- Instituto de Física “Gleb Wataghin” (IFGW), Universidade Estadual de Campinas (UNICAMP), Campinas, SP, Brazil
The urgent need to address the global sustainability issues that modern society is currently facing requires the development of micro and nanotechnologies, which rely largely on functional materials. Beyond studies focused solely on low-dimensional materials, broader research related to multifunctionality has shown that the major efforts to meet these criteria for new electronic, photonic, and optoelectronic concepts, particularly to achieve high-performance devices, are still challenging. By exploiting their unique properties, a comprehensive understanding of the implications of research for the synthesis and discovery of novel materials is obtained. The present article encompasses innovation research as an alternative optimization and design for sustainable energy development, bridging the scaling gap in atomically controlled growth in terms of surface heterogeneity and interfacial engineering. In addition, the corresponding research topics are widely regarded as a scientometric analysis and visualization for the evaluation of scientific contributions into the early 20 years of the 21st century. In this perspective, a brief overview of the global trends and current challenges toward high-throughput fabrication followed by a scenario-based future for hybrid integration and emerging structural standards of scalable control design and growth profiles are emphasized. Finally, these opportunities are unprecedented to overcome current limitations, creating numerous combinations and triggering new functionalities and unparalleled properties for disruptive innovations of Frontier technologies.
Introduction
This perspective describes completely cointegrated structures having a physical scale appropriate for use with the capability to tune interface compatibility engineering. It is an emerging interdisciplinary field that deals with the different ways of manipulating matter at the nanoscale. In turn, the conceptualization needed to correctly architect these materials to feasibleness (at least in its major applications in analysis) remains seriously lacking. The main purpose is to identify hybrid integration strategies and open issues, outlining approaches to the meticulous control design and future potential toward the combinatorial synthesis and characterization of self-assembled smart materials.
Emerging research domains in nanoscience and nanotechnology facing smart surfaces, devices, and systems demand a material combining selective assembly, structural dimensionality, stability, and a wide range of properties compatible with different key attributes toward sustainable energy development (Reyes-Belmonte, 2021). Suitable applications related to the differences in fundamental and functional properties (physical/electrochemical) for renewable energy harvesting and storage systems (Lau et al., 2019)—such as (opto)electronic memristors, supercapacitors, nanogenerators, batteries, and sensors—are adaptative strategies of special interest by the pressing need to fight global warming and energy shortages. This approach requires the development of advanced functional materials and complex systems (Kilner et al., 2012; Chung, 2021), which extend from conventional three-dimensional (3D) bulk materials beyond vertical stacking and lateral stitching of two-dimensional (2D) few-layered materials and assembling one- and zero-dimensional (1D and 0D) structures to large-area integration of nonconventional multijunction configurations and new metamaterials, having in mind the relevance of hybrid energy harvesters and the changes in terms of characteristic dimensions and strong correlation effects such as lattice mismatch, lightweight, carrier mobility dynamics, bandgap energies, and response to external stimuli for individually or simultaneously self-powered systems combining multiple sources (Bai et al., 2018).
Recent achievements in the sense of substantial amounts of energy collected by applying functionalized materials and metamaterials from the surrounding environment or intentionally supplied sources (external excitation) are used in various energy-harvesting modalities (Phillips, 2021), and the key benefits of boosting include 1) hybrid photovoltaic-thermal cells for 24 h/day power generation (as a continuous energy flow) at a density of 10–100 mW/m2 nighttime via a radiative cooling-based thermoelectric generator (Assawaworrarit et al., 2022); 2) spatial light (electro- and thermal-optical) sensing and modulators based on monolithic organic chromophores (JRD1) (Benea-Chelmus et al., 2021), oxide semiconductors, and 2D materials for digital or analog signal processing at terahertz (THz) frequencies (Yang et al., 2022); 3) different module configurations for single and multijunctions (or tandem solar cells) with complementary bandgaps and absorption edges (Martinho, 2021; Yamaguchi et al., 2021); 4) flexible and hybrid photovoltaic-piezo-triboelectric nanogenerators that efficiently convert harvested sunlight and mechanical energy into electricity (Chen et al., 2020; Wu et al., 2021); and 5) nanosized resonators and broadband metamaterial absorbers with embedded high electron mobility transistors and reconfigurable design operating at frequencies ranging from a few kHz to hundreds of THz (Yang et al., 2019; Yu et al., 2019).
These examples of enhanced photo-thermo-electroresponsive applications represent the continuous advancements in condensed matter physics, materials science, and engineering. Knowledge of the use of hybrid integration opens new opportunities for combining experiments of bottom-up assembly and top-down patterning (Isaacoff and Brown, 2017; Morales et al., 2018; Echeverrigaray et al., 2022) or additive manufacturing (or 3D printing) (Wang et al., 2021) using distinct types of materials, depicting the biggest trends driving the future. More explicitly, the complementary nature of the properties inherent in diversified characteristic couplings in materials with versatile dimensionality has demonstrated exceptional potential for fabricating multifunctional and high-performance devices. The miniaturization and atomic-scale structural modifications (e.g., functionalized hybridization and allotropes) (Guan and Han, 2019; Jeong et al., 2022) can establish given streamlines for advanced material innovation. For instance, graphene is a 2D building material involving all graphitic forms of other dimensionalities, such as representative designs that can be wrapped up into 0D buckyballs, rolled into 1D nanotubes, or stacked into 3D graphite (Wan et al., 2012). The definition of each spatial dimension will be given in the following section. The burgeoning research into multifunctional designs by harnessing the properties of remarkable materials has paved the way for energy-responsive devices in modern technologies as a driver of green transition. This multifunctionality can be integrated into different dimensional scales, with increasing interconnectivity between phase boundaries and engineering difficulty that takes place on small scales.
The effectiveness of up- and downscaling methods refers to changing chemical entities, hierarchical scales, and mesoscopic effects, which leads to stretching limits of existing materials or designing and developing materials with new functions and capabilities for specific applications and performance requirements regarding the sustainable processes and strategic use of raw materials. Almost all materials that have been employed until now support only a limited set of responses to external factors and are characterized by their inability to adapt to environmental changes in real time (Salonitis et al., 2010). To overcome the physical limitations imposed by natural (biotic, fiber, organic, and inorganic) materials, typical engineering materials, and discrete unifunctional (single purpose) subsystems, the integration of these emerging materials exhibits unprecedented synergetic properties and behaviors. However, there are still many processing challenges and constraints of multisource energy harvesters (MEHs) (Xie et al., 2022), and other integrated devices and systems regarding the viability (losses and costly fabrication), reproducibility, scale-up, and assembly into several highly ordered structures (Maggini and Ferreira, 2021)—driving a relentless quest for efficiency and optimization.
The introduction aforementioned serves to provide an overview of the key background necessary for subsequent discussions. Scientometric indicators from two established databases (Web of Science from Clarivate Analytics and Scopus from Elsevier) are also among the topics of this discussion. This perspective aims to explore the knowledge structure; analyze the current research hot spots; and discuss the development status, challenges, and potentials of advanced materials based on function-driven integrating multidimensional scaling, hybrid, and hierarchical interface architectures for quantum information processing, and sustainable energy technology and innovative solutions in times of crisis.
Research status: Scientometric analysis
The growth pattern of the literature survey on advanced functional materials indexed in the Web of Science (WoS) and Scopus database is depicted in Figure 1A. With this scientometrics, a contemporary quantitative study on scientific and technological activities allows us to predict the main trends and gaps of studies based on “multidimensional materials” or “multifunctional properties” or “hybrid interfaces” available from WoS and Scopus during 2002–2022 (20 years), before and after the beginning of the coronavirus (COVID-19) pandemic worldwide. This highlights the exceptional case which does not significantly affect the continuous growth of publications. The dynamics of WoS- and Scopus-related studies (articles, proceedings, and reviews) have been used to retrieve relevant data. A total of 60,940 and 69,277 research documents were published with an average of 1,016 and 1,155 articles per year (all subjects aforementioned) in WoS and Scopus, respectively. The dominant document types are articles, which account for 49,101 (78.8%) of the total publications in all subjects, followed by 8,269 (13.3%) proceedings and 4,946 (7.9%) reviews. In addition, the research status of these key materials and added publications has been an increasingly attractive trend for investigations in inter- and multidisciplinary fields. According to data analysis (Figure 1B), the variability and totality of multipurpose applications involving aforementioned all materials corresponding from the beginning of the modern science system until today are concerned with improved efficiency (e.g., electro- and photoactive devices and components) for emerging strategic industries, mainly, in computer science (artificial intelligence and information systems), telecommunications, renewable energy, and environmental sustainability.
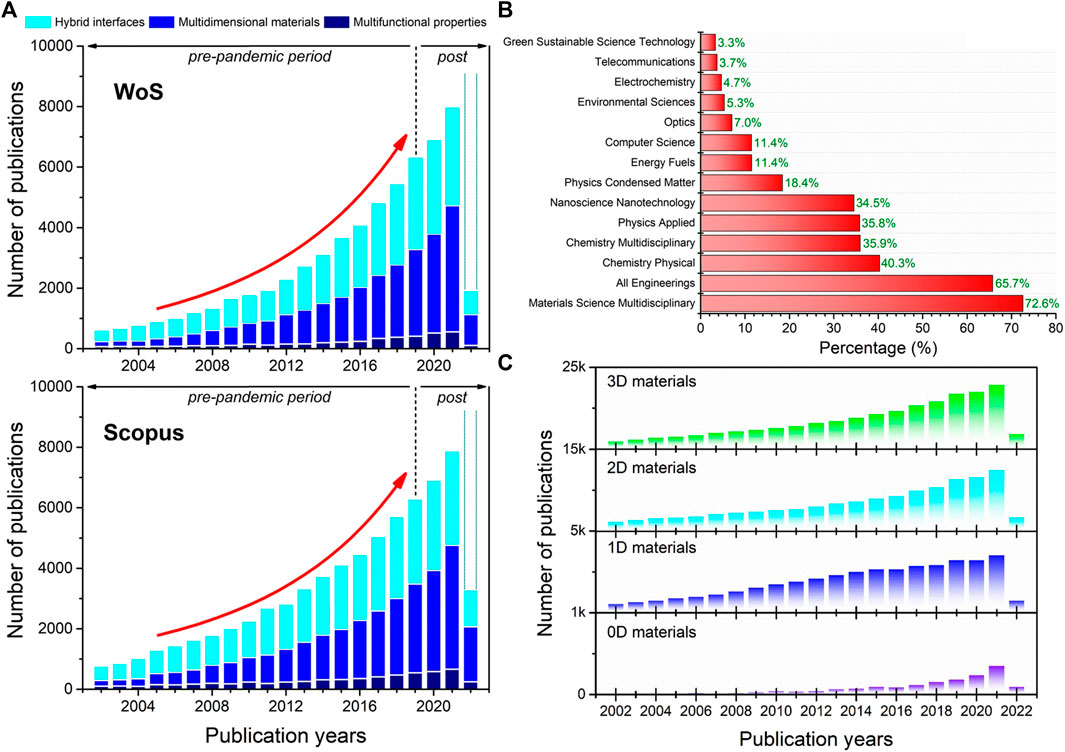
FIGURE 1. (A) Total number of annual publications (2002–2022) on the current progress of materials science and technology. It should be noted that only articles, proceedings, research studies, and reviews are considered. Source: ISI WoS (Institute for Scientific Information, Web of Science) and Scopus database (search: topic = “hybrid interfaces/structures” or “multidimensional materials” or “multifunctional properties”). The dotted lines represent the estimated proportion of the continuous uninterrupted stream of publications in progress. (B) The horizontal bar plot shows documents by subject area, indicating the sum of the analyzed survey results for percentage of 60.999 records of the research topics aforementioned (accessed May 2022). (C) Growth analysis of scientific publications per year for 0D, 1D, 2D, and 3D structure materials, and a total of well over 40,000 are expected by the end of 2022. Published documents on these prior to 2000 are not plotted.
Figure 1C shows the total number of annual publications in WoS on different dimensional materials over a 20-year period. The classification is based on the number of nanoscopic dimensions (< 100 nm range) of a material, which adds to the wide variety of phenomena that can be explored in nanoscience and technology. As observed in the graphic elements, there is an gradual increase in the number of publications from 12,158 (3.6%) in 2010 to 30,941 (9.1%) in 2020, around 8,103 (2.4%) in the middle of 2022. Another comparative analysis indicates that an increase of approximately 77.7% in the number of publications for all dimensional units is simply the corresponding difference between 32,469 records in the period of the first 5 years (2002–2006) and 145,566 in the last 5 years (2017–2021). Using structural materials of topic nD (n = 0, 1, 2, and 3), the total publications corresponded to 272,201 (80.0%) articles, 55,749 (16.0%) proceedings, and 12,209 (4.0%) reviews. It is seen from Table 1 that a total number of 340,159 documents have been indexed in the WoS entity during 2002–2022. After seeing year-wise productivity comparisons, it is concluded that research on materials innovation with different spatial dimensions has received increasing attention. Specifically, these dynamics in the current scenario represent a growing collaborative development among many universities, research institutes, and industrial companies, creating mandatory multifaceted teams with different subject-specific experts such as scientists, engineers, technicians, and environmentalists to obtain benefits from novel analytical concepts and understand the threats involved in the maximum sustainable yield due to monitoring and controlling social expansion, energy consumption, environmental degradation (air pollutants and biohazard waste) and other concerns that are still far from ideal for conserving and caring for our planet.
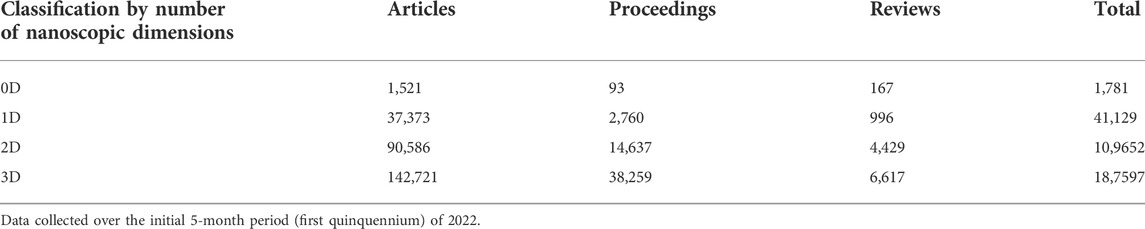
TABLE 1. Total number of publications in WoS during 2002–2022 in the subject nD structure materials (n = 0, 1, 2, and 3).
Global market drivers, ongoing challenges, and needs
The importance of advanced functional materials cannot be underestimated in the radically changing modern society, and problems related to energy efficiency and environmental safety have become integrated material design issues. These drivers of change (economic, technological, societal, and environmental) are intrinsically linked and large-scale disruption or crises can affect multiple factors at once, which is consistent with ISO Strategy 2030 International Organization for Standardization, 2022 (https://www.iso.org). A reliance on the development of semiconductor devices and their contributions that resonate as an integral part of supporting everyday life in our current society based on information and communication technologies with digital infrastructures has largely emerged and transformed into a global market in excess of US$ 556 billion in 2021, according to World Semiconductor Trade Statistics (WSTS) World Semiconductor Trade Statistics, 2022. This represents an increase of 26.2% from 2020, and the forecast is to exceed US$ 578 billion (10.4%) in 2022. A recent market report ReportLinker, 2022 (https://www.reportlinker.com) predicted that the current global market scenario for advanced functional materials is expected to generate an incremental growth of US$ 48 billion and a compound annual growth rate (CAGR) of around 9.0% with accelerating momentum during the forecast period (2022–2026) due to the cumulative impact of COVID-19. In addition, one of the major factors driving the market studied is the growing need for miniaturization and new coupling technologies, as well as advances in the energy, chemical, and healthcare industry. Increasing usage of integrated functional materials in microelectronics is the prevailing trend observed globally and, in the short term, plays a crucial role in technological problem solving as the market evolves. However, many challenges remain for processing and characterization in terms of the deployment of these strategies in technology fields.
The state-of-the-art research today is largely engaged in sustainable development under the concept of integrated architecture design for different kinds of materials that rely on their unique and specific properties. As previously mentioned, the advent of functional materials using various manufacturing processes (e.g., layer-by-layer, exfoliation, electrodeposition, atomic layer deposition, molecular beam epitaxy, and photolithography) prompts a comprehensive range of possibilities as a result of the relationship between cost-effectiveness analysis and involved mechanisms for the substantial differences that lend themselves to scaling challenges. Among them, one reason leading to an undesired behavior or a lack of expected functionality is dependency based on the system and processing conditions. These production thresholds have required changes in high-performance device design that almost fully utilize the benefits offered by atomically thin layers or nanoengineered materials.
Figure 2A schematically represents the multiscale fabrication of advanced functional materials with cointegration structures and hybrid boundaries, originating from different types of interfacial engineering. These possible interfaces and tailored physical properties directly depend on the synthesis conditions, precisely controlling the composition, size, shape, and singularities of the crystallographic structure (ordering), among others. Likewise, multifunctionality needs to be understood as a combining of materials, prescribed forms, and appropriate processes, in addition to the employment of new biomimicry-based strategies (nature-inspired materials), which enable the emulation of a certain mechanism such as artificial photosynthesis. Due to quantum mechanics, the electronic and optical properties (e.g., charge transport and bandgap energy) of low-dimensional materials (LDMs), in turn, are strongly coupled and differentiated from those of macroscopic systems. The elucidation of functional materials will be necessary to rule out their relative effects and mechanisms in an extensive actuation range based on different structural length scales, which provides one other research direction for the property of correlated quantum confinement, a more intrinsic attribute than one that is variable due to the atomic thinning effect that may occur with bulk materials (Franklin, 2015). Essentially, high-quality designs can be obtained by reasonably selecting and operating existing manufacturing technologies (bottom-up and top-down), for example, hierarchical assembly of heterojunctions based on 2D material (Chen et al., 2017; Khan, et al., 2020), such as stacking and stitching (epitaxial growth) or vertically aligned layers.
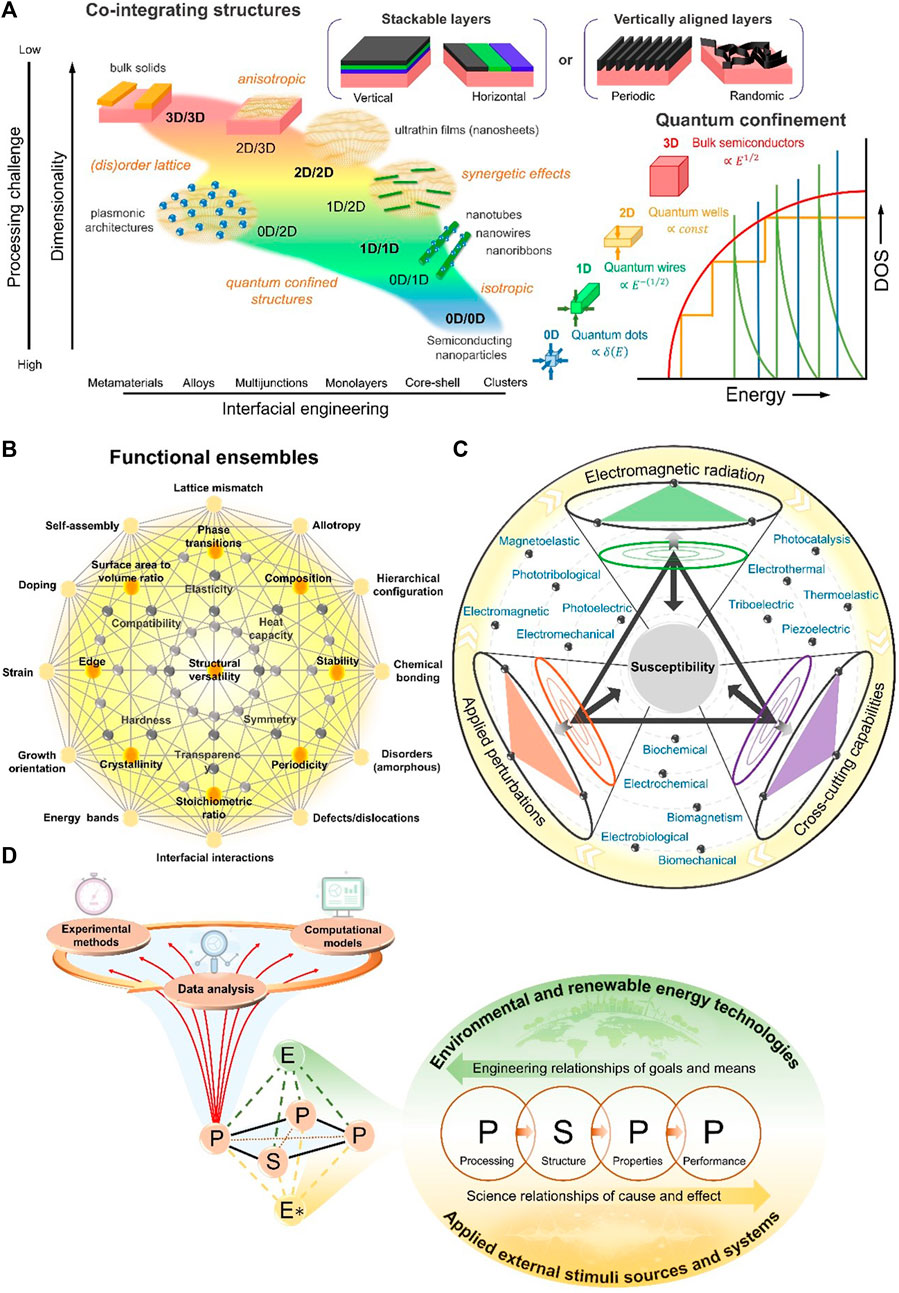
FIGURE 2. (A) Schematic overview of the proposed design guidelines on the co-integration structures (or combinatorial synthesis) in several dimensionalities and hybrid boundaries, originating from different types of interfacial engineering. Referring to quantum-confined structures, the differences in density of states (DOS) in terms of energy appear when the size and shape of nanocrystals are reduced to less than 100 nm. It should be noted that the spatial confinement of electron–hole pairs (excitons) occurs in one or more dimensions (de Broglie wavelength), resulting in more discrete electronic energy levels compared to the bulk materials with continuous behavior. Interrelationship diagrams of some intrinsic physicochemical characteristics kindred with function-driven engineering (B) and their susceptibilities (C) show the activity recognition by energy harvesting and storage derived from external sources and chemical/biological environments, which trigger a series of (non)systemic functional responses possible in smart materials. (D) Processing–structure–properties–performance (PSPP) relationship is represented by a material octahedron scheme with ends corresponding to two external factors.
Within the implications and remaining questions, the key processing challenges should be addressed by the following exploratory research topics: 1) modeling or behavior-prediction techniques that specifically identify the synthesizability of amorphous/crystalline materials and the complexity of interfaces in engineering systems using ab initio methods (Aykol et al., 2018; Davariashtiyani et al., 2021), 2) delimited pathways to synthesize and pattern such materials across multiple length scales required for functional device prototypes in outside-the-lab scenarios, 3) fabrication techniques customizable to the miniaturization and integration of LDMs needed to connect or ensure the designed multifunctional properties (particularly when using modified or novel manufacturing methods), 4) develop new and bespoke assembly methods of single- and multiphase materials with novel structural designs (via controlling shape, size, and orientation), paying more attention to meet a purpose or particular transformational application requirements, 5) new metamaterials (i.e., materials with properties not found in nature) (Zheng et al., 2016; McClintock et al., 2021) that offer the potential for manufacturing scalability and/or improved performance, 6) bioinspired hierarchical (Wang et al., 2020) and hybrid structures (Xie et al., 2021) prompt by additive manufacturing (“printable” functional materials), and 7) deciphered functionality using surface analytical tools, aiming at the interfacial recognition of the transition of chemical bonding character and identification of the physical interactions between two or more layers, which exhibit good adherence and compatibility. Without a doubt, the biggest challenges relate to the interfaces of the nanomaterials for controlled synthesis and configuration. Managing these implicit issues, placements, kinds of knowledge required in assembly strategies, and discovery of the cross-cutting concerns applicable in approaching fundamental limits seem possible.
Functional materials can be generically referred to as suitable interface modifiers for scientific and engineering solutions in energy technologies. Such materials include carbon-based compounds (graphene and diamond-graphite hybrids), inorganic compounds (metals and alloys, semiconductors, and ceramics), organic compounds (soft matter and polymers), and composites (inorganic–organic hybrids). Compared to bulk materials, low-dimensional (0D, 1D, and 2D) structures of sub-10 nm dimensions are pursued to achieve high efficiency and are easily affected by subtle factors. Among all of the classes of materials detailed, it is possible to divide them into four categories based on the state of dimensionality as listed below.
Zero-dimensional (0D) materials: they can tightly confine electrons or holes in all spatial dimensions–length, width, and height (i.e., no coordinate x-y-z-axis larger than 100 nm). The most common examples are atoms, small molecules, nanoclusters, nanocages (fullerenes), and colloidal semiconducting nanocrystals (CNs) or quantum dots (QDs) (García de Arquer et al., 2021). These organic–inorganic nanoparticles can be classified into different types according to their size, morphology, and physical and chemical properties.
One-dimensional (1D) materials: these refer to the confinement of electronic motion in two dimensions (x-y, x-z, or y-z-axes), whose lateral edges fall anywhere in the range of 1–100 nm. The morphologies, compositions, and structures cover a large variety including wires, tubes, rods, fibers, ribbons of metals, semimetals (metalloids), oxides (e.g., TiO2, VO2, MnO2, and MoO3), sulfides (ZnS and HfS2), halides (PbI2 and MgBr2), polyelectrolytes, and coordination polymers (CPs).
Two-dimensional (2D) materials: these refer to electrons confined in one dimension (x, y, or z) and allowed to move in any two directions (outside the nanoscale) in the system. 2D materials refer to crystalline solids–insulators, semiconductors, halfmetals, semimetals, metals, and superconductors–consisting of single- or few-layer atoms, in which the in-plane interatomic interactions are much stronger than those along the stacking direction. These 2D nanostructures (referred to as quantum wells) are an emerging class of materials (Zeng et al., 2018), including graphene and their derivates (Geim and Novoselov, 2007; Dideikin and Vul', 2019), black phosphorus (BPs) (Liang et al., 2014), transition metal dichalcogenides (TMDs, denoted as MX2 and M2X3) (Chowdhury et al., 2020), transition metal oxides (TMOs) (Guo et al., 2015), transition metal carbides/carbonitrides (MXenes) (Huang and Mochalin, 2020), semimetal chalcogenides (SMCs), hexagonal boron nitride (h-BN), perovskites (ABX3) (Miró et al., 2014), and other variations such as layered double hydroxides (LDHs) (Laipan et al., 2020) and TMD ordered alloys (e.g., MoS2xSe2-2x, MoxW1-xSe2, and WS2xSe2-2x) (Pandey et al., 2016).
Three-dimensional (3D) materials: correspond to those that are not confined to the nanoscale in any dimension. This includes bulk materials, compacted/densified powders, alloys, coatings, multilayers, particle suspensions, nanostructure bundles, and dendrimers.
A successful process integration scheme allows device structures suitable for multiple functionalities to scale-up or -down the system dimensions. The functional activities governing small-scale energy conversion systems denote a lower Schottky barrier, intriguing electronic coupling, intimate charge transfer, and efficient separation of excitons (electron–hole pairs) occurring at the atomically sharp interface, suggesting promising applications. Miniaturized and hybrid single devices have been increasingly attractive for investigations in both classical and quantum domains due to the interplay between one or more degrees of freedom coupling optical, electrical, and mechanical modes in nanoscales (Midolo et al., 2018; Zhang T. et al., 2022). Within this context, the emergent phenomena and functions remain a formidable challenge in the form of collective behaviors that arise from physical interactions among the lattice elements (8-polytope projection) and perturbations (strain, edge, magnetic, photo, electric, and thermal) as shown in Figures 2B, C, respectively. For instance, a large lattice mismatch between different materials results in structural defects and lattice distortion. This reflects the interdependence of the new phenomenon and the complex structure–function interplay in materials. Consequently, the intrinsic and extrinsic contributions of a material with respect to its environmental properties are largely dependent on the surface chemistry, morphology, and interface properties. On the other hand, material susceptibility occurs as stimuli-responsive changes for external energy sources, such as mechanical stress–strain deformation (piezoelectric and elastoplastic effect), acoustic waves (mechanical vibration), magnetic field fluctuations (spin–orbit effect), thermal gradient (thermomechanical vibration), electromagnetic waves (photocatalytic activity by light absorption in frequencies/wavelength ranges), and radioactive isotopes (betavoltaic effect). The progression toward continuous scaling and property tuning is extremely important when considering a variety of distinctive behaviors involving more distributed energy harvesting and conversion, for example, embedding QDs on sidewalls of self-catalyzed thin nanowires (Zhang Y. et al., 2022). Accordingly, the benefits of multifunctional materials may further open opportunities for energy-efficient quantum computing and sensing within integrated circuit systems in information and communication technologies.
Future directions in sustainable energy and quantum technologies
With the integration of various materials and dimensions being hybridized, entirely functional on-chip devices would become more readily available to researchers and technology developers, becoming a potential candidate for next-generation energy industries. This configuration unlocking and fine-tuning of single and multicomponents refers to the possible associated manufacturing methods for highly optimized devices and systems from lab-scale research to commercialization. A critical overview of the processing–structure–properties–performance (PSPP) relationship of complex and advanced materials by applying multiparty system technologies with an eco-wakening approach is schematically shown in Figure 2D by exploring functional ensembles with differing access to external stimuli, whether these are mediated by renewable energy resources (i.e., alternatives to fossil fuels) that produce little-to-no emissions or by intentionally applied agents, forces, or fields under precisely controlled conditions. The material paradigm framework provides a holistic perspective on materials science and engineering breakthroughs for gathering information and deploying sustainable technologies. According to the current knowledge, combinatorial synthesis and high-throughput characterization in tandem with computational models (www.materialsproject.org) have proven to be a powerful approach to identifying new and unexpected materials, enforcing the sophisticated integration for multipurpose large-scale applications.
Examples of securing future energy requirements for transboundary solutions in sustainable and quantum technologies include, for instance, noninvasive smaller and more integrated systems with lightweight and less energy consumption that may be distributed and wireless communication. LDMs systems enable artificial states of quantum matter as a promising solid-state platform for coherent sources of single-photon emitters, qubits, topological elements, and quantum sensors due to the coupling of spin to strain (Lemme et al., 2022). Regardless of the substrate, the layered materials are built by stacking and can create intrinsically thin (<1 nm monolayers) hybrid homo- or heterostructures (HHs) in a physical sense with preferentially oriented crystalline structures, tracing advantages for electronic devices, such as transistors and memristors, due to distinguished quantum confinement behavior (Franklin, 2015; Maggini and Ferreira, 2021; Chakraborty et al., 2022). Moreover, the control of interlayer physical interactions and immobilization of mixed-dimensional and functional stacked structures result in the van der Waals nano-opto-electro-mechanical couplers (Zhang T. et al., 2022). Micro and nanoelectromechanical systems (MEMS/NEMSs) offer also extremely high sensitivities in piezoresistive and optomechanical platforms that make them efficient signal transducers, providing input stimuli (i.e., signal forces) and reading out a mechanical response (i.e., output displacement) (Lemme et al., 2022; Rawat et al., 2022). The layered structures of 2D materials (e.g., MoS2 and h-BN) and carbon-based materials (graphite and hydrogenated diamond-like carbon) are favorable options as self-lubricating solids to achieve superlubricity behaviors (i.e., near-zero friction regime) at the sliding interfaces of mechanical systems from nanoelements to large-scale setups, including aerospace and wind energy applications (Echeverrigaray et al., 2018; Zhai and Zhou, 2019).
A new concept of designing artificial materials involves making tunable devices based on phase-changed metasurfaces using different sub-wavelength-sized resonators (Ali et al., 2022). This makes it possible to manipulate the polarized states of electromagnetic waves in real time via broadband cross-polarization converters (including microwave and THz frequencies). With this kind of layered metamaterials, there are emerging applications ranging from sensing to stealth technologies such as thermal emitters (Nishijima et al., 2019), imaging and edge detection functionalities (metalenses) (Zou et al., 2020), multiple modulators (or holographic projection) (Huang et al., 2018), and optical “invisible” cloaks (Lee et al., 2021).
Finally, the ever-increasing demand for designing versatile materials inspires the rapid development of tunable devices, creating new paradigms for energy-saving applications. Materials science and engineering drive innovation for fundamental and applied research in all industrial sectors, which is crucial to the development of quantum computers, particularly for implementations using solid-state hardware that becomes an accelerator for information processing. The key changes will be associated with the deployment of multidimensional functional hybrids, whose distinct types of predefined components, structures, and properties shed light on the design of high-efficiency devices. In the future, these modulating strategies adopted for technological development with eco-friendly alternatives made in the ongoing fourth industrial revolution may establish sustainability of artificial intelligence in the energy industry as a thriving global economy.
Concluding remarks
The continuous search of hybrid material systems for providing multifunctionality is based on unidimensional or mixed-dimensional structures with reconfigurable responses, resulting in a significant enhancement of functionality or even enabling new functions. Such multifunctional materials are at the core spotlight of technological applications and the forefront of materials research for more accessible green power resources beneficial to human needs. In a wider perspective, these concomitant needs represent a paradigm shift in the science and technology of advanced materials toward the future of global sustainable energy and digital social innovation. Using data indexed in the WoS and Scopus platforms, quantitative research is to generate knowledge and conduct a comparative, dynamic, and empirical analysis focusing on academic articles published between 2002 and 2022. The suggested design guidelines expand the relevant analysis based on the multiscale fabrication and hybrid integration of different emerging classes of materials, which should deal with current issues of fossil fuel depletion, energy consumption growth, and pollutant emissions. This perspective has interesting concepts that hold promis to unlock material combinations or groundbreaking changes in hybrid solid interfaces (e.g., metacrystals, intermetallics, LDMs, and HHs), opening new possibilities and driving the research for optimization of more effective design in multisource energy harvesting systems.
Data availability statement
The original contributions presented in the study are included in the article/Supplementary Material; further inquiries can be directed to the corresponding author.
Author contributions
FE: visualization, investigation, data curation, and writing–original draft and FA: supervision, project administration, and funding acquisition.
Funding
FE and FA acknowledge the funding received from the Research Support Foundation of the State of São Paulo (Project number FAPESP 2019/00757-0) and the Brazilian National Council of Scientific and Technological Development (Project number CNPq 2019/24095-7). FA is CNPq research fellow, 302370/2015-3.
Conflict of interest
The authors declare that the research was conducted in the absence of any commercial or financial relationships that could be construed as a potential conflict of interest.
Publisher’s note
All claims expressed in this article are solely those of the authors and do not necessarily represent those of their affiliated organizations, or those of the publisher, the editors, and the reviewers. Any product that may be evaluated in this article, or claim that may be made by its manufacturer, is not guaranteed or endorsed by the publisher.
References
Ali, A., Mitra, A., and Aïssa, B. (2022). Metamaterials and metasurfaces: A review from the perspectives of materials, mechanisms and advanced metadevices. Nanomater. (Basel). 12, 1027. doi:10.3390/nano12061027
Assawaworrarit, S., Omair, Z., and Fan, S. (2022). Nighttime electric power generation at a density of 50 mW/m2 via radiative cooling of a photovoltaic cell. Appl. Phys. Lett. 120, 143901. doi:10.1063/5.0085205
Aykol, M., Dwaraknath, S. S., Sun, W., and Persson, K. A. (2018). Thermodynamic limit for synthesis of metastable inorganic materials. Sci. Adv. 4, eaaq0148. doi:10.1126/sciadv.aaq0148
Bai, Y., Jantunen, H., and Juuti, J. (2018). Hybrid, multi-source, and integrated energy harvesters. Front. Mat. 5, 65. doi:10.3389/fmats.2018.00065
Benea-Chelmus, I. C., Meretska, M. L., Elder, D. L., Tamagnone, M., Dalton, L. R., and Capasso, F. (2021). Electro-optic spatial light modulator from an engineered organic layer. Nat. Commun. 12, 5928. doi:10.1038/s41467-021-26035-y
Chakraborty, S. K., Kundu, B., Nayak, B., Dash, S. P., and Sahoo, P. K. (2022). Challenges and opportunities in 2D heterostructures for electronic and optoelectronic devices. iScience 25, 103942. doi:10.1016/j.isci.2022.103942
Chen, K., Wan, X., and Xu, J. (2017). Epitaxial stitching and stacking growth of atomically thin transition‐metal dichalcogenides (TMDCs) heterojunctions. Adv. Funct. Mat. 27, 1603884. doi:10.1002/adfm.201603884
Chen, X., Ren, Z., Han, M., Wan, J., and Zhang, H. (2020). Hybrid energy cells based on triboelectric nanogenerator: from principle to system. Nano Energy 75, 104980. doi:10.1016/j.nanoen.2020.104980
Chowdhury, T., Sadler, E. C., and Kempa, T. J. (2020). Progress and prospects in transition-metal dichalcogenide research beyond 2D. Chem. Rev. 120, 12563–12591. doi:10.1021/acs.chemrev.0c00505
Chung, D. D. (2021). Functional materials: Electrical, dielectric, electromagnetic, optical and magnetic applications. 1st ed, 4. Singapure: World Scientific.
Davariashtiyani, A., Kadkhodaie, Z., and Kadkhodaei, S. (2021). Predicting synthesizability of crystalline materials via deep learning. Commun. Mat. 2, 115. doi:10.1038/s43246-021-00219-x
Dideikin, A. T., and Vul', A. Y. (2019). Graphene oxide and derivatives: The place in graphene family. Front. Phys. 6, 149. doi:10.3389/fphy.2018.00149
Echeverrigaray, F. G., de Mello, S. R., Leidens, L. M., da Costa, M. E. M., Alvarez, F., Burgo, T. A., et al. (2018). Towards superlubricity in nanostructured surfaces: The role of van der Waals forces. Phys. Chem. Chem. Phys. 20, 21949–21959. doi:10.1039/C8CP02508H
Echeverrigaray, F. G., Figueroa, C. A., Zanatta, A. R., and Alvarez, F. (2022). Heterophase interface and surface functionalization of TiOx/TiSix metastable nanofilms. Adv. Mat. Interfaces 9, 2200799. doi:10.1002/admi.202200799
Franklin, A. D. (2015). Nanomaterials in transistors: From high-performance to thin-film applications. Science 349, aab2750. doi:10.1126/science.aab2750
García de Arquer, F. P., Talapin, D. V., Klimov, V. I., Arakawa, Y., Bayer, M., and Sargent, E. H. (2021). Semiconductor quantum dots: Technological progress and future challenges. Science 373, eaaz8541. doi:10.1126/science.aaz8541
Geim, A. K., and Novoselov, K. S. (2007). The rise of graphene. Nat. Mat. 6, 183–191. doi:10.1038/nmat1849
Guan, G., and Han, M. Y. (2019). Functionalized hybridization of 2D nanomaterials. Adv. Sci. 6, 1901837. doi:10.1002/advs.201901837
Guo, T., Yao, M. S., Lin, Y. H., and Nan, C. W. (2015). A comprehensive review on synthesis methods for transition-metal oxide nanostructures. CrystEngComm 17, 3551–3585. doi:10.1039/C5CE00034C
Huang, L., Zhang, S., and Zentgraf, T. (2018). Metasurface holography: From fundamentals to applications. Nanophotonics 7, 1169–1190. doi:10.1515/nanoph-2017-0118
Huang, S., and Mochalin, V. N. (2020). Understanding chemistry of two-dimensional transition metal carbides and carbonitrides (MXenes) with gas analysis. ACS Nano 14, 10251–10257. doi:10.1021/acsnano.0c03602
International Organization for Standardization (2022). ISO Strategy 2030. Available at: https://www.iso.org/strategy2030 (Accessed May 10, 2022).
Isaacoff, B. P., and Brown, K. A. (2017). Progress in top-down control of bottom-up assembly. Nano Lett. 17, 6508–6510. doi:10.1021/acs.nanolett.7b04479
Jeong, J. H., Kang, S., Kim, N., Joshi, R. K., and Lee, G. H. (2022). Recent trends in covalent functionalization of 2D materials. Phys. Chem. Chem. Phys. 24, 10684–10711. doi:10.1039/D1CP04831G
Khan, K., Tareen, A. K., Aslam, M., Wang, R., Zhang, Y., Mahmood, A., et al. (2020). Recent developments in emerging two-dimensional materials and their applications. J. Mat. Chem. C 8, 387–440. doi:10.1039/C9TC04187G
Kilner, J. A., Skinner, S. J., Irvine, S. J. C., and Edwards, P. P. (2012). Functional materials for sustainable energy applications. 1st ed. United Kigdom: Woodhead Publishing Series in Energy, Elsevier.
Laipan, M., Yu, J., Zhu, R., Zhu, J., Smith, A. T., He, H., et al. (2020). Functionalized layered double hydroxides for innovative applications. Mat. Horiz. 7, 715–745. doi:10.1039/c9mh01494b
Lau, D., Song, N., Hall, C., Jiang, Y., Lim, S., Perez-Wurfl, I., et al. (2019). Hybrid solar energy harvesting and storage devices: The promises and challenges. Mat. Today Energy 13, 22–44. doi:10.1016/j.mtener.2019.04.003
Lee, K. T., Ji, C., Iizuka, H., and Banerjee, D. (2021). Optical cloaking and invisibility: From fiction toward a technological reality. J. Appl. Phys. 129, 231101. doi:10.1063/5.0048846
Lemme, M. C., Akinwande, D., Huyghebaert, C., and Stampfer, C. (2022). 2D materials for future heterogeneous electronics. Nat. Commun. 13, 1392. doi:10.1038/s41467-022-29001-4
Liang, L., Wang, J., Lin, W., Sumpter, B. G., Meunier, V., and Pan, M. (2014). Electronic bandgap and edge reconstruction in phosphorene materials. Nano Lett. 14, 6400–6406. doi:10.1021/nl502892t
Maggini, L., and Ferreira, R. R. (2021). 2D material hybrid heterostructures: Achievements and challenges towards high throughput fabrication. J. Mat. Chem. C 9, 15721–15734. doi:10.1039/D1TC04253J
Martinho, F. (2021). Challenges for the future of tandem photovoltaics on the path to terawatt levels: A technology review. Energy Environ. Sci. 14, 3840–3871. doi:10.1039/D1EE00540E
McClintock, H. D., Doshi, N., Iniguez‐Rabago, A., Weaver, J. C., Jafferis, N. T., Jayaram, K., et al. (2021). A fabrication Strategy for reconfigurable millimeter‐scale metamaterials. Adv. Funct. Mat. 31, 2103428. doi:10.1002/adfm.202103428
Midolo, L., Schliesser, A., and Fiore, A. (2018). Nano-opto-electro-mechanical systems. Nat. Nanotechnol. 13, 11–18. doi:10.1038/s41565-017-0039-1
Miró, P., Audiffred, M., and Heine, T. (2014). An atlas of two-dimensional materials. Chem. Soc. Rev. 43, 6537–6554. doi:10.1039/C4CS00102H
Morales, M., Droppa, R., De Mello, S. R. S., Figueroa, C. A., Zanatta, A. R., and Alvarez, F. (2018). Self-organized nickel nanoparticles on nanostructured silicon substrate intermediated by a titanium oxynitride (TiNxOy) interface. AIP Adv. 8, 015025. doi:10.1063/1.4993143
Nishijima, Y., Balčytis, A., Naganuma, S., Seniutinas, G., and Juodkazis, S. (2019). Kirchhoff’s metasurfaces towards efficient photo-thermal energy conversion. Sci. Rep. 9, 8284. doi:10.1038/s41598-019-44781-4
Pandey, M., Jacobsen, K. W., and Thygesen, K. S. (2016). Atomically thin ordered alloys of transition metal dichalcogenides: Stability and band structures. J. Phys. Chem. C 120, 23024–23029. doi:10.1021/acs.jpcc.6b07283
Phillips, J. D. (2021). Energy harvesting in nanosystems: Powering the next generation of the internet of things. Front. Nanotechnol. 3, 633931. doi:10.3389/fnano.2021.633931
Rawat, U., Anderson, J. D., and Weinstein, D. (2022). Design and applications of integrated transducers in commercial CMOS technology. Front. Mech. Eng. 8, 902421. doi:10.3389/fmech.2022.902421
ReportLinker (2022). Global advanced functional materials market 2022-206. Available at: https://www.reportlinker.com/p05793697/Global-Advanced-Functional-Materials-Market (Accessed May 15, 2022).
Reyes-Belmonte, M. A. (2021). The energy and environment connection, research trends based a bibliometric analysis. Energy Ecol. Environ. 6, 479. doi:10.1007/s40974-021-00220-7
Salonitis, K., Pandremenos, J., Paralikas, J., and Chryssolouris, G. (2010). Multifunctional materials: Engineering applications and processing challenges. Int. J. Adv. Manuf. Technol. 49, 803–826. doi:10.1007/s00170-009-2428-6
Wan, X., Huang, Y., and Chen, Y. (2012). Focusing on energy and optoelectronic applications: A journey for graphene and graphene oxide at large scale. Acc. Chem. Res. 45, 598–607. doi:10.1021/ar200229q
Wang, D., Chen, D., and Chen, Z. (2020). Recent progress in 3D printing of bioinspired structures. Front. Mat. 7, 286. doi:10.3389/fmats.2020.00286
Wang, Y., Ahmed, A., Azam, A., Bing, D., Shan, Z., Zhang, Z., et al. (2021). Applications of additive manufacturing (AM) in sustainable energy generation and battle against COVID-19 pandemic: The knowledge evolution of 3D printing. J. Manuf. Syst. 60, 709–733. doi:10.1016/j.jmsy.2021.07.023
World Semiconductor Trade Statistics (2022). WSTS market Statistics. Available at: https://www.wsts.org/61/MARKET-STATISTICS (Accessed May 12, 2022).
Wu, Y., Qu, J., Chu, P. K., Shin, D. M., Luo, Y., and Feng, S. P. (2021). Hybrid photovoltaic-triboelectric nanogenerators for simultaneously harvesting solar and mechanical energies. Nano Energy 89, 106376. doi:10.1016/j.nanoen.2021.106376
Xie, S., Erjawetz, J., Schuster, C., and Schift, H. (2021). Hybrid structures by direct write lithography—tuning the contrast and surface topography of grayscale photoresist with nanoimprint. J. Vac. Sci. Technol. B 39, 052603. doi:10.1116/6.0001206
Xie, Y., Zhang, Z., Zhou, H., Wang, Z., Lin, Y., Chen, Y., et al. (2022). Multisource energy harvester with coupling structure and multiplexing mechanism. Adv. Mat. Interfaces 9, 2200468. doi:10.1002/admi.202200468
Yamaguchi, M., Dimroth, F., Geisz, J. F., and Ekins-Daukes, N. J. (2021). Multi-junction solar cells paving the way for super high-efficiency. J. Appl. Phys. 129, 240901. doi:10.1063/5.0048653
Yang, H., Wang, Y., Tiu, Z. C., Tan, S. J., Yuan, L., and Zhang, H. (2022). All-optical modulation technology based on 2D layered materials. Micromachines 13, 92. doi:10.3390/mi13010092
Yang, R., Xu, J., Wang, J., Ma, R., and Zhang, W. (2019). Frequency-reconfigurable metamaterial absorber/reflector with eight operating modes. Opt. Express 27, 16550. doi:10.1364/OE.27.016550
Yu, P., Besteiro, L. V., Huang, Y., Wu, J., Fu, L., Tan, H. H., et al. (2019). Broadband metamaterial absorbers. Adv. Opt. Mat. 7, 1800995. doi:10.1002/adom.201800995
Zeng, M., Xiao, Y., Liu, J., Yang, K., and Fu, L. (2018). Exploring two-dimensional materials toward the next-generation circuits: From monomer design to assembly control. Chem. Rev. 118, 6236–6296. doi:10.1021/acs.chemrev.7b00633
Zhai, W., and Zhou, K. (2019). Nanomaterials in superlubricity. Adv. Funct. Mat. 29, 1806395. doi:10.1002/adfm.201806395
Zhang, T., Wang, H., Xia, X., Yan, N., Sha, X., Huang, J., et al. (2022). A monolithically sculpted van der Waals nano-opto-electro-mechanical coupler. Light. Sci. Appl. 11, 48. doi:10.1038/s41377-022-00734-7
Zhang, Y., Fonseka, H. A., Yang, H., Yu, X., Jurczak, P., Huo, S., et al. (2022). Thermally-driven formation method for growing (quantum) dots on sidewalls of self-catalysed thin nanowires. Nanoscale Horiz. 7, 311–318. doi:10.1039/D1NH00638J
Zheng, X., Smith, W., Jackson, J., Moran, B., Cui, H., Chen, D., et al. (2016). Multiscale metallic metamaterials. Nat. Mat. 15, 1100–1106. doi:10.1038/nmat4694
Keywords: renewable energy systems, multifunctional properties, mixed-dimensional structures, hybrid integration, interfacial engineering
Citation: Echeverrigaray FG and Alvarez F (2022) Searching guidelines for scalable and controllable design of multifunctional materials and hybrid interfaces: Status and perspective. Front. Chem. Eng. 4:980882. doi: 10.3389/fceng.2022.980882
Received: 29 June 2022; Accepted: 28 July 2022;
Published: 31 August 2022.
Edited by:
Mikhail Zheludkevich, Helmholtz Centre for Materials and Coastal Research (HZG), GermanyReviewed by:
Jianjian Wang, Chongqing University, ChinaIoannis A. Kartsonakis, National Technical University of Athens, Greece
Tiago L. P. Galvão, University of Aveiro, Portugal
Copyright © 2022 Echeverrigaray and Alvarez. This is an open-access article distributed under the terms of the Creative Commons Attribution License (CC BY). The use, distribution or reproduction in other forums is permitted, provided the original author(s) and the copyright owner(s) are credited and that the original publication in this journal is cited, in accordance with accepted academic practice. No use, distribution or reproduction is permitted which does not comply with these terms.
*Correspondence: Fernando G. Echeverrigaray, fgecheve@gmail.com, fgecheve@ifi.unicamp.br
†ORCID: Fernando G. Echeverrigaray, orcid.org/0000-0003-1900-6376; Fernando Alvarez, orcid.org/0000-0002-9393-1298