- 1Key Laboratory of Key Technical Factors in Zhejiang Seafood Health Hazards, Institute of Innovation & Application, Zhejiang Ocean University, Zhoushan, China
- 2Department of Environmental Engineering, Zhejiang Ocean University, Zhoushan, China
- 3State Environmental Protection Engineering Center for Pollution Treatment and Control in Textile Industry, College of Environmental Science and Engineering, Donghua University, Shanghai, China
Semiconductor-based photocatalysis is of great potential for tackling the environmental pollution. Herein, a novel hierarchical heterostructure of Bi2O2CO3 micro-flowers in-situ decorated with Ag3VO4 nanoparticles was developed by a facile method. Various characterization techniques have been employed to study the physical and chemical property of the novel catalyst. The novel catalyst was utilized for the photocatalytic removal of industrial dyes (rhodamine B, methyl orange) and tetracycline antibiotic under visible-light irradiation. The results indicated that Ag3VO4/Bi2O2CO3 heterojunctions showed a remarkably enhanced activity, significantly higher than those of bare Ag3VO4, Bi2O2CO3, and the physical mixture of Ag3VO4 and Bi2O2CO3 samples. This could be ascribed to an enhanced visible-light harvesting capacity and effective separation of charge carriers by virtue of the construction of hierarchical Ag3VO4/Bi2O2CO3 heterojunction. Moreover, Ag3VO4/Bi2O2CO3 also possesses an excellent cycling stability. The outstanding performance of Ag3VO4/Bi2O2CO3 in removal of toxic pollutants indicates the potential of Ag3VO4/Bi2O2CO3 in real environmental remediation.
Highlights
• Novel architectures of Ag3VO4 nanoparticles modified Bi2O2CO3 micro-flowers were constructed.
• Novel Ag3VO4/Bi2O2CO3 exhibited excellent photocatalytic activity and stability.
• Ag3VO4/Bi2O2CO3 heterojunctions significantly promote the charge separation.
Introduction
Semiconductor photocatalysis has been regarded as one of the most promising nanotechnologies for the treatment of environmental pollution (Bora and Mewada, 2017; Cates, 2017; Wang W. et al., 2017; Zhang and Ma, 2017; Zhu and Wang, 2017). A significant research topic of photocatalysis is the exploration of highly active photocatalysts (Li et al., 2014, 2017d, 2018b; Adhikari et al., 2015, 2016, 2017; Martin et al., 2015; Zhang et al., 2016, 2017; Mousavi et al., 2018; Yu et al., 2018).
The emergent Bi2O2CO3 has attracted much interest for its good photocatalytic performance in the removal of toxic pollutants (Ni et al., 2016; Yu et al., 2018). However, the photocatalytic properties are still far from satisfactory owing to low solar utilization and fast recombination of electron-hole pairs. To improve the visible-light photocatalytic activity of Bi2O2CO3, various strategies have been developed, such as design of microstructure (Zhao et al., 2011), deposition of metals (Yu et al., 2016), formation of heterojunction (Chen et al., 2016, 2017; Huang et al., 2016; Feng et al., 2017; Hu et al., 2017), and doping with ions (Dong et al., 2014; Xiong et al., 2015). The rational construction of heterojunctions can effectively ameliorate the visible-light absorption ability and significantly suppress the electron-hole recombination (Han et al., 2017; Li et al., 2017b,c,e; Zhong et al., 2018). The further development of novel Bi2O2CO3-based catalysts is still required to offer more potential candidates for practical application and to figure out the reasons for the synergetic effect between the components.
Ag3VO4, an active VLD photocatalyst, has drawn much attention in virtue of its unique band structures. Ag3VO4 has been coupled with other semiconductors (e.g., Bi2WO6 Li et al., 2017a; Zhang and Ma, 2017, BiOI Wang et al., 2015, BiOCl Wang et al., 2016, C3N4 Wang et al., 2014, BiVO4 Yan et al., 2016a, WO3 Yan et al., 2016b) to fabricate high-performance photocatalysts. To date, researches on Ag3VO4 nanoparticles decorated Bi2O2CO3 micro-flowers for the visible-light photo-degradation of toxic contaminants have not been reported.
Herein, we report Ag3VO4 nanoparticles evenly deposited on the surface of Bi2O2CO3 micro-flowers by a simple precipitation method. Ag3VO4 nanoparticles can optimize the visible-light response and facilitate the separation of charge carriers, endowing the novel heterojunction with excellent visible-light photocatalytic performance. The plausible visible-light photocatalysis mechanism of Ag3VO4/Bi2O2CO3 is also proposed.
Experiment
Chemicals
Bismuth citrate (BiO7C6H5), sodium carbonate (Na2CO3), ethanol (CH3CH2OH), silver nitrate (AgNO3), sodium vanadate (Na3VO4), rhodamine B (RhB), ammonium oxalate (AO), AgNO3, tetracycline hydrochloride (TC), p-benzoquinone (BQ), methyl orange (MO), and iso-propanol (IPA) were bought from Shanghai Chemical Reagent factory (China). All the reagents were analytic grade and used without further treatment.
Synthesis of Catalysts
Bi2O2CO3 was synthesized via a hydrothermal procedure. Briefly, 2 mmol of sodium carbonate (Na2CO3) and 2 mmol of bismuth citrate (BiO7C6H5) were sequentially dissolved in the solution containing 30 mL of deionized water and 5 mL of absolute ethanol with the assistance of ultra-sonication. The resulting solution was sealed in a 50 mL autoclave and heated at 160°C for 25 h. After the reactor system was cooled down, the precipitants were washed thoroughly with de-ionized water and dried at 80°C overnight.
Ag3VO4/Bi2O2CO3 heterojunctions were constructed by a simple precipitation method. Briefly, an appropriate amount of Bi2O2CO3 was ultrasonically suspended in 50 mL of H2O. Then, 3 mmol AgNO3 was dissolved in the above solution under magnetical stirring. After that, Na3VO4 (20 mL, 0.05 mol L−1) solution was slowly dropped into the mixture with vigorous stirring for 5 h. Lastly, the obtained solids were washed with deionized water four times and dried at 80°C for 10 h to get the Ag3VO4/Bi2O2CO3 heterojunctions. The heterojunctions with different Bi2O2CO3/Ag3VO4 weight ratios of 0.05/1, 0.10/1, 0.30/1, and 0.50/1 are labeled as AVO/BOC-5, AVO/BOC-10, AVO/BOC-30, and AVO/BOC-50, respectively. Ag3VO4 was prepared in the absence of Bi2O2CO3.
Characterization of Catalysts
The scanning electron microscopy (SEM, Hitachi S-4800) and transmission electron microscopy (TEM, JEM-2100 JEOL) were applied to characterize the morphology of the samples. Bruker Quantax 400 energy-dispersive X-ray spectroscopy (EDS) was used to identify the chemical composition. Powder X-ray diffractometer (XRD, MSAL XD2) was used to get the XRD patterns of the samples. UV–Vis diffuse reflectance spectra (DRS) were obtained on a spectrophotometer (Shimadzu UV−2600). Photoluminescence (PL) spectra of the samples were recorded on a Hitachi RF-6000 spectrophotometer.
Photocatalytic Tests
Pollutant [rhodamine B (RhB), methyl orange (MO), and tetracycline hydrochloride (TC)] removal performances were tested under visible-light irradiation, 300 W xenon lamp with filter (λ > 400 nm). The photocatalytic reaction was conducted in a glass reactor containing 80 mL of RhB (5 mg L−1), MO (5 mg L−1), or TC (20 mg L−1) solution, and 40 mg of catalyst. The solution was first ultrasonically dispersed for 1 min and then magnetically stirred in the dark for 1 h. 1.5 mL of solution was taken at specified time, and centrifuged to remove the solids. The pollutant concentrations were determined using a Shimadzu UV-2600 spectrophotometer. Total organic carbon (TOC) value of the pollutant solutions during reaction was detected on a Shimadzu TOC analyzer.
Results and Discussion
Characterization
Figure 1 displays the XRD patterns of the as-prepared pure Ag3VO4, Bi2O2CO3, and their heterojunctions (AVO/BOC-5, AVO/BOC-10, AVO/BOC-30, and AVO/BOC-50). The diffraction peaks of Ag3VO4 and Bi2O2CO3 prepared match well with monoclinic phase of Ag3VO4 (JCPDS 43-0542) and tetragonal phase of Bi2O2CO3 (JCPDS 41-1488), respectively.
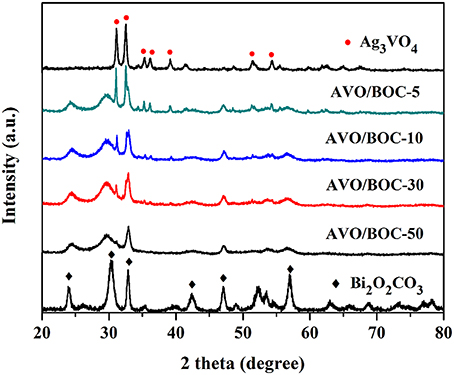
Figure 1. XRD patterns of Ag3VO4/Bi2O2CO3 heterojunctions (AVO/BOC-5, AVO/BOC-10, AVO/BOC-30, and AVO/BOC-50), pure Bi2O2CO3, and Ag3VO4.
When a small amount of Ag3VO4 was introduced, no diffraction peaks of Ag3VO4 can be observed in the XRD pattern of AVO/BOC-50. As the Ag3VO4 content increases, AVO/BOC-30, AVO/BOC-10, and AVO/BOC-5 show the diffraction peaks of both Bi2O2CO3 and Ag3VO4, indicating the successful fabrication of Ag3VO4/Bi2O2CO3 heterojunctions.
The microstructures of Bi2O2CO3 and Ag3VO4/Bi2O2CO3 heterojunctions were investigated by using SEM. The SEM images in Figures 2A,B show that the obtained Bi2O2CO3 exhibits flower-like microspheres constructed by countless nano-plates (Zhao et al., 2011). The SEM images in Figures 2C,D show the representative AVO/BOC-10 also possesses sphere-like morphology as that for pure Bi2O2CO3. Of note, numerous Ag3VO4 nanoparticles were deposited on the surfaces of AVO/BOC-10.
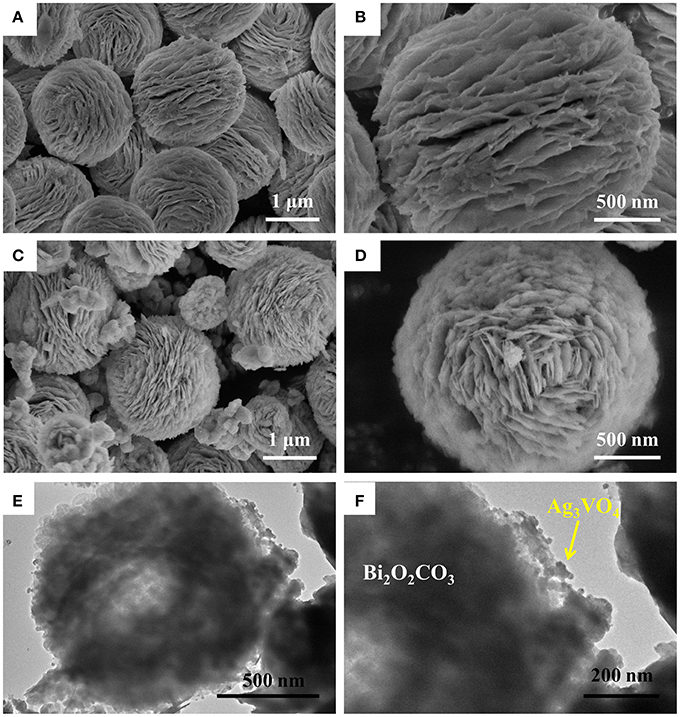
Figure 2. SEM images of pristine Bi2O2CO3 (A,B) and AVO/BOC-30 (C,D); TEM images of AVO/BOC-10 (E,F).
The more detailed microstructures of AVO/BOC-10 were studied by TEM. As shown in Figures 2E,F, AVO/BOC-10 consists of Bi2O2CO3 micro-flower (diameter: ~1.1 μm) and Ag3VO4 nanoparticles (size: ~26 nm), and they were tightly combined with each other to generate closely hybrid hetero-structure, in favor of transfer and separation of charge carriers (Huang et al., 2015; Zhang et al., 2016; Li et al., 2017a).
The corresponding EDS spectra of AVO/BOC-10 revealed that only signals for Ag, V, Bi, C, and O elements were detected, indicating the high purity of the sample (Figure 3). The above results verified that the facile precipitation method could successfully fabricate Ag3VO4/Bi2O2CO3 heterojunctions with intimate contact between two constituents.
The sunlight absorption capability and band structures of a photocatalyst usually exert a significant effect on its photocatalytic performance. Thus, the UV–Vis DRS spectra of bare Bi2O2CO3, Ag3VO4, and Ag3VO4/Bi2O2CO3 heterojunctions are measured and illustrated in Figure 4. Bi2O2CO3 exhibited a strong absorption in the UV region with the absorption edge at 385 nm, in accordance with the previous reports (Zhao et al., 2011; Hu et al., 2017). Ag3VO4 performed a 575 nm absorption edge in the VL region, consistent with the reported values (Wang et al., 2015; Yan et al., 2016b; Li et al., 2017a). Intriguingly, the combination of Ag3VO4 and Bi2O2CO3 substantially ameliorated the VL absorption properties of the heterojunctions, which is beneficial for the effective utilization of solar energy.
The bandgap width (Eg) was estimated according to the equation: Eg = 1240/λg (eV), and the Eg value of Ag3VO4 and Bi2O2CO3 are about 2.15 and 3.23 eV. The band positions (EVB and ECB) of Ag3VO4 and Bi2O2CO3 can be calculated using the following formula:
Where X [ca. 6.36 eV for Ag3VO4 (Li et al., 2017a), and ca. 6.54 eV for Bi2O2CO3 Liang et al., 2014] is the electronegativity of the semiconductor. Ee value equals to ~4.5 eV. On the basis of above data, the EVB and ECB of Ag3VO4 were determined as 0.01 and 2.14 eV, while those of Bi2O2CO3 were 0.2 and 3.53 eV.
Photocatalytic Property
The VLD photocatalytic activity of Ag3VO4/Bi2O2CO3 heterojunctions was studied through the degradation of RhB (Figure 5), MO (Figure S1), and TC (Figure 6). Figure 5A displays the concentration change of RhB dye solution under visible light with the as-prepared catalysts. The blank test conducted without the presence of catalyst showed that RhB was not degraded after 60 min of irradiation. The photocatalytic activity of pristine Bi2O2CO3 is much lower than other samples and the RhB degradation efficiency is 31.4%, mainly due to its large bandgap (Yu et al., 2016). Only 49.8% of RhB was removed by pure Ag3VO4 due to the high recombination rate of charge carriers (Yan et al., 2016b). Inspiringly, when Bi2O2CO3 was decorated with Ag3VO4, the catalytic activity of these heterojunctions was substantially improved. After 60 min of irradiation, the RhB degradation efficiencies by using AVO/BOC-5, AVO/BOC-10, AVO/BOC-30 and AVO/BOC-50 were 85.8, 98.4, 76.9, and 71.1%, respectively, much higher than that by using the pristine Bi2O2CO3, Ag3VO4, or the mechanical mixture (91 wt% Ag3VO4 + 9 wt% Bi2O2CO3). The activity of Ag3VO4/Bi2O2CO3 increases gradually and then declines regularly, while AVO/BOC-10 has the highest photocatalytic activity, indicating the vital role of Ag3VO4 in enhancing the activity.
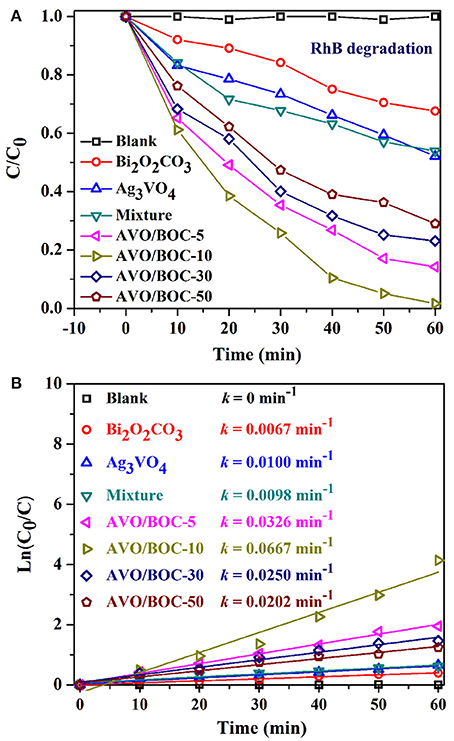
Figure 5. (A) Photocatalytic degradation efficiency of RhB over different catalysts under visible light. (B) Rate constants of RhB degradation during reaction over different catalysts.
The degradation rate constants (k) of RhB were presented in Figure 5B. The photocatalytic activity of AVO/BOC-10 achieved the maximum value of k = 0.0667 min−1, it was about 8.9, 5.7, and 5.8-folds higher than pure Bi2O2CO3 (0.0067 min−1), Ag3VO4 (0.0100 min−1), and the mechanical mixture (0.0098 min−1).
The degradation of antibiotic TC or industrial dye MO was also performed to further test the VL photocatalysis of AVO/BOC-10 (Figure 6 and Figure S1). Apparently, AVO/BOC-10 also showed high activity in the degradation of TC and MO (Figure S1). The TC or MO degradation efficiency with AVO/BOC-10 as the catalyst was 83.7 or 94.2% after 180 min of reaction. These results demonstrate that AVO/BOC-10 exhibits extraordinary photocatalytic activity in the removal of toxic pollutants.
To assess the mineralization capability of Ag3VO4/Bi2O2CO3, the TOC data during RhB (50 mg L−1) degradation over AVO/BOC-10 (200 mg) was recorded and analyzed (Figure 7A). It is found that the TOC removal efficiency of RhB with AVO/BOC-10 is 76.4% after 6 h of reaction, suggesting that AVO/BOC-10 possesses strong mineralization ability during the photocatalytic reaction.
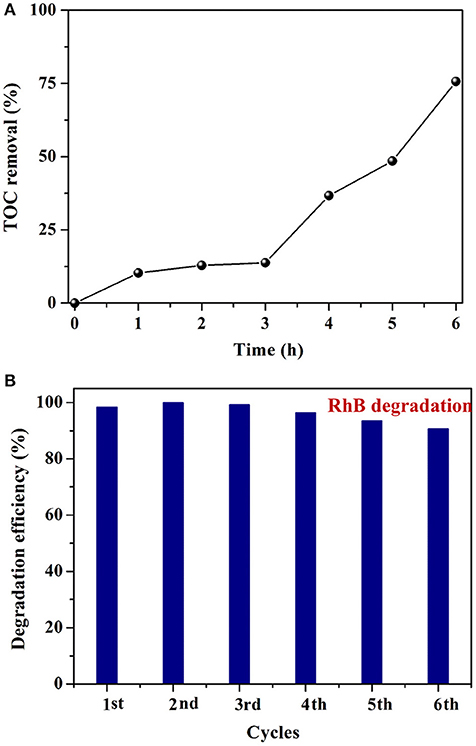
Figure 7. (A) TOC removal rate during RhB degradation with AVO/BOC-10; (B) The cycling performance of AVO/BOC-10.
For evaluating the stability of Ag3VO4/Bi2O2CO3, six successive cycles of RhB degradation with AVO/BOC-10 as a catalyst were carried out. Inspiringly, no apparent loss of activity of the catalyst in six times of reuse was observed, and the RhB removal efficiency retained 92.1% in the sixth run (Figure 7B). In addition, the catalyst before and after six runs was characterized by XRD technique (Figure S2), and no obvious changes in the crystalline phases was detected, verifying the good stability of AVO/BOC-10. Moreover, the cycling degradation involving TC antibiotic further confirms the good stability of AVO/BOC-10 (Figure S3). The photocatalytic tests demonstrate that AVO/BOC-10 endowed with high activity and stability is a kind of promising VLD photocatalysts, exhibiting great potential for wastewater treatment.
Origin of the Improved Performance
The photocatalytic activity depends strongly on the separation efficiency of photo-induced charge carriers (Hu et al., 2017; Li et al., 2017a), thus, photoluminescence (PL) spectrum of the samples were acquired to illustrate the electron-hole separation (Figure 8). Apparently, the PL intensity of AVO/BOC-10 is much weaker than that of pristine Bi2O2CO3. Since a weaker PL intensity signifies higher separation rate of charge carriers, AVO/BOC-10 should possess a higher separation efficiency compared with Bi2O2CO3. That is to say, the photo-induced electron-hole pairs were efficiently separated in AVO/BOC-10 system due to the interfacial charge transfer, resulting in the elevated photocatalytic activity.
For mechanistic study, various additives were employed to research the main active species in the photocatalytic reaction process (Figure 9) (Zhang and Ma, 2017; Li et al., 2018). When 1 mmol of BQ (benzoquinone, O scavenger) or AO (ammonium oxalate, h+ scavenger) was introduced, the activity of AVO/BOC-10 was substantially quenched, and the RhB degradation efficiency declined from 98.4 to 29.7% or 20.2%, revealing that O and h+ should play vital roles in the photocatalysis. On the contrary, no obvious decrease in the activity was observed with adding 1 mmol of IPA (isopropyl alcohol, ∙OH scavenger), signifying that ∙OH plays a minor role.
Based on this systematic investigation, a possible visible-light photocatalytic mechanism for pollutant degradation over Ag3VO4/Bi2O2CO3 is proposed (Figure 10). Apparently, the position of CB and VB between Ag3VO4 and Bi2O2CO3 are beneficial to achieve effective separation of photo-generated charge carriers (Wang F. F. et al., 2017; Ye et al., 2018). Photo-generated electrons and holes are produced on Ag3VO4 under visible-light illumination. Since the CB of Bi2O2CO3 is lower than that of Ag3VO4, the electrons can be injected readily from Ag3VO4 to Bi2O2CO3. The electrons on the CB of Bi2O2CO3 can react with O2 to form active O, degrading toxic pollutants such as RhB/MO/TC. Simultaneously, the holes with strong oxide capability in the VB of Ag3VO4 are available to take part in the decomposition of pollutants. In such a way, the electrons and holes can be effectively utilized, as evidenced by the result of PL spectra of Bi2O2CO3 and Ag3VO4/Bi2O2CO3 (Figure 8). In summary, the combination of Ag3VO4 and Bi2O2CO3 enhances the charge separation, leading to the high activity.
Conclusions
In this study, innovative Ag3VO4/Bi2O2CO3 heterojunction photocatalysts were synthesized via a simple procedure. The Ag3VO4/Bi2O2CO3 heterojunction (AVO/BOC-10) displayed the optimal photocatalytic properties toward the degradation of toxic pollutants (RhB dye, MO dye, and TC antibiotic), much higher than pristine Bi2O2CO3 and Ag3VO4. The close contact and the match of bandgap structure between both constituents boost the separation of electron-hole pairs, mainly accounting for the activity enhancement. The holes and O were determined as the primary active species responsible for the efficient removal and mineralization of the toxic pollutants. Therefore, Ag3VO4/Bi2O2CO3 holds huge potential for real wastewater treatment.
Author Contributions
SL designed and performed the experiments, and data analysis. SH, WJ, YuL, YaL, YZ, JL, and LM assisted with some of the tests. SL wrote the main content of the paper. All authors have read and approved the paper to be submitted.
Conflict of Interest Statement
The authors declare that the research was conducted in the absence of any commercial or financial relationships that could be construed as a potential conflict of interest.
Acknowledgments
This work has been financially supported by the National Natural Science Foundation of China (51708504 and 31501573), the Public Projects of Zhejiang Province (2017C32079 and LGN18E080003), the Science and Technology Project of Zhoushan (2017C41006, 2016C41012, 2015C21014, and 2015C21013), the National Key Research Development Program of China (2016YFC0400501), and the Research Startup Foundation of Zhejiang Ocean University (12215090117).
Supplementary Material
The Supplementary Material for this article can be found online at: https://www.frontiersin.org/articles/10.3389/fchem.2018.00255/full#supplementary-material
References
Adhikari, S. P., Dean, H., Hood, Z. D., Peng, R., More, K. L., Ivanov, I., et al. (2015). Visible-light-driven Bi2O3/WO3 composites with enhanced photocatalytic activity. RSC Adv. 5, 91094–91102. doi: 10.1039/C5RA13579F
Adhikari, S. P., Hood, Z. D., More, K. L., Chen, V. W., and Lachgar, A. (2016). A visible-light-active heterojunction with enhanced photocatalytic hydrogen generation. ChemSusChem 9, 1869–1879. doi: 10.1002/cssc.201600424
Adhikari, S. P., Hood, Z. D., Wang, H., Peng, R., Krall, A., Li, H., et al. (2017). Enhanced visible light photocatalytic water reduction from a g-C3N4/SrTa2O6 heterojunction. Appl. Catal. B 217, 448–458. doi: 10.1016/j.apcatb.2017.05.092
Bora, L. V., and Mewada, R. K. (2017). Visible/solar light active photocatalysts for organic effluent treatment: fundamentals, mechanisms and parametric review. Renew. Sustain. Energy Rev. 76, 1391–1421. doi: 10.1016/j.rser.2017.01.130
Cates, E. L. (2017). Photocatalytic water treatment: so where are we going with this? Environ. Sci. Technol. 51, 757–758. doi: 10.1021/acs.est.6b06035
Chen, J. L., Li, S. J., Hu, S. W., and Jiang, W. (2017). Enhanced visible-light photocatalytic activity of Ag/AgI coupled Bi2O2CO3 microspheres. Mater. Lett. 191, 123–127. doi: 10.1016/j.matlet.2016.12.096
Chen, J., Mei, W. G., Huang, Q. J., Chen, N. N., Lu, C. L., Zhu, H. J., et al. (2016). Highly efficient three-dimensional flower-like AgI/Bi2O2CO3 heterojunction with enhanced photocatalytic performance. J. Alloys Compd. 688, 225–234. doi: 10.1016/j.jallcom.2016.07.196
Dong, F., Xiong, T., Wang, R., Sun, Y. J., and Jiang, Y. K. (2014). Growth mechanism and photocatalytic activity of self-organized N-doped (BiO)2CO3 hierarchical nanosheet microspheres from bismuth citrate and urea. Dalton Trans. 43, 6631–6642. doi: 10.1039/c3dt53383b
Feng, X., Zhang, W., Sun, Y., Huang, H., and Dong, F. (2017). Fe(III) cluster-grafted (BiO)2CO3 superstructures: in situ DRIFTS investigation on IFCT-enhanced visible light photocatalytic NO oxidation. Environ. Sci. Nano 4, 604–612. doi: 10.1039/c6en00637j
Han, W., Li, Z., Li, Y., Fan, X., Zhang, F., Zhang, G., et al. (2017). The promoting role of different carbon allotropes cocatalysts for semiconductors in photocatalytic energy generation and pollutants degradation. Front. Chem. 5: 84. doi: 10.3389/fchem.2017.00084
Hu, J., Chen, D., Li, N., Xu, Q., Li, H., He, J., et al. (2017). In situ fabrication of Bi2O2CO3/MoS2 on carbon nanofibers for efficient photocatalytic removal of NO under visible-light irradiation. Appl. Catal. B 217, 224–231. doi: 10.1016/j.apcatb.2017.05.088
Huang, H. W., He, Y., Du, X., Chu, P. K., and Zhang, Y. H. (2015). A general and facile approach to heterostructured core/Shell BiVO4/BiOI p-n junction: room-temperature in situ assembly and highly boosted visible-light photocatalysis. ACS Sust. Chem. Eng. 3, 3262–3273. doi: 10.1021/acssuschemeng.5b01038
Huang, Y. C., Fan, W. J., Long, B., Li, H. B., Zhao, F. Y., Liu, Z. L., et al. (2016). Visible light Bi2S3/Bi2O3/Bi2O2CO3 photocatalyst for effective degradation of organic pollutions. Appl. Catal. B 185, 68–76. doi: 10.1016/j.apcatb.2015.11.043
Li, S., Hu, S., Jiang, W., Liu, Y., Liu, Y., Zhou, Y., et al. (2018). Ag2WO4 nanorods decorated with AgI nanoparticles: novel and efficient visible-light-driven photocatalysts for the degradation of water pollutants. Beilstein J. Nanotechnol. 9, 1308–1316. doi: 10.3762/bjnano.9.123
Li, S. J., Hu, S. W., Jiang, W., Liu, Y., Liu, J. S., and Wang, Z. H. (2017a). Facile synthesis of flower-like Ag3VO4/Bi2WO6 heterojunction with enhanced visible-light photocatalytic activity. J. Colloid Interface Sci. 501, 156–163. doi: 10.1016/j.jcis.2017.04.057
Li, S. J., Hu, S. W., Jiang, W., Liu, Y., Liu, J. S., and Wang, Z. H. (2017b). Synthesis of n-type TaON microspheres decorated by p-type Ag2O with enhanced visible light photocatalytic activity. Mol. Catal. 435, 135–143. doi: 10.1016/j.mcat.2017.03.027
Li, S. J., Hu, S. W., Jiang, W., Liu, Y. P., Zhou, Y. T., Liu, Y., et al. (2018b). Hierarchical architectures of bismuth molybdate nanosheets onto nickel titanate nanofibers: facile synthesis and efficient photocatalytic removal of tetracycline hydrochloride. J. Colloid Interface Sci. 521, 42–49. doi: 10.1016/j.jcis.2018.03.033
Li, S. J., Hu, S. W., Xu, K. B., Jiang, W., Liu, J. S., and Wang, Z. H. (2017c). A novel heterostructure of BiOI nanosheets anchored onto MWCNTs with excellent visible-light photocatalytic activity. Nanomaterials 7, 22–34. doi: 10.3390/nano7010022
Li, S. J., Hu, S. W., Zhang, J. L., Jiang, W., and Liu, J. S. (2017d). Facile synthesis of Fe2O3 nanoparticles anchored on Bi2MoO6 microflowers with improved visible light photocatalytic activity. J. Colloid Interface Sci. 497, 93–101. doi: 10.1016/j.jcis.2017.02.069
Li, S. J., Shen, X. F., Liu, J. S., and Zhang, L. S. (2017e). Synthesis of Ta3N5/Bi2MoO6 core-shell fiber-shaped heterojunctions as efficient and easily recyclable photocatalysts. Environ. Sci. Nano 4, 1155–1167. doi: 10.1039/C6EN00706F
Li, S. J., Zhang, L. S., Wang, H. L., Chen, Z. G., Hu, J. Q., Xu, K. B., et al. (2014). Ta3N5-Pt nonwoven cloth with hierarchical nanopores as efficient and easily recyclable macroscale photocatalysts. Sci. Rep. 4:3978. doi: 10.1038/srep03978
Liang, N., Wang, M., Jin, L., Huang, S. S., Chen, W. L., Xu, M., et al. (2014). Highly efficient Ag2O/Bi2O2CO3 p-n heterojunction photocatalysts with improved visible-light responsive activity. ACS Appl. Mater. Interfaces 6, 11698–11705. doi: 10.1021/am502481z
Martin, D. J., Liu, G., Moniz, S. J. A., Bi, Y., Beale, A. M., Ye, J., et al. (2015). Efficient visible driven photocatalyst, silver phosphate: performance, understanding and perspective. Chem. Soci. Rev. 44, 7808–7828. doi: 10.1039/c5cs00380f
Mousavi, M., Habibi-Yangjeh, A., and Pouran, S. R. (2018). Review on magnetically separable graphitic carbon nitride-based nanocomposites as promising visible-light-driven photocatalysts. J. Mater. Sci. Mater. Electron. 29, 1719–1747. doi: 10.1007/s10854-017-8166-x
Ni, Z. L., Sun, Y. J., Zhang, Y. X., and Dong, F. (2016). Fabrication, modification and application of (BiO)2CO3-based photocatalysts: a review. Appl. Surf. Sci. 365, 314–335. doi: 10.1016/j.apsusc.2015.12.231
Wang, F. F., Li, Q., and Xu, D. S. (2017). Recent progress in semiconductor-based nanocomposite photocatalysts for solar-to-chemical energy conversion. Adv. Energy Mater. 7:1700529. doi: 10.1002/aenm.201700529
Wang, P. F., Tang, H., Ao, Y. H., Wang, C., Hou, J., Qian, J., et al. (2016). In-situ growth of Ag3VO4 nanoparticles onto BiOCl nanosheet to form a heterojunction photocatalyst with enhanced performance under visible light irradiation. J. Alloys Compd. 688, 1–7. doi: 10.1016/j.jallcom.2016.07.180
Wang, S. M., Guan, Y., Wang, L. P., Zhao, W., He, H., Xiao, J., et al. (2015). Fabrication of a novel bifunctional material of BiOI/Ag3VO4 with high adsorption-photocatalysis for efficient treatment of dye wastewater. Appl. Catal. B 168–169, 448-457. doi: 10.1016/j.apcatb.2014.12.047
Wang, S. M., Li, D. L., Sun, C., Yang, S. G., Guan, Y., and He, H. (2014). Synthesis and characterization of g-C3N4/Ag3VO4 composites with significantly enhanced visible-light photocatalytic activity for triphenylmethane dye degradation. Appl. Catal. B 144, 885–892. doi: 10.1016/j.apcatb.2013.08.008
Wang, W., Li, G., Xia, D., An, T., Zhao, H., and Wong, P. K. (2017). Photocatalytic nanomaterials for solar-driven bacterial inactivation: recent progress and challenges. Environ. Sci. Nano 4, 782–799. doi: 10.1039/C7EN00063D
Xiong, T., Huang, H. W., Sun, Y. J., and Dong, F. (2015). In situ synthesis of a C-doped (BiO)2CO3 hierarchical self-assembly effectively promoting visible light photocatalysis. J. Mater. Chem. A 3, 6118–6127. doi: 10.1039/c5ta00103j
Yan, M., Wu, Y. L., Yan, Y., Yan, X., Zhu, F. F., Hua, Y. Q., et al. (2016a). Synthesis and characterization of novel BiVO4/Ag3VO4 heterojunction with enhanced visible-light-driven photocatalytic degradation of dyes. ACS Sustainable Chem. Eng. 4, 757–766. doi: 10.1021/acssuschemeng.5b00690
Yan, M., Wu, Y. L., Zhu, F. F., Hua, Y. Q., and Shi, W. D. (2016b). The fabrication of a novel Ag3VO4/WO3 heterojunction with enhanced visible light efficiency in the photocatalytic degradation of TC. Phys. Chem. Chem. Phys. 18, 3308–3315. doi: 10.1039/c5cp05599g
Ye, R., Zhao, J., Wickemeyer, B. B., Toste, F. D., and Somorjai, G. A. (2018). Foundations and strategies of the construction of hybrid catalysts for optimized performances. Nat. Catal. 1, 318–325. doi: 10.1038/s41929-018-0052-2
Yu, C. L., Zhou, W. Q., Zhu, L. H., Li, G., Yang, K., and Jin, R. C. (2016). Integrating plasmonic Au nanorods with dendritic like α-Bi2O3/Bi2O2CO3 heterostructures for superior visible-light-driven photocatalysis. Appl. Catal. B 184, 1–11. doi: 10.1016/j.apcatb.2015.11.026
Yu, S., Zhang, Y., Dong, F., Li, M., Zhang, T., and Huang, H. (2018). Readily achieving concentration-tunable oxygen vacancies in Bi2O2CO3: triple-functional role for efficient visible-light photocatalytic redox performance. Appl. Catal. B 226, 441–450. doi: 10.1016/j.apcatb.2017.12.074
Zhang, G. G., Lan, Z. A., and Wang, X. C. (2016). Conjugated polymers: catalysts for photocatalytic hydrogen evolution. Angew. Chem. Int. Ed. 55, 15712–15727. doi: 10.1002/anie.201607375
Zhang, J., and Ma, Z. (2017). Enhanced visible-light photocatalytic performance of Ag3VO4/Bi2WO6 heterojunctions in removing aqueous dyes and tetracycline hydrochloride. J. Taiwan Inst. Chem. Eng. 78, 212–218. doi: 10.1016/j.jtice.2017.06.002
Zhang, L., Zhang, Q., Xie, H., Guo, J., Lyu, H., Li, Y., et al. (2017). Electrospun titania nanofibers segregated by graphene oxide for improved visible light photocatalysis. Appl. Catal. B 201, 470–478. doi: 10.1016/j.apcatb.2016.08.056
Zhao, T. Y., Zai, J. T., Xu, M., Zou, Q., Su, Y. Z., Wang, K. X., et al. (2011). Hierarchical Bi2O2CO3 microspheres with improved visible-light-driven photocatalytic activity. CrystEngComm 13:4010. doi: 10.1039/c1ce05113j
Zhong, Y., Liu, Y., Wu, S., Zhu, Y., Chen, H., Yu, X., et al. (2018). Facile fabrication of BiOI/BiOCl immobilized films with improved visible light photocatalytic performance. Front. Chem. 6:58. doi: 10.3389/fchem.2018.00058
Keywords: Ag3VO4, Bi2O2CO3, heterojunction, visible-light-driven, toxic pollutant removal
Citation: Li S, Hu S, Jiang W, Liu Y, Liu Y, Zhou Y, Mo L and Liu J (2018) Ag3VO4 Nanoparticles Decorated Bi2O2CO3 Micro-Flowers: An Efficient Visible-Light-Driven Photocatalyst for the Removal of Toxic Contaminants. Front. Chem. 6:255. doi: 10.3389/fchem.2018.00255
Received: 14 May 2018; Accepted: 08 June 2018;
Published: 27 June 2018.
Edited by:
Junguang Tao, Hebei University of Technology, ChinaReviewed by:
Shiba Adhikari, Oak Ridge National Laboratory (DOE), United StatesWenyao Li, Shanghai University of Engineering Sciences, China
Copyright © 2018 Li, Hu, Jiang, Liu, Liu, Zhou, Mo and Liu. This is an open-access article distributed under the terms of the Creative Commons Attribution License (CC BY). The use, distribution or reproduction in other forums is permitted, provided the original author(s) and the copyright owner are credited and that the original publication in this journal is cited, in accordance with accepted academic practice. No use, distribution or reproduction is permitted which does not comply with these terms..
*Correspondence: Shijie Li, lishijie@zjou.edu.cn
Yingtang Zhou, zhouyingtang@zjou.edu.cn