- Division of Energy Processes, Department of Chemical Engineering, KTH Royal Institute of Technology, Stockholm, Sweden
The agriculture sector contributes to ∼40% of methane emissions globally. Methane is also 28 times (Assessment Report 5) more potent greenhouse gas than CO2. In this study, we assess the impact of measures for mitigating methane emissions from the agricultural sector on the achievement of all the 17 United Nations’ Sustainable Development Goals (SDGs). A keyword literature review was employed that focused on finding the synergies and trade-offs with non-fossil methane emissions from the agricultural sector and respective SDGs’ targets. The results were in broad consensus with the literature aimed at finding the relationship between SDGs and measures targeting climate change. There is a total of 88 synergies against eight trade-offs from the 126 SDGs’ targets that were assessed. It clearly shows that measures to mitigate methane emissions from the agricultural sector will significantly help in achieving the SDGs. Since agriculture is the primary occupation and the source of income in developing countries, it can further be inferred that methane mitigation measures in developing countries will play a larger role in achieving SDGs. Measures to mitigate methane emissions reduce poverty; diversify the source of income; promote health, equality, education, sanitation, and sustainable development while providing energy and resource security to the future generations.
1 Introduction
The Paris agreement is aimed at limiting the temperature rise to 2°C while pursuing ambitious efforts to limit its increase to 1.5°C, compared to the preindustrial levels (United Nations/Framework Convention on Climate Change, 2015). Methane has contributed to approximately 0.5°C rise in temperature in the past decade (IPCC, 2021). The agricultural sector is the highest contributor, accounting for ∼40% of total methane emissions in the world (United Nations Environment Programme, 2021a). Greenhouse gas (GHG) emissions like methane and nitrous oxide from the agricultural sector are usually overlooked and underestimated for many reasons that include 1) its importance in the context of food security, 2) distributed and dilute emission sources, and 3) difficulty in quantifying the emissions. There are several national and international bodies involved in tracking the emissions of the non-CO2 GHGs, among which International Methane Tracker (IEA, 2022) and International Methane Emissions Observatory (United Nations Environment Programme, 2021b) are some of the notable ones. Methane, from the agricultural sector, is primarily released from ruminants (cattle, sheep, etc.) through a process termed enteric fermentation (Gerber et al., 2013a). Nitrous oxide is emitted from the soil through microbial processes, nitrification and denitrification, from fertilizers used in agriculture (Mosier et al., 1996). Combined production of these gases can also be observed in low oxygen environment of animal manures which includes poultry farms, dairy farms, beef feedlots, entry and exit points in biogas plants, etc. (Gerber et al., 2013a).
The technologies available to mitigate methane can be broadly classified, based on their point of application in the emissions timeline, into preventive and reactive measures. The preventive measures as the name suggest work by preventing the release of methane in the first place. Some of these preventive measures in agriculture include the usage of various dietary supplements, manure handling and treatment strategies, and the measures to optimize fertilizer usage (Gerber et al., 2013a; Gerber et al., 2013b). The other category, reactive measures, acts upon the methane after its release into the environment. However, it is hard to mitigate these types of emissions as they are quickly diluted with other gases in the surroundings. One of the possibilities to address the emissions after their release is to develop and use technologies similar to direct air capture (DAC) (Sanz-Pérez et al., 2016) that separate CO2 from air at very low concentrations (∼400 ppm). In the case of non-CO2 greenhouse gases like methane, both separation- and conversion-based technologies have the potential to remove methane from the air. In addition, the removal of methane can be combined with the existing DAC technologies for CO2, to remove multiple greenhouse gases, a concept proposed by Sirigina, Goel, and Nazir (Sirigina et al., 2021). These technologies have a higher potential when placed very close to the source of emissions. In the agricultural sector, the ventilation stables (10–300 ppm methane) and manure storage (140–28,000 ppm methane) (Stolaroff et al., 2012) are potential spots to implement these technologies. Implementation of the measures depends on the support from respective stakeholders and policymakers. It is thus beneficial, beforehand, to study how non-fossil methane removal from the agricultural sector interacts with society and the environment to ensure sufficient and appropriate considerations have been made with respect to achieving United Nations’ Sustainable Development Goals (SDGs).
SDGs were introduced to act as an important milestone, by 2030, to call for global action on ending poverty, improving the livelihood prospects of people, and establishing a sustainable production-consumption pattern aimed at protecting the planet. There are several studies broadly relating agriculture to sustainable development goals in the body of literature (Haines et al., 2017; Setboonsarng and Gregorio, 2017; Nuthalapati et al., 2018; Ladha et al., 2020). However, to the best of our knowledge, no study specifically assessed the impact of mitigating non-fossil methane release from agriculture on sustainable development goals. With the help of the SDG framework, we qualitatively assess the impact of mitigating non-fossil methane from agriculture on various societal, economic, and environmental aspects and identify potential obstacles in the future implementation of its measures. This study presents an assessment of non-fossil methane emissions mitigation from the agricultural sector for the achievement of SDGs–synergies and trade-offs. The study considered all the activities (both preventive and reactive) aimed at mitigation for the assessment. Discussions in this study can be a basis to critically argue for developing and implementing methane emissions mitigation technologies.
2 Methodology
2.1 Scope
In this section, we outline the system and its scope considered for assessing the impact on SDGs. Figure 1 shows the schematic of the scope of the assessment. The impact of the measures for methane emissions mitigation will be considered as a system, and the boundary span from the actions taken to mitigate these emissions to their implications on the achievement of SDGs. We assume any measure (technological, behavioral, operational, etc.) that will result in mitigating methane emissions (both preventive and reactive measures) from the agricultural sector is within the scope. However, as depicted in Figure 1, the energy and material requirements to build these technologies are not within the scope of the system. The material and energy requirements were excluded because there are a wide range of technologies that can be implemented to mitigate non-fossil methane from agriculture. Since the study is conducted in a technology-neutral scenario, assessment of material and energy requirements—which vary considerably within a given technology—cannot be generalized over all the available technologies/measures for mitigation. For instance, a DAC-based technology for non-fossil methane mitigation has a completely different set of material and energy requirements when compared to measures using feed additives or more nature-based measures such as restoring terrestrial ecosystems. In such cases, a common estimate for these requirements cannot be assumed. Hence, they are excluded from the scope; however, we strongly acknowledge their impact on SDGs but they should be assessed on a case-to-case basis. Similarly, spatial and temporal dimensions for methane emissions are not within the scope. The results have been generalized without taking a specific geographic location, and we consider that the sources of methane emissions from the agriculture sector around the world are the same. The assessment was carried out only for the agricultural sector.
2.2 Methods
Implications of the outcome from implementing measures (non-fossil methane emissions mitigation from the agricultural sector) on SDGs and their targets were identified. In addressing the interlinkages between SDGs and the measures taken to mitigate non-fossil methane, the methodology of Fuso Nerini et al. (2018) was adopted. To map the synergies and trade-offs between energy and the SDGs, Fuso Nerini et al. (2018) performed a literature review to identify published evidence between the SDG targets and the actions to promote SDG 7. Their results were later characterized using a consensus-based expert elicitation process. However, in this study, the consensus-based expert elicitation was not performed.
A key-word based literature review was performed for methane emissions mitigation measures in the agricultural sector and their relation with each SDG and the respective targets. All 17 SDGs, and their 126 targets (without their means of implementation targets), were analyzed. From the research results corresponding to each of the analyzed targets, we answered the following question: Does implementing mitigation measures for non-fossil methane from agriculture present a synergy or/a trade-off in achieving that target? If there is a direct linkage in the literature on synergy and/or trade-off between mitigating non-fossil methane and the respective target, they were correspondingly marked in the excel sheet used for the assessment, which is attached as Supplementary Material with this article. However, it so happened that during the research, there were often indirect and reverse synergies and/or trade-offs that could be associated between the question and the SDG target. An indirect linkage is then marked in the following cases:
(i) A linkage is not established directly but can be inferred from the literature. For example, considering the SDG target 13.3 (improve education, awareness-raising, and human and institutional capacity on climate change mitigation, adaptation, impact reduction, and early warning), working on non-fossil methane mitigating technologies does not directly impact the institutional capacity as required by the target. However, implementing methane mitigating technologies can have an indirect effect on promoting the institutional capacity for climate change mitigation, adaptation, and impact reduction (Venkatram, 2021). An indirect interlinkage was marked in this case.
(ii) Only a partial linkage with the target is found in the literature. This is for the cases where only a part of the target is found to have an interaction with the question.
(iii) Several causal interactions are needed to mark a direct linkage to the target. This can be exemplified using target 14.2 (by 2020, sustainably manage and protect marine and coastal ecosystems to avoid significant adverse impacts, including strengthening their resilience, and taking action for their restoration in order to achieve healthy and productive oceans) and the implementation of non-fossil methane mitigating technologies. It is shown in the literature that climate change from anthropogenic emissions could possibly increase methane emissions from marine and coastal ecosystems, creating a positive feedback loop (Al-Haj and Fulweiler, 2020). The causal chain linkage for this to happen is extremely complex, although, a certain linkage was documented in the literature (Al-Haj and Fulweiler, 2020). An indirect interaction is marked in these cases.
A reverse synergy and/or trade-off is marked for cases when the causal relation is inversed, i.e., promoting the target promotes the question. Reverse interactions are not explicitly searched for, rather they were identified in the process of finding interlinkages between the question and the target. Additionally, a reverse linkage does not automatically imply a direct linkage, hence marking the linkage as such is only justified. Reverse interactions can be understood as answering the following question: Does achieving/promoting the target present synergy and/or a trade-off with mitigating non-fossil methane from agriculture?
A particular target can have all the possible interactions with the question, i.e., synergies and trade-offs. The results from peer-reviewed and grey literature (conference proceedings, government reports, etc.) were considered to mark published evidence for potential synergy and trade-off with the target. One piece of published evidence was regarded enough to mark an interaction. No quantitative ranking for the synergies or trade-offs was assigned. This means even with multiple synergies being reported in the literature, it was just marked as one single synergy. A similar methodology was employed in analyzing trade-offs. Citing a lack of literature for synergy and/or trade-off, a null relationship (no impact) was marked for the respective target. That does not mean that such a relationship does not exist; it only means that it is not yet documented or was not found in our research. This is not a systematic literature review as that can be extremely complex considering that we need to identify at least 126 interactions. An exclusion criterion was therefore not set up. For an interaction to exist, it is sufficient that we find at least one piece of published evidence that mitigating non-fossil methane promotes/poses a trade-off with the target. It is not required that such a measure/technology is currently in operation and has a documented experience in mitigation. Results were assimilated into three pillars of sustainable development as adopted by Vinuesa et al. (2020): society, economy, and environment.
Means of implementation targets, under each SDG, describe the necessary resources (including technological, financial, skill-related, and institutional) required for the successful implementation of the respective goal. It is to be noted that means of implementation targets for the first 16 SDGs were not included in this assessment. SDG 17 aims to strengthen the means of implementation and revitalize the global partnership, thereby not having means of implementation targets separately.
2.3 Limitations of the Study
The analysis was done at a broader level with respect to mitigation of methane emissions from the agricultural sector, without regard to a specific location. Therefore, the relations specified herein are not comprehensive and would merit a region-specific study to draw inferences for a particular locality. Only economic aspects of the technology were considered leaving out the material aspects of implementing the technology. For example, methane removal from the air in ventilation stables, through conversion to CO2 and H2O, require the use of rare-earth metals as a catalyst to catalyze the oxidation reactions (Sirigina et al., 2021). This would require mining metals and the employment of more circular techniques to ensure sustainability. In addition, mining and importing metals were reported to have a lot of implications on the achievement of SDGs (Nansai et al., 2019; Jane and Michael, 2021). The availability of these materials could add to the bottleneck of processes in implementing these technologies. The energy requirement for implementing these technologies was not assessed. An increase in energy consumption because of climate mitigation/adaptation technologies could further increase the burden in developing countries resulting in trade-offs. It is thus paramount to consider efficiency improvement while studying similar technologies. However, we acknowledge the impact of energy and material on SDGs, and it needs to be looked at when considering the impact of a particular technology on SDGs. Considering the broader aim of this study to look at all the measures that can mitigate non-fossil methane emissions from agriculture, the inclusion of individual material and energy interactions can quickly make the study more complex.
The study was carried out in a way to prevent interconnection with CO2 emissions reduction—thus in a broad manner targeting climate change—while accounting for synergies and trade-offs. It broadly reflects the implications of mitigating climate change on SDGs but has been narrowly characterized to just consider non-fossil methane emissions from the agricultural sector. In addition, when the interactions between more GHGs (CO2, CH4, and N2O) and the target take place, untangling the relationship between only CH4 and the target is hard, and in that case, an indirect interlinkage was marked (only if published evidence is found). It is imperative that this study might carry some over- or/and underweighting in identifying the connections. This, although, can be countered through the usage of a quantitative basis in identifying the connections with the SDGs.
SDGs and their targets are interconnected with each other through complex interlinkages (Bali Swain and Ranganathan, 2021). In this assessment, each SDG and its target were assessed independently without exploring the interlinkages between them. Additionally, the implications of SDGs have to be temporally resolved owing to the short life span of methane in the atmosphere compared to CO2.
3 Results and Discussions
All 17 SDGs were assessed for the implications of non-fossil methane emissions mitigation measures in the agricultural sector. In our assessment, we found a total of 88 synergies (21-direct, 23-indirect, and 44-reverse synergies) with eight trade-offs (4-direct and 4-reverse trade-offs) from the 126 targets that were assessed. Means of implementation targets were excluded from our assessment. The ratio of synergies to trade-offs (only direct and indirect) indicate that mitigating methane emissions from the agricultural sector significantly enables the achievement of the SDGs. Similarly, from the ratio of reverse synergies to trade-offs, we can qualitatively describe a positive correlation between achieving the SDGs and non-fossil methane mitigation in the agricultural sector. It is to be noted that interpretation of these results is only valid within the purview of the scope considered in this assessment. The compiled data on the mapping of synergies and trade-offs, and the detailed explanations for each target, can be found in the Supplementary Material that is published along with this study. However, we briefly discuss the main results in the following subsections for the three different categories of SDGs: the society, economy, and environment. Figure 2 depicts the synergies and trade-offs of methane emissions mitigation in the agricultural sector in achieving all the 17 SDGs.
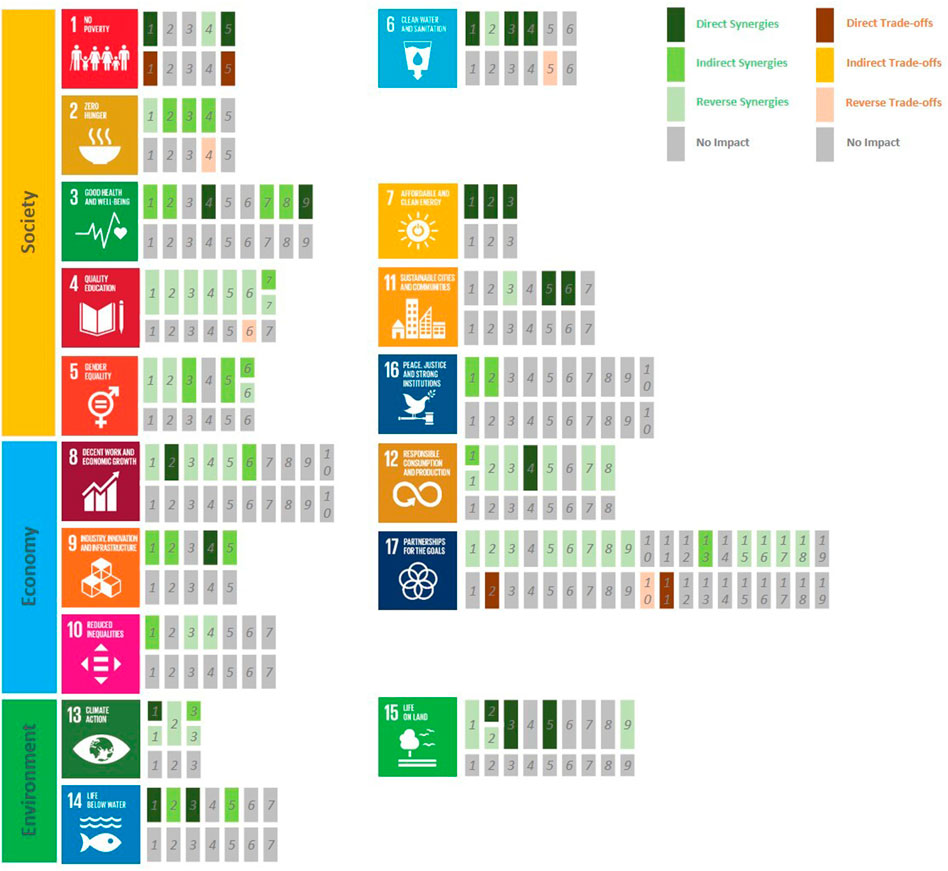
FIGURE 2. Synergies and trade-offs of mitigating non-fossil methane emissions from the agricultural sector on 17 UN Sustainable Development Goals. Graphics in the figure have been adapted from Nerini et al. (2019).
3.1 Impacts on Society
Society is represented by SDGs 1–6,7,11, and 16 in the UN SDG framework. In order to differentiate between various interlinkages, the results are categorized by whether mitigating non-fossil methane poses a direct, indirect, or reverse interaction with the analyzed targets.
3.1.1 Direct Interactions
The world’s poorest will be drastically affected by climate change due to the change in extreme temperatures and variability in hydrological cycles. Climate-related extreme events such as droughts and floods will have negative effects on societies dependent on agriculture as their primary source of income (Hall et al., 2014). Therefore, actions targeting climate change, predominantly methane emissions, in developing countries reduce the vulnerability of the poor to extreme climate-related events, in synergy with SDG 1 (No Poverty) (Fatma, 2002; Pradel et al., 2006; Hall et al., 2014; Satoh et al., 2017; Byers et al., 2018). A large share of agriculture dominant countries are dependent on cattle or crop farming for their livelihood but these activities result in a large share of methane emissions to the atmosphere. Using technologies to mitigate methane emissions, will limit the emissions even if production is scaled up leading to a reduction in poverty. The use of more efficient technologies in dairy farming was shown to reduce poverty (Pradel et al., 2006). It is noteworthy to point out that in the same study, although emissions per unit of milk were found to be reduced, overall emissions increased due to an increase in the overall production (Pradel et al., 2006). It is necessary that these technologies are implemented to build the resilience of the people below poverty levels (Target 1.5). However, those who are already below poverty levels will find it economically challenging to implement new mitigation measures. This poses a direct trade-off to implement these methane mitigating measures (Targets 1.1 and 1.5). Therefore, policy intervention is necessary to enable methane emissions mitigation in the agricultural sector (FAO, 2016). There are direct interactions with SDG 3 (good health and well-being) with respect to targets 3.4 and 3.9. A study aimed at measuring the impact of climate change-induced increase in pollutant concentration (PM 2.5 and ground-level ozone) on premature mortality has shown a very strong correlation between them (Target 3.4). Additionally, the same study showed that methane release leads to increased background ozone and later concluded the importance of methane reduction to slow the climate change while also reducing the direct risks it poses to human health (Fang et al., 2013). Preventing the release of these methane emissions, as a result, will reduce premature mortality, enabling target 3.4 (Fang et al., 2013; Silva et al., 2016; Garcia-Gonzales et al., 2019). Methane avoidance projects were shown to significantly improve local health conditions, posing a direct synergy to target 3.9. In the last century, water demand, globally, has increased by 600% and is likely to experience a significant increase in the coming decades. Agriculture accounts for 70% of total water consumption in the world. The provision of water and wastewater treatment is likely to play a key role by 2050 (Boretti and Rosa, 2019). Methane is released from a large share of processes during the treatment and management of domestic and industrial wastewater. Advancements of treatment techniques can mitigate methane release while increasing the availability of fresh water and water for sanitation (Targets 6.1, 6.3) (Bogner et al., 2008; Caniani et al., 2015; Hwang et al., 2016; Parravicini et al., 2016; Boretti and Rosa, 2019). Water conservation alternatives to the flooding of rice paddies, for example, alternate wetting and drying of rice fields, can reduce methane emissions by 45%. In addition, emissions from the livestock sector can be reduced by ∼41% by adopting sustainable practices (improved feed quality, health management of livestock, etc.), thereby promoting target 6.4 (FAO, 2016). Waste-to-energy (WTE) technologies provide opportunities to utilize agricultural waste in its conversion to energy while preventing the release of methane from waste (SDG 7) (AlQattan et al., 2018). Waste biomass is used to produce methane to provide access to modern energy services in rural areas, preventing its release into the environment (Target 7.1) (Balachandra, 2012; Balachandra, 2013a; Balachandra, 2013b). Waste from various agricultural sources is deposited in landfills (Alatzas et al., 2019). Methane, constituting about 55–60% of landfill gas, can be utilized to increase the share of renewable energy in the global energy mix (Target 7.2) (Al-Dabbas, 1998; Chynoweth et al., 2001; Sims, 2004; Scheutz et al., 2009). Higher feed quality and improvement in feed digestibility results in significantly lower enteric methane emissions from the ruminants (Target 7.3) (Johnson and Johnson, 1995; Karakurt et al., 2012; Baptista et al., 2013; Mottet et al., 2017). Most of the developing countries depend on agriculture as the primary source of their livelihood. Climate change-induced extreme weather events could increase deaths caused by disasters while also causing direct economic losses, and this effect can be dominant in developing countries (Aggarwal, 2008; Rossati, 2017). Measures promoting the reduction of emissions (methane accounts for approximately half of the total GHG emissions in CO2 eq. from agriculture) could at least prevent the future climate-related events and their effects (Target 11.5). Waste-to-energy technologies prevent the release of methane emissions improving the air quality while also producing energy (AlQattan et al., 2018).
3.1.2 Indirect Interactions
Health benefits from preventing methane emissions have been reported in the literature (FAO, 2016). Reduced malnutrition is an indirect benefit of implementing improved technologies that reduce methane emissions (Target 2.2) (Pradel et al., 2006). Improvement in farmers’ productivity and income is observed (Target 2.3) (FAO, 2016). Non-fossil methane mitigation could promote improved and sustainable agricultural production systems, and resilient agricultural practices whilst strengthening the capacity for adaptation to climate change and its effects (Target 2.4) (FAO, 2016). Mitigating methane emissions, by shifting away from solid biomass (from agricultural sources) usage for household consumption (such as cooking), and by improving energy efficiency, could result in the energy being saved to be used for providing access to essential health-care services, reducing, for example, maternal mortality (pregnancy-related death). This is reported to further increase the access to universal healthcare services (SDG 3) (Smith et al., 2013). Sustainable development is achieved through the promotion of methane mitigating technologies (Target 4.7). Measures to mitigate climate change require consideration of gender equity and social equality which in turn promotes a harmonious society characterized by lower violence (Target 5.3) (Ursula Oswald Spring 2019). Increasing equality will reduce violence and harmful practices (child marriage, early and forced marriage, and female genital mutilation) against women, fostering their participation in leadership roles to achieve climate targets (Targets 5.3, 5.5) (Irene, 2002; Farhana, 2014; Sophia et al., 2020).
With respect to SDG 16 (peace, justice, and strong institutions), measures to mitigate methane emissions from the agricultural sector will prevent drastic effects of climate change, which otherwise threatens the livelihood in developing countries (Rodriguez et al., 2020). Climate change can have varied effects on women and children ranging from increased violence (economic stress due to climate shocks and increase in aggression due to higher temperatures), abuse, exploitation, and related deaths (Targets 16.1, 16.2) (Hanna and Oliva, 2016). A direct interlinkage between mitigating methane emissions and the reduction of violence or ending abuse and exploitation of children is hard to establish. Nevertheless, there is clear documented evidence (Hanna and Oliva, 2016; Rodriguez et al., 2020) showing the increased effects of violence, affecting targets 16.1 and 16.2, because of climate change. Therefore, we can ascribe at least some prevention of increased violence in the future due to impending climate change to the current implementation of mitigation activities. An indirect linkage is most suitable here.
3.1.3 Reverse Interactions
Working on emissions reduction requires ownership and control over the resources (land, finances, natural resources, etc.). There is published evidence on the very limited role women could play in mitigation in developing countries where they are socially backward compared to men (no ownership and control over resources) (Fatma, 2002). Similar inferences could be drawn to men or people in general who have no ownership of land, natural resources, or access to technology or financial services; they play a very limited role in mitigation measures. Promoting target 1.4 can therefore promote the implementation of methane mitigating technologies, marking a reverse synergy. With respect to SDG 2 (zero hunger), loss of nutritional content of foods from the effects of climate change (in this case due to methane emissions) can trigger health issues in developing countries (FAO, 2016). Working on the target supports the implementation of mitigation technologies. With respect to SDG 4 (Quality Education), mitigating methane emissions requires promoting necessary education to people, achieved through improvement of education standards leading to better opportunities (Tajul Ariffin and Yogeeswari, 2019; Subramaniam and Masron, 2020). Education related to environmental studies has been identified as a necessity to achieve climate targets (Target 4.6) (Jalil, 2014; Williamson, 2017). Efforts to achieve a more equal distribution of power through, for instance, equitable income distribution, wider literacy, and greater political liberties and civil rights will positively help in developing measures to improve the environment, especially in developing countries (Target 4.7) (Torras and Boyce, 1998 in Supplementary Material). Overall, ensuring inclusive and equitable quality education is shown to instill a strong sense of environmental awareness. This increased awareness is shown to encourage the measures aimed at conserving the environment. On the other hand, education is shown to worsen the methane emissions in gulf countries (Jalil, 2014). Women with low socioeconomic status will be at higher risk from climate change disasters (dependency on natural resources for livelihood, most of the agriculture work is carried out by women, responsibility for the collection of fuel and water, etc.), thus requiring greenhouse gases mitigation policies to benefit/include women/gender perspective. This reduces gender discrimination while increasing equality, promoting SDG 5 (Gender Equality) (Eric and Thomas, 2007; Geraldine, 2009). Emissions from open defecation pits can be eliminated through equitable sanitation techniques. This in turn could promote the development of waste water technologies, offering co-benefits such as treating wastewater from agriculture (target 6.2) (Bogner et al., 2008; Reid et al., 2014). Sustainable urban settlements with better waste management have lower methane emissions from landfills (Target 11.3) (Ahmad and Choi, 2010).
Overall, mitigating non-fossil methane emissions from the agricultural sector will strongly promote SDGs 2, 3, 4, 5, 6, 7, 11, and 16 while acting as an enabler for SDG 1, with 39 synergies against five trade-offs.
3.2 Impacts on Economy
The economic dimension of the UN SDG framework is carried by SDGs 8–10, 12, and 17. We present here the direct, indirect, and reverse interlinkages with SDG economic indicators and non-fossil methane emissions mitigation from agriculture.
3.2.1 Direct Interactions
Non-fossil methane mitigation from agriculture poses only three direct synergies with the assessed targets from the economy.
Promoting methane emission reduction measures could result in technology development through, for example, biogas production and help increase diversifying farmers’ income (FAO, 2017). Food and agricultural organization (FAO) Climate-smart Agriculture (CSA) is targeted at productivity increase and adaptive capacity whilst reducing emissions (FAO, 2017). All these actions are a direct synergy to target 8.2. Reducing methane emissions through retrofits (e.g., regenerative thermal oxidizer) to address the leakages in biogas plants, or advancing the technologies in wastewater treatment, promotes environmental sustainability in the infrastructure (Target 9.4) (Kvist and Aryal, 2019). Target 12.4 is achieved through emissions reduction as a result of implementing effective management techniques for food waste and manure that is used in agriculture (Adhikari et al., 2006; Montes et al., 2013). Reorientation of funds to climate change mitigation that were meant for poverty alleviation could pose a direct trade-off to target 17.2 (Agrawala et al., 2004; Lokshall, 2006; Michaelowa and Michaelowa, 2007; Watts et al., 2015; Clapp and Pillay, 2017; Massé et al., 2020).
3.2.2 Indirect Interactions
Methane avoidance projects were shown to improve employment at municipality levels (Yadira, 2019). Since target 8.6 explicitly requires the reduction of youth unemployment, methane avoidance projects can have at least an indirect synergy with the target. With regards to SDG 9, implementing mitigation measures can indirectly promote resilient infrastructure, and sustainable industrialization boosting the economic status of the society and the country (Blignaut et al., 2008; Singh et al., 2018). Furthermore, it could open new possibilities in research on wastewater treatment, biogas production from household and agricultural waste, and biogas plants, thereby leading to innovation and attracting public funding in the sector (Target 9.5) (Makisha, 2016; Taseli and Kilkis, 2016). Methane avoidance projects were shown to improve income at municipality levels, progressively achieving economic growth (Target 10.1) (Yadira, 2019). An interesting thing to note here is the interlinkage between the targets 10.1 and 8.6, i.e., achieving one target at least partially achieves the other. Several such interlinkages exist between the targets in the SDG framework, some acting as enablers while others inhibiting the other targets in the framework. It is therefore important to choose a project in such a manner so as to not only maximize the overall positive interactions with the targets but also minimize the internal negative interactions. Ensuring sustainable consumption and production patterns (SCP), and mitigating methane emissions from increased waste by establishing a waste management hierarchy (refuse, reduction, reuse, recycle, and recovery), will help both developing and developed countries (Target 12.1) (Ari and Şentürk, 2020). For this, and some other targets in the study, an interaction is present in both ways (direct or indirect and/or reverse). Working on SCP promotes non-fossil methane mitigation technologies (solid waste management where part of the agricultural waste is dumped), and at the same time working on methane mitigation technologies (solid waste management) can help deliver this target. An increase in the productivity of agricultural land (resource use) could result in higher macroeconomic stability. This could promote the target and the methane mitigating technologies (Riahi et al., 2007).
3.2.3 Reverse Interactions
A study was carried out to test the validity of the environmental Kuznets curve (EKC) hypothesis for methane emissions–economic growth in six Association of Southeast Asian Nations (ASEAN) countries (Adeel-Farooq et al., 2020). The study confirmed the validity of the EKC curve hypothesis, i.e., methane emissions increased until a threshold economic growth, later following a downward trend. Sustaining the economic growth achieved, as required by target 8.1, can only be achieved by implementing increased emissions control measures (Adeel-Farooq et al., 2020). Implementing development-oriented policies was associated with lower methane emissions through the implementation of mitigation measures, for example, sustainable farming (Target 8.3) (FAO, 2014). Improved resource efficiency, sustainable consumption, and production policies can be associated with up to 90% lower GHG emissions (Target 8.4) (Oberle et al., 2019). Boosting labor productivity, through climate mitigating technologies, can provide opportunities for new employment (Target 8.5) (FAO, 2014). However, implementation of methane emissions mitigation measures often comes with requiring policy interventions to share the economic burden associated with them.
Reducing global inequality can improve environmental outcomes (Targets 10.3, 10.4) (Berthe and Elie, 2015; Rao and Min, 2018).
Sustainable agriculture aims at the efficient use of arable land and water with improved agricultural practices while mitigating methane release from the sector, promoting target 12.2 (Meena et al., 2019). Reducing food waste minimizes resources used in food production while also reducing emissions from this waste (with respect to target 12.3) (Hall et al., 2009; Ryzhko and Aliksievich, 2014). Practicing prevention, reduction, recycling, and reuse can reduce waste generation and the resulting methane emissions (Target 12.5) (Ari and Şentürk, 2020). Promoting public procurement practices for organic-based foods and foods cultivated through integrated production practices reduces the emissions resulting from food production (Target 12.7) (Cerutti et al., 2016). There is also a lack of awareness in the general public about the link between the type of food consumption and its environmental impact (for example meat consumption) (Macdiarmid et al., 2016). Improving public awareness in this regard will not only make the general public more environmentally conscious but also assists in choosing appropriate management practices throughout the life cycle of farming and consumption (Target 12.8).
Strengthened domestic resource mobilization boosted international support to developing countries in terms of finance, resource, and technology sharing, and assisted them in building capacities to develop climate positive measures (Target 17.1) (RSIF, 2021). An increase in global trade and exports increases the nitrous oxide and methane emissions from the agriculture sector (partly because it is more pesticide and fertilizer intensive related to national market production). Therefore, policies targeting mitigation will penalize trade lowering the exports from developing countries (Target 17.11) (Hussein et al., 2013; Drabo, 2017; Haider et al., 2020). Climate financing, green bonds, mitigation, and adaptation funds could replace/redirect official development assistance (ODA) funds, originally meant for poverty reduction, to address climate change (Targets 17.2, 17.3, 17.5) (Agrawala et al., 2004; Lokshall, 2006; Michaelowa and Michaelowa, 2007; Watts et al., 2015; Clapp and Pillay, 2017; Massé et al., 2020). Enhanced international cooperation through knowledge and technology sharing provides opportunities to work on climate issues in a collective way (Targets 17.6, 17.7, 17.17) (Lu et al., 2020). An operational technology bank that helps in capacity building can horizontally deploy best management practices to mitigate methane emissions (Targets 17.8, 17.18) (Ngunga et al., 2020). International support in terms of finance or technology could help in implementing technologies for mitigation (Targets 17.9, 17.16) (Boyd, 2012; Trung et al., 2020). Implementing a rule-based, open, and equitable multilateral trading system might hinder the measures targeting climate change (Target 17.10) (Elbehri et al., 2011). Production from agriculture for exports has higher CH4 emissions when compared to the production for local markets (Hussein et al., 2013; Drabo, 2017; Haider et al., 2020). Implementing policies that mitigate these increasing emissions increases the production costs and reduces the exports (Hussein et al., 2013). This is seen as a direct trade-off to target 17.11. Policy coherence between SDGs and respective national/international policies (Duraiappah and Bhardwaj, 2007) should be implemented to achieve methane reduction through appropriate measures (17.15).
Overall, mitigating methane emissions will improve the economy, albeit indirectly, of developing countries where agriculture is the primary source of income with three trade-offs against 34 synergies. It strongly promotes achieving SDGs 8, 9, 10, and 12, while acting as an enabler for SDG 17.
3.3 Impacts on Environment
In this section, we discuss the impact of mitigating methane emissions on SDGs 13, 14, and 15, which are climate action, life below water, and life on land, respectively. Methane emissions contribute significantly to global warming and therefore any mitigation measure will have a climate-positive impact (IPCC, 2021).
3.3.1 Direct Interactions
Climate-smart agricultural practices to strengthen resilience and adaptive capacity to climate-related hazards can additionally mitigate methane emissions in developing countries. Similarly, climate-resilient livestock farming has been shown to reduce methane emissions (Rashamol and Sejian, 2018; Venkatramanan et al., 2019). Due to the interconnected nature of SDGs, it is hard to ascertain whether promoting this goal enables methane mitigation or vice versa as both the statements appear equally valid. Effects of climate change are imminent and climate-smart agriculture strengthens resilience and adaptive capacity to climate-related hazards while preventing further damage to the environment (Target 13.1). Following integrated manure management strategies has manifold benefits including reduced environmental impact (from reducing methane emissions), marine eutrophication, terrestrial acidification, particulate matter formation, and high N use efficiency (Target 14.1) (De Vries et al., 2015). Ocean acidification is minimized and adverse impacts on marine and coastal ecosystems can be prevented, promoting target 14.3 in the Supplementary Material (Bach et al., 2016). Practicing the best management techniques in the conversion of terrestrial ecosystems to agricultural lands and on extremely degraded soils restore their natural capability to act as methane sinks (Target 15.2) (Lal et al., 2018). Improved yield from agriculture lowers methane emissions and also frees up the land used (Target 15.5) (Awuchi et al., 2020). This land could be used to rebuild the habitat for the species threatened with extinction from agriculture.
3.3.2 Indirect Interactions
Improving education, awareness, and institutional capacity are crucial to implementing methane emissions mitigation measures in the agricultural sector (Target 13.3) (Venkatram, 2021). Methane emissions from vegetated coastal ecosystems increase due to climate change. Methane mitigation technologies can prevent further climate change and can be indirectly linked to protect them from further impacts from climate change (Targets 14.2, 14.5) (Al-Haj and Fulweiler, 2020).
3.3.3 Reverse Interactions
Increased resilience and adaptive capacity to climate-related hazards, integrating climate change measures into national policies, and improving education and climate awareness were all associated with implementing methane mitigating measures especially in developing countries (Targets 13.1,13.2 and 13.3) (Prasad and Sejian, 2015; Rashamol and Sejian, 2018; Venkatramanan et al., 2019; Venkatram, 2021). Policy intervention is necessary to adopt climate-friendly practices in developing countries where agriculture is a primary component of the economy in order to reduce methane emissions (Target 13.2) (Prasad and Sejian, 2015). The conversion of terrestrial ecosystems to row-crop agriculture was marked by the reduction of methanotroph diversity in the soils. Consequently, the capability of the soils to act as methane sinks was reduced. Mitigation of methane emissions from the agricultural sector will require managing the land use conversion to ensure the conservation of local microbial biodiversity, enabling target 15.1 (Levine et al., 2011). Conversion of terrestrial ecosystems to agricultural lands reduces their ability to act as GHGs sinks (Lal et al., 2018). Implementation of sustainable management of all types of forests restores their natural capacity as a sink and can be seen as a methane mitigating measure (Allen et al., 2009; Lal et al., 2018).
A detailed explanation of the interlinkages for all the assessed targets can be found in the Supplementary Material of this study.
Since methane is a GHG, the implementation of its mitigation technologies ought to interact strongly with the environmental dimension of the SDG framework. Correspondingly, we found 15 synergies with no trade-offs with SDGs 13,14, and 15 in the environment. We would like to reemphasize that the absence of trade-offs does not necessarily exclude the negative interactions with the environment or with the other categories. It only means there is an absence of published evidence within the scope of this study. Expanding the study to include, for instance, materials could be an inhibitor to many of the SDGs. An explanation for this is due to the mining associated with materials used as catalysts in some of the methane mitigating technologies (Nansai et al., 2019). This, although, is dependent on the specific technology in relation to the study.
4 Conclusion
Implications of the SDGs and their targets for mitigating non-fossil methane emissions from the agricultural sector are presented in this study. We conclude that synergies with SDGs for mitigating non-fossil methane emissions from the agricultural sector far outweigh the trade-offs showing clear potential in implementing mitigation measures. Mitigating non-fossil methane emissions from the agricultural sector can promote the achievement of all the 17 SDGs in developing countries where agriculture is the primary source of income and livelihood. The economic aspect of implementation is a major trade-off for developing countries. Suitable policy intervention will be required to overcome the identified economic trade-offs in implementing measures to mitigate methane emissions from agriculture. This can promote many of the SDGs and as a result enrich the living standards in developing countries. It is worth mentioning here that interconnections between different SDGs are not presented. However, studying the interconnections can give a more detailed analysis of impacts on SDGs. In addition, this study broadly considered all the possible technologies for implementing mitigating measures but excluded the social, material, and energy aspects of their implementation. A more comprehensive study is warranted in order to examine the relationship between a specific technology and its impact on SDGs.
Data Availability Statement
The original contributions presented in the study are included in the article/Supplementary Material; further inquiries can be directed to the corresponding authors.
Author Contributions
DSS performed the literature review and identified the synergies and trade-offs. Both DSS and SMN had detailed discussions about the content of the manuscript, reviewing, and editing it. Both the authors contributed to conclusions derived from the study.
Funding
The work is part of the project “Energieffektiv negativa utsläpp från jordbrukssektorn” [In English: Energy-efficient negative emissions from agriculture and farming] (project number 50340-1) funded by Energimyndigheten (Swedish Energy Agency). This work is also cofinanced by the Swedish governmental initiative StandUp for Energy. Project website: https://www.mgm-negaf.proj.kth.se/.
Conflict of Interest
The authors declare that the research was conducted in the absence of any commercial or financial relationships that could be construed as a potential conflict of interest.
Publisher’s Note
All claims expressed in this article are solely those of the authors and do not necessarily represent those of their affiliated organizations, or those of the publisher, the editors, and the reviewers. Any product that may be evaluated in this article, or claim that may be made by its manufacturer, is not guaranteed or endorsed by the publisher.
Supplementary Material
The Supplementary Material for this article can be found online at: https://www.frontiersin.org/articles/10.3389/fceng.2022.838265/full#supplementary-material
References
Adeel-Farooq, R. M., Raji, J. O., and Adeleye, B. N. (2020). Economic Growth and Methane Emission: Testing the EKC Hypothesis in ASEAN Economies. Meq 32 (2), 277–289. doi:10.1108/meq-07-2020-0149
Adhikari, B. K., Barrington, S., and Martinez, J. (2006). Predicted Growth of World Urban Food Waste and Methane Production. Waste Manag. Res. 24 (5), 421–433. doi:10.1177/0734242x06067767
Aggarwal, P. K. (2008). Global Climate Change and Indian Agriculture: Impacts, Adaptation and Mitigation. Indian J. Agric. Sci. 78, 911–919.
Agrawala, S., Moehner, A., Gagnon-Lebrun, F., Baethgen, W. E., Martino, D. L., Lorenzo, E., et al. (2004). “Development and Climate Change in Uruguay: Focus on Coastal Zones, Agriculture and Forestry,” in Working Party on Global and Structural Policies, Working Party on Development Co-operation and Environment. Paris: Organisation for Economic Co-operation and Development.
Ahmad, S., and Choi, M. J. (2010). Urban India and Climate Change Mitigation Strategies towards Inclusive Growth. Theor. Empir. Res. Urban Manag. 5 (615), 60–73.
Al-Dabbas, M. d. A. F. (1998). Reduction of Methane Emissions and Utilization of Municipal Waste for Energy in Amman. Renew. Energy 14 (1), 427–434. doi:10.1016/s0960-1481(98)00099-8
Al-Haj, A. N., and Fulweiler, R. W. (2020). A Synthesis of Methane Emissions from Shallow Vegetated Coastal Ecosystems. Glob. Chang. Biol. 26 (5), 2988–3005. doi:10.1111/gcb.15046
Alatzas, S., Moustakas, K., Malamis, D., and Vakalis, S. (2019). Biomass Potential from Agricultural Waste for Energetic Utilization in Greece. Energies 12 (6), 1095. doi:10.3390/en12061095
Allen, D. E., Mendham, D. S., Cowie, A., Wang, W., Dalal, R. C., and Raison, R. J.Bhupinderpal-Singh (2009). Nitrous Oxide and Methane Emissions from Soil Are Reduced Following Afforestation of Pasture Lands in Three Contrasting Climatic Zones. Soil Res. 47 (5), 443–458. doi:10.1071/sr08151
AlQattan, N., Acheampong, M., Jaward, F. M., Ertem, F. C., Vijayakumar, N., and Bello, T. (2018). Reviewing the Potential of Waste-To-Energy (WTE) Technologies for Sustainable Development Goal (SDG) Numbers Seven and Eleven. Renew. Energy Focus 27, 97–110. doi:10.1016/j.ref.2018.09.005
Ari, I., and Şentürk, H. (2020). The Relationship between GDP and Methane Emissions from Solid Waste: A Panel Data Analysis for the G7. Sustain. Prod. Consum. 23, 282–290. doi:10.1016/j.spc.2020.06.004
Awuchi, C., Awuchi, C., Ukpe, A., Asoegwu, C., Uyo, C., and Ngoka, K. (2020). Environmental Impacts of Food and Agricultural Production: A Systematic Review. Eur. Acad. Res. 8, 1120–1135.
Bach, V., Möller, F., Finogenova, N., Emara, Y., and Finkbeiner, M. (2016). Characterization Model to Assess Ocean Acidification within Life Cycle Assessment. Int. J. Life Cycle Assess. 21 (10), 1463–1472. doi:10.1007/s11367-016-1121-x
Balachandra, P. (2013b). Adopting Low Carbon Strategy for Ensuring Universal Energy Access in India: Innovative Governance and Institutional Mechanisms. Tokyo, Japan: United Nations University Headquarters.
Balachandra, P. (2012). Mainstreaming Access to Modern Energy Services in Rural India: A Low Carbon Strategy. Rio de Janeiro: International Society for Ecological Economics.
Balachandra, P. (2013a). Modern Bioenergy Technologies for Universalizing Energy Access in India: Solving the Conflicting Challenges of Climate Change and Development. Champaign, IL, USA: Common Ground Publishing, University of Illinois Research Park.
Bali Swain, R., and Ranganathan, S. (2021). Modeling Interlinkages between Sustainable Development Goals Using Network Analysis. World Dev. 138, 105136. doi:10.1016/j.worlddev.2020.105136
Baptista, F., Silva, L. L., de Visser, C., Gołaszewski, J., Meyer-Aurich, A., Briassoulis, D., et al. (2013). “Energy Efficiency in Agriculture,” in Complete Communications of the 5th International Congress on Energy and Environment Engineering and Management. Lisbon: Portalegre Polytechnic Institute and Extremadura University.
Berthe, A., and Elie, L. (2015). Mechanisms Explaining the Impact of Economic Inequality on Environmental Deterioration. Ecol. Econ. 116, 191–200. doi:10.1016/j.ecolecon.2015.04.026
Blignaut, J., Marais, C., Rouget, M., Mander, M., Turpie, J., Klassen, T., et al. (2008). “Making Markets Work for People and the Environment: Employment Creation from Payment for Eco-Systems Services,” in An Initiative of the Presidency of South Africa, Hosted by Trade and Industrial Policy Strategies (Pretoria, South Africa: Trade and Industrial Policy Strategies).
Bogner, J., Pipatti, R., Hashimoto, S., Diaz, C., Mareckova, K., Diaz, L., et al. (2008). Mitigation of Global Greenhouse Gas Emissions from Waste: Conclusions and Strategies from the Intergovernmental Panel on Climate Change (IPCC) Fourth Assessment Report. Working Group III (Mitigation). Waste Manag. Res. 26 (1), 11–32. doi:10.1177/0734242x07088433
Boretti, A., and Rosa, L. (2019). Reassessing the Projections of the World Water Development Report. npj Clean. Water 2 (1), 15. doi:10.1038/s41545-019-0039-9
Boyd, A. (2012). Informing International UNFCCC Technology Mechanisms from the Ground up: Using Biogas Technology in South Africa as a Case Study to Evaluate the Usefulness of Potential Elements of an International Technology Agreement in the UNFCCC Negotiations Process. Energy Policy 51, 301–311. doi:10.1016/j.enpol.2012.08.020
Byers, E., Gidden, M., Leclère, D., Balkovic, J., Burek, P., Ebi, K., et al. (2018). Global Exposure and Vulnerability to Multi-Sector Development and Climate Change Hotspots. Environ. Res. Lett. 13 (5), 055012. doi:10.1088/1748-9326/aabf45
Caniani, D., Esposito, G., Gori, R., and Mannina, G. (2015). Towards A New Decision Support System for Design, Management and Operation of Wastewater Treatment Plants for the Reduction of Greenhouse Gases Emission. Water 7 (10), 5599–5616. doi:10.3390/w7105599
Cerutti, A. K., Contu, S., Ardente, F., Donno, D., and Beccaro, G. L. (2016). Carbon Footprint in Green Public Procurement: Policy Evaluation from a Case Study in the Food Sector. Food Policy 58, 82–93. doi:10.1016/j.foodpol.2015.12.001
Chynoweth, D. P., Owens, J. M., and Legrand, R. (2001). Renewable Methane from Anaerobic Digestion of Biomass. Renew. Energy 22 (1), 1–8. doi:10.1016/s0960-1481(00)00019-7
Clapp, C., and Pillay, K. (2017). “Green Bonds and Climate Finance,” in Climate Finance. Toh Tuck Link: World Scientific Publishing Co. Pte. Ltd., 79–105.
Cornwall, A. (2016). Women's Empowerment: What Works? J. Int. Dev. 28 (3), 342–359. doi:10.1002/jid.3210
De Vries, J. W., Groenestein, C. M., Schröder, J. J., Hoogmoed, W. B., Sukkel, W., Groot Koerkamp, P. W. G., et al. (2015). Integrated Manure Management to Reduce Environmental Impact: II. Environmental Impact Assessment of Strategies. Agric. Syst. 138, 88–99. doi:10.1016/j.agsy.2015.05.006
Drabo, A. (2017). Climate Change Mitigation and Agricultural Development Models: Primary Commodity Exports or Local Consumption Production? Ecol. Econ. 137, 110–125. doi:10.1016/j.ecolecon.2017.03.014
Duraiappah, A. K., and Bhardwaj, A. (2007). Measuring Policy Coherence Among the MEAs and MDGs. International Institute for Sustainable Development Winnipeg.
Elbehri, A., Genest, A., and Burfisher, M. E. (2011). Global Action on Climate Change in Agriculture: Linkages to Food Security, Markets and Trade Policies in Developing Countries. Rome: Trade and Markets Division, Food and Agriculture Organization of the United Nations.
Eric, N., and Thomas, P. (2007). The Gendered Nature of Natural Disasters: The Impact of Catastrophic Events on the Gender Gap in Life Expectancy, 1981–2002. Ann. Assoc. Am. Geogr. 97 (3), 551–566. doi:10.1111/j.1467-8306.2007.00563.x
Fang, Y., Mauzerall, D. L., Liu, J., Fiore, A. M., and Horowitz, L. W. (2013). Impacts of 21st Century Climate Change on Global Air Pollution-Related Premature Mortality. Clim. Change 121 (2), 239–253. doi:10.1007/s10584-013-0847-8
FAO (2014). The State of Food and Agriculture 2014: Innovation in Family Farming. Rome, Italy: Food & Agriculture Organization of the United Nations.
FAO (2016). The State of Food and Agriculture 2016: Climate Change, Agriculture and Food Security. Rome, Italy: Food & Agriculture Organization of the United Nations.
FAO (2017). The State of Food and Agriculture 2017: Leveraging Food Systems for Inclusive Rural Transformation. Rome, Italy: Food & Agriculture Organization of the United Nations.
Farhana, S. (2014). Gendering Climate Change: Geographical Insights. Prof. Geogr. 66 (3), 372–381. doi:10.1080/00330124.2013.821730
Fatma, D. (2002). Climate Change Vulnerability, Impacts, and Adaptation: Why Does Gender Matter? Gend. Dev. 10 (2), 10–20. doi:10.1080/13552070215903
Fuso Nerini, F., Tomei, J., To, L. S., Bisaga, I., Parikh, P., Black, M., et al. (2018). Mapping Synergies and Trade-Offs between Energy and the Sustainable Development Goals. Nat. Energy 3 (1), 10–15. doi:10.1038/s41560-017-0036-5
Garcia-Gonzales, D. A., Popoola, O., Bright, V. B., Paulson, S. E., Wang, Y., Jones, R. L., et al. (2019). Associations Among Particulate Matter, Hazardous Air Pollutants and Methane Emissions from the Aliso Canyon Natural Gas Storage Facility during the 2015 Blowout. Environ. Int. 132, 104855. doi:10.1016/j.envint.2019.05.049
Geraldine, T. (2009). No Climate Justice without Gender Justice: an Overview of the Issues. Gend. Dev. 17 (1), 5–18. doi:10.1080/13552070802696839
Gerber, P. J., Hristov, A. N., Henderson, B., Makkar, H., Oh, J., Lee, C., et al. (2013a). Technical Options for the Mitigation of Direct Methane and Nitrous Oxide Emissions from Livestock: a Review. Animal 7 (Suppl. 2), 220–234. doi:10.1017/s1751731113000876
Gerber, P. J., Steinfeld, H., and Henderson, B. (2013b). Tackling Climate Change through Livestock: A Global Assessment of Emissions and Mitigation Opportunities. Rome: Food and Agriculture Organization of the United Nations.
Haider, A., Bashir, A., and Husnain, M. I. u. (2020). Impact of Agricultural Land Use and Economic Growth on Nitrous Oxide Emissions: Evidence from Developed and Developing Countries. Sci. Total Environ. 741, 140421. doi:10.1016/j.scitotenv.2020.140421
Haines, A., Amann, M., Borgford-Parnell, N., Leonard, S., Kuylenstierna, J., and Shindell, D. (2017). Short-lived Climate Pollutant Mitigation and the Sustainable Development Goals. Nat. Clim. Change 7 (12), 863–869. doi:10.1038/s41558-017-0012-x
Hall, J. W., Grey, D., Garrick, D., Fung, F., Brown, C., Dadson, S. J., et al. (2014). Coping with the Curse of Freshwater Variability. Science 346 (6208), 429–430. doi:10.1126/science.1257890
Hall, K. D., Guo, J., Dore, M., and Chow, C. C. (2009). The Progressive Increase of Food Waste in America and its Environmental Impact. PLoS ONE 4 (11), e7940. doi:10.1371/journal.pone.0007940
Hanna, R., and Oliva, P. (2016). Implications of Climate Change for Children in Developing Countries. Future Child. 26, 115–132. doi:10.1353/foc.2016.0006
Hussein, Z., Hertel, T., and Golub, A. (2013). Climate Change Mitigation Policies and Poverty in Developing Countries. Environ. Res. Lett. 8 (3), 035009. doi:10.1088/1748-9326/8/3/035009
Hwang, K.-L., Bang, C.-H., and Zoh, K.-D. (2016). Characteristics of Methane and Nitrous Oxide Emissions from the Wastewater Treatment Plant. Bioresour. Technol. 214, 881–884. doi:10.1016/j.biortech.2016.05.047
IEA (2022). Global Methane Tracker, 2022. Paris. Available at: https://www.iea.org/reports/global-methane-tracker-2022.
IPCC (2021). “Summary for Policymakers”. Climate Change 2021: The Physical Science Basis. Contribution of Working Group I to the Sixth Assessment Report of the Intergovernmental Panel on Climate Change. Editors V. Masson-Delmotte, P. Zhai, A. Pirani, S. L. Connors, C. Péan, S. Bergeret al. (Cambridge, United Kingdom and New York, NY, USA: Cambridge University Press).
Irene, D. (2002). Climate Change: Learning from Gender Analysis and Women's Experiences of Organising for Sustainable Development. Gend. \& Dev. 10 (2), 21–29. doi:10.1080/13552070215899
Jalil, S. A. (2014). Carbon Dioxide Emission in the Middle East and North African (MENA) Region: a Dynamic Panel Data Study. Jeeir 2 (3), 5–14. doi:10.24191/jeeir.v2i3.9629
Jane, H. H., and Michael, H. S. (2021). Climate Change and Sustainability as Drivers for the Next Mining and Metals Boom: The Need for Climate-Smart Mining and Recycling. Resour. Policy 74, 101205.
Johnson, K. A., and Johnson, D. E. (1995). Methane Emissions from Cattle. J. Animal Sci. 73 (8), 2483–2492. doi:10.2527/1995.7382483x
Karakurt, I., Aydin, G., and Aydiner, K. (2012). Sources and Mitigation of Methane Emissions by Sectors: A Critical Review. Renew. Energy 39 (1), 40–48. doi:10.1016/j.renene.2011.09.006
Katukiza, A. Y., Ronteltap, M., Oleja, A., Niwagaba, C. B., Kansiime, F., and Lens, P. N. L. (2010). Selection of Sustainable Sanitation Technologies for Urban Slums - A Case of Bwaise III in Kampala, Uganda. Sci. Total Environ. 409 (1), 52–62. doi:10.1016/j.scitotenv.2010.09.032
Kvist, T., and Aryal, N. (2019). Methane Loss from Commercially Operating Biogas Upgrading Plants. Waste Manag. 87, 295–300. doi:10.1016/j.wasman.2019.02.023
Ladha, J. K., Jat, M. L., Stirling, C. M., Chakraborty, D., Pradhan, P., Krupnik, T. J., et al. (2020). “Achieving the Sustainable Development Goals in Agriculture: The Crucial Role of Nitrogen in Cereal-Based Systems,” in Advances in Agronomy. Editor D. L. Sparks (Cambridge, Massachusetts: Academic Press), 39–116. doi:10.1016/bs.agron.2020.05.006
Lal, R., Smith, P., Jungkunst, H. F., Mitsch, W. J., Lehmann, J., Nair, P. K. R., et al. (2018). The Carbon Sequestration Potential of Terrestrial Ecosystems. J. Soil Water Conservation 73 (6), 145A–152A. doi:10.2489/jswc.73.6.145a
Levine, U. Y., Teal, T. K., Robertson, G. P., and Schmidt, T. M. (2011). Agriculture's Impact on Microbial Diversity and Associated Fluxes of Carbon Dioxide and Methane. Isme J. 5 (10), 1683–1691. doi:10.1038/ismej.2011.40
Lokshall, E. W. (2006). Climate Change and Technology in a Globalizing World: A Study on How the Clean Development Mechanism Facilitates Introduction of Climate Friendly Technology in Africa. Oslo: University of Oslo.
Lu, Y., Norse, D., and Powlson, D. (2020). Agriculture Green Development in China and the UK: Common Objectives and Converging Policy Pathways. Front. Agric. Sci. Eng. 7 (1), 98–105. doi:10.15302/j-fase-2019298
Macdiarmid, J. I., Douglas, F., and Campbell, J. (2016). Eating like There's No Tomorrow: Public Awareness of the Environmental Impact of Food and Reluctance to Eat Less Meat as Part of a Sustainable Diet. Appetite 96, 487–493. doi:10.1016/j.appet.2015.10.011
Makisha, N. (2016). Waste Water and Biogas - Ecology and Economy. Procedia Eng. 165, 1092–1097. doi:10.1016/j.proeng.2016.11.824
Massé, J., Gerber, P. J., Halpern, C., and Baedeker, T. (2020). Review: Climate Finance Readiness of the Animal Protein Sector: Overview of Experience in Linking the Sector to Climate Finance, and Options to Address Bottlenecks. Animal 14 (S3), s491–s499. doi:10.1017/s1751731120001755
Meena, R. S., Kumar, S., Bohra, J. S., and Jat, M. L. (2019). Sustainable Management of Soil and Environment. Gateway East, Singapore: Springer.
Michaelowa, A., and Michaelowa, K. (2007). Climate or Development: Is ODA Diverted from its Original Purpose? Clim. change 84 (1), 5–21. doi:10.1007/s10584-007-9270-3
Montes, F., Meinen, R., Dell, C., Rotz, A., Hristov, A. N., Oh, J., et al. (2013). SPECIAL TOPICS - Mitigation of Methane and Nitrous Oxide Emissions from Animal Operations: II. A Review of Manure Management Mitigation Options1. J. Animal Sci. 91 (11), 5070–5094. doi:10.2527/jas.2013-6584
Mosier, A. R., Duxbury, J. M., Freney, J. R., Heinemeyer, O., and Minami, K. (1996). “Nitrous Oxide Emissions from Agricultural Fields: Assessment, Measurement and Mitigation,” in Progress in Nitrogen Cycling Studies: Proceedings of the 8th Nitrogen Workshop Held at the University of Ghent. Editors O. Van Cleemput, G. Hofman, and A. Vermoesen (Dordrecht: Springer Netherlands), 589–602. doi:10.1007/978-94-011-5450-5_97
Mottet, A., Henderson, B., Opio, C., Falcucci, A., Tempio, G., Silvestri, S., et al. (2017). Climate Change Mitigation and Productivity Gains in Livestock Supply Chains: Insights from Regional Case Studies. Reg. Environ. Change 17 (1), 129–141. doi:10.1007/s10113-016-0986-3
Nansai, K., Kondo, Y., Giurco, D., Sussman, D., Nakajima, K., Kagawa, S., et al. (2019). Nexus between Economy-wide Metal Inputs and the Deterioration of Sustainable Development Goals. Resour. Conservation Recycl. 149, 12–19. doi:10.1016/j.resconrec.2019.05.017
Nerini, F., Hughes, N., Cozzi, L., Cosgrave, E., Howells, M., Tavoni, M., et al. (2019). Connecting Climate Action with Other Sustainable Development Goals. Nat. Sustain. 2, 1–7. doi:10.1038/s41893-019-0334-y
Ngunga, D., Nzogela, B., and Paul, B. K. (2020). Environmental Management in Dairy Production-A Review of Capacity Building Material and Opportunities for Maziwa Zaidi in Tanzania. Nairobi: Alliance of Bioversity International and CIAT.
Nuthalapati, C., Bathla, S., KumarA., , and Jha, G. (2018). Agriculture and Sustainable Development Goals: an Overview and Issues. Agric. Econ. Res. Rev. 31, 1–7. doi:10.5958/0974-0279.2018.00016.2
Oberle, B., Bringezu, S., Hatfeld-Dodds, S., Hellweg, S., Schandl, H., Clement, J., et al. (2019). Global Resources Outlook 2019: Natural Resources for the Future We Want. Nairobi, Kenya: United Nations Environment Programme.
Parravicini, V., Svardal, K., and Krampe, J. (2016). Greenhouse Gas Emissions from Wastewater Treatment Plants. Energy Procedia 97, 246–253. doi:10.1016/j.egypro.2016.10.067
Pradel, W., Yanggen, D., and Polastri, N. (2006). Trade Offs between Economic Returns and Methane Greenhouse Gas Emissions in Dairy Production Systems in Cajamarca, Peru. Livest. Res. Rural. Dev. Int. Electron. Mag. 18, 41.
Prasad, C. S., and Sejian, V. (2015). Climate Change Impact on Livestock Sector: Visioning 2025. New Delhi: Springer India, 479–489.
Rao, N. D., and Min, J. (2018). Less Global Inequality Can Improve Climate Outcomes. WIREs Clim. Change 9 (2), e513. doi:10.1002/wcc.513
Rashamol, V., and Sejian, V. (2018). Climate Resilient Livestock Production: Way Forward. California: Juniper Publishers.
Reid, M. C., Guan, K., Wagner, F., and Mauzerall, D. L. (2014). Global Methane Emissions from Pit Latrines. Environ. Sci. Technol. 48 (15), 8727–8734. doi:10.1021/es501549h
Riahi, K., Grübler, A., and Nakicenovic, N. (2007). Scenarios of Long-Term Socio-Economic and Environmental Development under Climate Stabilization. Technol. Forecast. Soc. Change 74 (7), 887–935. doi:10.1016/j.techfore.2006.05.026
Rodriguez, H. G., Maiti, R., Ivanova, N. S., and Kumari, C. A. (2020). “Chemical Composition of Woods of 37 Woody Species of Tamaulipan Thorn Scrub, Northeast Mexico: A Case Study,” in Sustainable Bioresource Management. New York: Apple Academic Press, 129–151. doi:10.1201/9780429284229-10
Rossati, A. (2017). Global Warming and its Health Impact. Int. J. Occup. Environ. Med. 8 (1), 7–20. doi:10.15171/ijoem.2017.963
RSIF (2021). RSIF Tanzania Country Report 2021. Nairobi: Regional Scholarship and Innovation Fund (RSIF).
Sanz-Pérez, E. S., Murdock, C. R., Didas, S. A., and Jones, C. W. (2016). Direct Capture of CO2 from Ambient Air. Chem. Rev. 116 (19), 11840–11876. doi:10.1021/acs.chemrev.6b00173
Satoh, Y., Kahil, T., Byers, E., Burek, P., Fischer, G., Tramberend, S., et al. (2017). Multi-model and Multi-Scenario Assessments of Asian Water Futures: The Water Futures and Solutions (WFaS) Initiative. Earth's Future 5 (7), 823–852. doi:10.1002/2016ef000503
Scheutz, C., Kjeldsen, P., Bogner, J. E., De Visscher, A., Gebert, J., Hilger, H. A., et al. (2009). Microbial Methane Oxidation Processes and Technologies for Mitigation of Landfill Gas Emissions. Waste Manag. Res. 27 (5), 409–455. doi:10.1177/0734242x09339325
Setboonsarng, S., and Gregorio, E. (2017). Achieving Sustainable Development Goals through Organic Agriculture: Empowering Poor Women to Build the Future. Mandaluyong City: Asian Development Bank.
Silva, R. A., West, J. J., Lamarque, J.-F., Shindell, D. T., Collins, W. J., Dalsoren, S., et al. (2016). The Effect of Future Ambient Air Pollution on Human Premature Mortality to 2100 Using Output from the ACCMIP Model Ensemble. Atmos. Chem. Phys. 16 (15), 9847–9862. doi:10.5194/acp-16-9847-2016
Sims, R. E. H. (2004). Renewable Energy: a Response to Climate Change. Sol. Energy 76 (1), 9–17. doi:10.1016/s0038-092x(03)00101-4
Singh, A., Lund, H. G., and Barr, J. (2018). Chapter 14 - Challenges in Building Climate-Resilient Quality Energy Infrastructure in Africa, in Resilience. Editors Z. Zommers, and K. Alverson (Amsterdam: Elsevier), 181–189. doi:10.1016/b978-0-12-811891-7.00014-1
Sirigina, D. S. S. S., Goel, A., and Nazir, S. M. (2021). “Multiple Greenhouse Gases Mitigation (MGM): Process Intensification to Mitigate Non-CO2 Gases and CO2 from Air,” in AIChE Annual Meeting (Boston, USA: American Institute of Chemical Engineers (AIChE)).
Smith, K. R., Frumkin, H., Balakrishnan, K., Butler, C. D., Chafe, Z. A., Fairlie, I., et al. (2013). Energy and Human Health. Annu. Rev. Public Health 34 (1), 159–188. doi:10.1146/annurev-publhealth-031912-114404
Sophia, H., Mariola, A., Tatiana, G., and Jasmin, I. J. I. (2020). Can We Turn the Tide? Confronting Gender Inequality in Climate Policy. Gend. Dev. 28 (3), 571–591. doi:10.1080/13552074.2020.1836817
Stolaroff, J. K., Bhattacharyya, S., Smith, C. A., Bourcier, W. L., Cameron-Smith, P. J., and Aines, R. D. (2012). Review of Methane Mitigation Technologies with Application to Rapid Release of Methane from the Arctic. Environ. Sci. Technol. 46 (12), 6455–6469. doi:10.1021/es204686w
Subramaniam, Y., and Masron, T. A. (2020). Education, Methane Emission and Poverty in Developing Countries. J. Environ. Econ. Policy 9 (3), 355–369. doi:10.1080/21606544.2019.1689175
Tajul Ariffin, M., and Yogeeswari, S. (2019). Does Poverty Cause Environmental Degradation? Evidence from Developing Countries. J. Poverty 23 (1), 44–64. doi:10.1080/10875549.2018.1500969
Taseli, B. K., and Kilkis, B. (2016). Ecological Sanitation, Organic Animal Farm, and Cogeneration: Closing the Loop in Achieving Sustainable Development-A Concept Study with On-Site Biogas Fueled Trigeneration Retrofit in a 900-bed University Hospital. Energy Build. 129, 102–119. doi:10.1016/j.enbuild.2016.07.030
Trung, N. D., Thang, N. T., Anh, L. H., Babu, T. S. A., and Sebastian, L. (2020). Analysing the Challenges in Implementing Vietnam's Nationally-Determined Contribution (NDC) in the Agriculture Sector under the Current Legal, Regulatory and Policy Environment. Cogent Environ. Sci. 6 (1), 1792670. doi:10.1080/23311843.2020.1792670
United Nations Environment Programme (2021b). An Eye on Methane: International Methane Emissions Observatory 2021. Nairobi: United Nations Environment Programme.
United Nations Environment Programme (2021a). Global Methane Assessment: Benefits and Costs of Mitigating Methane Emissions. Nairobi: United Nations Environment Programme.
United Nations/Framework Convention on Climate Change (2015). Adoption of the Paris Agreement, 21st Conference of the Parties. Paris: United Nations.
Ursula Oswald Spring (2019). Gender, Climate Change and Sustainable Development Goals. Cham: Springer International Publishing, 225–241.
Venkatram, R. (2021). “Role of Agricultural Education in Understanding Climate Change,” in Role of Education in Combating Climate Change. Bangalore: MTC Global, 79.
Venkatramanan, V., and Shah, S. (2019). “Climate Smart Agriculture Technologies for Environmental Management: The Intersection of Sustainability, Resilience, Wellbeing and Development,” in Sustainable Green Technologies for Environmental Management. Editors S. Shah, V. Venkatramanan, and R. Prasad (Singapore: Springer Singapore), 29–51. doi:10.1007/978-981-13-2772-8_2
Vinuesa, R., Azizpour, H., Leite, I., Balaam, M., Dignum, V., Domisch, S., et al. (2020). The Role of Artificial Intelligence in Achieving the Sustainable Development Goals. Nat. Commun. 11 (1), 233. doi:10.1038/s41467-019-14108-y
Watts, N., Adger, W. N., Agnolucci, P., Blackstock, J., Byass, P., Cai, W., et al. (2015). Health and Climate Change: Policy Responses to Protect Public Health. Lancet 386 (10006), 1861–1914. doi:10.1016/s0140-6736(15)60854-6
Williams-Breault, B. D. (2018). Eradicating Female Genital Mutilation/Cutting: Human Rights-Based Approaches of Legislation, Education, and Community Empowerment. Health Hum. Rights 20 (2), 223–233.
Williamson, C. (2017). Emission, Education, and Politics: an Empirical Study of the Carbon Dioxide and Methane Environmental Kuznets Curve. Park Place Econ. 25 (1), 9.
Keywords: sustainable development goals, non-fossil methane emissions, agriculture, impact assessment, society, environment, economy, non-CO2 greenhouse gases
Citation: Sirigina DSSS and Nazir SM (2022) Non-Fossil Methane Emissions Mitigation From Agricultural Sector and Its Impact on Sustainable Development Goals. Front. Chem. Eng. 4:838265. doi: 10.3389/fceng.2022.838265
Received: 17 December 2021; Accepted: 21 April 2022;
Published: 01 June 2022.
Edited by:
Fabrizio Bezzo, University of Padua, ItalyReviewed by:
Tim Patterson, University of South Wales, United KingdomCopyright © 2022 Sirigina and Nazir. This is an open-access article distributed under the terms of the Creative Commons Attribution License (CC BY). The use, distribution or reproduction in other forums is permitted, provided the original author(s) and the copyright owner(s) are credited and that the original publication in this journal is cited, in accordance with accepted academic practice. No use, distribution or reproduction is permitted which does not comply with these terms.
*Correspondence: Devesh Sathya Sri Sairam Sirigina, c2lyaWdpbmFAa3RoLnNl; Shareq Mohd Nazir, c21uYXppckBrdGguc2U=