- 1State Key Laboratory of Polymer Physics and Chemistry, Changchun Institute of Applied Chemistry, Chinese Academy of Sciences, Changchun, China
- 2University of Science and Technology of China, Hefei, China
- 3University of Chinese Academy of Sciences, Beijing, China
The development of non-fullerene acceptor molecules have remarkably boosted power conversion efficiency (PCE) of polymer solar cells (PSCs) due to the improved spectral coverage and reduced energy loss. An introduction of fullerene molecules into the non-fullerene acceptor-based blend may further improve the photovoltaic performance of the resultant ternary PSCs. However, the underlying mechanism is still debatable. Herein, the ternary PSCs based on PBDB-T:ITIC:PC71BM blend were fabricated and its PCE was increased to 10.2% compared to 9.2% for the binary PBDB-T:ITIC devices and 8.1% for the PBDB-T:PC71BM PSCs. Systematic investigation was carried out to disclose the effect of PC71BM on the blend morphology and charge transport behavior. It is found that the PC71BM tends to intermix with the PBDB-T donor compared to the ITIC counterpart. A small amount of PC71BM in the ternary blend is helpful for ITIC to aggregate and form efficient electron-transport pathways. Accordingly, the electron mobility is increased and the density of electron traps is decreased in the ternary blend in comparison with the PBDB-T:ITIC blend. Finally, the suppressed bimolecular recombination and enhanced charge collection lead to high PCE for the ternary solar cells.
Introduction
Non-fullerene acceptors have drawn great research interests in the community of polymer solar cells (PSCs) in recent years. The power conversion efficiencies (PCE) of PSCs employing non-fullerene acceptors have increased rapidly as compared to the PSCs using fullerene derivatives as acceptors (Lin et al., 2015; Cao et al., 2017; Fan et al., 2017; Li et al., 2017; Xiao et al., 2017; Dai et al., 2018). Non-fullerene acceptors possess some advantages such as strong absorption in the visible region and tunable energy levels with regard to fullerene derivatives, and thus allows for suitable combination of donor/acceptor blend to improve the spectral coverage and reduce the energy loss (Holliday et al., 2016; Li et al., 2016, 2018; Qiu et al., 2017). In addition to the strong and complementary absorption and the matched energy levels of the donor/acceptor combination, the donor/acceptor morphology with a suitable phase separation is equally important for realizing a high PCE. More recently, addition of another type of donor or acceptor in the binary non-fullerene PSCs to fabricate so-called ternary PSCs have drawn great interests. This kind of ternary strategy is to some extent powerful to enhance the photovoltaic performance of the devices (Cheng et al., 2014; Gasparini et al., 2016). As it is argued, ternary PSCs possess some features such as more complementary absorption (Jiang et al., 2018) and more appropriate microstructure relative to the binary counterparts (Wang et al., 2017) and easier fabrication compared with tandem solar cells. With these superiorities, ternary PSCs have developed very quickly and become a research focus in the field (Yu et al., 2017; Zhang G. et al., 2017; Zhao et al., 2017; Wang et al., 2018). Nonetheless, the morphology of ternary blends is more complex resulting in the underlying mechanism debatable. It is proposed that the ternary morphology can be divided into four types according to the relative position of third component to the donor phases and acceptor phases, namely the third component embedded in one phase, located at the interfaces, formed alloy structure with either the donor or acceptor material and parallel-like bulk heterojunction structure with the donor or acceptor (Lu et al., 2015). In fact, the ternary morphology is too complicated to be clearly identified using the current technology. Typical electron acceptor fullerene derivatives, such as PC71BM and Bis-PC71BM have been used as additives in non-fullerene PSCs. For example, Bo et al. have reported high-performance ternary PSCs employing non-fullerene and fullerene acceptors simultaneously for the first time, in which they found that a small amount of fullerene is in favor of boosting the photovoltaic performance of the devices (Lu et al., 2016). Hou et al. also reported the high-efficiency ternary PSCs using Bis-PC71BM as the third component (Zhao et al., 2017). They proposed that Bis-PC71BM mainly exists in the upper surface of active layer and promotes electron transport in their ternary blend PSCs. For the ternary PSCs, the third component such as PC71BM may have strong effect on the resultant ternary morphology and hence its photovoltaic properties. Although some researches on the function of third component were carried out (Yu et al., 2017; Zhang J. et al., 2017; Wang et al., 2018), the underlying mechanism is still debatable.
Herein, a reported wide bandgap polymer poly[(2,6- (4,8-bis(5-(2-ethylhexyl)thiophen-2-yl)-benzo[1,2-b:4,5-b′] dithiophene))-alt-(5,5-(1′,3′-di-2-thienyl-5′,7′-bis(2-ethylhexyl) benzo[1′,2′-c:4′,5′-c′]dithiophene-4,8-dione))] (PBDB-T) is used as the donor, organic molecule (3,9-bis(2-methylene-(3- (1,1-dicyanomethylene)-indanone))- 5,5,11,11-tetrakis(4-hexylphenyl)-dithieno[2,3-d:2′,3′-d′]-s-indaceno[1,2-b:5,6-b′] dithiophene) (ITIC) is used as the acceptor and [6,6]-phenyl-C71-butyric acid methyl ester (PC71BM) is used as the third component to prepare the ternary PSCs. The optimized PSCs based on ternary PBDB-T:ITIC:PC71BM blend demonstrate a higher PCE of 10.2% than 9.2% of the binary PBDB-T:ITIC devices and 8.1% of the PBDB-T:PC71BM PSCs. Further studies are mainly focused on the effect of PC71BM on the blend morphology and charge transport behavior. It is found that the PC71BM tends to intermix with the PBDB-T donor compared to the ITIC counterpart. A small amount of PC71BM in the ternary blend is helpful for ITIC to aggregate and form efficient electron-transport pathways. Accordingly, the electron mobility is increased and the density of electron traps is decreased in the ternary blend in comparison with the PBDB-T:ITIC blend. Finally, the suppressed bimolecular recombination and enhanced charge collection lead to an enhanced PCE for the ternary solar cells.
Experimental Section
Both the polymer PBDB-T donor and small molecule ITIC acceptor were bought from Solarmer Ltd. The PBDB-T has a molecular weight Mn of 21.5 kDa and a PDI of 1.9. PC71BM was bought from American Dye Source. The interfacial material PDINO was provided by Dr. Zhiguo Zhang in Institute of Chemistry, Chinese Academy of Sciences. All materials were used as received without further purification.
Polymer solar cells were fabricated with a structure of ITO/PEDOT: PSS/active layer/PDINO/Al. The ITO substrates were subject to routine cleaning procedure of detergent, acetone and deionized water. After drying in an oven for 30 min, the ITO substrates were treated with UV-ozone for 25 min. The PEDOT:PSS layer was first deposited via spin-coating and dried at 140°C for 30 min in air. The subsequent active layer and buffer layer were spin-coated in a glove box. The active layers consisting of PBDB-T:PC71BM, PBDB-T:ITIC, or ternary PBDB-T:ITIC:PC71BM were spin-coated from their respective solutions in CB containing 0.5% DIO with a total concentration of 20 mg/mL. The spin-coating rate for the active layers was kept at 2,500 rpm for 1 min. The samples were annealed at 160°C for 10 min. A cathode buffer layer of PDINO was spin-coated on the active layer from its methanol solution with a concentration of 1 mg/mL at 3,000 rpm for 30 s. Finally, the Al cathode with a thickness of 100 nm was thermally deposited in a vacuum chamber. The electron—and hole-only devices were fabricated under the same procedure with a structure of ITO/PEIE/active layer/PDINO/Al and ITO/PEDOT:PSS/active layer/MoO3/Al, respectively.
The current density-voltage (J-V) curves of the PSCs were traced by a computer-controlled Keithley 2400 Source Meter under simulated solar light illumination (AM 1.5G, 100 mW/cm2). The EQE data were measured by solar cell spectral response measurement system (QE-R 3011, Enli Tech. Co.). The film absorption and fluorescence spectra were recorded on Agilent Cary 60UV-Vis spectrophotometer and Perkin-Elmer LS 55 spectrofluorometer, respectively. The thicknesses of individual layers were measured with a surface profilometer. The 2D-GIXD data were acquired at station 14B in Shanghai Synchrotron Radiation Facility.
Results and Discussion
The chemical structures of PBDB-T, ITIC and PC71BM are shown in Figure 1A. The normalized UV-vis absorption spectra of neat PBDB-T, ITIC and PC71BM films are plotted in Figure 1B. It is clearly seen from Figure 1B that the main peaks of PBDB-T and ITIC are located at 625 and 710 nm, respectively, and their complementary absorption can strongly improve the spectral coverage in the visible region. PC71BM exhibits absorption in short wavelength region but its absorption capability is relatively weak. In this study, the PSCs with a conventional device structure of ITO/PEDOT:PSS/active layer/PDINO/Al were fabricated, and their energy levels were plotted in Figure 1C. The polymer PDINO was used as cathode buffer layer referenced to the literature (Zhang Z. et al., 2014). The total ratio of donor component to acceptor component is kept at a fixed ratio of 1:1 (w/w). The ratio of PBDB-T:ITIC:PC71BM is marked as the D:A1:A2.
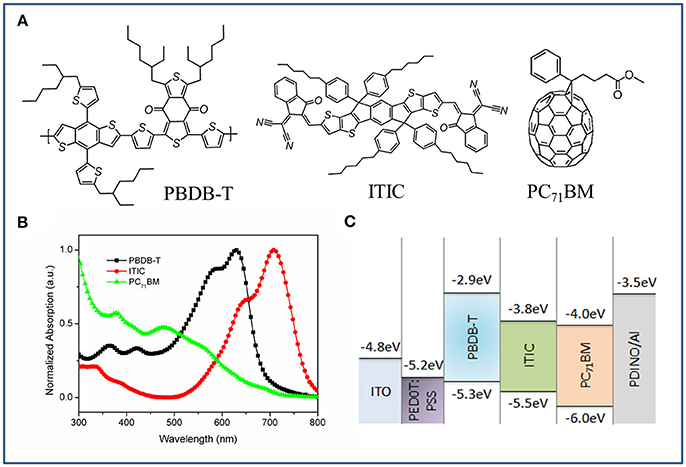
Figure 1. (A) Chemical structures of PBDB-T, ITIC, and PC71BM. (B) Normalized absorption of pure PBDB-T, ITIC, and PC71BM films. (C) Energy level diagram of solar cells.
The photovoltaic performance of the PSCs based on the PBDB-T:ITIC:PC71BM (1:1-x:x) blend were firstly evaluated. The ratio of A1:A2 is varied from 1:0 to 0:1 while the ratio of D:(A1+A2) is fixed at 1:1 in order to keep a constant active layer thickness. The detailed photovoltaic parameters of the resultant PSCs with different ratio of two acceptors are listed in Table S1 in Supplementary Information. The dependence of VOC, JSC, FF, and PCE on the PC71BM content for the resultant PSCs are plotted in Figure 2A. In the case of VOC, it undergoes gradually decrease from 0.902 V for the binary PBDB-T:ITIC PSCs to 0.856 V for the binary PBDB-T: PC71BM PSCs with increasing PC71BM content from 0 to 1 in the ternary blend PSCs. The change is reasonable since the LUMO of ITIC is higher than that of PC71BM, and the electron transport and collection will occur in PC71BM phases when the PC71BM amount is larger. The interesting thing is that the JSC are initially increased and then decreased with the increase of PC71BM contents for the resultant ternary PSCs. The FF shows similar trend. Considering the intrinsic absorption properties between ITIC and PC71BM, the substitution of small amount of ITIC by PC71BM would decrease the total absorption of the ternary PBDB-T:ITIC:PC71BM blend active layer. Thus, the improved JSC and FF for the ternary PBDB-T:ITIC:PC71BM PSCs may imply that both the exciton dissociation and charge-collection efficiencies are enhanced in comparison to the binary PBDB-T:ITIC PSCs. It is plotted the illuminated J-V curves of the PBDB-T:ITIC PSCs, the PBDB-T:PC71BM PSCs and the optimized ternary PBDB-T:ITIC:PC71BM PSCs in Figure 2B, respectively. The non-fullerene PSCs based on binary PBDB-T:ITIC blend demonstrate a VOC of 0.902 V, a JSC of 15.06 mA/cm2, a FF of 0.69, respectively, leading to a PCE of 9.38%. Due to the limited spectral coverage, the PBDB-T:PC71BM PSCs show a lower JSC of 13.64 mA/cm2, and finally result in a PCE of 8.21% together with a VOC of 0.856 V and a FF of 0.703. The ternary PBDB-T:ITIC:PC71BM (1:0.8:0.2) PSCs demonstrate a VOC of 0.892 V, a JSC of 15.98 mA/cm2, a FF of 0.717 and an overall PCE of 10.22%. Although the VOC is a little lowered in comparison to the binary PBDB-T:ITIC PSCs, the increased JSC and FF boost the PCE enhancement of the ternary PSCs. The external quantum efficiency (EQE) curves of the three devices are shown in Figure 2C. The spectral response of the PBDB-T:PC71BM PSCs cover at a range of 300–700 nm with EQE higher than 70% at 450–650 nm. The PBDB-T:ITIC PSCs show extended spectral coverage of 300–800 nm due to the narrow bandgap of ITIC. The ternary PBDB-T:ITIC:PC71BM PSCs show similar spectral response profile but high EQE compared to the PBDB-T:ITIC PSCs. The internal quantum efficiency (IQE) of these devices are also measured to clarify the absolute quantum efficiency in these devices and the curves are plotted in Figure 2D. It indicates that the photon-to-electron conversion efficiency is really improved by adding some amount of PC71BM into the PBDB-T:ITIC blend. The absorption spectra of the active layers in three kinds of PSCs are plotted in Figure 3A. The PBDB-T:ITIC:PC71BM (1:0.8:0.2) active layer shows a little low absorption at 550–750 nm due to decreased ITIC content compared to the PBDB-T:ITIC (1:1) film. Although the PBDB-T:PC71BM (1:1) film demonstrates enhanced absorption at 300–500 nm, its absorption at 550–700 nm is remarkably decreased. Photoluminescence (PL) quenching experiments were carried out to check the charge transfer status in these films. As shown in Figure 3B, the introduction of PC71BM favors to quench the excitons dominated on the PBDB-T donor.
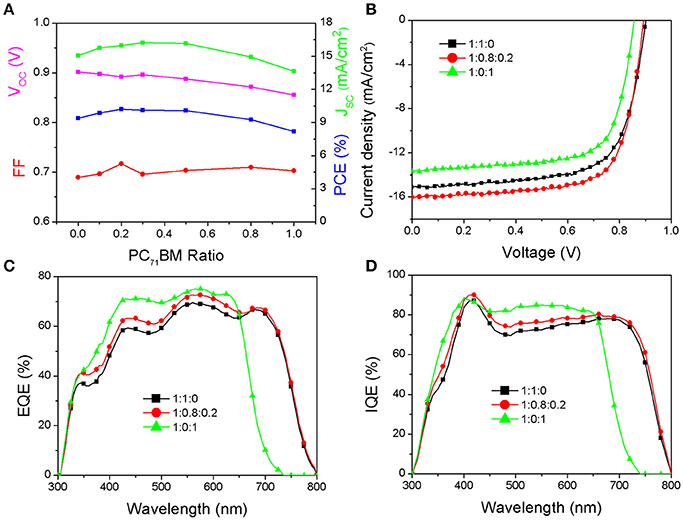
Figure 2. (A) The photovoltaic parameters of the PBDB-T:ITIC:PC71BM (1:1-x:x) PSCs. The J-V (B), EQE (C), and IQE (D) curves of PSCs based on PBDB-T:ITIC (1:1), PBDB-T:ITIC:PC71BM (1:0.8:0.2), and PBDB-T:PC71BM (1:1) blend films, respectively.
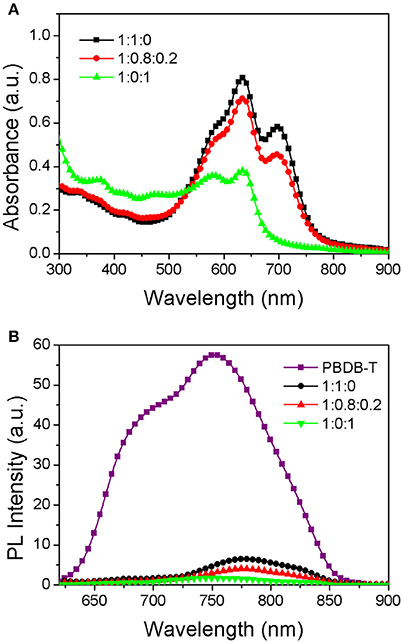
Figure 3. (A) Absolute absorption spectra of PBDB-T:ITIC (1:1), PBDB-T:ITIC:PC71BM (1:0.8:0.2), and PBDB-T:PC71BM (1:1) blend films. (B) Photoluminescent spectra of pure PBDB-T, PBDB-T:ITIC (1:1), PBDB-T:ITIC:PC71BM (1:0.8:0.2), and PBDB-T:PC71BM (1:1) films.
The relationship between photocurrent (Jph) and effective voltage (Veff) is investigated to judge the charge generation and collection status in the PSCs based on the PBDB-T:ITIC (1:1), PBDB-T:ITIC:PC71BM (1:0.8:0.2), and PBDB-T:PC71BM (1:1) blends (Yuan et al., 2017; Zhang G. et al., 2017). As shown in Figure 4A, Jph is given by Jph = JL − JD, where JL & JD are the current density under illumination and in the dark, respectively. Veff is defined as V0 − Vappl, V0 is the voltage when Jph = 0 and Vappl is the applied voltage during the measurement. The Jph is supposed to be saturated at a Veff of 3 V, which are 16.55 mA/cm2, 17.40 mA/cm2 and 14.45 mA/cm2, respectively, for the PSCs based on the PBDB-T:ITIC (1:1), PBDB-T:ITIC:PC71BM (1:0.8:0.2), and PBDB-T:PC71BM (1:1) blends. The device based on ternary PBDB-T:ITIC:PC71BM (1:0.8:0.2) blend demonstrates the highest saturation current, indicating its highest charge-generation capability. Charge dissociation probability P(E,T) is defined as Jph/Jsat. When a high bias is applied, the charge recombination is suppressed and most of photo-generated charges are extracted, leading to P(E,T) close to100%. It is calculated that the P(E,T) values under short-circuit condition (Vappl = 0 V) are 90.6, 91.9, and 94.9%, respectively, for the PSCs based on the PBDB-T:ITIC (1:1), PBDB-T:ITIC:PC71BM (1:0.8:0.2), and PBDB-T:PC71BM (1:1) blends. The PBDB-T:PC71BM (1:1) device shows the best charge-extraction capability, whereas the charge-extraction ability is a little poor for the PBDB-T:ITIC (1:1) device. The ternary PBDB-T:ITIC:PC71BM (1:0.8:0.2) shows improved exciton dissociation and charge extraction compared to the PBDB-T:ITIC (1:1) device. The charge recombination status in these devices is also investigated via the dependence of JSC on the incident light intensity as shown in Figure 4B. JSC is dependent on the incident light intensity with JSC∝Iα, in which exponential factor α would be close to unity without bimolecular recombination (Gao et al., 2016; Xu et al., 2017, 2018). The PBDB-T:PC71BM (1:1) device has the highest α value of 0.964, whereas α value of the PBDB-T:ITIC (1:1) device is merely 0.935 implying severe bimolecular recombination within the film. Such kind of bimolecular recombination in the PBDB-T:ITIC (1:1) device is to some extent suppressed with α value of 0.955 in ternary PBDB-T:ITIC:PC71BM (1:0.8:0.2) device.
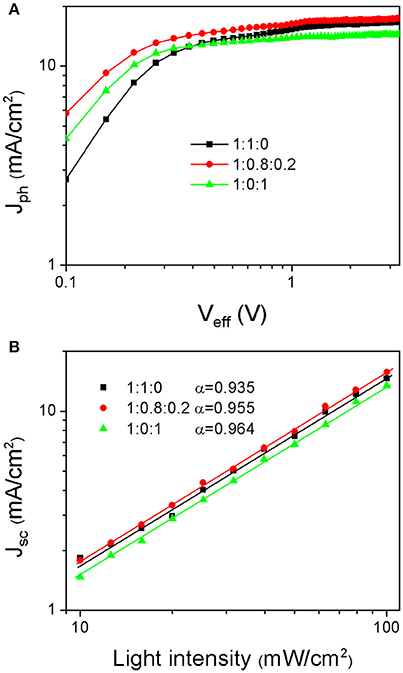
Figure 4. (A) Jph – Veff curves and (B) JSC dependence on the illuminated light intensity of PSCs based on PBDB-T:ITIC (1:1), PBDB-T:ITIC:PC71BM (1:0.8:0.2), and PBDB-T:PC71BM (1:1) blend films, respectively.
As discussed above, with the introduction of PC71BM into the PBDB-T:ITIC blend, the resultant ternary PSCs demonstrate an enhanced exciton-dissociating and charge-extracting property, leading to improved PCE compared to the PBDB-T:ITIC PSCs. The detailed mechanism accounting for the enhancement is further investigated. The electron and hole transport properties of the various blend films were firstly measured via space-charge limited current (SCLC) method (Bin et al., 2016). The J-V curves of the devices were plotted as in Figure S1 and the calculated electron and hole mobility are listed in Table S2. The PBDB-T:PC71BM (1:1) blend film shows relatively high electron and hole mobility of 8.45 × 10−4 and 5.30 × 10−4 cm2V−1s−1, respectively. However, the electron and hole mobility of the PBDB-T:ITIC (1:1) film is 3.05 × 10−4 and 2.70 × 10−4 cm2V−1s−1. After incorporating PC71BM, the electron and hole mobility of the resultant PBDB-T:ITIC:PC71BM (1:0.8:0.2) film is increased to 4.55 × 10−4 and 3.37 × 10−4 cm2V−1s−1. As it is known, the high and balanced charge transport may help to efficient charge extraction and hence low charge recombination. This is in agreement with the recombination status in these PSCs. It is speculated that the incorporation of PC71BM improves the PBDB-T and ITIC interpenetrating networks and thus the photovoltaic performance.
The morphology of the PBDB-T:ITIC (1:1), PBDB-T:ITIC:PC71BM (1:0.8:0.2), and PBDB-T:PC71BM (1:1) blend films are investigated and their AFM and TEM images are shown in Figures S2, S3. All the blend films are smooth with a root mean square (RMS) roughness of <2 nm. However, the morphology of the ternary blend is not changed obviously compared to the PBDB-T:ITIC (1:1) blend film. The structural information of the blended films are further investigated by grazing incidence X-ray diffraction (GIXD). The 2D-GIXD images of the pure PBDB-T and ITIC films are plotted in Figure S4. The pure PBDB-T film shows dominant peak at qxy = 0.29 Å−1 in the in-plane direction, attributing to its strong (100) diffraction. The pure ITIC film demonstrates two featured peaks at qz = 0.26 Å−1 and qz = 0.53 Å−1 in out-of-plane direction and one peak in in-plane direction at qxy = 0.36 Å−1. 2D-GIXD patterns and line-cut profiles of the PBDB-T:ITIC (1:1), PBDB-T:ITIC:PC71BM (1:0.8:0.2) and PBDB-T:PC71BM (1:1) films are shown in Figure 5. The diffractions originated from PBDB-T aggregation is observed both in PBDB-T:ITIC (1:1) and PBDB-T:PC71BM (1:1) films in contrast to the pure PBDB-T film. However, the diffraction signal originated from the ITIC component is not obviously presented in PBDB-T:ITIC blend film, implying its poor aggregation. In the PBDB-T:ITIC:PC71BM (1:0.8:0.2) blend film, the diffraction signal from PBDB-T is still presented but weakened. More importantly, two additional peaks marked 1 and 2 are presented in the ternary blend film compared to the PBDB-T:ITIC (1:1) counterpart, which corresponds to the featured diffraction from ITIC in the in-plane and out-of-plane directions. Bo et al. observed similar phenomenon in their work (Lu et al., 2016). This indicates that the ITIC aggregation is enhanced in the PBDB-T:ITIC:PC71BM (1:0.8:0.2) blend film though the ITIC content is decreased. In other words, the small amount of PC71BM may serve as “lubricant” to favor ITIC molecules to aggregate out of the polymer PBDB-T matrix.
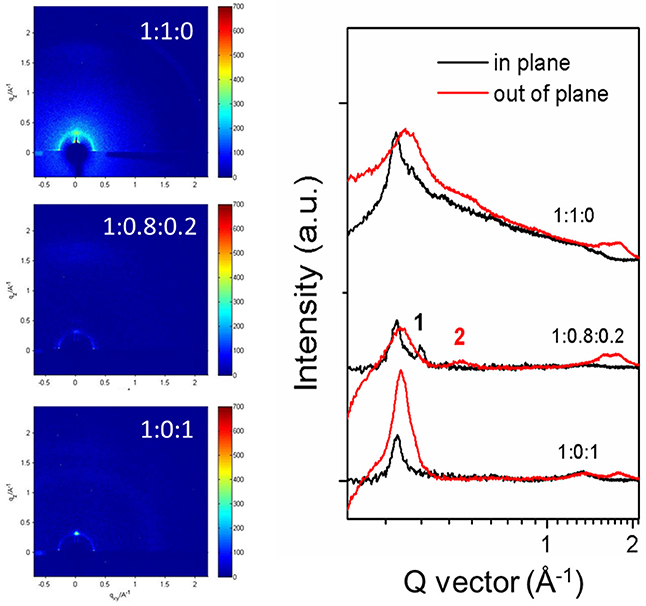
Figure 5. 2D-GIXD images of PBDB-T:ITIC (1:1), PBDB-T:ITIC:PC71BM (1:0.8:0.2), and PBDB-T:PC71BM (1:1) blend films (Left). The in-plane and out-of-plane line profiles of the films (Right).
The prerequisite to get such a conclusion is that the compatibility between PBDB-T and PC71BM should be better than that between PBDB-T and ITIC. The electron mobility of the PBDB-T:ITIC (1:x) and PBDB-T:PC71BM (1:x) blend films are measured and its dependence on the acceptor ratio is plotted in Figure 6. It is supposed that the acceptor phase forms continuous tunnels when the electron mobility of the blend films exceeds 10−5 cm2V−1s−1. Both the as-prepared film deposited in CB solution and the annealed film deposited in CB:DIO (0.5%, v/v) solution are tested. The results show that the continuous electron-transport tunnels is formed in the PBDB-T:ITIC film with the ITIC/PBDB-T ratio <20%. The DIO additive and thermal annealing donot change the electron transport remarkably. For the as-prepared PBDB-T:PC71BM film, the critical PC71BM content is increased to 25%. The DIO additive and thermal annealing lowers the critical PC71BM content. This indicates that the PBDB-T is inclined to intermix with PC71BM better than with ITIC. In the ternary PBDB-T:ITIC:PC71BM (1:0.8:0.2) blend film, the small amount of PC71BM may mix with PBDB-T and does not form networks. Moreover, the existence of PC71BM favors ITIC aggregating to form electron-transporting networks. This is confirmed by the increased electron mobility and the XRD results compared to the PBDB-T:ITIC (1:1) film.
The electron trap-state density in these blend films is further investigated. From the I–V curves of the electron-only devices based on the PBDB-T:ITIC (1:1), PBDB-T:ITIC:PC71BM (1:0.8:0.2) and PBDB-T:PC71BM (1:1) blend shown in Figure 7, the electron trap density N is calculated by the equation as below (Yang et al., 2016):
where VTFL is the trap-filled limit voltage, e is the elementary charge of electron, L is the thickness of film, ϵ0 is the vacuum permittivity, and ϵr is the relative dielectric constant (ϵr = 3). The VTFL of three devices is 0.12, 0.05, and 0.02 V. The calculated deep trap density is 3.3 × 1015, 1.5 × 1015, and 7.3 × 1014 cm−3, respectively. It confirms that the incorporation of PC71BM is helpful for ITIC molecules to move out of PBDB-T phases and thus the density of electron traps is reduced. The increased electron mobility and the decreased electron traps are helpful to enhance the photovoltaic performance of the PBDB-T:ITIC:PC71BM (1:0.8:0.2).
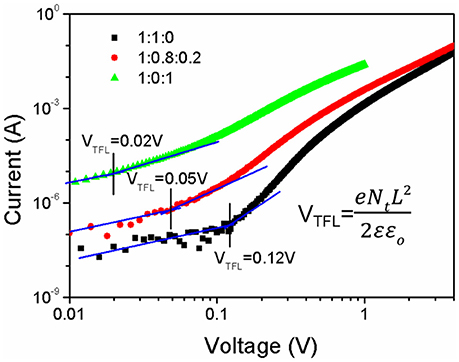
Figure 7. The current-voltage curves of the electron-only devices based on the PBDB-T:ITIC (1:1), PBDB-T:ITIC:PC71BM (1:0.8:0.2), and PBDB-T:PC71BM (1:1) blend films.
Conclusion
In summary, the ternary PSCs based on PBDB-T:ITIC:PC71BM (1:0.8:0.2) blend were fabricated and its PCE was increased to 10.2% compared to 9.2% for the PBDB-T:ITIC (1:1) devices. The mechanism accounting for the enhanced photovoltaic performance is discussed in detail. It is found that the PC71BM tends to intermix with the PBDB-T donor compared to the ITIC counterpart. A small amount of PC71BM in the ternary blend is helpful for ITIC to aggregate and form efficient electron-transport pathways. The electron mobility is increased and the density of electron traps is decreased in the ternary PBDB-T:ITIC:PC71BM (1:0.8:0.2) blend in comparison with the PBDB-T:ITIC blend. Finally, the suppressed bimolecular recombination and enhanced charge collection lead to a high PCE for the ternary solar cells.
Author Contributions
BW and CY conceived and designed the experiments. BW performed the experiments. RZ performed 2D-GIXD measurements. QY performed the absorption and PL experiments. BW and YF analyzed data. BW wrote the manuscript. All authors discussed and commented on the paper.
Conflict of Interest Statement
The authors declare that the research was conducted in the absence of any commercial or financial relationships that could be construed as a potential conflict of interest.
Acknowledgments
This work is supported by the National Key Basic Research and Development Program of China (Nos. 2014CB643504, 2015CB655001) and the National Natural Science Foundation of China (nos.21774122, 21334006, 51611530705, 51773195, 51703222). The financial support of the Strategic Priority Research Program of the Chinese Academy of Sciences (XDB12030200) is also acknowledged. We also thank Shanghai Synchrotron Radiation Facility (SSRF) beamline 14B for the grazing X-ray measurements.
Supplementary Material
The Supplementary Material for this article can be found online at: https://www.frontiersin.org/articles/10.3389/fchem.2018.00198/full#supplementary-material
References
Bin, H., Zhang, Z. G., Gao, L., Chen, S., Zhong, L., Xue, L., et al. (2016). Non-fullerene polymer solar cells based on alkylthio and fluorine substituted 2D-conjugated polymers reach 9.5% efficiency. J. Am. Chem. Soc. 138, 4657–4664. doi: 10.1021/jacs.6b01744
Cao, Q., Xiong, W., Chen, H., Cai, G., Wang, G., Zheng, L., et al. (2017). Design, synthesis, and structural characterization of the first dithienocyclopentacarbazole-based ntype organic semiconductor and its application in non-fullerene polymer solar cells. J. Mater. Chem. A 5, 7451–7461. doi: 10.1039/C7TA01143A
Cheng, P., Li, Y., and Zhan, X. (2014). Efficient ternary blend polymer solar cells with indene-C60 bisadduct as an electron-cascadeacceptor. Energy Environ. Sci. 7, 2005–2011. doi: 10.1039/c3ee44202k
Dai, S., Li, T., Wang, W., Xiao, Y., Lau, T. K., Li, Z., et al. (2018). Enhancing the performance of polymer solar cells via core engineering of nir-absorbing electron acceptors. Adv. Mater. 30:e1706571. doi: 10.1002/adma.201706571
Fan, Q., Su, W., Guo, X., Wang, Y., Chen, J., Ye, C., et al. (2017). Side-chain engineering for efficient non-fullerene polymer solar cells based on a wide-bandgap polymer donor. J. Mater. Chem. A 5, 9204–9209. doi: 10.1039/C7TA02075A
Gao, L., Zhang, Z. G., Bin, H., Xue, L., Yang, Y., Wang, C., et al. (2016). High-efficiency nonfullerene polymer solar cells with medium bandgap polymer donor and narrow bandgap organic semiconductor acceptor. Adv. Mater. 28, 8288–8295. doi: 10.1002/adma.201601595
Gasparini, N., Jiao, X., Heumueller, T., Baran, D., Matt, G., Fladischer, S., et al. (2016). Designing ternary blend bulk heterojunction solar cells with reduced carrier recombination and a fill factor of 77%. Nat. Energy 1:16118. doi: 10.1038/nenergy.2016.118
Holliday, S., Ashraf, R. S., Wadsworth, A., Baran, D., Yousaf, S. A., Nielsen, C. B., et al. (2016). High-efficiency and air-stable P3HT-based polymer solar cells with a new non-fullerene acceptor. Nat. Commun. 7:11585. doi: 10.1038/ncomms11585
Jiang, W., Yu, R., Liu, Z., Peng, R., Mi, D., Hong, L., et al. (2018). Ternary nonfullerene polymer solar cells with 12.16% efficiency by introducing one acceptor with cascading energy level and complementary absorption. Adv. Mater. 30:1703005. doi: 10.1002/adma.201703005
Li, S., Ye, L., Zhao, W., Zhang, S., Ade, H., and Hou, J. (2017). Signifcant influence of the methoxyl substitution position on optoelectronic properties and molecular packing of small-molecule electron acceptors for photovoltaic cells. Adv. Energy Mater. 7:1700183. doi: 10.1002/aenm.201700183
Li, S., Ye, L., Zhao, W., Zhang, S., Mukherjee, S., Ade, H., et al. (2016). Energy-level modulation of small-molecule electron acceptors to achieve over 12% effciency in polymer solar cells. Adv. Mater. 28, 9423–9429. doi: 10.1002/adma.201602776
Li, S., Zhan, L., Liu, F., Ren, J., Shi, M., Li, C. Z., et al. (2018). An unfused-core-based nonfullerene acceptor enables high-efficiency organic solar cells with excellent morphological stability at high temperatures. Adv. Mater. 30:1705208. doi: 10.1002/adma.201705208
Lin, Y., Wang, J., Zhang, Z. G., Bai, H., Li, Y., Zhu, D., et al. (2015). An electron acceptor challenging fullerenes for efficient polymer solar cells. Adv. Mater. 27, 1170–1174. doi: 10.1002/adma.201404317
Lu, H., Zhang, J., Chen, J., Liu, Q., Gong, X., Feng, S., et al. (2016). Ternary-blend polymer solar cells combining fullerene and nonfullerene acceptors to synergistically boost the photovoltaic performance. Adv. Mater. 28, 9559–9566. doi: 10.1002/adma.201603588
Lu, L., Kelly, M., You, W., and Yu, L. (2015). Status and prospects for ternary organic photovoltaics. Nat. Photonics 9, 491–500. doi: 10.1038/nphoton.2015.128
Qiu, N., Zhang, H., Wan, X., Li, C., Ke, X., Feng, H., et al. (2017). A new nonfullerene electron acceptor with a ladder type backbone for high-performance organic solar cells. Adv. Mater. 29:1604964. doi: 10.1002/adma.201604964
Wang, J., Peng, J., Liu, X., and Liang, Z. (2017). Efficient and stable ternary organic solar cells based on two planar nonfullerene acceptors with tunable crystallinity and phase miscibility. ACS Appl. Mater. Interfaces 9, 20704–20710. doi: 10.1021/acsami.7b03757
Wang, Z., Zhu, X., Zhang, J., Lu, K., Fang, J., Zhang, Y., et al. (2018). From alloy-like to cascade blended structure: designing high-performance all small molecule ternary solar cells. J. Am. Chem. Soc. 140, 1549–1556. doi: 10.1021/jacs.7b13054
Xiao, Z., Jia, X., Li, D., Wang, S., Geng, X., Liu, F., et al. (2017). 26 mA cm−2 Jsc from organic solar cells with a low-bandgap nonfullerene acceptor. Sci. Bull. 62, 1494–1497. doi: 10.1016/j.scib.2017.10.017
Xu, S., Wang, X., Feng, L., He, Z., Peng, H., Cimrová, V., et al. (2018). Optimizing the conjugated side chains of quinoxaline based polymers for nonfullerene solar cells with 10.5% efficiency. J. Mater. Chem. A 6, 3074–3083. doi: 10.1039/C7TA10262C
Xu, X., Bi, Z., Ma, W., Wang, Z., Choy, W. C. H., Wu, W., et al. (2017). Highly efficient ternary-blend polymer solar cells enabled by a nonfullerene acceptor and two polymer donors with a broad composition tolerance. Adv. Mater. 29:1704271. doi: 10.1002/adma.201704271
Yang, D., Yang, R., Ren, X., Zhu, X., Yang, Z., Li, C., et al. (2016). Hysteresis-suppressed high-efficiency flexible perovskite solar cells using solid-state ionic-liquids for effective electron transport. Adv. Mater. 28, 5206–5213. doi: 10.1002/adma.201600446
Yu, R., Zhang, S., Yao, H., Guo, B., Li, S., Zhang, H., et al. (2017). Two well-miscible acceptors work as one for efficient fullerene-free organic solar cells. Adv. Mater. 29:1700437. doi: 10.1002/adma.201700437
Yuan, J., Guo, W., Xia, Y., Ford, M. J., Jin, F., Liu, D., et al. (2017). Comparing the device physics, dynamics and morphology of polymer solar cells employing conventional PCBM and non-fullerene polymer acceptor N2200. Nano Energy 35, 251–262. doi: 10.1016/j.nanoen.2017.03.050
Zhang, G., Zhang, K., Yin, Q., Jiang, X. F., Wang, Z., Xin, J., et al. (2017). High-performance ternary organic solar cell enabled by a thick active layer containing a liquid crystalline small molecule donor. J. Am. Chem. Soc. 139, 2387–2395. doi: 10.1021/jacs.6b11991
Zhang, J., Zhao, Y., Fang, J., Yuan, L., Xia, B., Wang, G., et al. (2017). Enhancing performance of large-area organic solar cells with thick film via ternary strategy. Small 13:1700388. doi: 10.1002/smll.201700388
Zhang, Z., Qi, B., Jin, Z., Chi, D., Qi, Z., Li, Y., et al. (2014). Perylene diimides: a thickness-insensitive cathode interlayer for high performance polymer solar cells. Energy Environ. Sci. 7, 1966–1973. doi: 10.1039/c4ee00022f
Keywords: ternary organic solar cells, morphology, aggregation, charge transport, trap density
Citation: Wang B, Fu Y, Yan C, Zhang R, Yang Q, Han Y and Xie Z (2018) Insight Into the Role of PC71BM on Enhancing the Photovoltaic Performance of Ternary Organic Solar Cells. Front. Chem. 6:198. doi: 10.3389/fchem.2018.00198
Received: 09 April 2018; Accepted: 15 May 2018;
Published: 05 June 2018.
Edited by:
Donghong Yu, Aalborg University, DenmarkReviewed by:
Junwu Chen, South China University of Technology, ChinaZhishan Bo, Beijing Normal University, China
Copyright © 2018 Wang, Fu, Yan, Zhang, Yang, Han and Xie. This is an open-access article distributed under the terms of the Creative Commons Attribution License (CC BY). The use, distribution or reproduction in other forums is permitted, provided the original author(s) and the copyright owner are credited and that the original publication in this journal is cited, in accordance with accepted academic practice. No use, distribution or reproduction is permitted which does not comply with these terms.
*Correspondence: Zhiyuan Xie, xiezy_n@ciac.ac.cn