- 1Department of Chemical Engineering, Indian Institute of Technology (Indian School of Mines) Dhanbad, Dhanbad, India
- 2CSIR-Center for Cellular and Molecular Biology, Hyderabad, India
In this research work, for the first time, we have developed and demonstrated a COVID-19 repellent coating on cotton cloth that not only repels the virus but also most of the human body fluids (superhemophobic). The coating was tested in the BSL3 lab. The controlled experiments revealed no significant increase in the log viral particles on coated fabric compared to the uncoated surface, evidence that the coated fabric resisted the SARS-CoV-2 inoculum. Further, the coated cloth exhibited excellent dust-free nature and stain resistance against body fluids (blood, urine, bovine serum, water, and saliva aerosol). It also shows sufficient robustness for repetitive usage. The fabrication process for the developed COVID-19 repellent cloth is simple and affordable and can be easily scaled up for mass production. Such coating could be applied on various surfaces, including daily clothes, masks, medical clothes, curtains, etc. The present finding could be a mammoth step towards controlling infection spread, including COVID-19.
Introduction
In late 2019, a new virus, SARS-CoV-2 (also known as COVID-19) emerged. Within a few months of emerging, it created a havoc situation worldwide, and eventually, WHO declared it a pandemic in March 2020 (Cucinotta and Vanelli, 2020). Due to its highly transmissible nature, nearly all nations reported infection cases from COVID-19. Most infected patients experience mild to moderate respiratory illness, mainly common flu symptoms, and recover with standard flu treatment. However, some people with medical history such as diabetes, chronic respiratory disease, and cardiovascular disease may also experience severe illness or death. It is estimated that COVID-19 has resulted in more than 6.5 million deaths and billions of dollars in losses (Who, 2020b). Infection of the virus occurs through the respiratory system via the direct transmission of airborne droplets (sneeze, cough), direct contact transmission (blood or other liquid, skin), indirect transmission from a surface (respirator mask, cloth, carpet, handles, switches, and table).
PPEs are not 100% safe as the virus remains alive on them for many days and requires very careful disposal practices to avoid further infection spread. Besides this, people face a tough time working with wearing these single-use multilayer full-body cover PPEs. As these PPEs are uncomfortable and expensive, most people bypass the protocols. Therefore, if COVID-19-repellent affordable PPEs are developed, which can be used multiple times, this will help to control the spread of infection to a huge extent.
The viral-repellent coating can be done by designing a surface with an excellent anti-wetting state that comprises a high static contact angle and low tilt angle with body fluid (blood, urine, protein, saliva aerosol, and water) (Galante et al., 2020). Typically, such a surface comprises nano and micro rough structures trapping air which makes a liquid-air interface and leads to superhemophobicity (blood static contact angle greater than 150° and tilt angle less than 10°). Designing a superhemophobic surface is more challenging as blood has a much lower surface tension (about 54 mN/m) (Tang and Hu, 2005; Leszczak et al., 2013). Body fluid is a direct carrier of transport viruses, microbes, and pathogens that lead to people associated with infected patients.
In view of the above findings, we have developed a novel COVID-19 repellent coating on cloth via an easy and economical surface modification method. This coated cloth is COVID-19 repellent in nature and was tested against COVID-19 virus in BSL-3, which confirmed excellent COVID-19 repellency. There are proposals on COVID-19 repellency earlier; however, the present study is the first to demonstrate it in reality.
Experimental details
To develop COVID-19-repellent coated cloth, modification of the cloth surface was done by using an immersion technique. Before coating, the as-received cloth was cleaned in water and acetone mixture solution in ultrasonication for 30 min and dried in a hot air oven at a temperature of 60°C. Later on, the coating solution was prepared by adding a 1% w/v of pefluorodecltriethoxysilane (PFDTES, > 97%, procured from Sigma-Aldrich Co., Ltd) into 40 ml ethanol (procured from Changshu Hongsheng Fine Chemical Co. Ltd.) and stirred for 2 h at the room temperature. The clean and dried cloth was immersed in the prepared coating solution for 24 h. Then, the sample was taken out and dried in a hot air oven at 90°C for 2 h.
The coated and uncoated surfaces were tested against the SARS-CoV2 virus (Indian/a3i clade/2020 isolate) in the BSL3 lab of CSIR-CCMB (ASTM E1052-20, 2020) for 30 and 60 min of contact with the virus. Briefly, the coated and uncoated cloth surface was co-incubated with the virus inoculum for 30 and 60 min, respectively. After the specified incubation time, the virus inoculum (0.1 MOI) from both surfaces was added to the Vero cells and left for 3 h post-infection, the viral inoculum was replaced with fresh media containing 10% FBS and was maintained at 37°C, 5% CO2, until 72 h. After 72 h, the cell supernatant was collected and spun for 10 min at 6,000 g to remove cell debris, and the supernatant was transferred to fresh collection tubes. The viral RNA was extracted using MagMAX™ Viral/Pathogen Extraction Kit (Applied Biosystems, Thermofisher Scientific), and an automated RNA extraction machine (KingFisher Flex (version 1.01, Thermofisher Scientific) was used as instructed by the manufacturer. The SARS-CoV2 viral copies were detected using COVID-19 RT-q PCR Detection Kit (Fosun 2019-nCoV qPCR, Shanghai Fosun Long March Medical Science Co. Ltd.) following the manufacturer’s instructions (Baller et al., 2020). The Ct values of the N gene, E gene, and ORF 1 ab were considered to estimate the % viral reduction and log viral particles.
The liquid (blood, urine, protein, water) contact angle measurements were performed using Tensiometer (DSA 25, Kruss, Germany) by placing a droplet of 3–5 μl on uncoated and coated samples at room temperature. The experiment was repeated at five different locations with each sample to calculate the average value. The surface morphology of the samples was carried out using a scanning electron microscope (SEM, JEOL, JSM-6480LV). The elemental analysis of the samples was performed using X-ray Photo-Electron Spectroscopy (XPS) with Auger Electron Spectroscopy (AES) module and C60 sputter gun (PHI 5000 Versa Probe II, FEI Inc.).
In the self-cleaning experiment, uncoated and coated samples were forcefully dipped into the muddy water for 10 min using the tweezer. Later, the samples were removed from the muddy water, and the behavior of the muddy water droplets was studied on the surfaces. The stain resistance experiment was performed by placing the liquid (urine and blood) droplets on uncoated and coated samples and later drying them by keeping the samples on the heating plate at 60°C for a few minutes. The urine and bloodstains left on the coated and uncoated surfaces were studied later.
The water absorption capacity of the uncoated and coated samples was measured. Initially, all the samples were immersed in water for 10 min. After draining the excess water, the weight of the samples (wa) was measured and compared with the weight of the sample before immersion in water (wd). The absorption capacity of the samples was calculated using Eq. 1.
Results and discussion
In this study, COVID-19 repellent cloth was developed by immersing cloth samples in PFDTES solution.
In virus testing, there was no significant reduction observed in the log viral number on exposing the viral inoculum on the coated and uncoated surface for 30 and 60 min (Figure 1). This may be due to the liquid-repellent nature of the coated surface. The contact of viral particles on the coated material was eliminated, and no viral increase was observed. This data proves the repellant nature of the test surface’s viral suspension repellent nature and supports the intactness of the droplet containing viral particles over the tested time (Figure 2).
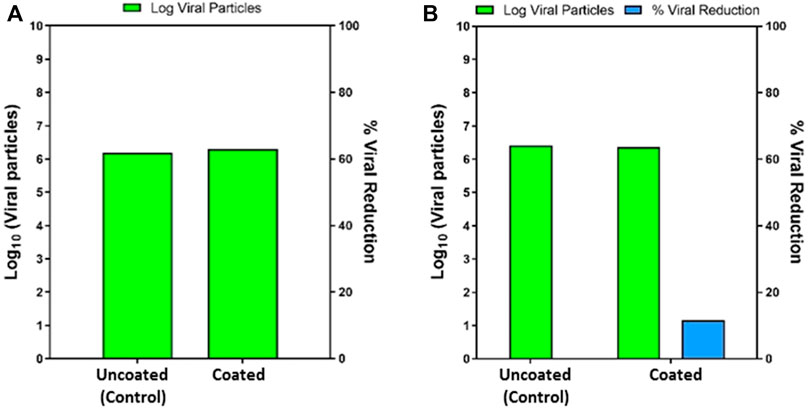
FIGURE 1. (A) The log viral particles at 30 min exposure on uncoated and coated surface (left). (B) The log viral particles at 60 min exposure on an uncoated and coated surface (right).
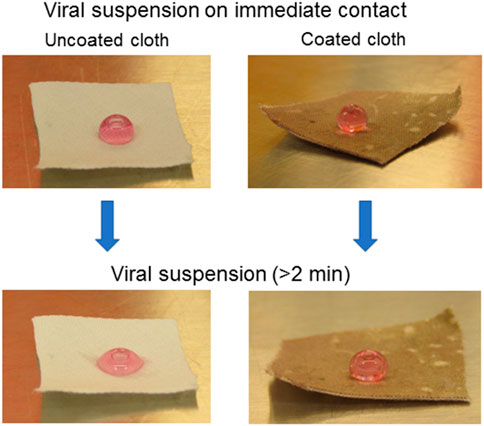
FIGURE 2. The optical images of viral suspension on immediate contact and after some time (>2 min) on uncoated and coated cloth.
The surface wettability of coated cloth was further measured and compared with the uncoated cloth. The cotton fabric exhibits superhemophilic behavior with a blood static contact angle of ∼0°. The blood repellency of coated cloth is measured at a static contact angle of 155°, indicating that the fabric has attained superhemophobicity. Additionally, body fluids (urine, protein, saliva aerosol, and water) also show good repellency with contact angles of more than 150° (Figure 3A, Table 1). The Supplementary Video shows the body fluid’s behavior on the coated surface (Supplementary Video S1).
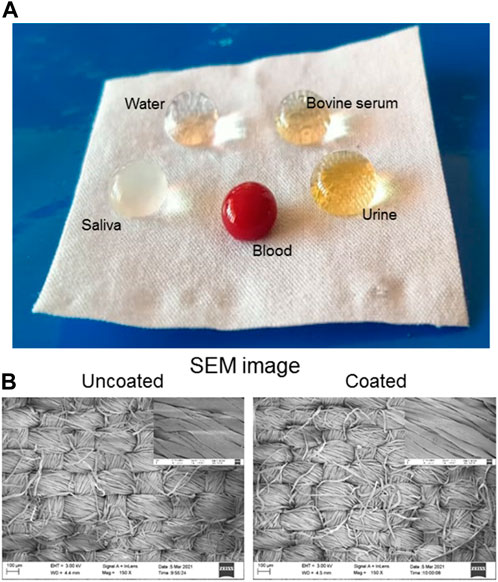
FIGURE 3. (A) Optical image of coated cloth surface containing blood, urine, bovine serum, saliva, and water droplets. (B) SEM images of uncoated and coated cloth. Right corner SEM images corresponding to the high magnification.
The surface morphology of the cloth surface after coating was examined by SEM analysis, as shown in Figure 3B. After coating, no change in morphology is observed due to the formation of a monolayer on its surface. The XPS results in Figure 4 confirm the chemical compositions of uncoated and coated samples. The XPS survey (Figure 4A) scan of uncoated samples confirms C, O, and Si of cellulosic structure and SiO2 as textile impurities. For coated cloth, C, O, and Si of cloth and PFDTS, and F of PFDTS are identified. The high-resolution core-level C 1s spectra (Figure 4B) of the coated and uncoated samples are easily distinguishable. The uncoated cloth’s C1s spectra exhibits three major peaks at 284.2, 285.7, and 287.7 eV corresponding -C-H/C-C, C-OH, and C-O-C functionalities confirm the cellulosic structure (Wang et al., 2020). The C 1s spectrum of coated cloth exhibit six major peaks at 284.1, 285.7, 287.1, 287.8, 290.8, and 293.1 eV corresponding to C-H/C-C, C-OH/C-O-Si, C-Si, C-OC, -CF2, and -CF3 functionalities confirms the presence of PFDTS and cellulosic structure (Korolkov et al., 2020). The high-resolution F1s spectra (Figure 4C) of coated cloth exhibits a peak at 688.0 eV corresponding to C-F functionality (Korolkov et al., 2020). The high-resolution O1s (Figure 4D) reveals a prominent peck shifting for coated (531.6 eV) and uncoated (532.2 eV) samples indicating a significant change in the oxygen chemical environment (Korolkov et al., 2020; Wang et al., 2020). Besides the carbon-oxygen bond of cellulose in the coated sample, oxygen is also bonded with silicon. The high-resolution Si 2p spectra of samples are presented in Figure 4E. The spectrum of uncoated cloth reveals peaks at 101.9 and 104.0 eV for 2p3/2 and 2p1/2 electrons corresponding to SiO2 impurities often found in textiles. The spectrum of coated cloth exhibits peaks at 102.4 and 104.0 eV for 2p3/2 and 2p1/2 electrons corresponding to the silane group of PFDTS (Korolkov et al., 2020). It is also observed that the Si content increases in coated cloth compared to uncoated cloth. The observed XPS data not only confirms the PFDTS but also indicates the (PFDTS) Si-O-C (cellulose) interaction leading to a stable PFDTS coating on the cloth surface. A probable scheme is presented in Figure 2F displaying the coating formation mechanism.
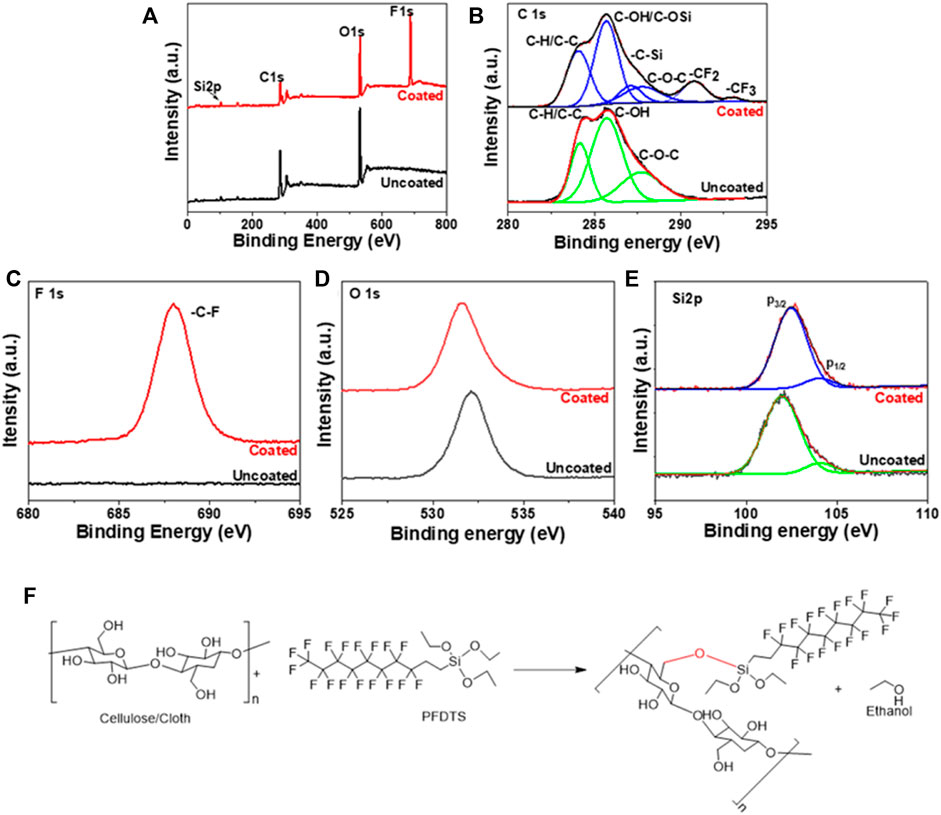
FIGURE 4. (A) Survey (B) C1s (C) F1s (D) O1s, and (E) Si2p XPS spectra of uncoated and coated cloth. (F) Mechanistic pathway of PFDTS coating on cloth.
The ability of a coated cloth to sustain against the dirt is demonstrated in Figure 5. For the experiment, uncoated and coated samples were immersed in a mud water solution for 10 min. It is noted that the coated fabric floats over the muddy solution due to its water repellency and is difficult to immerse; so, it is forcefully immersed in the solution. After 10 min immersion, the uncoated surfaces were soaked with muddy water, whereas coated cloth remained clean, indicating that mud particles do not adhere to the coated surface due to the air-solid interface present on the surface.
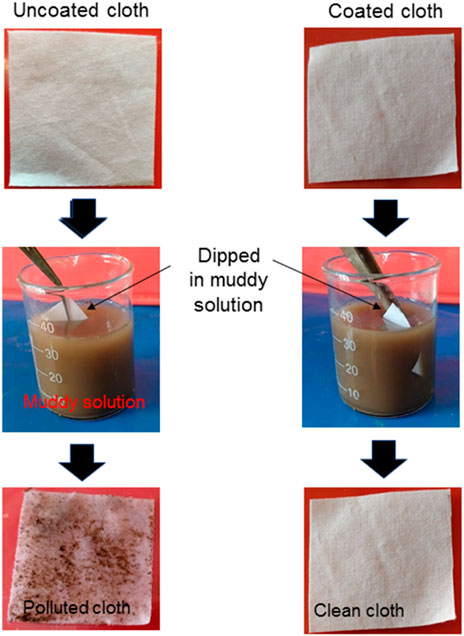
FIGURE 5. Optical images of uncoated and coated cloth, before and after immersing in the muddy solution, showing the ability of a coated cloth to sustain against the mud.
Body fluid (mainly blood and urine) leaves stain marks on the medical clothes/devices, including the apron, masks, bedsheets, and covers due to the inherent hydrophilic nature of the cloth fabric surface. In most cases, these stain marks do not fade away even after rigorous washing. In consideration of this, we have performed anti-stain and liquid absorption tests. Results of the anti-stain property of cloth before and after coating demonstrated that the blood droplets are entirely absorbed by uncoated cloth and spread over it, whereas these droplets make spherical shapes, as shown in Figure 6. A similar observation was seen for colored water and urine. Later these droplets containing samples were kept in a hot air oven at 50°C. After evaporation, these fluids leave stain marks on the uncoated cloth surface. Whereas there are negligible stain marks on a coated cloth surface, showing its anti-stain property.
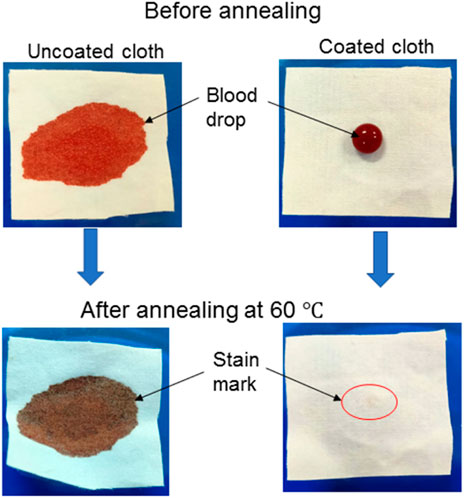
FIGURE 6. The optical images of blood droplets before and after drying on uncoated cloth (left the stain mark) and coated cloth (exhibit negligible stain marks), showing the stain-resistant ability of both coated cloths.
In the liquid absorption test, the uncoated and coated cloth was immersed in urine, as shown Figure 7. Initially, the weight of all samples was 0.043 g. After immersion, it was 0.167 and 0.047 g for uncoated and coated cloth, respectively. The calculated urine absorption capacity of cloth is shown in Figure 7. The uncoated cloth shows high urine absorption of 281% which is attributed to the cloth’s inherent superhydrophilic characteristic. The coated cloth exhibits a urine absorption of 8%, which is only 2.8% of the uncoated cloth. This result reveals that the urine absorbing capability is higher in uncoated samples compared to coated samples. The coated samples also show small urine absorption due to the non-homogeneity coating on its edges side.
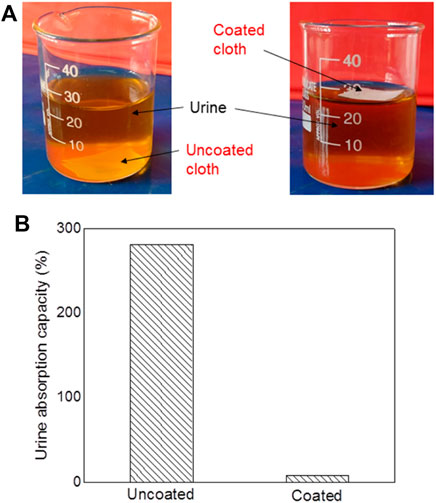
FIGURE 7. (A) The optical images of uncoated and coated cloth immersed in urine for 10 min, show the urine absorption ability. (B) Results of urine absorption capacity for uncoated and both coated cloths.
The global supplies for PPEs and masks due to their regular use are a constraint and make it harder for industries to meet the requirements. Incorporating thermal disinfection, UV-Illumination, or hydrogen peroxide vapor exposure (Celina et al., 2020) is a known time-consuming disinfection method. The present coating reduces the need for the aforementioned sanitization processes to a simple one, such as cleaning with a non-reactive disinfectant to the coating for 99% elimination of the viral particles.
Conclusion
In this paper, we have demonstrated a facile and durable COVID-19 repellent coating on cloth fabric. Results of the coated cloth showed the complete repellency of body fluid (blood, urine, bovine serum, water, saliva aerosol) with a static contact angle greater than 150°. Coated cloth showed non-adherence to dirt, stains from the body fluid, and negligible body fluid adsorption. The interaction of COVID-19 viruses with uncoated and coated was examined at BSL-3 research facilities, and it was found that coated cloth showed complete repellency to the viruses. Experimentally coated cloth showed good washing durability, i.e., it can be used multiple times. Since this COVID-19 repellent-coated cloth is made by a straightforward immersion technique, scaling up at the industrial level will be easily feasible. Commercializing such a product for general public use at economical rates will really help fight against the COVID-19 pandemic.
Data availability statement
The original contributions presented in the study are included in the article/Supplementary Material, further inquiries can be directed to the corresponding author.
Ethics statement
The SARS CoV2 study was approved by the Institutional Bio-safety Committee of CSIR Center for Cellular and Molecular Biology, Hyderabad, India.
Author contributions
All authors listed have made a substantial, direct, and intellectual contribution to the work and approved it for publication.
Acknowledgments
The authors are indebted to the Central Research Facilities, IIT (ISM) Dhanbad for coating characterization. The authors are indebted to the FRS (IIT(ISM) Dhanbad), MRP (TEQIP-III, IIT(ISM) Dhanbad), and SERB-DST (Grant No. CRG/2018/001277) for financial support.
Conflict of interest
The authors declare that the research was conducted in the absence of any commercial or financial relationships that could be construed as a potential conflict of interest.
Publisher’s note
All claims expressed in this article are solely those of the authors and do not necessarily represent those of their affiliated organizations, or those of the publisher, the editors and the reviewers. Any product that may be evaluated in this article, or claim that may be made by its manufacturer, is not guaranteed or endorsed by the publisher.
Supplementary material
The Supplementary Material for this article can be found online at: https://www.frontiersin.org/articles/10.3389/fceng.2022.1066184/full#supplementary-material
References
Astm E1052-20, (2020). Standard practice to assess the activity of microbicides against viruses in suspension. West Conshohocken, PA, USA: ASTM International.
Baller, A., Diaz, J., Pfeifer, D., Kerkhove, M. V., Otsu, S., and Peabody, R. (2020). Clinical management of severe acute respiratory infection when novel coronavirus (nCoV) infection is suspected. Geneva, Switzerland: WHO 2020.
Celina, M. C., Martinez, E., Omana, M. A., Sanchez, A., Wiemann, D., Tezak, M., et al. (2020). Extended use of face masks during the COVID-19 pandemic - thermal conditioning and spray-on surface disinfection. Polym. Degrad. Stab. 179, 109251. doi:10.1016/j.polymdegradstab.2020.109251
Cucinotta, D., and Vanelli, M. (2020). WHO declares COVID-19 a pandemic. Acta Biomed. 91, 157–160. doi:10.23750/abm.v91i1.9397
Galante, A. J., Haghanifar, S., Romanowski, E. G., Shanks, R. M. Q., and Leu, P. W. (2020). Superhemophobic and antivirofouling coating for mechanically durable and wash-stable medical textiles. ACS Appl. Mat. Interfaces 12, 22120–22128. doi:10.1021/acsami.9b23058
Gittings, S., Turnbull, N., Henry, B., Roberts, C. J., and Gershkovich, P. (2015). Characterisation of human saliva as a platform for oral dissolution medium development. Eur. J. Pharm. Biopharm. 91, 16–24. doi:10.1016/j.ejpb.2015.01.007
Korolkov, I. V., Kuandykova, A., Yeszhanov, A. B., Güven, O., Gorin, Y. G., and Zdorovets, M. V. (2020). Modification of PET ion-track membranes by silica nanoparticles for direct contact membrane distillation of salt solutions. Membranes 10, 322. doi:10.3390/membranes10110322
Le, T. T. Y., Hussain, S., Tsay, R. Y., Noskov, B. A., Akentiev, A., and Lin, S. Y. (2022). On the equilibrium surface tension of aqueous protein solutions – bovine serum albumin. J. Mol. Liq. 347, 118305. doi:10.1016/j.molliq.2021.118305
Leszczak, V., Smith, B. S., and Popat, K. C. (2013). Hemocompatibility of polymeric nanostructured surfaces. J. Biomaterials Sci. Polym. Ed. 24, 1529–1548. doi:10.1080/09205063.2013.777228
Tang, L., and Hu, W. (2005). Molecular determinants of biocompatibility. Expert Rev. Med. Devices 2, 493–500. doi:10.1586/17434440.2.4.493
Thomas, E. A., Poritz, D. H., and Muirhead, D. L. (2009). Urine advancing contact angle on several surfaces. J. Adhesion Sci. Technol. 23, 1917–1923. doi:10.1163/016942409x12508517390879
Wang, Q., Xie, D., Chen, J., Liu, G., and Yu, M. (2020). Superhydrophobic paper fabricated via nanostructured titanium dioxide-functionalized wood cellulose fibers. J. Mat. Sci. 55, 7084–7094. doi:10.1007/s10853-020-04489-7
WHO, . (2020). Available at www.who.int.
Keywords: COVID-19, SARS-CoV-2, anti-viral, superhemophobic, virucidal
Citation: Pandit SK, Chauhan P, Sinhamahapatra A, Parekh Y, Ghalib Enayathullah M, Bokara KK and Kumar A (2022) COVID-19 repellent cloth. Front. Chem. Eng. 4:1066184. doi: 10.3389/fceng.2022.1066184
Received: 10 October 2022; Accepted: 21 November 2022;
Published: 02 December 2022.
Edited by:
Nicola Maria Pugno, University of Trento, ItalyReviewed by:
Priya Varshney, National Institute of Technology Rourkela, IndiaShabi Thankaraj Salammal, Advanced Materials and Processes Research Institute (CSIR), India
Copyright © 2022 Pandit, Chauhan, Sinhamahapatra, Parekh, Ghalib Enayathullah, Bokara and Kumar. This is an open-access article distributed under the terms of the Creative Commons Attribution License (CC BY). The use, distribution or reproduction in other forums is permitted, provided the original author(s) and the copyright owner(s) are credited and that the original publication in this journal is cited, in accordance with accepted academic practice. No use, distribution or reproduction is permitted which does not comply with these terms.
*Correspondence: Aditya Kumar, aditya@iitism.ac.in, adityaku43@gmail.com; Kiran Kumar Bokara, bokarakiran@ccmb.res.in