964–1969. doi: 10.1126/science.287.5460.1964
Schreiber, S. L., Kotz, J. D., Li, M., Aubé, J., Austin, C. P., Reed, J. C., et al. (2015). Advancing biological understanding and therapeutics discovery with small-molecule probes. Cell 161, 1252–1265. doi: 10.1016/j.cell.2015.05.023
Scott, D. E., Bayly, A. R., Abell, C., and Skidmore, J. (2016). Small molecules, big targets: drug discovery faces the protein-protein interaction challenge. Nat. Rev. Drug Discov. 15, 533–550. doi: 10.1038/nrd.2016.29
Shimokawa, J. (2014). Divergent strategy in natural product total synthesis. Tetrahedron Lett. 55, 6156–6162. doi: 10.1016/j.tetlet.2014.09.078
Spring, D. R. (2003). Diversity-oriented synthesis; a challenge for synthetic chemists. Org. Biomol. Chem. 1, 3867–3870. doi: 10.1039/b310752n
Stephens, T. C., Lawer, A., French, T., and Unsworth, W. P. (2018). Iterative assembly of macrocyclic lactones using successive ring expansion reactions. Chem. Eur. J. 24, 13947-13953 doi: 10.1002/chem.201803064
Stephens, T. C., Lodi, M., Steer, A. M., Lin, Y., Gill, M. T., and Unsworth, W. P. (2017). Synthesis of cyclic peptide mimetics by the successive ring expansion of lactams. Chem. Eur. J. 23, 13314–13318. doi: 10.1002/chem.201703316
Tan, D. S. (2005). Diversity-oriented synthesis: exploring the intersections between chemistry and biology. Nat. Chem. Biol. 1, 74–84. doi: 10.1038/nchembio0705-74
Villar, E. A., Beglov, D., Chennamadhavuni, S., Porco, J. A. Jr., Kozakov, D., Vajda, S., et al. (2014). How proteins bind macrocycles. Nat. Chem. Biol. 10, 723–731. doi: 10.1038/nchembio.1584
Warner, K. D., Hajdin, C. E., and Weeks, K. M. (2018). Principles for targeting RNA with drug-like small molecules. Nat. Rev. Drug Discov. 17, 547–558. doi: 10.1038/nrd.2018.93
Wellington, S., Nag, P. P., Michalska, K., Johnston, S. E., Jedrzejczak, R. P., Kaushik, V. K., et al. (2017). A small-molecule allosteric inhibitor of Mycobacterium tuberculosis tryptophan synthase. Nat. Chem. Biol. 13, 943–950. doi: 10.1038/nchembio.2420
Wipf, P. (2012). Diversity-oriented synthesis of peptidomimetics: how and why. Diver. Orient. Synthes. 1, 6–10. doi: 10.2478/dos-2012-0002
Yu, X., and Sun, D. (2013). Macrocyclic drugs and synthetic methodologies toward macrocycles. Molecules 18, 6230–6268. doi: 10.3390/molecules18066230
Zhang, J., Wu, J., Hong, B., Ai, W., Wang, X., Li, H., et al. (2014). Diversity-oriented synthesis of Lycopodium alkaloids inspired by the hidden functional group pairing pattern. Nat. Commun. 5, 4614–4622. doi: 10.1038/ncomms5614
Keywords: diversity-oriented synthesis, build/couple/pair, ring-distortion, natural product, macrocycle, privileged structure
Citation: Yi S, Varun BV, Choi Y and Park SB (2018) A Brief Overview of Two Major Strategies in Diversity-Oriented Synthesis: Build/Couple/Pair and Ring-Distortion. Front. Chem. 6:507. doi: 10.3389/fchem.2018.00507
Received: 08 August 2018; Accepted: 03 October 2018;
Published: 22 October 2018.
Edited by:
Gwilherm Evano, Free University of Brussels, BelgiumReviewed by:
Raphaël Frédérick, Université Catholique de Louvain, BelgiumSteven Ballet, Vrije Universiteit Brussel, Belgium
Laurent Commeiras, Aix-Marseille Université, France
Copyright © 2018 Yi, Varun, Choi and Park. This is an open-access article distributed under the terms of the Creative Commons Attribution License (CC BY). The use, distribution or reproduction in other forums is permitted, provided the original author(s) and the copyright owner(s) are credited and that the original publication in this journal is cited, in accordance with accepted academic practice. No use, distribution or reproduction is permitted which does not comply with these terms.
*Correspondence: Seung Bum Park, sbpark@snu.ac.kr
†These authors have contributed equally to this work