- 1Department of Chemistry, Abbottabad University of Science & Technology, Havelian, Pakistan
- 2Department of Chemistry, COMSATS Institute of Information Technology, Abbottabad, Pakistan
- 3UoN Chair of Oman's Medicinal Plants and Marine Natural Products, University of Nizwa, Nizwa, Oman
- 4Department of Pharmacy, University of Peshawar, Peshawar, Pakistan
- 5Department of Chemistry, Quaid-i-Azam University, Islamabad, Pakistan
- 6School of Chemistry, Cardiff University, Cardiff, United Kingdom
In a continuation of our previous work for the exploration of novel enzyme inhibitors, two new coumarin-thiazole 6(a–o) and coumarin-oxadiazole 11(a–h) hybrids have been designed and synthesized. All the compounds were characterized by 1H- and 13C-NMR spectroscopy and elemental analysis. New hybrid analogs were evaluated against acetylcholinesterase (AChE) and butyrylcholinesterase (BuChE) in order to know their potential for the prevention of Alzheimer's disease (AD). In coumarinyl thiazole series, compound 6b was found as the most active member against AChE having IC50 value of 0.87 ± 0.09 μM, while the compound 6j revealed the same efficacy against BuChE with an IC50 value of 11.01 ± 3.37 μM. In case of coumarinyl oxadiazole series, 11a was turned out to be the lead candidate against AChE with an IC50 value of 6.07 ± 0.23 μM, whereas compound 11e was found significantly active against BuChE with an IC50 value of 0.15 ± 0.09 μM. To realize the binding interaction of these compounds with AChE and BuChE, the molecular docking studies were performed. Compounds from coumarinyl thiazole series with potent AChE activity (6b, 6h, 6i, and 6k) were found to interact with AChE in the active site with MOE score of −10.19, −9.97, −9.68, and −11.03 Kcal.mol−1, respectively. The major interactions include hydrogen bonding, π-π stacking with aromatic residues, and interaction through water bridging. The docking studies of coumarinyl oxadiazole derivatives 11(a–h) suggested that the compounds with high anti-butyrylcholinesterase activity (11e, 11a, and 11b) provided MOE score of −9.9, −7.4, and −8.2 Kcal.mol−1, respectively, with the active site of BuChE building π-π stacking with Trp82 and water bridged interaction.
Introduction
Alzheimer's disease (AD), the most common cause of dementia, is a neurodegenerative disorder mainly characterized by progressive deterioration of memory and cognition (Terry and Buccafusco, 2003). One of the key therapeutic strategies adopted for primarily symptomatic AD is based on the cholinergic hypothesis targeting cholinesterase enzymes (acetylcholinesterase and butyrylcholinesterase; Cummings et al., 2007), two important enzymes from the group of serine hydrolases. Structurally, these serine hydrolases belong to the class of proteins known as the esterase/lipase family within the α/β-hydrolase fold superfamily (Cygler et al., 1993). The major role of AChE is the inhibition of the hydrolysis of acetylcholine in cholinergic synapses. Thus, blocking its metabolic activity and increasing the ACh concentration ultimately leading to a possible symptomatic treatment option for AD, whereas, the functional activity of butyrylcholinesterase (BChE) is less understood because it can hydrolyze ACh as well as other esters (Groner et al., 2007; Chiou et al., 2009). Butyrylcholinesterase has recently been considered as a potential target because it also plays an important role in regulating ACh level (Mesulam et al., 2002). AChE inhibitors currently approved as drugs for the treatment of Alzheimer's disease are donepezil, rivastigmine, galantamine, and tacrine (Figure 1). Although, donepezil is most commonly used AChE inhibitor, its Aβ formation inhibition activity is weak (Bartolini et al., 2003). In view of the limited number of cholinesterase inhibitors currently available for the treatment of AD, the search for new and potent inhibitors is of significant interest and a progressive area of current research.
Among oxygenated heterocycles, coumarin compounds have sustained efficacy as they inhibit both acetyl- and butyrylcholinesterase enzymes and help to slow down the formation of amyloid compounds (de Souza et al., 2016). Coumarins, both natural and synthetic demonstrate a wide spectrum of biological functions as they offer a wide range of structural changes on benzopyran ring. Activities like anti-tubercular (Manvar et al., 2011), anti-tumor (Maddi et al., 2007), anti-HIV (Kashman et al., 1992), anti-inflammatory (Ronad et al., 2010), anti-cancer (Olmedo et al., 2012), and anticoagulant (Martin-Aragón et al., 2001) have been reported. In addition, thiazole and oxadiazole skeletons are fundamentally important and versatile structural analogs of five-membered heterocyclic compounds. They show a vast majority of biological activities (Klimesová et al., 2004; Hang and Honek, 2005; Campiglia et al., 2009; Siddiqui et al., 2009; Jaishree et al., 2012; Romagnoli et al., 2012; Helal et al., 2013; Naveena et al., 2013; Venugopala et al., 2013; Yavari et al., 2014) in addition to be a part of numerous complex natural products like vitamin B1, penicillin (Shaker, 2006), and thiamine pyrophosphate, an important co-enzyme.
In the present study, two new coumarin-thiazole 6(a–o) and coumarin-oxadiazole 11(a–h) hybrids were synthesized and evaluated for their acetylcholinesterase (AChE) and butyrylcholinesterase (BuChE) inhibitory activity. Furthermore, the molecular docking studies on both series were also performed to explore their binding interactions.
Results and Discussion
Chemistry
Two series of coumarinyl thiazoles 6(a–o) and oxadiazoles 11(a–h) were prepared with the aim to identify new and potent inhibitors of acetylcholinesterase and butyrylcholinesterase. Coumarinyl thiazole derivatives 6(a–o) were accessed through a multi-component reaction approach which starts with the preparation of 3-(2-bromoacetyl)-2H-chromen-2-one (3) via base-catalyzed condensation of readily available starting materials (salicylaldehyde and ethyl acetoacetate) followed by bromination (Scheme 1; Ibrar et al., 2016). An acid-catalyzed one-pot reaction of intermediate 3, different substituted acetophenones (4) and thiosemicarbazide (5) provided the title compounds 6(a–o) in good yields (Ibrar et al., 2016).
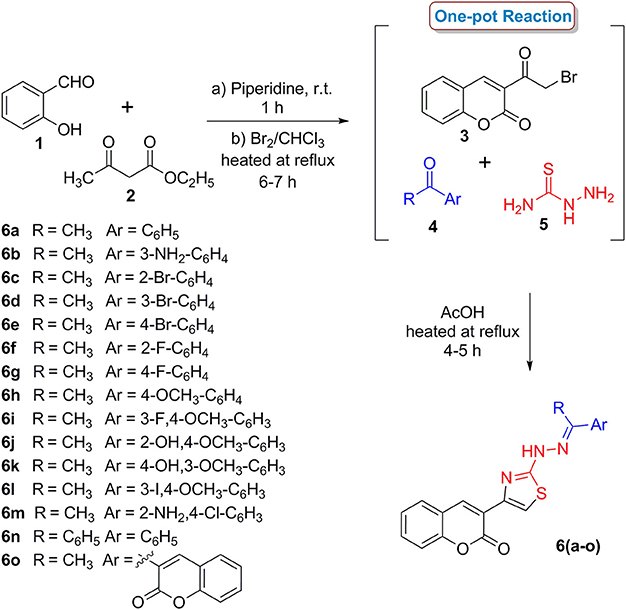
Scheme 1. One-pot multi-component synthetic protocol for the preparation of coumarinyl thiazole derivatives 6(a–o).
In a second series, coumarinyl oxadiazole-2(3H)-thione conjugates 11(a–h), the central intermediate 3-(5-thioxo-4,5-dihydro-1,3,4-oxadiazol-2-yl)-2H-chromen-2-one (8) was prepared by the reaction of coumarinyl hydrazide (7) with carbon disulfide in ethanolic solution of KOH in good yield (Pattan et al., 2009). A one-pot reaction of compound 8, paraformaldehyde (9) and different (aliphatic and aromatic) amines (10) gave the desired compounds 11(a–h) in good yields (Scheme 2). The compounds were characterized by various spectroscopic techniques and full spectro-analytical data is described in our recent report (Ibrar et al., 2016).
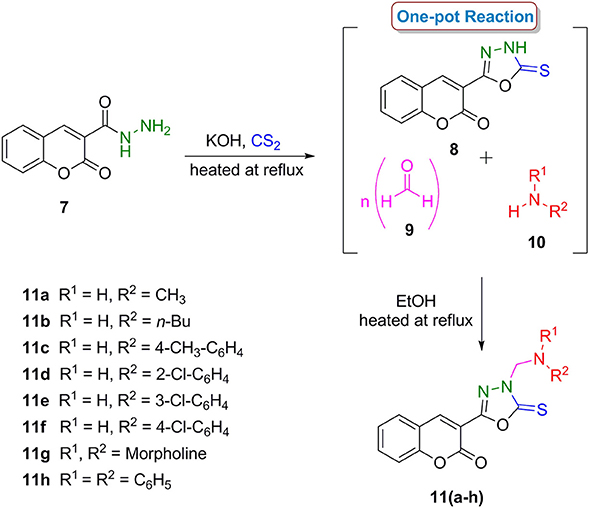
Scheme 2. One-pot multi-component synthetic protocol for the preparation of coumarin-oxadiazole-2(3H)-thione conjugates 11(a–h).
Pharmacology
The target compounds, coumarinyl thiazoles 6(a–o) and coumarinyl oxadiazoles 11(a–h), were screened for their inhibitory activity against AChE and BuChE by Ellman's method. All the assays were carried out at micromolar level using neostigmine and donepezil as standard inhibitors having IC50 values of 28.2 ± 2.01 and 7.23 ± 0.13 μM for AChE, whereas 16.1 ± 1.13 and 0.03 ± 0.003 μM for BuChE, respectively. The results obtained for both series 6(a–o) and 11(a–h) are summarized in Tables 1, 2. The IC50 values revealed that most of the synthesized compounds displayed potent and selective inhibition toward cholinesterases.
Among them, 6b of the coumarinyl thiazole series was found to be the strongest AChE inhibitor with an IC50 value of 0.87 ± 0.09 μM (Table 1, Figure 2). This compound inhibited AChE ~32-fold more strongly than the standard neostigmine, and nine-fold as effective against AChE as the second standard donepezil (IC50 = 7.23 ± 0.12 μM). The strong inhibitory potential of 6b could be credited to the electron-donating amine group present at meta-position of the aryl ring. The introduction of a bromo group at the meta-position produced comparable results (6d; IC50 = 30.06 ± 1.73 μM) to the neostigmine. A slight decrease in the inhibition (IC50 = 1.08 ± 0.84 μM) was observed in case of compound 6h having methoxy group at para-position but the inhibition was still 26-fold stronger than the standard neostigmine (Figure 2). However, compounds 6i and 6k bearing a double substitution at the aryl ring showed IC50 values of 2.34 ± 1.34 and 5.86 ± 0.15 μM, respectively. These compounds incorporate a combination of different electron-donating and electron-withdrawing groups which could potentially lead to increase the several folds in AChE inhibition than the neostigmine and comparable inhibition (in case of 6k) to the donepezil (Figure 2). In the same series (6a–o), a slight decrease in the inhibition was observed in compounds 6j, 6n, 6o, and 6l as compared to the potent analogs, but the inhibition was still stronger compared to neostigmine.
On the other hand, in the coumarinyl oxadiazole series (11a–h), compound 11a was found to be the most potent AChE inhibitor having IC50 value of 6.07 ± 0.23 μM (Table 2, Figure 3). This inhibitory potency might be attributed to an aliphatic methyl group substituted on the amine moiety. A slight decrease in the inhibition was observed when the methyl group was replaced by another aliphatic (n-Bu) group as revealed by compound 11b (IC50 = 9.41 ± 0.55 μM). When these aliphatic groups were replaced by aromatic substitutions as in 11c–f, reduced inhibition was observed (Figure 3).
Moreover, oxadiazole compounds with morpholine substituent (11g) and two phenyl groups (11h) were also found as moderate inhibitors of AChE with two- and three-fold higher inhibition as compared to neostigmine (Figure 4). Overall, among the tested compounds, coumarinyl thiazoles appeared as potent AChE inhibitors than the coumarinyl oxadiazoles.
All the synthesized analogs were also evaluated for butyrylcholinesterase inhibition and several compounds were found to possess potent inhibitory activity higher than the standard neostigmine. Among the coumarinyl thiazoles, compound 6j with dual electron-donating groups was the lead inhibitor with IC50 value of 11.01 ± 3.37 μM. Compounds 6d and 6h with meta-bromo and para-methoxy substituents were also moderate inhibitors of BuChE (Figure 5). The other compounds in the series showed weak inhibition for BuChE.
However, the coumarinyl oxadiazoles were strong inhibitors of BuChE. Compound 11e bearing meta-chloro substituent inhibited the BuChE with an IC50 value of 0.15 ± 0.09 μM. This compound was about 107-fold more potent than neostigmine. The replacement of chloro phenyl with an aliphatic methyl and n-Bu group (11a; IC50 = 0.341 ± 0.06 μM, 11b; IC50 = 0.77 ± 0.08 μM) directed a small decrease in the inhibition but the compounds were still several-folds more active than neostigmine (Figure 6). The other compounds in the series 11c, 11d, 11f, 11h, and 11g revealed significant inhibition more than the reference standard.
In general, among the synthesized analogs, the compounds from coumarinyl thiazole series (6a–o) were excellent AChE inhibitors than the two reference drugs neostigmine and donepezil while coumarinyl oxadiazoles (11a–h) showed strong inhibition for BuChE than the standard neostigmine. All in all, the target analogs proved to be very potent inhibitors of cholinesterase and by considering their strong inhibitory potential, these heterocyclic hybrid compounds hold great potential for the development of new targets for AD therapy.
Molecular Docking Studies
Possible binding modes of coumarinyl thiazole and oxadiazole derivatives were explored by MOE (Molecular Operating Environment) software. Molecular docking studies revealed that the thiazoles having high anti-acetylcholinesterase activity (6b, 6h, 6i, and 6k) represented better interaction with the active site of AChE (4EY7) with MOE score of −10.19, −9.97, −9.68, and −11.03 Kcal.mol−1, respectively, whereas oxadiazoles having high activity against butyrylcholinesterase (11e, 11a, and 11b) represented better interaction with BuChE (4BDS) with MOE score of −9.9, −7.4, and −8.2 Kcal.mol−1, respectively, as compared to reference ligands (neostigmine and donepezil) as shown in Table 3. Compound 6b (IC50 = 0.87 ± 0.09 μM) demonstrated conventional hydrogen bonding with Glu202 (1.48 Å) and Ser203 (1.69 Å) due to amino group of aryl ring and π-π stacking with Trp86, Trp286, and Tyr341 along with water bridging with Tyr337, Tyr124, and Ser125 as shown in Figure 7A. The impact of amino group on the activity of compound was already mentioned earlier in the structure-activity relationship (Figure 2). Compound 6h (IC50 = 1.08 ± 0.84 μM) represented hydrogen bonding with Tyr72 (2.89 Å) and π-π stacking with Trp86, Trp286, and Tyr341 and Phe338 along with water bridging with Tyr72, Tyr124, and Thr83 as shown in Figure 7B. Compound 6i (IC50 = 2.34 ± 1.43 μM) also demonstrated almost similar interactions (as with compound 6h) except the length of hydrogen bond with Tyr72 (2.93 Å). Compound 6k represented hydrogen bond with Tyr124 (2.21 Å), π-π stacking with Trp86 and Tyr341 (not with Trp286) and water bridging with Thr83, Ser125, and Asp74 as shown in Figure 7C. Reference compound (co-crystallized ligand) donepezil was also docked to compare and confirm our docking results where it was observed that it makes only π-π stacking with Trp86 and Trp286 along with water bridging with Tyr337 and Tyr341 as shown in Figure 7D. In agreement to the in-vitro results, molecular docking studies suggested that compounds 6b, 6h, 6i, and 6k represented better interaction than donepezil; (i) due to hydrogen bonding, (ii) due to extra π-π stacking with aromatic residues, and (iii) due to more interaction through water bridging as shown in Figure 7. Active site of the enzyme ribbon model (4EY7) and molecular docking comparison of the most active compound 6b (magenta) with reference ligand donepezil (yellow) was depicted in Figure 8a, whereas hydrophobic surface and active site cavity of the enzyme docked with compound 6b (magenta) was represented in Figure 8b.
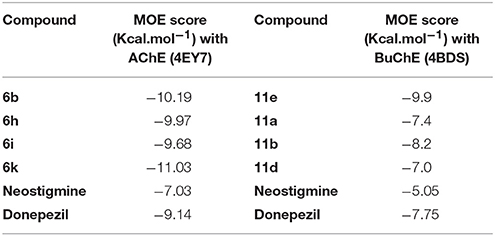
Table 3. MOE score of highly ranked coumarinyl thiazole and oxadiazole derivatives with the active site of AChE and BuChE.
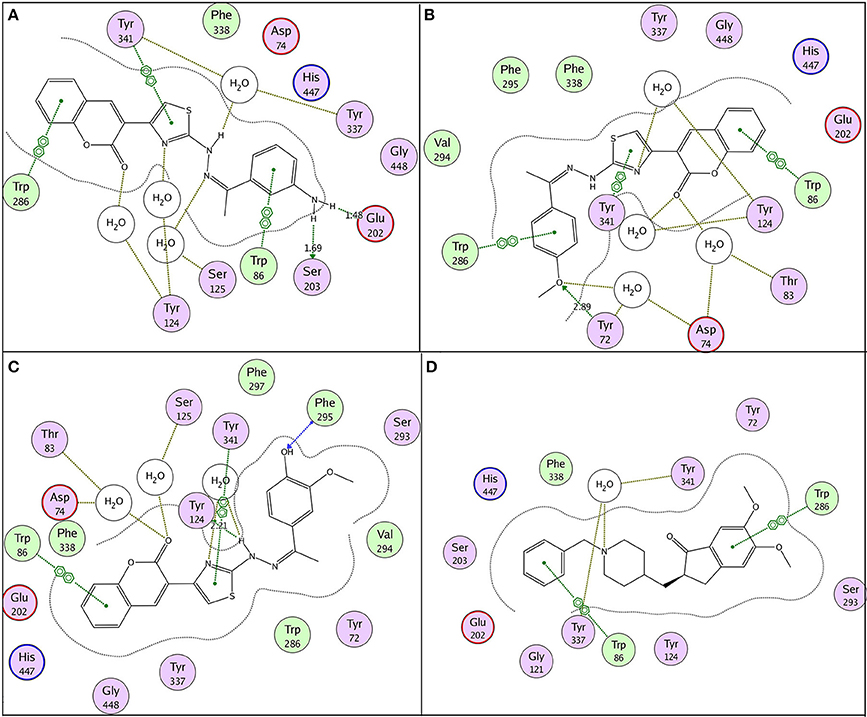
Figure 7. 2D binding pose representation of compound (A) 6b, (B) 6h, (C) 6k, and (D) donepezil with the active site of AChE (4EY7) chain A.
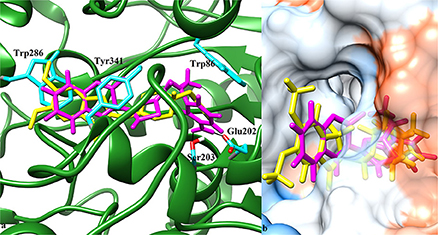
Figure 8. (a) 3D ribbon model of AChE (4EY7) chain A (green) docked with compound 6b (magenta) and co-crystalized ligand donepezil (yellow). Interacting residues shown in ball and stick model (cyan). Black solid lines represent hydrogen bonding. (b) Hydrophobic surface model and active site cavity of the enzyme bound with donepezil (yellow) and compound 6b (magenta).
According to molecular docking results, the highest ranked anti-butyrylcholinesterase compound 11e (IC50 = 0.15 ± 0.09 μM) illustrated π-π stacking with Trp82 and interaction through water bridging with Asp70 and Ser79 as shown in Figure 9A. Compound 11a (IC50 = 0.34 ± 0.06 μM) and 11b (IC50 = 0.77 ± 0.08 μM) represented conventional hydrogen bonding with Glu197 (1.5 Å) and Ser198 (3.0 Å), respectively as shown in Figures 9B,C. However their low affinity binding poses also demonstrated π-π stacking with Trp82 and water bridging. Reference ligand donepezil represented π-π stacking with Trp82 and interaction through water bridging with Asp70, Ser79, Thr120, Ser287, and Pro285 as shown in Figure 9D. Docking comparison of compound 11e (pink) with reference ligand donepezil (yellow) in the active site of BuChE (4BDS) ribbon model and surface model was depicted in Figures 10a,b, respectively.
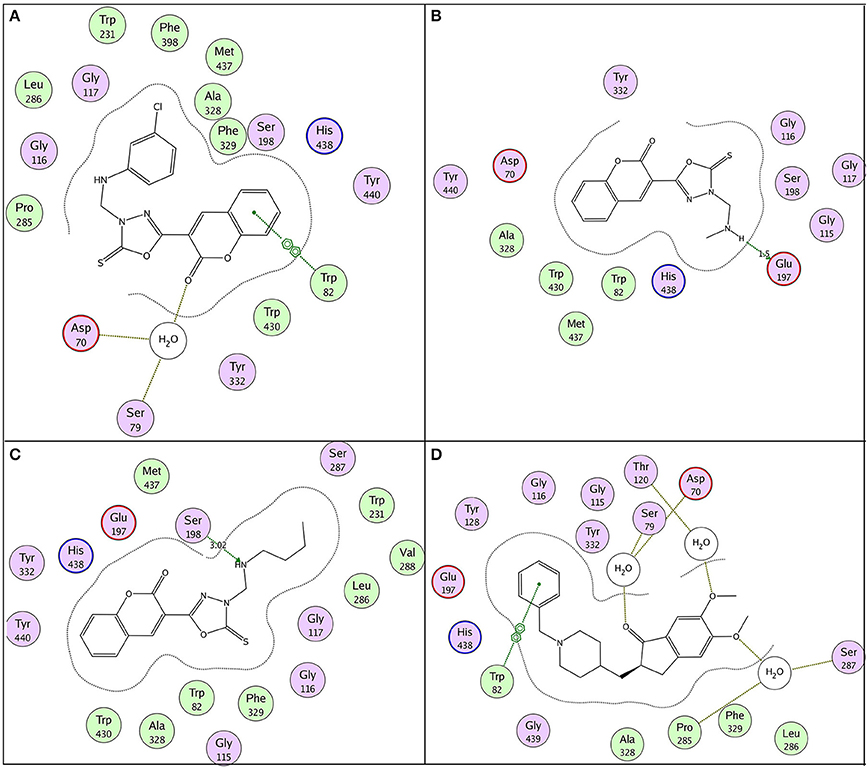
Figure 9. 2D binding pose representation of compound (A) 11e, (B) 11a, (C) 11b, and (D) donepezil with the active site of BuChE (4BDS).
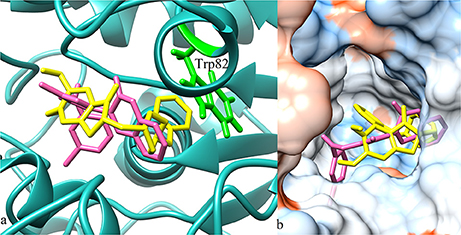
Figure 10. (a) 3D ribbon model (blue) of BuChE (4BDS) docked with compound 11e (pink) and reference ligand donepezil (yellow). (b) Hydrophobic surface and active site cavity of the enzyme bound with donepezil (yellow) and compound 11e (pink).
Pharmacokinetics Prediction
Early prediction of in-silico ADMET properties of lead molecules has now realized as an effective tool in the drug discovery and development process. Therefore, Lipinski's criteria and oral rat LD50 value were estimated for top ranked active compounds by using TEST (Toxicity Estimation Software Tool) and Molinspiration online software. Top five compounds selected from coumarinyl thiazoles and oxadiazole (6b, 6h, 6i, 11a, and 11e) for the analysis and results were summarized in Table 4. Polar surface area (tPSA) values are important for the determination of blood brain barrier (BBB) penetration. According to Waterbeemd the cutoff value is 90 Å2 or less. Almost all the compounds fulfilled the criteria except compound 6b with cutoff value slightly higher i.e., 93. Number of rotatable bonds (nROT) is an additional property that measures the flexibility of the molecule. The drugs that are BBB +ve, usually reported to have fewer nROT bonds. Another extension in RO5 to improve the prediction of drug-likeness is molar refractivity (MR) which should be 40–130. Oral rat LD50 was also predicted for these compounds and the compounds were found slightly toxic according to Hodge and Sterner scale. All the criteria were fulfilled by the compounds and no Lipinski's violation was found as shown in Table 4.
Conclusions
In summary, the present report clearly revealed that the new hybrid molecules show remarkable inhibition of AChE and BuChE enzymes. Compound 6b from coumarinyl thiazole series was emerged as the most potent inhibitor of AChE, whereas 11e from coumarinyl oxadiazole derivatives inhibited the BuChE with highest potency. Both the identified inhibitors follow Lipinski's RO5, slightly toxic and near the range of blood brain barrier crossing. In future, these compounds and their functionalized derivatives may be helpful in the development of potent drugs for Alzheimer's disease.
Experimental
Synthesis of Coumarinyl Thiazole 6(a–o) and Oxadiazole 11(a–h) Derivatives
The coumarinyl thiazole 6(a–o) and oxadiazole 11(a–h) analogs were prepared according to our recently published report (Ibrar et al., 2016).
Pharmacological Protocols
Methodology for Determining AChE and BuChE Inhibitory Activity
For the determination of cholinesterase inhibition, electric eel, and horse serum were used as source of AChE and BuChE, respectively. The Ellman's spectrophotometric method was used to determine the AChE and BuChE inhibitory activity with a slight modification (Ellman et al., 1961). The compounds with 1 Mm concentration were prepared in DMSO. Assay was carried out in 96 well-plate in triplicates. The reaction mixture comprised of 20 μL of buffer (tris HCl 50 mM, 0.02 M MgCl2.6H2O, 0.1 mM NaCl) at pH 8, 10 μL of the test compound, 10 μL enzyme acetylcholine or butyrylcholinesterase of 0.03 U/mL (500 U of AChE and 700 U/mg of BuChE). The contents were incubated for 10 min at 25°C followed by the addition of 1 mM of 10 μL of substrate acetylcholine iodide for AChE and butyrylthiocholine iodide for BuChE and incubated again at 25°C for 15 min. A 50 μL of 3 mM DTNB as a coloring agent was added and incubated at 25°C for further 10 min. The amount of product formed was measured by using micro plate reader (Bio-Tek ELx 800, Instruments Inc., Winooski, VT, USA) at 405 nm. The enzyme dilutions were made by using buffer of pH 8 (tris base 50 mM and having 0.1% BSA). The compounds which depict inhibitory activity more than 50% were further tested by making 9–12 serial dilutions in assay buffer and IC50 values were calculated by graph pad prism.
Molecular Docking
The molecular construction of the compounds was performed using ChemBioDraw Ultra 14 suite (PerkinElmer Inc.) and converted into 3D conformations by ChemBio3D (Mills, 2006). Molecular docking studies of the compounds were carried out using MOE (Molecular Operating Environment) software (ChemicalComputingGroup, 2008). The structures of the compounds were energy minimized using MMFF94x forcefield and gradient: 0.05. Crystal structures of the enzymes, acetylcholinesterase (PDB: 4EY7) and butyrylcholinesterase (PDB: 4BDS) were retrieved from Protein Data Bank (Berman et al., 2006). The co-crystallized ligands in the active site of AChE and BuChE, donepezil (PDB: E20), and Tacrine (PDB: THA) were taken as possible binding site. Ligand neostigmine was taken from PubChem (CID:4456) (Kim et al., 2015). The target proteins were prepared by the addition of hydrogen. All other parameters were used with the default settings. Donepezil and neostigmine were taken as reference ligands for comparison purposes. For each ligand 10 conformations were generated. The images in 2D were captured through MOE ligand binding interaction. 3D images were taken using UCSF Chimera 1.11 software (Pettersen et al., 2004).
Author Contributions
All authors listed have made a substantial, direct and intellectual contribution to the work, and approved it for publication.
Conflict of Interest Statement
The authors declare that the research was conducted in the absence of any commercial or financial relationships that could be construed as a potential conflict of interest.
Acknowledgments
Generous support from Higher Education Commission of Pakistan (project No. 21- 978/SRGP/R&D/HEC/2016) is gratefully acknowledged.
References
Bartolini, M., Bertucci, C., Cavrini, V., and Andrisano, V. (2003). β-Amyloid aggregation induced by human acetylcholinesterase: inhibition studies. Biochem. Pharmacol. 65, 407–416. doi: 10.1016/S0006-2952(02)01514-9
Berman, H. M., Westbrook, J., Feng, Z., Gilliland, G., Bhat, T. N., Weissig, H., et al. (2006). “The protein data bank, 1999–,” in International Tables for Crystallography Volume F: Crystallography of Biological Macromolecules, eds M. G. Rossmann and E. Arnold (Dordrecht: Springer), 675–684. doi: 10.1107/97809553602060000722
Campiglia, P., Scrima, M., Grimaldi, M., Cioffi, G., Bertamino, A., Sala, M., et al. (2009). A new series of 1, 3-Dihidro-Imidazo [1, 5-c] thiazole-5, 7-Dione derivatives: synthesis and interaction with Aβ (25-35) Amyloid Peptide. Chem. Biol. Drug Des. 74, 224–233. doi: 10.1111/j.1747-0285.2009.00853.x
ChemicalComputingGroup, M. (2008). Molecular Operating Environment: Chemical Computing Group Montreal. Quebec, QC.
Chiou, S. Y., Huang, C. F., Hwang, M. T., and Lin, G. (2009). Comparison of active sites of butyrylcholinesterase and acetylcholinesterase based on inhibition by geometric isomers of benzene-di-N-substituted carbamates. J. Biochem. Mol. Toxicol. 23, 303–308. doi: 10.1002/jbt.20286
Cummings, J. L., Doody, R., and Clark, C. (2007). Disease-modifying therapies for Alzheimer disease challenges to early intervention. Neurology 69, 1622–1634. doi: 10.1212/01.wnl.0000295996.54210.69
Cygler, M., Schrag, J. D., Sussman, J. L., Harel, M., Silman, I., Gentry, M. K., et al. (1993). Relationship between sequence conservation and three-dimensional structure in a large family of esterases, lipases, and related proteins. Protein Sci. 2, 366–382. doi: 10.1002/pro.5560020309
de Souza, L. G., Rennó, M. N., and Figueroa-Villar, J. D. (2016). Coumarins as cholinesterase inhibitors: a review. Chem. Biol. Interact. 254, 11–23. doi: 10.1016/j.cbi.2016.05.001
Ellman, G. L., Courtney, K. D., Andres, V. Jr, and Featherstone, R. M. (1961). A new and rapid colorimetric determination of acetylcholinesterase activity. Biochem. Pharmacol. 7, 88–95. doi: 10.1016/0006-2952(61)90145-9
Groner, E., Ashani, Y., Schorer-Apelbaum, D., Sterling, J., Herzig, Y., and Weinstock, M. (2007). The kinetics of inhibition of human acetylcholinesterase and butyrylcholinesterase by two series of novel carbamates. Mol. Pharmacol. 71, 1610–1617. doi: 10.1124/mol.107.033928
Hang, P. C., and Honek, J. F. (2005). Electronic structure calculations on the thiazole-containing antibiotic thiostrepton: molecular mechanics, semi-empirical and ab initio analyses. Bioorg. Med. Chem. Lett. 15, 1471–1474. doi: 10.1016/j.bmcl.2004.12.076
Helal, M. H., Salem, M. A., El-Gaby, M. S., and Aljahdali, M. (2013). Synthesis and biological evaluation of some novel thiazole compounds as potential anti-inflammatory agents. Eur. J. Med. Chem. 65, 517–526. doi: 10.1016/j.ejmech.2013.04.005
Ibrar, A., Tehseen, Y., Khan, I., Hameed, A., Saeed, A., Furtmann, N., et al. (2016). Coumarin-thiazole and-oxadiazole derivatives: synthesis, bioactivity and docking studies for aldose/aldehyde reductase inhibitors. Bioorg. Chem. 68, 177–186. doi: 10.1016/j.bioorg.2016.08.005
Jaishree, V., Ramdas, N., Sachin, J., and Ramesh, B. (2012). In vitro antioxidant properties of new thiazole derivatives. J. Saudi Chem. Soc. 16, 371–376. doi: 10.1016/j.jscs.2011.02.007
Kashman, Y., Gustafson, K. R., Fuller, R. W., Cardellina, J. H., McMahon, J. B., Currens, M. J., et al. (1992). HIV inhibitory natural products. Part 7. The calanolides, a novel HIV-inhibitory class of coumarin derivatives from the tropical rainforest tree, Calophyllum lanigerum. J. Med. Chem. 35, 2735–2743.
Kim, S., Thiessen, P. A., Bolton, E. E., Chen, J., Fu, G., Gindulyte, A., et al. (2015). PubChem substance and compound databases. Nucleic Acids Res. 44, D1202–D1213. doi: 10.1093/nar/gkv951
Klimesová, V., Zahajská, L., Waisser, K., Kaustová, J., and Möllmann, U. (2004). Synthesis and antimycobacterial activity of 1, 2, 4-triazole 3-benzylsulfanyl derivatives. Il Farmaco. 59, 279–288. doi: 10.1016/j.farmac.2004.01.006
Maddi, V., Mamledesai, S., Satyanarayana, D., and Swamy, S. (2007). Synthesis and antiinflammatory activity of substituted (2-oxochromen-3-yl) benzamides. Indian J. Pharm. Sci. 69, 847–849. doi: 10.4103/0250-474X.39452
Manvar, A., Bavishi, A., Radadiya, A., Patel, J., Vora, V., Dodia, N., et al. (2011). Diversity oriented design of various hydrazides and their in vitro evaluation against Mycobacterium tuberculosis H37Rv strains. Bioorg. Med. Chem. Lett. 21, 4728–4731. doi: 10.1016/j.bmcl.2011.06.074
Martin-Aragón, S., de las Heras, B., Sanchez-Reus, M. I., and Benedi, J. (2001). Pharmacological modification of endogenous antioxidant enzymes by ursolic acid on tetrachloride-induced liver damagein rats and primary cultures of rat hepatocytes. Exp. Toxicol. Pathol. 53, 199–206. doi: 10.1078/0940-2993-00185
Mesulam, M. M., Guillozet, A., Shaw, P., Levey, A., Duysen, E. G., and Lockridge, O. (2002). Acetylcholinesterase knockouts establish central cholinergic pathways and can use butyrylcholinesterase to hydrolyze acetylcholine. Neuroscience 110, 627–639. doi: 10.1016/S0306-4522(01)00613-3
Mills, N. (2006). ChemDraw Ultra 10.0 CambridgeSoft, 100 CambridgePark Drive, Cambridge, MA: Commercial Price: 1910fordownload, 2150 for CD-ROM; Academic Price: 710fordownload, 800 for CD-ROM: ACS Publications. Available online at: www.cambridgesoft.com
Naveena, C. S., Poojary, B., Arulmoli, T., Manjunatha, K., Prabhu, A., and Kumari, N. S. (2013). Synthesis and evaluation of biological and nonlinear optical properties of some novel 2, 4-disubstituted [1, 3]-thiazoles carrying 2-(aryloxymethyl)-phenyl moiety. Med. Chem. Res. 22, 1925–1937. doi: 10.1007/s00044-012-0195-7
Olmedo, D., Sancho, R., Bedoya, L. M., López-Pérez, J. L., Del Olmo, E., Muñoz, E., et al. (2012). 3-Phenylcoumarins as inhibitors of HIV-1 replication. Molecules 17, 9245–9257. doi: 10.3390/molecules17089245
Pattan, S. R., Rabara, P., Pattan, J. S., Bukitagar, A., Wakale, V., and Musmade, D. (2009). Synthesis and evaluation of some novel substituted 1, 3, 4-oxadiazole and pyrazole derivatives for antitubercular activity. Indian J. Chem. Sec. B 48, 1453–1456. Available online at: http://nopr.niscair.res.in/handle/123456789/6111
Pettersen, E. F., Goddard, T. D., Huang, C. C., Couch, G. S., Greenblatt, D. M., Meng, E. C., et al. (2004). UCSF Chimera—a visualization system for exploratory research and analysis. J. Comput. Chem. 25, 1605–1612. doi: 10.1002/jcc.20084
Romagnoli, R., Baraldi, P. G., Salvador, M. K., Camacho, M. E., Preti, D., Tabrizi, M. A., et al. (2012). Synthesis and biological evaluation of 2-substituted-4-(3′, 4′, 5′-trimethoxyphenyl)-5-aryl thiazoles as anticancer agents. Bioorg. Med. Chem. 20, 7083–7094. doi: 10.1016/j.bmc.2012.10.001
Ronad, P. M., Noolvi, M. N., Sapkal, S., Dharbhamulla, S., and Maddi, V. S. (2010). Synthesis and antimicrobial activity of 7-(2-substituted phenylthiazolidinyl)-benzopyran-2-one derivatives. Eur. J. Med. Chem. 45, 85–89. doi: 10.1016/j.ejmech.2009.09.028
Shaker, R. M. (2006). The chemistry of mercapto-and thione-substituted 1, 2, 4-triazoles and their utility in heterocyclic synthesis. ARKIVOC 9, 59–112. doi: 10.3998/ark.5550190.0007.904
Siddiqui, N., Arshad, M. F., and Khan, S. A. (2009). Synthesis of some new coumarin incorporated thiazolyl semicarbazones as anticonvulsants. Acta Pol. Pharm. Drug Res. 66, 161–167.
Terry, A. V., and Buccafusco, J. (2003). The cholinergic hypothesis of age and Alzheimer's disease-related cognitive deficits: recent challenges and their implications for novel drug development. J. Pharmacol. Exp. Ther. 306, 821–827. doi: 10.1124/jpet.102.041616
Venugopala, K. N., Krishnappa, M., Nayak, S. K., Subrahmanya, B. K., Vaderapura, J. P., Chalannavar, R. K., et al. (2013). Synthesis and antimosquito propertiesof 2, 6-substituted benzo [d] thiazole and 2, 4-substituted benzo [d] thiazole analogues against Anopheles arabiensis. Eur. J. Med. Chem. 65, 295–303. doi: 10.1016/j.ejmech.2013.04.061
Keywords: coumarin thiazoles, coumarin oxadiazoles, cholinesterase inhibition, molecular docking, MOE score
Citation: Ibrar A, Khan A, Ali M, Sarwar R, Mehsud S, Farooq U, Halimi SMA, Khan I and Al-Harrasi A (2018) Combined in Vitro and in Silico Studies for the Anticholinesterase Activity and Pharmacokinetics of Coumarinyl Thiazoles and Oxadiazoles. Front. Chem. 6:61. doi: 10.3389/fchem.2018.00061
Received: 03 December 2017; Accepted: 26 February 2018;
Published: 26 March 2018.
Edited by:
Daniela Schuster, Paracelsus Private Medical University of Salzburg, AustriaReviewed by:
Sobia Ahsan Halim, Kinnaird College for Women University, PakistanSuresh Reddy Chidipudi, Spiro Organics Private Limited, India
Copyright © 2018 Ibrar, Khan, Ali, Sarwar, Mehsud, Farooq, Halimi, Khan and Al-Harrasi. This is an open-access article distributed under the terms of the Creative Commons Attribution License (CC BY). The use, distribution or reproduction in other forums is permitted, provided the original author(s) and the copyright owner are credited and that the original publication in this journal is cited, in accordance with accepted academic practice. No use, distribution or reproduction is permitted which does not comply with these terms.
*Correspondence: Ajmal Khan, ajmalchemist@yahoo.com
Imtiaz Khan, kimtiaz@hotmail.co.uk
Ahmed Al-Harrasi, aharrasi@unizwa.edu.om