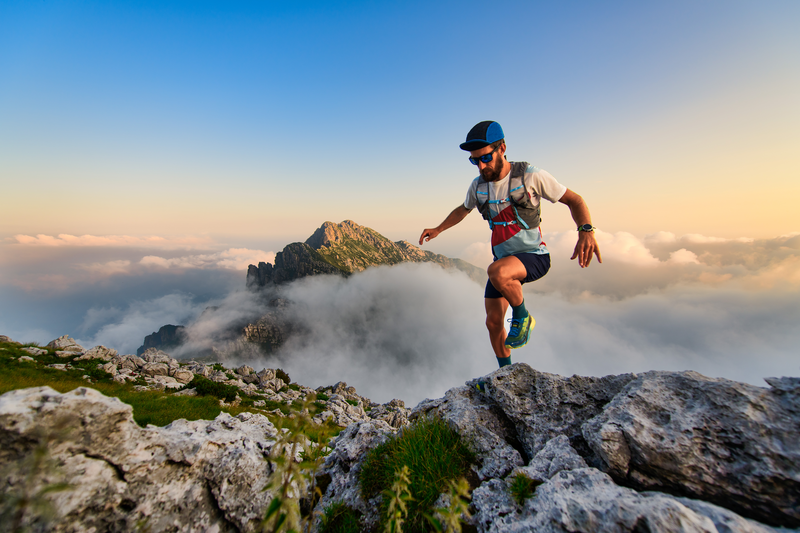
94% of researchers rate our articles as excellent or good
Learn more about the work of our research integrity team to safeguard the quality of each article we publish.
Find out more
MINI REVIEW article
Front. Chem. Eng. , 23 June 2022
Sec. Biochemical Engineering
Volume 4 - 2022 | https://doi.org/10.3389/fceng.2022.851822
This article is part of the Research Topic Biochemical Engineering: Advances and Reviews View all 3 articles
Persistence of ammonium nitrogen and heavy metals in wastewater still remains a challenge, and many wastewater treatment plants face the challenge of removing nitrogen under heavy metal stresses. There is no preferred method for the biological treatment of wastewater containing nitrogen and heavy metals with the possible exception of the anaerobic ammonium oxidation (anammox), since it has shown promise for removing nitrogen under heavy metal stresses. This article reviews the recent research results of the nitrogen-removal performance and mechanism by the anammox process under heavy metal stresses, mainly discussing the enhancing and inhibition effects of heavy metals on the performance of the Anammox reactor. The influencing mechanism of heavy metals on the microbial community and extracellular polymeric substances is also presented, and examples are given for explanation. The main problems of the present research are pointed out, and it is proposed that unifying the metal ion concentrations of inhibiting or promoting anammox activity is necessary for the development and industrial application of the anammox process. The information of this review can offer a great possibility for achieving desired nitrogen removal in wastewater treatment under heavy metal stresses and with significant energy savings.
Anaerobic ammonium oxidation (anammox) is an energy- and resource-efficient biological process compared with conventional nitrification–denitrification (Rabbani et al., 2021) in wastewater-treatment plants (WWTPs). Therefore, the anammox process has been studied by most wastewater treatment operators and researchers. Six candidatus genera associated with Anammox (Brocadia, Kuenenia, Jettenia, Anammoxoglobus, Anammoximicrobium, and Scalindua) have been found in many circumstances (Wan et al., 2018), but the industrial-scale installation of the anammox process has still been hindered due to the anammox bacteria’s sensitivity to various environmental factors (Zekker et al., 2016), such as temperature, substrate concentration, and heavy metal. In particular, heavy metal elements have become one of the most critical influences on account of their hard biodegraded and easily enriched characteristics (Xu L.-Z.-J. et al., 2019; Liu et al., 2020); luckily, not all heavy metals have an inhibitory action on anammox.
Heavy metals can be easily released into wastewater during different stages of their life cycle including production, manufacturing, and consumption (Zhang et al., 2018a; Zhang et al., 2019a; Xu J.-J. et al., 2019; Zhang et al., 2022). As one of the major acceptors, there is strong evidence exhibiting that many heavy metals have already been present in WWTPs, such as a class of substances called metal oxide nanoparticles (MONPs) (Walden and Zhang, 2016), chromium (Cr (II)) (Boparai et al., 2013; Chen et al., 2014; Shi, 2019), zinc (Zn (II)) (Ma et al., 2020), Ag nanoparticles (AgNPs), nickel (Ni), lead (Pb), copper (Cu) (Zhang et al., 2019a), cadmium (Cd) (Awata et al., 2013; Sun et al., 2020), arsenic (III) (Ma et al., 2021), and so on. The heavy metals, after entering the municipal and/or industrial wastewater treatment plants, usually give a negative impact on the microorganisms in activated sludge and the wastewater treatment performance (Wang et al., 2017).
Many scholars focus on the effect of heavy metals on anammox (Zhang et al., 2018b; Zhang et al., 2018c; Li et al., 2018; Xu L.-Z.-J. et al., 2019; Shi, 2019). However, in the published articles, we can see that there are few studies on wastewater containing heavy metals and some of the results are not consistent, for example, Zn2+ promotes anammox to a certain extent, but the IC50 values in these articles are often different. In addition, there are many uncertainties in the change of EPS and the evolution of microbial community during the treatment of wastewater containing heavy metals by the anammox process. Therefore, the article reviews the inhibitory or promoted effects of heavy metals on anammox in wastewater treatment, and the promotion and inhibition mechanisms are also presented from two aspects of EPS and microbial community changes, which will be conducive to further research and application of the anammox process in the treatment of wastewater containing nitrogen and heavy metals.
Low concentrations of Mn2+, Zn2+, Fe2+, and Cu2+ are the essential micronutrients and components of many enzymes and co-enzymes for anammox bacteria (Strous et al., 1998; Aktan et al., 2018). When the metal ions were added into the wastewater entering the anammox system, the denitrification performance of anammox could be encouraged (Zhen et al., 2014; Li et al., 2018). As an example, in a recent short-term test, all three elements of Mn2+ (0.5–5 mg/L), Zn2+ (0.5–3 mg/L), and Cu2+ (0.2–2 mg/L) were found to improve the nitrogen removal rate (NRR) and the optimal concentrations were 2.0 mg/L of Mn2+, 2.0 mg/L of Zn2+ and 0.5 mg/L of Cu2+ respectively, accordingly, the NRR were enhanced 54.62, 45.93, and 44.09% (Li et al., 2018). Zhang et al. (2015) suggesting that a low concentration of Cu2+ (<1.0 mg/L) promoted the anammox bacteria activity and had positive nitrogen removal efficiency. Huang et al. (2014) indicated that the nitrogen removal increased at the concentrations of 0.08 mmol/L Fe2+and 0.05 mmol/L Mn2+ and the maximum removal efficiency was nearly 95%. Similarly, the effects of heavy metals on anammox were investigated by long-term operation, as showed in Table 1. The denitrification capacity of the anammox process has been greatly improved when low concentrations of Mn2+, Zn2+, Fe2+, Cu2+, and Cr2+ were added in the treatment system, which indicated that these heavy metals were beneficial to anammox in both short-term and long-term experiments. However, there were also some contradictory or surprising conclusions reported in the literature. For example, Kimura and Isaka found limited effects of Zn2+ on anammox activity at a low concentration (0.1–5 mg/L). Li’ work showed that only Mn2+ addition could enhance the long-term anammox process under the same operating conditions as the short-term, the amendment of Zn2+ (2 mg/L) reduced the NRR and Cu2+ (0.5 mg/L) had no significant effect on the NRR (Li et al., 2018). Fe2+ in 1–5 mg/L was reported that it could obviously enhance anammox (Zhang et al., 2018d), while another study suggested that 5.3 mg/L Fe 2+ effectively promoted the start-up of anammox and reached high nitrogen removal (Tang S.-m. et al., 2020). It might be explained by the unique microbial community composition and microbial growth rates or the different characteristics in various anammox processes. The studies could indicate the synergistic effects of trace elements on anammox, both short-term encouraging activities of anammox and the long-term altering microbial community.
In addition to the enhancing effects of heavy metals on the anammox performance, there are also some heavy metals that can inhibit anammox. Most of these metals are biotoxic and can be often found in highly ammonium-loaded wastewaters, such as Cd(II) and Cr(VI), which often inhibit anammox even at low concentrations (Jiang et al., 2018; Vardhan et al., 2019; Madeira and Araújo, 2021). Some engineering MONPs may not have an obvious inhibition effect on the denitrification performance, but may have an impact on the formation of anammox flora (Wang et al., 2017; Zhang et al., 2019a; Xu J.-J. et al., 2019; Zhang et al., 2022).
Cu2+ and Zn2+ have a promotion effect on anammox at low concentrations, but can lead to the inhibition of anammox at high concentrations (Li et al., 2014; Li et al., 2018; Zhang et al., 2018e; Gutwinski et al., 2021). A dramatic inhibition behavior was observed beyond 10 mg/L of Zn2+ (Kimura and Isaka, 2014) and the suppression occurred when Cu2+ concentration ranged from 5 mg/L to 10 mg/L (Zhang et al., 2015). The IC50 values of Cu (II), Zn (II), and Mn (II) were calculated to be 30, 25, and 4.83 mg/L, respectively, and the NAA (normalized Anammox activity) decreased rapidly with the increase of heavy metal concentrations (Zhang et al., 2016; Tang et al., 2020b). Gutwinski et al. (2021) found that the mixture of Ni (II), Cu (II), and Zn (II) could strongly inhibit the nitrogen removal in an anammox batch reactor and Zn (II) was considered responsible for the inhibition. It was reported that Fe2+ in 10–30 mg L−1 reversibly suppressed anammox (Zhang et al., 2018d). However, another study reported that anammox was suppressed and completely inhibited by the addition of 109.29 and 378.57 mg/L Fe2+, respectively, via uncompetitive inhibition (Li et al., 2020). As (III) (≥10 mg/L) also had negative but reversible effects on the anammox process (Ma et al., 2021). Usually, many metal ions affect the anammox process, all of which promote anammox activity at low concentrations and inhibit it at high concentrations (Gutwinski et al., 2021; Huang et al., 2022).
The NRR decreased under the action of Cd (II) and Cr (VI), but some authors reported the different NRR performances even at the same dosing of Cd (II). For example, Xu L.-Z.-J. et al. (2019) determined the short-term effect of Cd (II) on anammox and found that adding 1 mg Cd (II) had a slight positive effect, then had an inhibitory effect with the increased dose. In the same short-term experiment, Zhang et al. (2018b) found that 1 mg/L Cd (II) displayed a disparate result in which the NRR declined by 62% in the anammox system. For the different results, it might be induced by different seed sludge and dominant bacteria (Xu L.-Z.-J. et al., 2019). The same goes for the long-term experiments. Xu L.-Z.-J. et al. (2019) indicated well adaptation of anammox bacteria for good NRR performance at the Cd (II) concentrations of 1, 2, and 5 mg/L, respectively. However, inconsistent conclusions were observed in the study of Zhang et al. (2018b), the anammox bacteria could not work properly at the Cd (II) concentration of 5 mg/L. Cr (VI) was one of the most stable and abundant metal ions in industrial wastewater (Yu et al., 2016). 2 mg/L Cr (VI) had a strong inhibitory effect on anammox, which indicated its strong biological toxicity (Jiang et al., 2018). Moreover, when Cd (II) and Cr (VI) were removed, the NRR could be restored to some extent, but not to the initial NRR performance. The results indicated that anammox bacteria had some resistance to Cd (II) and Cr (VI), but the damage was irreversible due to the decline of biodiversity and functional bacteria (Zhang et al., 2018c; Jiang et al., 2018). However, it was reported that the mixture of Cd2+, Cr3+, and Pb2+, even at the highest concentrations, did not adversely affect the course of the anammox process (Gutwinski et al., 2021). Maybe further studies should focus on the examination of the join effects of different metal mixtures.
EPS is the most important factor that enables the microorganisms to survive shocks and protects them from damage (Awata et al., 2013; Zhang et al., 2018c; Ma et al., 2021). Metal ions can cause the change of EPS (as shown in Figure 1) and promote the nitrogen-removal performance of anammox. Since anammox bacteria generally inhabit the inner layer of granules (Vlaeminck et al., 2010), and heavy metals can only enter into anammox through EPS, it is very important to study the effect of heavy metals on the EPS secretion of anammox.
It could be seen in several literatures (Zhang et al., 2018c; Zhang et al., 2018e; Li et al., 2018), that EPS production increased due to the addition of Mn2+, Zn2+, Ag2+, and Cu2+. Most of the 343 mg/L EPS was obtained under the action of Ag+, while the effect of other metal ions was less for EPS production, which was probably because Mn2+, Cu2+, and Zn2+ were the trace elements for bacterial growth and Ag+ was toxic. The toxicity of Ag+ made anammox bacteria produce a lot of EPS, and EPS could be used as an adsorption carrier and permeability barrier to reduce Ag+ directly contacting and interacting with anammox cells (Zhang et al., 2018e). The production of EPS protected bacteria from damage under the action of Ag+ and the deposition of AgNPs was observed in the outer layer of anammox granules. Since EPS could combine with cations (Geddie and Sutherland, 1993), heavy metal ions, as cations, would inevitably combine with EPS. Liu et al. (2020) indicated that the adsorption preference was in the order: Pb (II) > Cd (II) > Cr (VI). The role of anammox sludge EPS in the protection against Cu (II) was elucidated by Li et al. (2020a), which was that low concentrations of Cu (II) resulted in a bridging effect, leading to agglomeration and compaction of the colloids. However, it is still unclear how EPS intercepts heavy metals and where the final destination of the heavy metals is, which needs further research. Proteins (PN) and polysaccharides (PS) were often the restriction components (Hou et al., 2015). It was inferred that there were many binding sites located in PN and anammox bacteria including amino, carboxyl, hydroxyl, and phosphate functional groups (Zhang et al., 2016; Ma et al., 2021). The addition of heavy metals could led to the increase of the PN content to resist the toxic effects of heavy metals (Zhang et al., 2018b). The results will be helpful to study how heavy metals are intercepted outside anammox cells and guide us to mainly study PN rather than the whole EPS.
What is apparent in the aforementioned argument is that EPS plays an important role to protect anammox bacteria from damage, but why is the anammox activity still inhibited in the presence of some heavy metals? As we know, the anammox bacteria are surrounded and compressed by EPS. When heavy metals enter the influent water, the negatively charged functional groups in PN and PS could effectively bound with heavy metal ions, which were considered as a kind of osmotic protective agents to prevent the metals from entering the intracellular environment (Hou et al., 2015; Li et al., 2020b). In addition, some exogenous enzymes contained in EPS could also bind and inhibit the migration of heavy metal ions (Xu L.-Z.-J. et al., 2019) and protect the anammox bacteria from damage. However, the protection was obviously limited. In a long-term experiment, only 1–2 mg/L Cr (VI) in influent could inhibit an anammox reaction. In the study of Zhang et al. (2018c), since EPS could not intercept Zn (II) immediately and Zn (II) would migrate from EPS to the cell surface or cell, excessive Zn (II) taken in by cells led to the lower anammox activity, EPS secretion reduced and finally worsened to cell death. At higher Cu (II) concentrations, the secondary structure of the EPS proteins was disrupted to a loosened state (Li et al., 2020a). In many literatures, unfortunately, only the content of heavy metals on the biofilm carrier was reported without further analysis.
As discussed previously, EPS has a certain protective effect on anammox. Heavy metals can increase the secretion of EPS, and PS and PN also have different changes. However, EPS can only intercept part of the heavy metals, not completely. Therefore, high concentrations of heavy metals (such as Cr6+, Zn2+ and Cu2+ etc.) can enter the anammox cells, causing reversible or irreversible damage to anammox bacteria, then the activity of anammox bacteria reduces and results in the reduction of NRR, even more seriously, cell death.
With the participation of heavy metals, the key microorganisms about anammox may transform, including their activity, abundance, and structure (as shown in Figure 2), resulting in changes in nitrogen metabolism and denitrification performance. As mentioned before, low concentrations of Mn2+, Cu2+ and Zn2+ are generally beneficial to the key microorganisms concerning the denitrification performance of anammox. In a long-term experiment, Mn2+ had the greatest effect on NRR, followed by Zn2+, then Cu2+, and finally Ag+. This was directly related to the abundance of ammonia oxidizing bacteria (AOB) and the anammox bacteria. Mn2+ not only increased the abundance of AOB, but also anammox bacteria, which had a rise from 2.68 to 42.74% and 4.50–43.1%, respectively (Li et al., 2018). However, the abundance of AOB and anammox bacteria did not change after Cu2+ addition, thus, the NRR has hardly increased. The presence of 10–50 mg/L AgNPs could significantly increase the diversity and richness of the microbial community and also improved the resistance of the anammox particles to AgNPs (Zhang et al., 2018e). It had been proved that kuenenia abundance was positively correlated with NRR (Zhang et al., 2018f), in which 1 mg/L to 50 mg/L of AgNPs could make kuenenia have the maximum abundance at the genus level and other genus did not change much compared with the control group (Zhang et al., 2018e). The result showed that the presence of Ag+ did not always have adverse effects on the denitrification performance of anammox and damaged some key bacteria, but it could not significantly promote the denitrification performance of anammox. The specific reasons for the results need more research, but one possibility is that AgNPs has limited ability to release Ag+ and will not pose a threat to the viability of the anammox bacteria living in the particles.
Zn (II) is one kind of essential micronutrient for vital cofactors of metalloproteinases and certain enzymes, and are often found in nitrogen-rich wastewaters (Ma et al., 2020). With the long-term addition of Zn (II), the new metal-resistant microorganisms appeared and the microbial diversity increased in the anammox reactor (Mertens et al., 2010; Miao et al., 2016; Zhang et al., 2018c). The bacterial abundance associated with anammox also increased, but notably, the AOB increased significantly while anammox had little change. This might be due to the specific binding of Zn2+ to the active site of ammonia monooxygenase in AOB and its positive contribution to its growth and metabolic activities (Gilch et al., 2009; Lee et al., 2011). High AOB abundance resulted in a higher concentration of NO2--N, which inhibited anammox activity and reduced NRR (Li et al., 2018). In another long-term domestication study, it was found that 10 mg/L of Zn2+ increased NRR (Zhang et al., 2018e), but the effect was not particularly good. 2 mg/L Zn2+ had no significant improvement on NRR, which was not consistent with the previous statement, such as Li’s work (Li et al., 2018). The reason for the difference might be that the anammox bacteria domesticated in the anammox reactor had a certain tolerance to Zn2+, low concentration of Zn2+ could be absorbed by AOB (Li et al., 2018) and the abundance of Candidatus kuenenia and Candidatus brocadia reached the maximum at 10 mg/L of Zn2+ (Zhang et al., 2018c). Candidatus brocadia was more advantageous to metal ions (Zhang et al., 2018g). The conclusions provide the theoretical support, that is, anammox can treat high ammonia–nitrogen wastewater containing Zn2+, but it needs a long time of domestication to have a better treatment performance when the Zn2+ concentration in wastewater is no more than 10 mg/L.
Heme C is concerned with hydrazine dehydrogenase (HDH), which is the key enzyme responsible for the transformation of N2H4 to final N2 in the anammox reaction (Lee et al., 2011). The increase of heme C could accelerate the growth of the anammox bacteria and the production of heme C involved with Fe2+. Higher Fe2+ concentration produced more heme C; therefore, adding Fe2+ into the reactor could start up the anammox process quickly. Zhen et al. (2014) found that the number of 16S rRNA gene of Anammox strain was 1.97×109 copies/g biomass after 150 days of operation with 0.05 mm Fe2+, which was 1.73 times higher than that of the reactor without Fe2+. The result could be attributed to the increase of exogenous Fe (II) content in anammox reactor. However, the promotion of Fe (II) is special while other heavy metals play an inhibitory role, such as Cd (II) and Cr (VI), because they are not essential elements for microbial life and often cause some damage to normal life activities of microorganisms.
As for the microbial community, the number of bacteria in connection with protein production would increase after adding some heavy metals (Chen et al., 2007; Enshaei et al., 2011; Zhou et al., 2014; Giovanella et al., 2017), even protein-producing bacteria will possibly become the preponderant phylum (Zhang et al., 2018d). At the genus level, Candidatus kuenenia has always been dominant and has profoundly affected the denitrification performance. In the literature reviewed, the NRR decreased with the decrease of Candidatus kuenenia abundance (Zhang et al., 2018b). For example, when the Cd (II) added exceeded the adaptive capacity of Candidatus kuenenia, the abundance of Candidatus kuenenia decreased, and the denitrification performance of the reactor decreased. Even not adding Cd (II) later, the abundance of Candidatus kuenenia still decreased, which indicated that Cd (II) had a persistent inhibitory effect on the denitrification performance. In addition, it was observed that Candidatus Brocadia was more sensitive to Cd (II) than Candidatus kuenenia in the long-term experiment (Zhang et al., 2018b). As one of the key bacteria for nitrogen removal, the increase of Candidatus kuenenia was conducive to the recovery of the reactor performance, while unfortunately, it was difficult to recover the initial performance. The reasons were diverse, one of which might be that the Cd (II) entered cells and caused both DNA and protein oxidation damage at low concentrations (Simmons et al., 2011; Rusanov et al., 2015), and the performance of the reactor was difficult to recover as the damage was irreversible. Another possibility was that Cd (II) in the influent would adhere to the biofilm carriers generally existing in anammox reactors, resulting in the continuous inhibition of the denitrification performance of anammox (Zhang et al., 2018b). The As(III) stress was a direct threat to anammox bacteria, and the relative abundance of Ca. Kuenenia decreased from 16 to 5% when 50 mg/L As(III) was added. Metal oxide nanoparticles did not affect the denitrification performance of anammox, but affected the microbial community structure. In recent reports, kuenenia tended to use nitrate instead of nitrite as the electron receptor in some denitrification processes. The nitrate produced might be converted into nitrite by heterotrophic denitrifying bacteria (such as Thauera) and could be reused as a substrate by anammox bacteria (Ma et al., 2017; Zhang et al., 2019a; Xu J.-J. et al., 2019; Zhang et al., 2022). However, it is unclear why MONPs can make the heterotrophic denitrification existing in the anammox reactor and not affect the denitrification performance of the reactor.
Anammox is a promising process for treating wastewater containing nitrogen and heavy metals. The types and concentrations of heavy metals will affect the performance of the anammox reactor in the practical application, including the microbial community composition and EPS secretion of anammox bacteria. The changes of EPS and key microorganisms in the anammox reactor can also obviously be responses to the nitrogen-removal performance changes under heavy metal stresses, which will possibly provide a possible strategy for anammox to resist the toxicity of heavy metals.
However, the following fundamental problems need research efforts. 1) There is no reference in the literature that unifies the inhibitory or promoted concentration of metal ions; different values appeared in different studies. Therefore, it is crucial to get clear values of the different heavy metals in response to the inhibition or promotion on the anammox, which can benefit the functional stability and facilitate the engineering application of the anammox system under heavy metals stresses. 2) The changes of the microbial community structure and EPS in the anammox reactor play the important role in the resistance of heavy metals and improvement of the performance of anammox reactor. It was uncertain that whether the changes occurred synchronously or the heavy metals changed the microbial community first, and then led to the change of EPS, or reversely, which need to be tackled. 3) To increase our knowledge for the effect of heavy metals on anammox, developing a clear understanding of the effect mechanism of heavy metals is required, such as a clear cognition of the heavy metals on the LB- EPS and TB-EPS changes, etc. 4) Hopefully, further studies will accurately determine how the anammox process interacts with the different types and concentrations of heavy metals, how heavy metals cycle in the anammox environment, and what the role of other components in wastewater, such as organic compounds in this process, is. Though challenges remain, anammox treatment has offered a great possibility for the wastewater treatment for desired nitrogen removal under heavy metals stress with significant energy savings.
JG conceived the presented theme and designed the manuscript including supervising the project and editing. QR and CW wrote the original draft. All authors contributed to the article and approved the submitted version.
This work was supported by the Open Fund of Shaanxi Key Laboratory of Advanced Stimulation Technology for Oil and Gas Reservoirs (grant No. KFJJ-XB-2019-2).
The authors declare that the research was conducted in the absence of any commercial or financial relationships that could be construed as a potential conflict of interest.
All claims expressed in this article are solely those of the authors and do not necessarily represent those of their affiliated organizations, or those of the publisher, the editors, and the reviewers. Any product that may be evaluated in this article, or claim that may be made by its manufacturer, is not guaranteed or endorsed by the publisher.
Authors acknowledge the help received from the scholars whose articles are cited and included in references to this manuscript. Special thanks to Qingchun Li who supports the work. The authors are also grateful to the editors and publisher of Frontiers from where this article will be reviewed and discussed.
Awata, T., Oshiki, M., Kindaichi, T., Ozaki, N., Ohashi, A., and Okabe, S. (2013). Physiological Characterization of an Anaerobic Ammonium-Oxidizing Bacterium Belonging to the "Candidatus Scalindua" Group. Appl. Environ. Microbiol. 79, 4145–4148. doi:10.1128/AEM.00056-13
Bi, Z., Qiao, S., Zhou, J., Tang, X., and Zhang, J. (2014). Fast Start-Up of Anammox Process with Appropriate Ferrous Iron Concentration. Bioresour. Technol. 170, 506–512. doi:10.1016/j.biortech.2014.07.106
Boparai, H. K., Joseph, M., and O’Carroll, D. M. (2013). Cadmium (Cd2+) Removal by Nano Zerovalent Iron: Surface Analysis, Effects of Solution Chemistry and Surface Complexation Modelingffects of Solution Chemistry and Surface Complexation Modeling. Environ. Sci. Pollut. Res. 20, 6210–6221. doi:10.1007/s11356-013-1651-8
Chen, H.-b., Wang, D.-b., Li, X.-m., Yang, Q., Luo, K., Zeng, G.-m., et al. (2014). Effects of Cd(II) on Wastewater Biological Nitrogen and Phosphorus Removalffects of Cd (II) on Wastewater Biological Nitrogen and Phosphorus Removal. Chemosphere 117, 27–32. doi:10.1016/j.chemosphere.2014.05.057
Chien, C.-C., Hung, C.-W., and Han, C.-T. (2007). Removal of Cadmium Ions during Stationary Growth Phase by an Extremely Cadmium-Resistant Strain of Stenotrophomonas Sp. Environ. Toxicol. Chem. 26, 664–668. doi:10.1897/06-280R.1
Enshaei, M., Khanafari, A., and Sepahey, A. A. (2011). Metallothionein Induction in Two Species of Pseudomonas Exposed to Cadmium and Copper Contamination. Iran. J. Environ. Health Eng. 7 (4), 287–298. https://tspace.library.utoronto.ca/bitstream/1807/62348/1/se10033.pdf.
Geddie, J. L., and Sutherland, I. W. (1993). Uptake of Metals by Bacterial Polysaccharides. J. Appl. Bacteriol. 74, 467–472. doi:10.1111/j.1365-2672.1993.tb05155.x
Gilch, S., Meyer, O., and Schmidt, I. (2009). A Soluble Form of Ammonia Monooxygenase in Nitrosomonas Europaea. Biol. Chem. 390, 863–873. doi:10.1515/BC.2009.085
Giovanella, P., Cabral, L., Costa, A. P., de Oliveira Camargo, F. A., Gianello, C., and Bento, F. M. (2017). Metal Resistance Mechanisms in Gram-Negative Bacteria and Their Potential to Remove Hg in the Presence of Other Metals. Ecotoxicol. Environ. Saf. 140, 162–169. doi:10.1016/j.ecoenv.2017.02.010
Gutwiński, P., Cema, G., Ziembińska-Buczyńska, A., Wyszyńska, K., and Surmacz-Górska, J. (2021). Long-term Effect of Heavy Metals Cr(III), Zn(II), Cd(II), Cu(II), Ni(II), Pb(II) on the Anammox Process Performance. J. Water Process Eng. 39, 101668. doi:10.1016/j.jwpe.2020.101668
Hou, X., Liu, S., and Zhang, Z. (2015). Role of Extracellular Polymeric Substance in Determining the High Aggregation Ability of Anammox Sludge. Water Res. 75, 51–62. doi:10.1016/j.watres.2015.02.031
Huang, D.-Q., Fu, J.-J., Li, Z.-Y., Fan, N.-S., and Jin, R.-C. (2022). Inhibition of Wastewater Pollutants on the Anammox Process: A Review. Sci. Total Environ. 803, 150009. doi:10.1016/j.scitotenv.2021.150009
Huang, X., Gao, D., Peng, S., and Tao, Y. (2014). Effects of Ferrous and Manganese Ions on Anammox Process in Sequencing Batch Biofilm Reactors. J. Environ. Sci. 26, 1034–1039. doi:10.1016/S1001-0742(13)60531-8
Jiang, X.-Y., Cheng, Y.-F., Zhu, W.-Q., Bai, Y.-H., Xu, L.-Z. -J., Wu, X.-Q., et al. (2018). Effect of Chromium on Granule-Based Anammox Processesffect of Chromium on Granule-Based Anammox Processes. Bioresour. Technol. 260, 1–8. doi:10.1016/j.biortech.2018.03.055
Kalkan Aktan, C., Uzunhasanoglu, A. E., and Yapsakli, K. (2018). Speciation of Nickel and Zinc, its Short-Term Inhibitory Effect on Anammox, and the Associated Microbial Community Compositionffect on Anammox, and the Associated Microbial Community Composition. Bioresour. Technol. 268, 558–567. doi:10.1016/j.biortech.2018.08.011
Kimura, Y., and Isaka, K. (2014). Evaluation of Inhibitory Effects of Heavy Metals on Anaerobic Ammonium Oxidation (Anammox) by Continuous Feeding Tests. Appl. Microbiol. Biotechnol. 98, 6965–6972. doi:10.1007/s00253-014-5735-2
Lee, S., Cho, K., Lim, J., Kim, W., and Hwang, S. (2011). Acclimation and Activity of Ammonia-Oxidizing Bacteria with Respect to Variations in Zinc Concentration, Temperature, and Microbial Population. Bioresour. Technol. 102, 4196–4203. doi:10.1016/j.biortech.2010.12.035
Li, G.-F., Ma, W.-J., Cheng, Y.-F., Li, S.-T., Zhao, J.-W., Li, J.-P., et al. (2020b). A Spectra Metrology Insight into the Binding Characteristics of Cu2+ onto Anammox Extracellular Polymeric Substances. Chem. Eng. J. 393, 124800. doi:10.1016/j.cej.2020.124800
Li, G., Puyol, D., Carvajal-Arroyo, J. M., Sierra-Alvarez, R., and Field, J. A. (2014). Inhibition of Anaerobic Ammonium Oxidation by Heavy Metals. J. Chem. Technol. Biotechnol. 90, 830–837. doi:10.1002/jctb.4377
Li, H., Yao, H., Zhang, D., Zuo, L., Ren, J., Ma, J., et al. (2018). Short- and Long-Term Effects of Manganese, Zinc and Copper Ions on Nitrogen Removal in Nitritation-Anammox Process. Chemosphere 193, 479–488. doi:10.1016/j.chemosphere.2017.11.002
Li, Z., Peng, Y., and Gao, H. (2020a). A Novel Strategy for Accelerating the Recovery of a Fe(II)-inhibited Anammox Reactor by Intermittent Addition of Betaine: Performance, Kinetics and Statistical Analysis. Chemosphere 251, 126362. doi:10.1016/j.chemosphere.2020.126362
Liu, Y., Han, J., Dong, S., Li, Y., Liu, S., Zhou, Q., et al. (2020). Competitive Adsorption of Heavy Metals by Anaerobic Ammonium-Oxidizing (Anammox) Consortia. Chemosphere 258, 127289. doi:10.1016/j.chemosphere.2020.127289
Ma, B., Qian, W., Yuan, C., Yuan, Z., and Peng, Y. (2017). Achieving Mainstream Nitrogen Removal through Coupling Anammox with Denitratation. Environ. Sci. Technol. 51, 8405–8413. doi:10.1021/acs.est.7b01866
Ma, W.-J., Ren, Z.-Q., Yu, L.-Q., Wu, X.-X., Yao, Y.-X., Zhang, J.-T., et al. (2021). Deciphering the Response of Anammox Process to Heavy Metal and Antibiotic Stress: Arsenic Enhances the Permeability of Extracellular Polymeric Substance and Aggravates the Inhibition of Sulfamethoxazole. Chem. Eng. J. 426, 130815. doi:10.1016/j.cej.2021.130815
Ma, X., Yan, Y., Wang, W., Guo, J., and Wang, Y. (2020). Metatranscriptomic Analysis of Adaptive Response of Anammox Bacteria Candidatus 'Kuenenia Stuttgartiensis' to Zn(II) Exposure. Chemosphere 246, 125682. doi:10.1016/j.chemosphere.2019.125682
Madeira, C. L., and de Araújo, J. C. (2021). Inhibition of Anammox Activity by Municipal and Industrial Wastewater Pollutants: A Review. Sci. Total Environ. 799, 149449. doi:10.1016/j.scitotenv.2021.149449
Mertens, J., Springael, D., De Troyer, I., Cheyns, K., Wattiau, P., and Smolders, E. (2006). Long-term Exposure to Elevated Zinc Concentrations Induced Structural Changes and Zinc Tolerance of the Nitrifying Community in Soil. Environ. Microbiol. 8, 2170–2178. doi:10.1111/j.1462-2920.2006.01100.x
Miao, L., Wang, C., Hou, J., Wang, P., Ao, Y., Li, Y., et al. (2016). Aggregation and Removal of Copper Oxide (CuO) Nanoparticles in Wastewater Environment and Their Effects on the Microbial Activities of Wastewater Biofilmsffects on the Microbial Activities of Wastewater Biofilms. Bioresour. Technol. 216, 537–544. doi:10.1016/j.biortech.2016.05.082
Rabbani, A., Zainith, S., Deb, V. K., Das, P., Bharti, P., Rawat, D. S., et al. (2021). “Microbial Technologies for Environmental Remediation: Potential Issues, Challenges, and Future Prospects,” in Microbe Mediated Remediation of Environmental Contaminants. Editors A. Kumar, V. K. Singh, P. Singh, and V. K. Mishra (UK: Woodhead Publishing), 271–286. doi:10.1016/b978-0-12-821199-1.00022-5
Rusanov, A. L., Smirnova, A. V., Poromov, A. A., Fomicheva, K. A., Luzgina, N. G., and Majouga, A. G. (2015). Effects of Cadmium Chloride on the Functional State of Human Intestinal Cellsffects of Cadmium Chloride on the Functional State of Human Intestinal Cells. Toxicol. Vitro. 29, 1006–1011. doi:10.1016/j.tiv.2015.03.018
Shi, X. D. (2019). Effects of Heavy Metals and Antibiotics on Anammox Process for Treating Livestock Breeding Wastewater. Chongqing: Chongqing University Press.
Simmons, S. O., Fan, C.-Y., Yeoman, K., Wakefield, J., and Ramabhadran, R. (2011). NRF2 Oxidative Stress Induced by Heavy Metals Is Cell Type Dependent. Curr. Chem. Genomics. 5, 1–12. doi:10.2174/1875397301105010001
Strous, M., Heijnen, J. J., Kuenen, J. G., and Jetten, M. S. M. (1998). The Sequencing Batch Reactor as a Powerful Tool for the Study of Slowly Growing Anaerobic Ammonium-Oxidizing Microorganisms. Appl. Microbiol. Biotechnol. 50, 589–596. doi:10.1007/s002530051340
Sun, Q., Zhao, B. H., Fan, S., Zhou, B. L., and Li, Y. Q. (2020). Effect of Ni(Ⅱ) on Anaerobic Ammonium Oxidation and Changes in Kinetics. Huan Jing Ke Xue. 41 (6), 2779–2786. doi:10.13227/j.hjkx.201912051
Tang, C. J., Zhang, L., Feng, F., Xiong, L., Mahmood, Q., Zeng, W., et al. (2020b). Long‐term Domestication to Mn Stresses Alleviates the Inhibition on Anammox Process. Water Environ. Res. 92, 1966–1974. doi:10.1002/wer.1316
Tang, S.-m., Xu, Z.-h., Liu, Y.-l., Yang, G.-f., Mu, J., Jin, R.-c., et al. (2020a). Performance, Kinetics Characteristics and Enhancement Mechanisms in Anammox Process under Fe(II) Enhanced Conditions. Biodegradation 31, 223–234. doi:10.1007/s10532-020-09905-y
Vardhan, K. H., Kumar, P. S., and Panda, R. C. (2019). A Review on Heavy Metal Pollution, Toxicity and Remedial Measures: Current Trends and Future Perspectives. J. Mol. Liq. 290, 111197. doi:10.1016/j.scitotenv.2021.14944910.1016/j.molliq.2019.111197
Vlaeminck, S. E., Terada, A., Smets, B. F., De Clippeleir, H., Schaubroeck, T., Bolca, S., et al. (2010). Aggregate Size and Architecture Determine Microbial Activity Balance for One-Stage Partial Nitritation and Anammox. Appl. Environ. Microbiol. 76 (3), 900–909. doi:10.1016/S0921-5093(03)00563-X10.1128/aem.02337-09
Walden, C., and Zhang, W. (2016). Biofilms versus Activated Sludge: Considerations in Metal and Metal Oxide Nanoparticle Removal from Wastewaterfilms versus Activated Sludge: Considerations in Metal and Metal Oxide Nanoparticle Removal from Wastewater. Environ. Sci. Technol. 50, 8417–8431. doi:10.1021/acs.est.6b01282
Wang, S., Liu, Z., Wang, W., and You, H. (2017). Fate and Transformation of Nanoparticles (NPs) in Municipal Wastewater Treatment Systems and Effects of NPs on the Biological Treatment of Wastewater: a Review. RSC Adv. 7, 37065–37075. doi:10.1039/C7RA05690G
Xu, J.-J., Cheng, Y.-F., Xu, L.-Z. -J., Liu, Y.-Y., Zhu, B.-Q., Fan, N.-S., et al. (2019). The Revolution of Performance, Sludge Characteristics and Microbial Community of Anammox Biogranules under Long-Term NiO NPs Exposure. Sci. Total Environ. 649, 440–447. doi:10.1016/j.scitotenv.2018.08.386
Xu, L.-Z. -J., Wu, J., Xia, W.-J., Jin, L.-Y., Zhao, Y.-H., Fan, N.-S., et al. (2019). Adaption and Restoration of Anammox Biomass to Cd(II) Stress: Performance, Extracellular Polymeric Substance and Microbial Community. Bioresour. Technol. 290, 121766. doi:10.1016/j.biortech.2019.121766
Yang, W., He, S., Han, M., Wang, B., Niu, Q., Xu, Y., et al. (2018). Nitrogen Removal Performance and Microbial Community Structure in the Start-Up and Substrate Inhibition Stages of an Anammox Reactor. J. Biosci. Bioeng. 126 (1), 88–95. doi:10.1016/j.jbiosc.2018.02.004
Yu, C., Song, Y.-X., Chai, L.-Y., Duan, C.-S., Tang, C.-J., Ali, M., et al. (2016). Comparative Evaluation of Short-Term Stress of Cd(II), Hg(II), Pb(II), As(III) and Cr(VI) on Anammox Granules by Batch Test. J. Biosci. Bioeng. 122, 722–729. doi:10.1016/j.jbiosc.2016.06.008
Zekker, I., Rikmann, E., Mandel, A., Kroon, K., Seiman, A., Mihkelson, J., et al. (2016). Step-wise Temperature Decreasing Cultivates a Biofilm with High Nitrogen Removal Rates at 9°C in Short-Term Anammox Biofilm Tests. Environ. Technol. 37 (15), 1933–1946. doi:10.1080/09593330.2015.1135995
Zhang, C., Hu, Z., and Deng, B. (2016e). Silver Nanoparticles in Aquatic Environments: Physiochemical Behavior and Antimicrobial Mechanisms. Water Res. 88, 403–427. doi:10.1016/j.watres.2015.10.025
Zhang, Q.-Q., Zhang, Z.-Z., Guo, Q., Chen, Q.-Q., Jin, R.-C., and Jia, X.-Y. (2015). Variation in the Performance and Sludge Characteristics of Anaerobic Ammonium Oxidation Inhibited by Copper. Sep. Purif. Technol. 142, 108–115. doi:10.1016/j.seppur.2014.11.048
Zhang, S., Zhang, L., Yao, H., Rong, H., and Li, S. (2021). Responses of Anammox Process to Elevated Fe(III) Stress: Reactor Performance, Microbial Community and Functional Genes. J. Hazard. Mater. 414, 125051. doi:10.1016/j.jhazmat.2021.125051
Zhang, X., Chen, Z., Ma, Y., Zhao, J., Chen, T., Fu, H., et al. (2018b). Acute and Persistent Toxicity of Cd(II) to the Microbial Community of Anammox Process. Bioresour. Technol. 261, 453–457. doi:10.1016/j.biortech.2018.04.055
Zhang, X., Chen, Z., Ma, Y., Zhou, Y., Zhao, S., Wang, L., et al. (2018c). Influence of Elevated Zn (II) on Anammox System: Microbial Variation and Zinc Tolerancefluence of Elevated Zn (II) on Anammox System: Microbial Variation and Zinc Tolerance. Bioresour. Technol. 251, 108–113. doi:10.1016/j.biortech.2017.12.035
Zhang, X., Chen, Z., Zhou, Y., Ma, Y., Ma, C., Li, Y., et al. (2019b). Impacts of the Heavy Metals Cu (II), Zn (II) and Fe (II) on an Anammox System Treating Synthetic Wastewater in Low Ammonia Nitrogen and Low Temperature: Fe (II) Makes a Difference. Sci. Total Environ. 648, 798–804. doi:10.1016/j.scitotenv.2018.08.206
Zhang, X., Chen, Z., Zhou, Y., Ma, Y., Zhang, H., Zhou, L., et al. (2019a). Comparisons of Nitrogen Removal and Microbial Communities in Anammox Systems upon Addition of Copper-Based Nanoparticles and Copper Ion. Ind. Eng. Chem. Res. 58 (19), 7808–7816. doi:10.1021/acs.iecr.9b00182
Zhang, X., Wei, D., Zhang, H., He, Y., Zhang, S., Dai, J., et al. (2022). Comprehensive Analysis of the Impacts of Iron-Based Nanoparticles and Ions on Anammox Process. Biochem. Eng. J. 180, 108371. doi:10.1016/j.bej.2022.108371
Zhang, X., Zhou, Y., Xu, T., Zheng, K., Zhang, R., Peng, Z., et al. (2018g). Toxic Effects of CuO, ZnO and TiO2 Nanoparticles in Environmental Concentration on the Nitrogen Removal, Microbial Activity and Community of Anammox Processffects of CuO, ZnO and TiO Nanoparticles in Environmental Concentration on the Nitrogen Removal, Microbial Activity and Community of Anammox Process. Chem. Eng. J. 332, 42–48. doi:10.1016/j.cej.2017.09.072
Zhang, X., Zhou, Y., Zhao, S., Zhang, R., Peng, Z., Zhai, H., et al. (2018d). Effect of Fe (II) in Low-Nitrogen Sewage on the Reactor Performance and Microbial Community of an ANAMMOX Biofilter. Chemosphere 200, 412–418. doi:10.1016/j.chemosphere.2018.02.131
Zhang, Z.-Z., Cheng, Y.-F., Xu, L.-Z. -J., Bai, Y.-H., and Jin, R.-C. (2018f). Anammox Granules Show Strong Resistance to Engineered Silver Nanoparticles during Long-Term Exposure. Bioresour. Technol. 259, 10–17. doi:10.1016/j.biortech.2018.03.024
Zhang, Z.-Z., Cheng, Y.-F., Xu, L.-Z. -J., Bai, Y.-H., Xu, J.-J., Shi, Z.-J., et al. (2018a). Evaluating the Effects of Metal Oxide Nanoparticles (TiO2, Al2O3, SiO2 and CeO2) on Anammox Process: Performance, Microflora and Sludge Propertiesffects of Metal Oxide Nanoparticles (TiO2, Al2O3, SiO2 and CeO2) on Anammox Process: Performance, Microflora and Sludge Properties. Bioresour. Technol. 266, 11–18. doi:10.1016/j.biortech.2018.06.052
Zhang, Z.-Z., Zhang, Q.-Q., Xu, J.-J., Deng, R., Ji, Z.-Q., Wu, Y.-H., et al. (2016). Evaluation of the Inhibitory Effects of Heavy Metals on Anammox Activity: A Batch Test Study. Bioresour. Technol. 200, 208–216. doi:10.1016/j.biortech.2015.10.035
Keywords: anaerobic ammonium oxidation, heavy metals, performance, mechanism, promotion, inhibition
Citation: Ren Q, Gao J and Wang C (2022) Effects of Heavy Metals on the Performance and Mechanism of Anaerobic Ammonium Oxidation for Treating Wastewater. Front. Chem. Eng. 4:851822. doi: 10.3389/fceng.2022.851822
Received: 10 January 2022; Accepted: 09 May 2022;
Published: 23 June 2022.
Edited by:
Shang-Tian Yang, The Ohio State University, United StatesReviewed by:
Joan Dosta, University of Barcelona, SpainCopyright © 2022 Ren, Gao and Wang. This is an open-access article distributed under the terms of the Creative Commons Attribution License (CC BY). The use, distribution or reproduction in other forums is permitted, provided the original author(s) and the copyright owner(s) are credited and that the original publication in this journal is cited, in accordance with accepted academic practice. No use, distribution or reproduction is permitted which does not comply with these terms.
*Correspondence: Junling Gao, anVubGluZ2dhb0Bmb3htYWlsLmNvbQ==
†These authors have contributed equally to this work and share first authorship
Disclaimer: All claims expressed in this article are solely those of the authors and do not necessarily represent those of their affiliated organizations, or those of the publisher, the editors and the reviewers. Any product that may be evaluated in this article or claim that may be made by its manufacturer is not guaranteed or endorsed by the publisher.
Research integrity at Frontiers
Learn more about the work of our research integrity team to safeguard the quality of each article we publish.