- Department of Chemical Engineering, Indian Institute of Technology (ISM), Dhanbad, India
Solar water interfacial evaporation (SWIE) has attracted much attention for harvesting clean water. Over the last few decades, researchers have developed an innovative photo-thermal material for high-performance solar water interfacial evaporation. For higher evaporation performance, TiO2-based materials gain attention as a promising photo-thermal material due to their light absorption capacity. This study compared conceptual designs of TiO2-based materials for SWIE. Structural design and engineering strategies for improving evaporation rates and higher thermal conversion efficiency were reviewed. In addition, the material’s thermal stability and heat management were analyzed. This review provides an overview of the current advances in photo-thermal TiO2 materials to motivate research and translation efforts from the laboratory to large-scale solar water clean water production. Additional benefits of TiO2 materials on solar water interfacial evaporation should be investigated beyond containers to solve interconnected water, environmental, and energy progression.
1 Introduction
Shortage of fresh water is a great menace to sustained world economic growth. The scenario of scarce freshwater with increasing demand requires the development of specific dulling strategies to overcome this crisis. Recent strategies such as reverse osmosis, electrodialysis, membrane and thermal distillation have disadvantages including cost, need for heavy machinery, power requirements, waste generation, and high maintenance. Therefore, there is a need for technology that overcomes these problems and enhances water production in an eco-friendly way. Amid the search for new technology, solar water evaporation has emerged as an environmentally benign method of water purification. The usage of renewable solar energy has significant industrial applications including desalination (Liu et al., 2014), power generation (Hsieh and Lin, 2003), sterilization (Su et al., 2011), wastewater treatment (Li et al., 2013), and hygiene systems. In the conventional solar water evaporation process, the solar absorber collects solar energy and transforms it into thermal energy. This photo-thermal mechanism converts energy, increases the water temperature, and generates steam (Jiang et al., 2017). A solar water evaporation system that uses natural conditions or traditional methods is less efficient due to its poor light-absorption rate and higher heat loss (Ying et al., 2020). To optimize the current techniques, three main classes of photo-thermal mechanisms have been developed including the generation and relaxation of an electron hole, plasmonic localized heating (Zhao et al., 2015) and molecular thermal vibrations (Manuscript, 2018a). The generation of heat through thermal vibrations of molecules promotes solar water interfacial evaporation (SWIE) using advanced materials, allowing the generation of vapors and efficient production of clean water (Chen et al., 2019).
Material selection is based on high efficiency and a broad solar spectrum of photo-thermal materials (Ye and Li, 2022) such as plasmonic-metal nanostructures including Au (Chen H. et al., 2010) and Al (Zhou et al., 2016), carbon-based materials including wood-based composites (K. K. Liu et al., 2017) including graphene oxide (GO) (Shi et al., 2017) and CNT, semiconductors including Ti2O3 (J. Wang et al., 2017b), MoS2 (X. Yang et al., 2018), WOx (Ming et al., 2018), and NiO (D. Wu et al., 2019), nanostructured carbon-based frameworks (Geng et al., 2019), and conductive polymers. For efficient photo-thermal conversion, the optical and thermal properties of materials should be considered. Optical and thermal properties include the material’s absorption spectrum, absorption coefficient, thermal conductivity, heat transfer, and loss (Zhu et al., 2018). Light-to-heat conversion efficiency can be enhanced further by modifications such as the hybridization of light-absorbing materials, structural composition tuning, and multi-layer coating designs (Y. Yang et al., 2018). Despite the exceptional optical characteristics of nanocarbon materials, these materials are still not widely used due to some serious issues including 1) their time-consuming synthesis methodology, 2) expensive fabrication costs, and 3) difficulty in producing high-quality samples that are scalable (X. Wu et al., 2019). Among various photo-thermal materials, nanocarbon materials have excellent features including high absorbance in a broad spectrum, high stiffness, and self-floating ability that is suitable for SWIE (Jiang et al., 2017). Semiconductor materials with tunable bandgap energy also promote light-to-heat conversion. When the energy of incident light is higher or equal to the bandgap energy of a semiconductor, this excites an electron hole pair and releases energy in the form of a photo-excited electron, which causes photo-thermal conversion (Hessel et al., 2011).
During the last few decades, the semiconductor TiO2 has gained attention as an absorbing material due to its high photoactivity, abundancy, high thermal stability, and low cost (Shayegan et al., 2018). However, unmodified TiO2 possesses a large bandgap (−3.2 eV anatase, −3.0 eV rutile, and −3.3 eV brookite) with respect to the visible light spectrum, so efforts have been made to expand solar absorption by narrowing the bandgap (Ye et al., 2016) (Naldoni et al., 2012). Some effective methods include increasing oxygen vacancy/Ti3+ impurities [25] (Sinhamahapatra, Jeon and Yu, 2015), the hydroxylation of TiO2 (Fan et al., 2015), and doping or co-doping with metals and non-metals (Kanakaraju et al., 2022) (Basavarajappa et al., 2020). Modified or reduced TiO2 also has good applicability as a photo-thermal material for SWIE because it possesses sufficient light absorption capacity and the ability to convert light to heat, along with the enhanced inherent properties of primitive/un-modified TiO2 (Wang et al., 2017a) (Chen et al., 2020)(Wang P. et al., 2019). Furthermore, these TiO2 nanomaterials have received more attention than bulk TiO2 due to their high surface-to-volume ratio, which leads to an increased surface area, enhanced light absorption rate, and photo reduction rate, resulting in high photo-activity (Li et al., 2017a).
Recent reviews published on solar water interfacial evaporation have summarized the current development of SWIE in terms of photothermal conversion materials, structural design, energy management with an emphasis on the salt-rejection capability (Zechang et al., 2022), improvement in self salt propelling mechanisms (Sheng et al., 2021), recent advances in photothermal materials in wastewater purification (Li et al., 2022), effect of super-wettability on SWIE (Luo et al., 2022), different material-based SWIE (Guan et al., 2021) (Dong et al., 2022) (Yan et al., 2022), and many others (Han et al., 2022) (He et al., 2021) (Bai et al., 2020). However, when reviewing the different aspects of advances in SWIE, none has discussed TiO2-based photothermal materials that might empower SWIE as a whole, which is one of the most exciting materials in solar water evaporation. Thus, the current review aims to provide a systematic overview of the recent progress on TiO2-based materials for SWIE. The article first provides an all-inclusive summary of the advanced development of SWIE using photo-thermal semiconductor materials. Subsequently, the physical mechanism and photo-induced application of TiO2 in SWIE are discussed. The structural tuning of TiO2 nanoparticles (NPs) and hybridization of TiO2 nanostructures with plasmonic metal, semiconductors, and carbon-based materials imparting superior photo-thermal capabilities are then featured. Finally, the conclusions and prospects of TiO2 materials and their advanced development for efficient and productive SWIE are presented. In addition, the review includes new achievements and guidelines for efficient SWIE operation.
2 Modification of solar water interfacial evaporation using semiconductors
2.1 Basic mechanism of solar water interfacial evaporation
Generally, during the process of solar evaporation, solar energy is converted to thermal energy to evaporate water (Liu et al., 2016). Normally, this type of solar evaporation occurs in nature, such as transpiration in plants and the evaporation of seawater into the atmosphere recycled by rain. Usually, the process of evaporation occurs at the air/water interphase, and this natural process has low efficiency (Bai et al., 2020). The modern approach for water purification by solar energy is photo-thermal evaporation.
Like any other solar thermal application, SWIE requires a solar-absorbing material to convert light to heat. In solar-driven interfacial water evaporation, solar absorbers (photo-thermal materials) receive and absorb solar energy and convert it to thermal energy (Figure 2B). This converted energy is used to vaporize the water present at the interface (Liu et al., 2017) (Shang and Deng, 2016). Solar absorbers, evaporation structures, thermal insulators, and collectors are the main functional components of solar-driven interfacial evaporation systems (Ying et al., 2020) (Bai et al., 2020). For proper absorber design, some critical criteria need to be fulfilled: 1) effective solar absorption capacity, 2) productive light-to-heat transfer, and 3) proper thermal regulation (Manuscript, 2018a). For the design of the required solar absorber, solar absorbing materials including nano-carbon (Ito et al., 2015), plasmonic metal NPs (Zhou et al., 2016) (Bae et al., 2015), semiconductors, and polymers (Manuscript, 2018a) have been investigated. These photo-thermal materials have been used in the form of membranes (Huang et al., 2020), foams (Manuscript, 2018a), hydrogels (Lei et al., 2021), and porous sheets (Namboorimadathil Backer et al., 2020). In addition to these requisite properties, the position of the photo-thermal material plays a vital role in SWIE functioning (Figure 1A). We categorized the various forms of solar heating as 1) bottom heating, 2) volumetric heating, and 3) interfacial heating. In bottom heating, heat is generated at the receiver and transferred to the bulk. This leads to unavoidable heat loss and low solar-water conversion efficiency. In contrast, the volumetric heating approach enhances the evaporation efficiency using photo-thermal material dispersion throughout the water system. However, the firm dispersion and insensitivity toward a high temperature question its use. Compared with the other two methods, interfacial heating is a new discovery that has been studied widely. This method has been classified as non-isolate and isolated solar heating. Interfacial heating localizes the solar energy at the evaporation interface, which suppresses the maximum heat loss while achieving high evaporation efficiency. This is achieved by employing a photo-thermal layer floating at the air–water interface, separated from the bulk working fluid. Interfacial heating with confined thermal energy at the absorber surface can be further categorized into non-isolated and isolated interfacial heating. The only difference between the two methods is the use of thermal insulation. Isolated interfacial heating avoids contact between the absorber and water, thereby minimizing massive conduction loss resulting in extremely high energy conversion efficiency compared with non-isolated heating (Figure 1B).
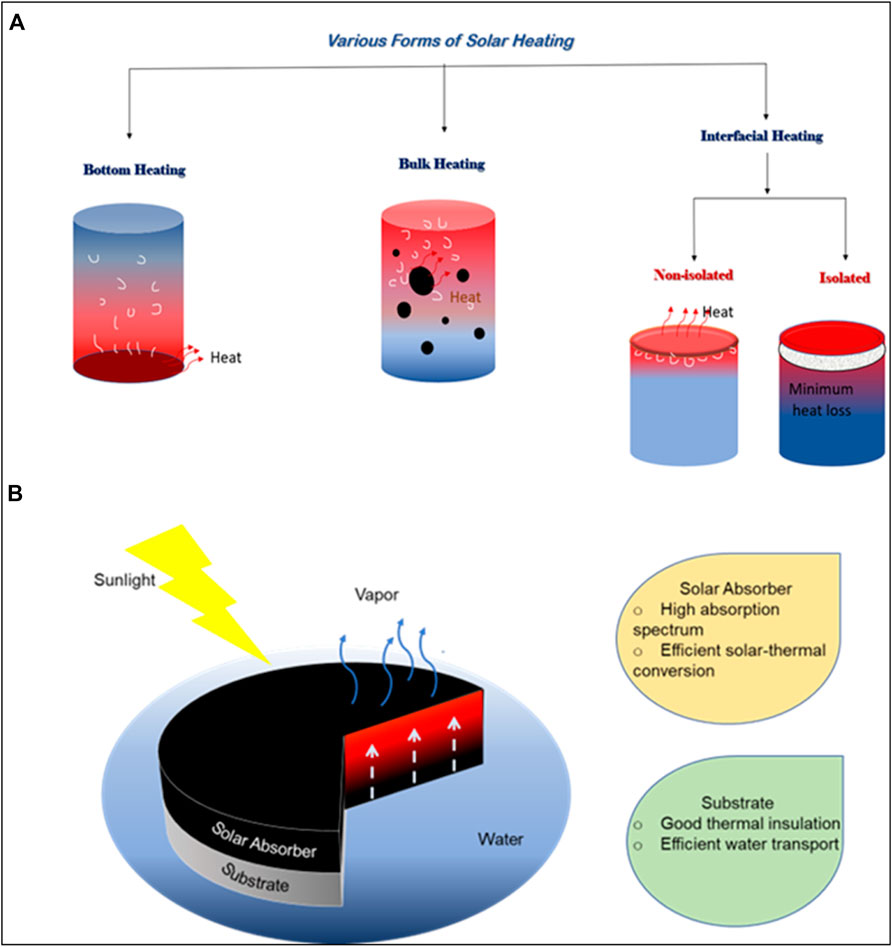
FIGURE 1. (A) Different types of solar water evaporation, and (B) schematic illustration of solar-driven interfacial water evaporation (isolated).
In order to alleviate heat loss encountered in the earlier designs, a thermal insulator or substrate has become an essential part of SWIE, not only providing lower thermal conductivity to the system but efficient also water transport to the absorber. He et al. (2021) presented a comparison between designs using plasmonic materials, and they demonstrated interfacial was more effective with a maximum water evaporation efficiency of 85% achieved at 10 Sun (1 Sun = 1 kWm−2) (X. Wang et al., 2017).
2.2 Semiconductors and their application in water evaporation
Over the past few decades, tremendous efforts have been made in material science to advance solar absorbers and supporting substrates to improve the performance of solar evaporation (Cao et al., 2019). Ideal photo-thermal materials for absorbers should meet the following criteria: 1) capacity to absorb the entire solar spectrum range (Yao et al., 2018), 2) less emissivity assuring high photo-thermal efficiency (J. Wang et al., 2017a), and 3) cost-effectiveness and abundant availability of the element (Guo et al., 2018).
Semiconductors are useful as solar absorbers for SWIE because their bandgap energy governs light-to-heat conversion. Some examples include MoS2 (Ghim et al., 2018) (Manuscript, 2018b), Ti-based structures (Ye et al., 2016) (Zhu et al., 2016), NiO (H. Liu et al., 2017), CuFeS2 (Manuscript, 2018b), and Mxene (Li et al., 2017b). Semiconductors absorb visible light irradiation with energy higher than the bandgap to generate electron hole pairs referred to as a semiconductor photo-excited state (Chen et al., 2020). Semiconductor excites electron hole pairs from the Valence band (VB) and conduction band (CB) by absorbing incident light energy equal to or higher than the bandgap and extra energy released in the form of photons, which is converted to heat as shown in Figures 2A, B. (Cao et al., 2019) (Zhu et al., 2018) (Ding et al., 2021)
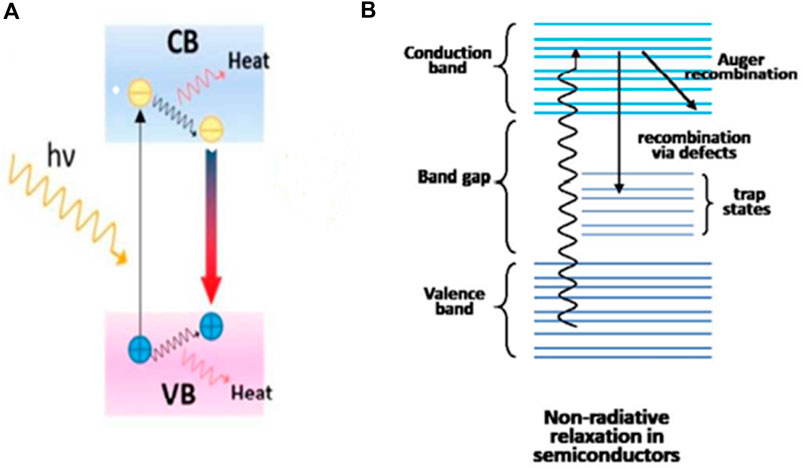
FIGURE 2. (A) Illustration of electron-hole generation and relaxation in a typical semiconductor (J. Wang et al., 2017b); Reproduced with permission. Copyright 2017 John Wiley and Sons. (B) Schematic of non-radiative relaxation in a semiconductor (Gao et al., 2019); Reproduced with permission. Copyright 2019 Royal Society of Chemistry.
Semiconductors generate electron hole pairs to illuminate the same energy as the bandgap. The energy released by the excited electrons is radiative in the form of photons from the conduction band and non-radiative in the form of phonons (heat). Energy generated from phonons produces temperature distribution due to induced local heating based on optical absorption (Zada et al., 2020). A narrow bandgap semiconductor is widely suggested because electron hole recombination occurs near band edges in broad bandgap semiconductors with a narrow absorption wavelength—generating photons also affects the photo-thermal efficiency. The position of the VB and/or CB can be altered by varying the size, structure, and composition of the semiconductors, thus regulating the energy bandgap and light absorption capabilities of the materials (Kamat, 2008). Solar evaporation has also been recorded using hierarchical copper phosphate (HCuPO) (Hua et al., 2017). Some Ti-based semiconductors, such as black/reduced TiO2 (Ullattil et al., 2018), and black/reduced TiOx (Huang et al., 2020), were previously exploited as innovative light absorbers for solar steam generation.
3 TiO2-based materials for solar evaporation
Photo-induced processes have been studied in diverse ways. During this process, energy can be used chemically (photocatalyst), electrically (solar cells), or thermally (photo-thermal). Despite different processes and applications, all the processes have a similar origin (Carp et al., 2004). Here, we focused on TiO2 because of its importance and its primary use as a photo-active compound for many applications. Apart from being an excellent photo-responsive material, its semiconductor properties enhance water quality free from organic pollutants in SWIE (Ding et al., 2021). Figure 3A shows the promising properties of TiO2 as a prominent photo-responsive candidate for SWIE, and the mechanism of this photocatalytic property is presented in Figure 3B.
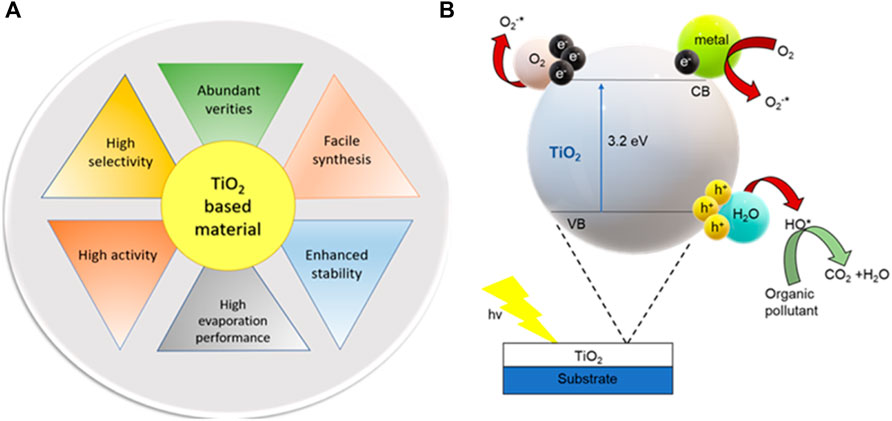
FIGURE 3. (A) Merits of TiO2 materials in solar evaporation and (B) the photocatalytic process of TiO2 semiconductors. Electrons and holes are generated by the UV light absorption of TiO2.
Photo-thermal materials with an irregular surface or nanostructure ensure a high evaporation performance (Zhu et al., 2018). In this context, TiO2 nanocomposites including nanocages (Zhu et al., 2016), nanotubes (Liu et al., 2021), NPs (Zhu et al., 2021), and submicrons (Wang et al., 2021) have been investigated by researchers. TiO2 NPs were used to fabricate films, membranes, and meshes with mechanical, thermal, and chemical stability under diverse conditions (Li et al., 2017a). In addition, a combination of several NPs forming a composite structure showed remarkable properties for SWIE (Tudu et al., 2020) (Huang et al., 2017a).
Despite these numerous advantages, TiO2 has a wide bandgap and low absorption capacity. Of note, the bandgap plays a vital role in functioning. Semiconductors with a narrow bandgap offer a broad solar absorption spectrum for photocatalysis and have the highest solar conversion efficiency with better SWIE performance. A narrower bandgap enables photons with low energy to excite electrons, thus extending the solar absorption spectrum, which is the basic criterion of SWIE functioning. In particular, when unmodified TiO2 is subjected to different bandgap engineering (i.e., lowering of the bandgap), it demonstrates the same effects. For instance, Ying et al. (2020) compared the bandgap and evaporation rates of pristine TiO2, N-doped titanium dioxide (N-TiO2), Ti3+-doped titanium dioxide (Ti3+-TiO2), and N, Ti3+-co-doped Ti dioxide (N, Ti3+−TiO2) prepared using nontreatment, nitrogen doping, self-doping, and co-doping strategies, respectively. When the measured bandgaps were 3.24, 3.15, 2.23, and 2.40 eV, respectively, the Ti3+-TiO2 membrane, with a narrower bandgap, had the highest evaporation rate of 1.20 kg m−2 h−1 with a solar-heat conversion efficiency of 77.1%. Therefore, the narrower the bandgap, the higher the evaporation rate and effective SWIE is achieved. Ullattil et al. (2018) observed a significant reduction in the TiO2 bandgap of around 1.23 eV via hydrogenation with an optical efficiency near 1,000 nm in the near-infrared region, beating the capability of pure TiO2 (anatase or rutile form), which only captures light in the UV spectrum. Black or reduced TiO2 (−1.5 eV) is another material that absorbs sufficient solar energy and efficiently converts light to heat (Chen et al., 2011) (Ullattil et al., 2018). Increasing reports based on black TiO2 photo-thermal material have been published recently, including Mg reduction-based black TiOx NPs (Ye et al., 2016), unique nanocage structures of black titania (Zhu et al., 2016), moth-eye-like nanostructures of nanocomposite black titania (Liu and Cheng, 2018), black TiO2 synthesis using NaN3 deflagration (Zheng et al., 2020), and Ti2O3 NPs with a narrow-bandgap (J. Wang et al., 2017a). Recently, the development of metal@TiO2 core-shell NPs demonstrated effective photocatalytic reactions and utilization of sunlight by TiO2 (Tom et al., 2003). Different studies have examined the effectiveness of TiO2-coated superhydrophobic surfaces for solar evaporation, such as Au-TiO2 coated films (Huang et al., 2017b), synthesis of Fe3O4-TiO2 NPs (Shi et al., 2017), and superhydrophobic steel meshes (Zhang et al., 2015). The metal@TiO2 has the dual advantage of both metals and TiO2 NPs to achieve remarkable photo-reactions and solar water evaporation efficiency. The core-shell structure of TiO2 increases the internal light scattering of the TiO2 shell. A thin film metal structure also improves the photodegradation and evaporation rates by concentrating heat through the NPs (Huang et al., 2017a).
3.1 TiO2-based materials under different structural engineering methods
An ideal photo-thermal material should possess a high light absorption capacity and cover the whole solar spectrum; however, commercial TiO2 only absorbs UV light due to its wide band structure. A narrow band structure of TiO2 is desired to broaden the solar spectrum range. To narrow the bandgap, creating oxygen vacancies, doping, and synthesis methods creating nanostructures are the most commonly used techniques to achieve a high solar absorption efficiency (J. Wang et al., 2017a). For instance, Xue et al. (2022) prepared ultrathin TiO2 nanosheets@Ti plates by the hydrothermal treatment of Ti plates. The synthesized sample was incorporated with polystyrene to maintain its floatation properties on water. C, N co-doping was performed to enhance the absorption capacity of the prepared nanosheet structures. Furthermore, a comparative study of the performance of TiO2 film@Ti plates and C, N co-doped TiO2 nanosheets showed the highest water evaporation rate and solar-to-vapor conversion efficiency of about 1.57 kg m−2 h−1 and 73.5%, respectively, under simulated solar light irradiation. Likewise, Ying et al. (2020) prepared N-doped TiO2, Ti3+ doped TiO2, and N, Ti3+ co-doped TiO2 by N2 doping, self-doping, and co-doping techniques, respectively, and correspondingly measured their bandgaps. The comparative study was carried out based on their band structures to identify a relationship between the electronic structure and performance in solar water evaporation systems (Figures 6(1)a, b). Among all Ti3+ doped, TiO2 showed the highest evaporation rates of 1.2 kg m−2 hr−1 (Figure 6(1)c) with a higher solar conversion efficiency of around 70% (Figure 6(1)d) at the narrowest bandgap of 2.23 eV with properties of excellent stability, pollutant degradation capability, and low cost for clean water production.
Chen et al. (2020) prepared rutile TiO2 (L-TiO2) with plentiful oxygen vacancies by the laser ablation in liquid (LAL) one-step method. These TiO2 NPs were used to form superhydrophobic nickel foams (NFs), leading to higher solar absorption (Figures 4(2) (a,b,c)). L-TiO2 has abundant oxygen vacancies that narrow the bandgap to improve optical absorption. In solar water evaporation experiments, the water evaporation rates at different temperatures increased and then stabilized to 1.25 kgm−2h−1 with a high efficiency of 78.5% (Figure 4(2)(d,e)) under 1 Sun radiation. The rates and efficiency were 1.81 times those of commercial titania-based evaporators (C-TiO2/NF) (Figure 4(2)f).
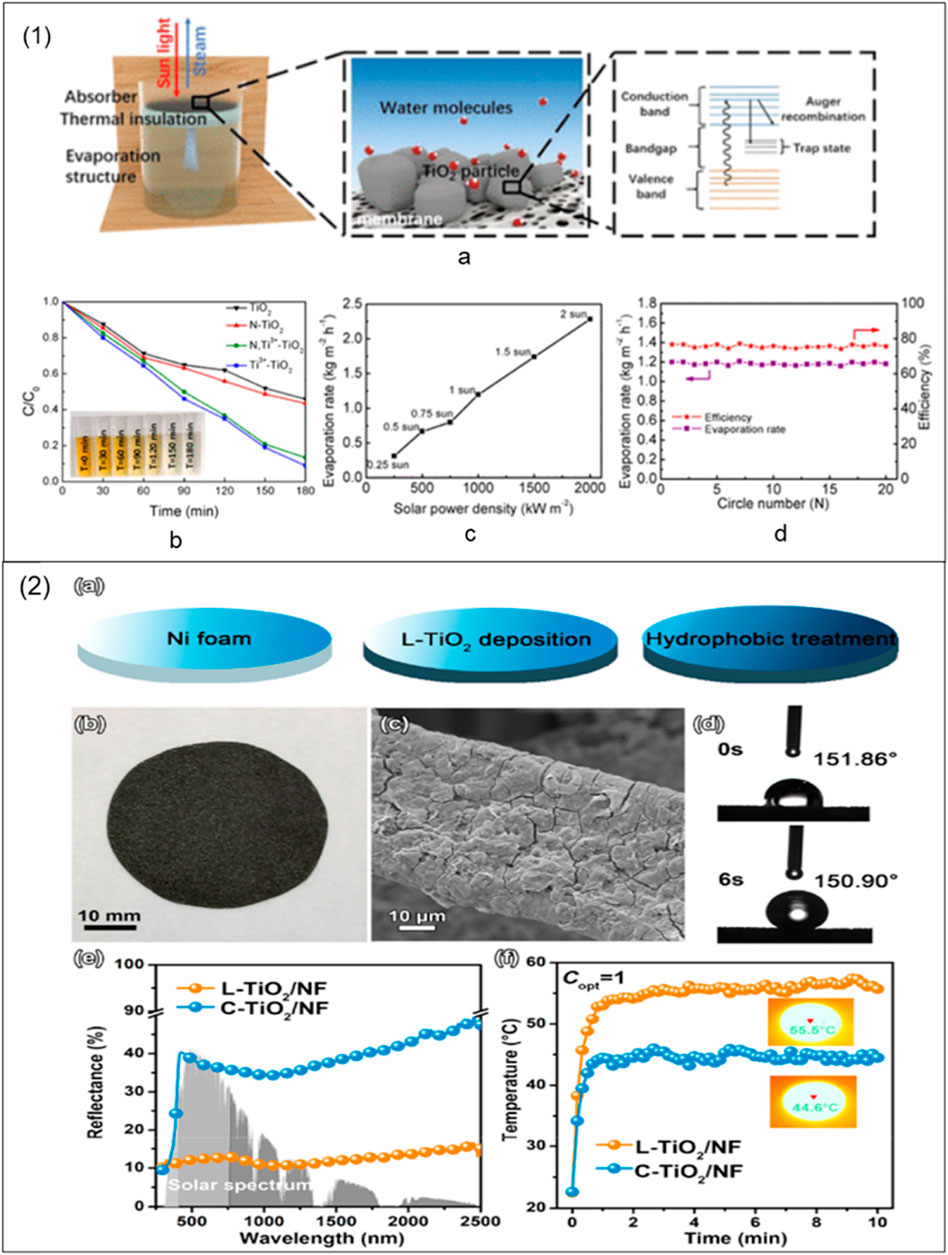
FIGURE 4. (1) (a) Schematic diagram of the proposed mechanism for solar-driven interfacial evaporation of semiconductor materials. (b) Photocatalytic activity of different samples under xenon lamp irradiation with time. (c) Evaporation rates under irradiation by different light intensities. (d) Measured recycle performance of Ti3+-TiO2 (Ying et al., 2020); Reproduced with permission. Copyright 2020 American Chemical Society. (2) (a) Schematic diagram of the preparation of an L-TiO2/NF evaporator. (b) Photograph and (c) SEM image of L-TiO2/NF. (d) Mass change of water as a function of irradiation time with different structures under 1 Sun irradiation. Diffuse reflectance spectra of L-TiO2/NF and C-TiO2/NF. Along with solar spectrum at the background (f) Surface temperature vs time plots for L-TiO2/NF and C-TiO2/NF exposed to one sun's worth of radiation. The relevant temperature distribution pictures at steady state are shown in the insets. (Chen et al., 2020); Reproduced with permission. Copyright 2020 American Chemical Society.
The aforementioned findings suggest that a logical design of TiO2 nanosheets could meet advanced technology’s demand for enhanced water filtration. Different studies demonstrated that structural engineering is a feasible way to improve the performance of TiO2-based systems for solar-driven water interfacial evaporation systems.
3.2 Black/reduced TiO2—A modified version of TiO2
During the past few decades, efforts have been made to develop suitable light-absorbing efficient photo-thermal materials. In this regard and in contrast to TiO2, black/reduced TiO2 is a modified version that has emerged as a useful material with excellent light absorption capacity with a tunable and reduced bandgap depending on the extent of reduction (Pelaez et al., 2012) (Sinhamahapatra et al., 2015). In recent years, much effort has been made to widen the range of light absorption of TiO2 by elemental doping (Liu et al., 2020), lattice disorder, and defect engineering (Pan et al., 2013), where the light absorption of a black body nano porous material was found to be greater. Chen et al. (2011) was the first to describe the preparation of black/reduced TiO2 as a superior light-absorbing photocatalytic material, and subsequently, researchers started to investigate the performance of black/reduced TiO2 as a solar-thermal material.
Ye et al. (2016) prepared a PFOTS-modified superhydrophobic black/reduced TiOx-coated stainless steel mesh for SWIE. They successfully synthesized TiOx (x < 2) NPs with different Ti/O ratios via magnesium reduction. Higher the Ti/O ratio, higher is the reduction that produces darker TiOx and, as a result, boosts the efficiency of solar water conversion. The maximum steam generation efficiency was 50.30% at 1 Sun irradiation with the darkest TiO2 NPs when compared with bare mesh and water (Figure 5(1)).
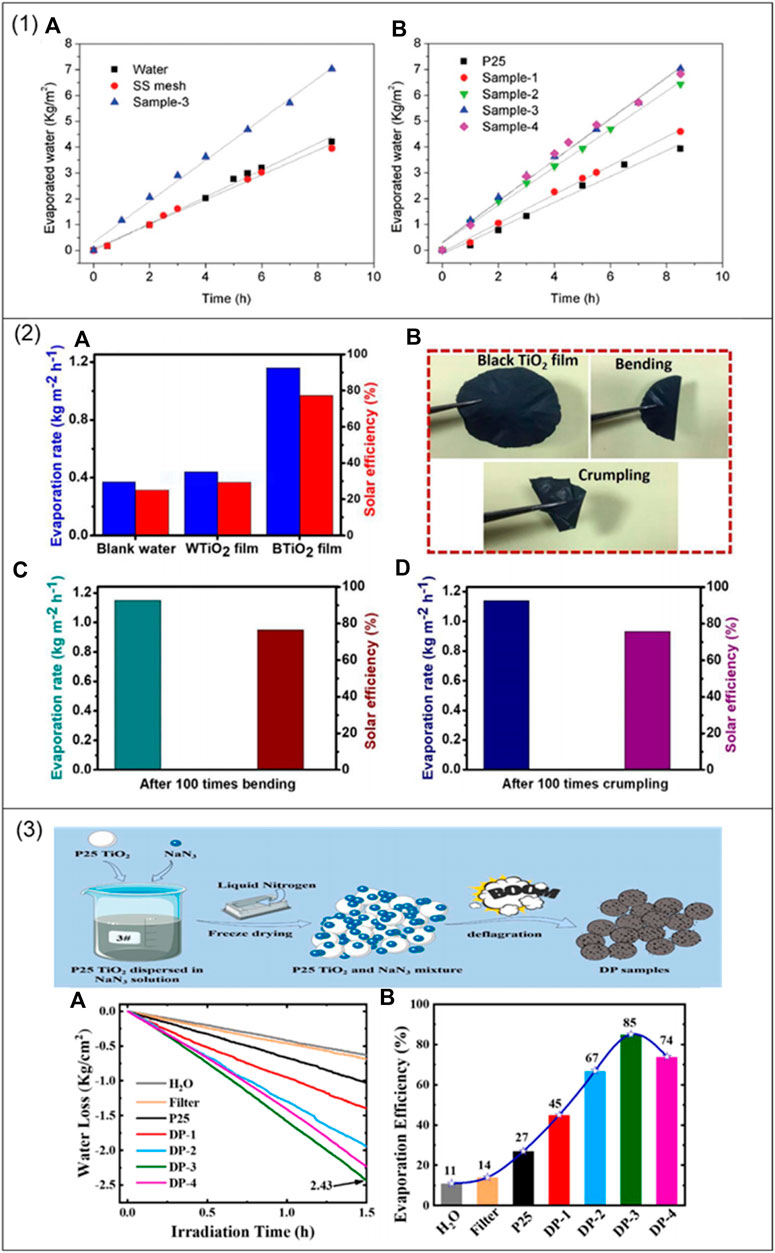
FIGURE 5. (1) Mass of evaporated water as a function of the radiation time in the absence or presence of an SS mesh coated with different TiOx NPs (Ye et al., 2016); Reproduced with permission. Copyright 2016 John Wiley and Sons. (2) (A) Evaporation rate and solar efficiency of blank water, WTiO2, and BTiO2. (B) Images of BTiO2 bending and crumpled film. (C, D) Evaporation rates of bending and crumpled BTiO2 films (Zada et al., 2020); Reproduced with permission. Copyright 2020 Elsevier. (3) Schematic fabrication of DP samples. (A) Evaporation rate of water. (B) Evaporation efficiency of different samples (Zheng et al., 2020); Reproduced with permission. Copyright 2020 Elsevier.
Recently, advances have been made in the preparation of black/reduced TiO2 with an easy and fast route for high solar water evaporation efficiency. Zada et al. (2020) synthesized a random size distributed black TiO2 film with polyvinylidene fluoride (PVDF) for a SWIE alike random close packing photonic structure (Dong et al., 2010). The constructed BTiO2 film possesses sufficient oxygen vacancies, surface defects with several small cavities (due to its wide size distribution), and improved optical absorption in the UV–Vis and NIR range due to multiple light reflection and scattering properties. An evaporation test based on a localized heating concept showed the acquired efficiency for blank water, WTiO2, and BTiO2 film was 25%, 29.26%, and 77.14% (Figures 5(2)(A)), respectively, under 1 Sun illumination. In an efficiency study, a BTiO2 film was stable over 10 cycles (1 h each) with high mechanical stability and robustness (Figure 5(2)(B, C, D)).
Zheng et al. (2020) prepared a black/reduced (tunable) colored TiO2 with defective sites and Ti3+/oxygen vacancies (named DP-X sample) by NaN3 deflagration using an ultrafast and one-step method while maintaining the intrinsic anatase TiO2 structure (Figure 5(3)). They synthesized different DP-X samples with an adjustable amount of NaN3 possessing a diversified light absorption range. The presence of these defective Ti3+/oxygen vacancies modified the intrinsic energy band and increased the light absorption capacity in the solar spectrum. Based on the evaporation rates and light conversion efficiency of DP films, the solar conversion efficiency of the DP-3 sample was highest at 85%, with a steady-state evaporation rate of about 1.624 kg m−2h−1 under 1 Sun illumination.
Previous promising results revealed that black/reduced titania sheets paired with a thermal insulating medium and a water supply line might achieve a photo-thermal energy efficiency of 90%, although the device becomes more complex due to the usage of thermal insulators such as polystyrene foam and a water supply line. Without thermal insulating management and a water supply path, the evaporation efficiency of the black/reduced titania sheet is limited to 50%–71%. As a result, a simple, self-insulating, self-watering, and easier-to-use and deploy assembly of PMs/systems with high mechanical stability/flexibility for effective solar steam generation is required (Zada et al., 2020). Table 1 summarizes the various studies on black/reduced TiO2 using a potential photo-thermal material with a higher solar conversion efficiency.
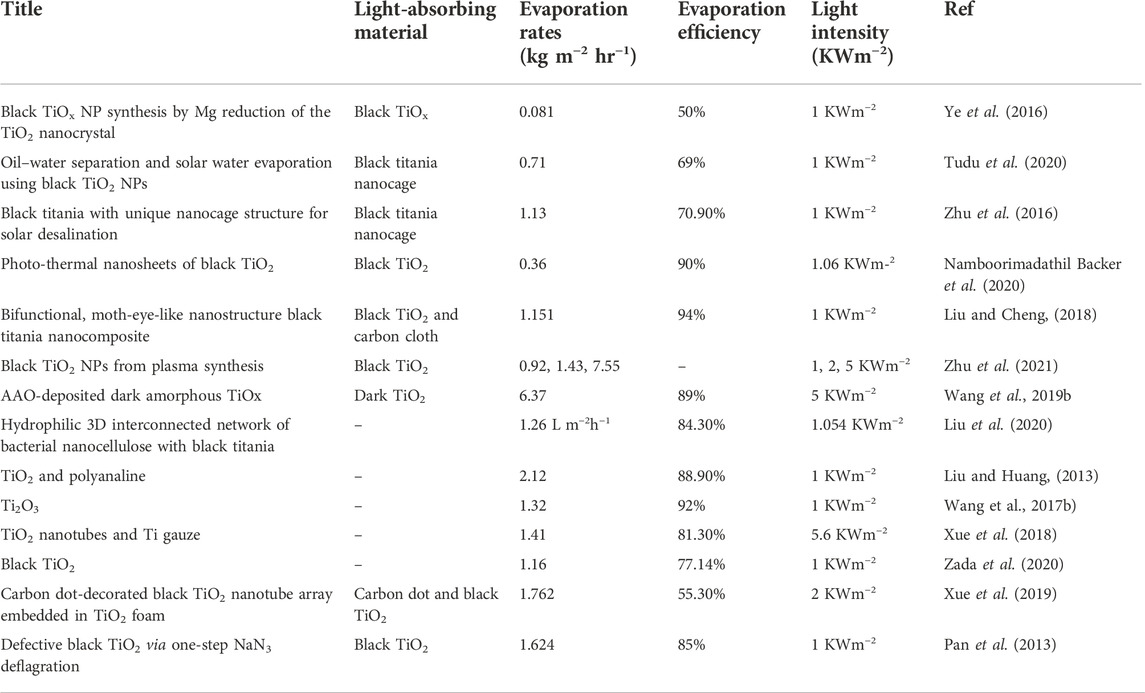
TABLE 1. Different TiO2 and black/reduced TiO2 materials with their solar evaporation and efficiency rates.
3.3 TiO2 with enhanced performance using hybridized materials
3.3.1 TiO2 in combination with plasmonic materials
Plasmonic-based photo-thermal therapy represents a promising approach toward solar water evaporation through their noble metal nanostructure (Chen H. et al., 2010). This nanostructure has great application in the enlargement of the absorption bandwidth, effectively enhancing the localized thermal energy conversion capacity and significantly improving the photo-thermal conversion efficiency as a whole (Zhou et al., 2016) (Søndergaard et al., 2012) (Aydin et al., 2011). When noble metallic NPs of different sizes are simulated by incident light of a particular wavelength, free electrons oscillate and generate an electric field, which assists plasmonic oscillation around NPs, known as localized surface plasmon resonance (LSPR) (Figure 6). This LSPR effect of plasmonic metal strongly amplifies the light absorption and thermal conversion efficiency, which enhances the complete photo-thermal effect. Some metallic NPs such as Au and Ag are commonly used materials for photo-thermal conversion due to their high LSPR response toward incident light (Lalisse et al., 2015) (Chou and Chen, 2014).
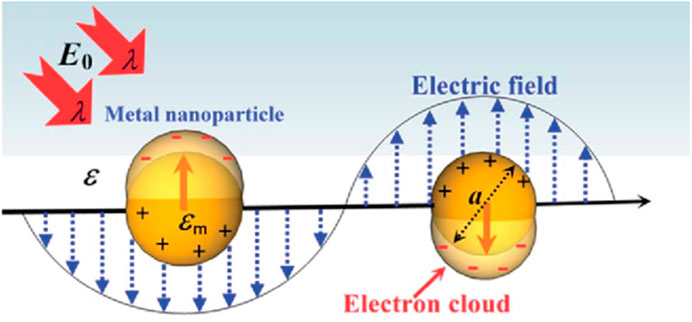
FIGURE 6. Systematic demonstration of light responses of plasmonic materials (Chou and Chen, 2014); Reproduced with permission. Copyright 2014 Royal Society of Chemistry.
However, these metallic NPs only utilize a portion of the solar spectrum due to the narrow spectra of the LSPR band. To broaden the spectra of the LSPR band, different sized NPs were assembled onto a porous film and hybridized with other substrates (X. Wu et al., 2019). First, Wang et al. (2014) used a plasmon-based free-floating AuNP membrane to enhance the evaporation rate and control the process temperature leading to a self-assembled, recyclable AuNP porous film utilizing the maximum NPs in evaporation.
Furthermore, the bifunctional membrane incorporation of TiO2 NPs has been developed to improve the evaporation rate. For example, Liu et al. (2016) prepared a bifunctional TiO2-Au-AAO membrane consisting of an Au NP plasmonic photo-thermal layer in combination with a TiO2 NP photocatalytic layer that has functions in solar water evaporation and photocatalytic degradation. These layers are supported by an anodized Al2O3 coating (AAO) that produces clean water through localized heating using simulated solar light. The AAO layer covered with TiO2 NPs shows good absorbance in the low wavelength range. Therefore, laying Au NPs underneath the TiO2 NP layer increases light absorbance capacity due to its plasmonic nature (Figure 7(1)). The absorption capacity also decreases, changing the amount of TiO2 NPs in the layer. Along with the water evaporation capability of the prepared photo-thermal material and due to the photocatalytic activity of TiO2, the remaining water in the beaker is purified. Therefore, this bifunctional design offers a revolutionary method for water filtration, as well as an alternative strategy for maximizing solar energy conversion and consumption.
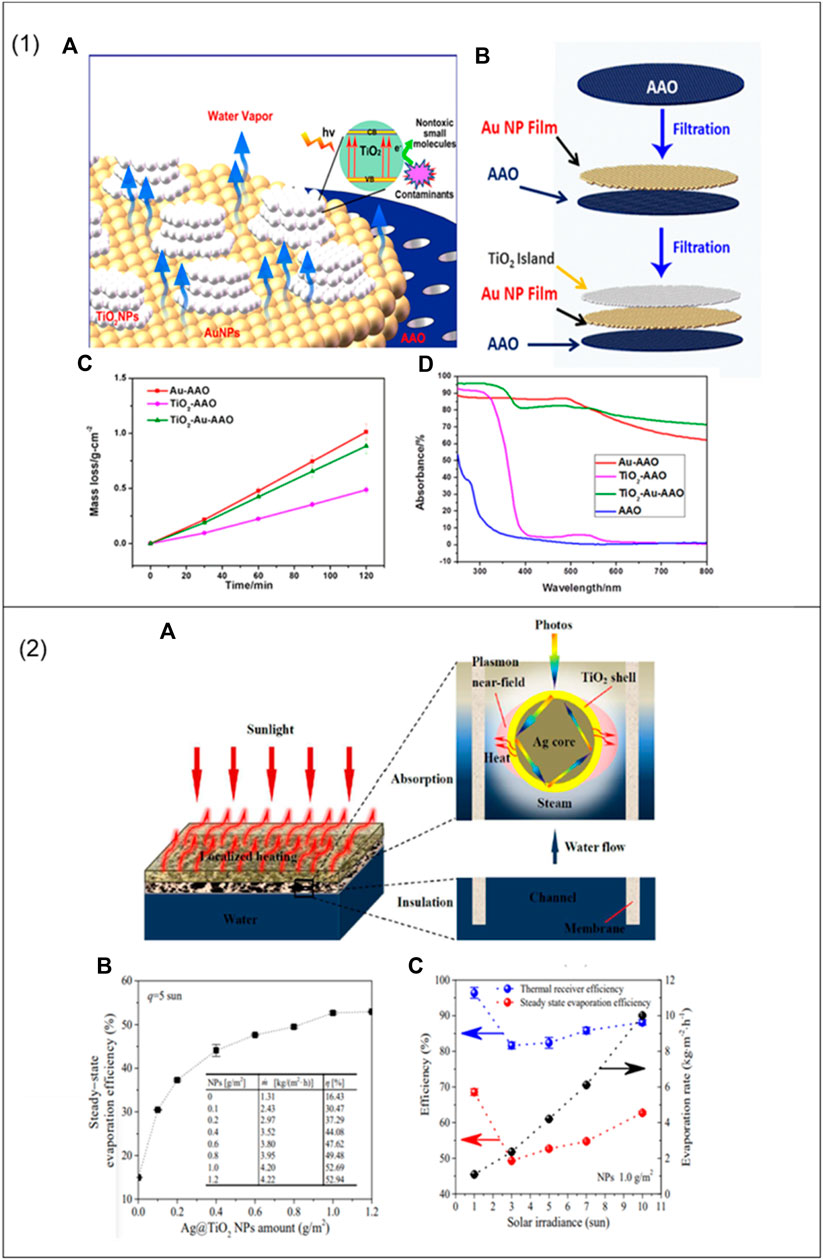
FIGURE 7. (1) (A) Structure of three layers incorporated into a bi-functional photo-thermal TiO2-Au-AAO membrane. (B) Demonstration of a bifunctional membrane. (C) Evaporation of different samples in the presence of simulated solar light. (D) Absorption spectra of different samples (Liu et al., 2016); Reproduced with permission. Copyright 2016 American Chemical Society. (2) (A) Illustration of a schematic of the photo-thermal conversion process. (B) Evaporation efficiency with Ag@TiO2 NP amounts. (C) Evaporation rate, evaporation efficiency, and thermal receiver efficiency under steady-state conditions as functions of solar irradiance (Li et al., 2017b); Reproduced with permission. Copyright 2017 Elsevier.
Moreover, different methods were recently developed for the preparation of metal@TiO2 core-shell NPs. In particular, Huang et al., 2017a synthesized a compound Au@TiO2 core-shell NP film by the vacuum filtration method, in which the core had Au NPs spherically surrounded by TiO2 shells. In the prepared Au@TiO2 film, both the photocatalysis and water evaporation advantages were linked due to the presence of Au and TiO2 NPs. When compared with other NP suspensions, the Au@TiO2 film was better than the former methods at solar water evaporation.
Despite the advantageous surface plasmon resonance effect, Au NPs are seriously limited for solar evaporation due to their high cost and availability. Another promising nanosized plasmonic metal, Ag, showed an excellent LSPR effect and had strong absorbance at a lower price (Liu and Huang, 2013). However, Ag NPs have a drawback, that is, they easily get oxidized when exposed to air. To avoid this, Ag NPs are embedded with metal oxides (Awazu et al., 2008). For instance, Li et al. (2017a) synthesized a flexible Ag@TiO2 NPs floatable membrane by vacuum drawing and filtration processes, which provided more localized heating. It was observed that metal coated with semiconductor oxide (TiO2) could widen the bandwidth of plasmonic NPs and successfully enhance their absorption capability (Figure 7(2)(A)). A water evaporation experiment performed at room temperature by floating the self-assembled NP membrane (NPM) showed that the solar evaporation performance of low conductivity and densely deposited NP membrane around 1.0 gm−2 resulted in a high water evaporation efficiency of 69.2% under 1 Sun illumination (Figures 7(2)(B, C)). Also, the effects of different factors, including the density of NPM, light intensity, and membrane durability in evaporation efficiency, were analyzed.
Although the noble metallic NPs have much potential for photo-thermal conversion, the high cost of the raw material prevents their mass commercial use, which unavoidably restricts their industrial applications. Other than the comparatively expensive noble metals aforementioned, other selective materials such as black chromium, copper, or black nickel have been developed and studied as solar selective coatings for heating fluids with improved photo-thermal solar energy conversion efficiency but with a cheap cost (X. Wu et al., 2019).
3.3.2 TiO2 with carbonaceous materials
Carbon-based materials such as photo-thermal materials are advantageous due to their high light absorption capacity over the entire solar spectrum. Due to their strong π-bond optical transition, black carbon materials are the most promising light-absorbing material. Black carbon, graphite, graphene, and carbon nanotubes are carbon-based materials commonly used in SWIE. Of these, the 3D carbon nanotubes are the pre-eminent form possessing low density, high optical absorbance rate, excellent light-to-heat conversion efficiency, and good mechanical and thermal stability (X. Wu et al., 2019). Despite the high absorption range, further enhancement is needed to increase their intensity and minimize surface reflection. Hybrid structures, such as plasmonic or semiconductor particle-coated nanocarbon materials, were developed to increase the photo-thermal effect further (Manuscript, 2018a) (Liu et al., 2015). Previous studies demonstrated that the modification of TiO2 with a carbon material enhanced the optical absorption efficiency and improved the stability of the system.
Wang et al. (2019a) prepared a carbon-based photo-thermal material by annealing a fruit residue and deposited the carbon material on a porous amorphous TiO2 layer. The amorphous TiO2 porous layer has sufficient photo-thermal conversion and water evaporation properties but has limited light absorption in the UV region. Therefore, the TiO2 layer was deposited with carbon NPs by hydrothermal-assisted annealing treatment of fruit residues (Figure 8(2)). A membrane generated from grape residues showed a high evaporation rate and photo-thermal efficiency of about 1.42 kg m−2 h−1 and 59.43%, respectively, (Figures 8(2)(A, B)) under 1.5 Sun (Figure 8(2)(C)) light irradiation along with excellent light absorption capacity in the UV–Vis–NIR region.
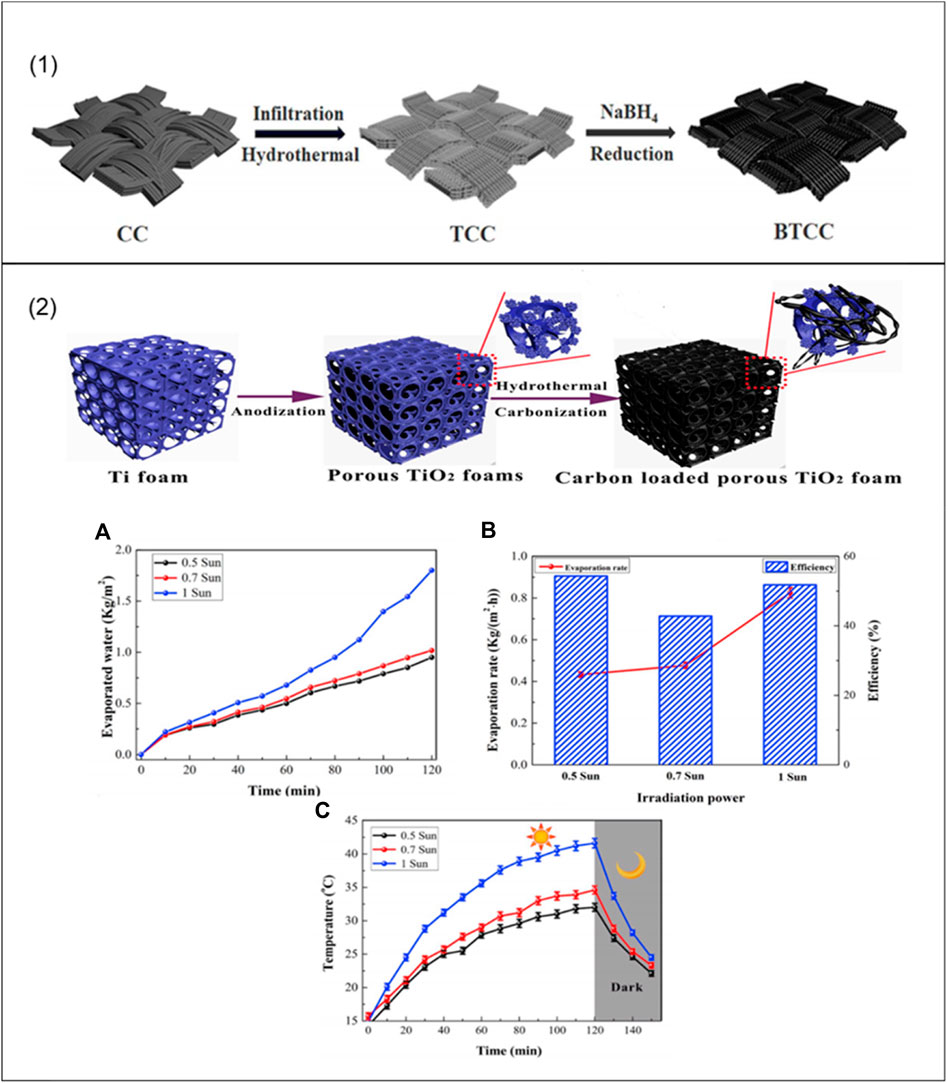
FIGURE 8. (1) Demonstration of the preparation of a BTCC nanocomposite (Liu and Cheng, 2018); Reproduced with permission. Copyright 2018 American Society of Chemistry. (2) (A) Solar water evaporation performance. (B) Evaporation rates and conversion efficiency. (C) Water temperature with time (Wang et al., 2019b); Reproduced with permission. Copyright 2019 Elsevier.
Black/reduced TiO2 can be modified using carbon-based materials. For this, Liu and Cheng (2018) fabricated a moth eye-inspired black titania nanostructure on carbon cloth (BTCC). Three steps were used to prepare the BTCC via infiltration, followed by hydrothermal and sodium borohydride reactions (Figure 8(1)).
The bifunctional BTCC-prepared nanocomposite produces clean water via solar steam generation and photocatalytic degradation. These TiO2-based nanorod structures provide a large number of active sites for photocatalytic reactions, and the carbon cloth transmits electrons and reduces electron hole recombination. The originating oxygen vacancies, surface disorder, and productive light absorption path of the BTCC nanocomposite allow full spectrum absorption for solar evaporation and photocatalytic processes with evaporation efficiency and vaporization rates of 94% and 1.515 kg m−2 h−1 at 1 KW m−2 light illumination, respectively. The new concept and strategy of water purification with bifunctional applications with improved efficiency provides a promising and effectual solution for clean water production.
3.3.3 TiO2 with co-semiconductors
The combination of solar-driven water evaporation and photodegradation functions expands the useful solar light range and generates pure water with high conversion efficiency. Solar-driven water evaporation and photocatalysis were improved by microstructure engineering. Significant attempts have recently been undertaken to enhance solar energy usage by regulating the microstructures of solar absorbers (Yuan et al., 2021). Regarding this, Ren and Yang (2019) designed and synthesized a bifunctional TiO2 nanotube mesh decorated with Cu2-xSe (CTNM). For proper utilization of solar light by CTNM, two mechanisms were used, namely LSPR (Herzog et al., 2014) and electron transmission (Yang et al., 2009). The bifunctional CTNM generates pure and purified water through a combination of solar water evaporation (photo-thermal conversion) with a photodegradation function. The photogenerated hot electrons aid the photodegradation reaction on the CB of TiO2 and Cu2-xSe (Figure 9(1)). Using an optimum amount of Cu2-xSe can improve light absorption, and the relative conversion efficiency was near 80% under 2.5 kW m−2. Along with evaporation, a combination of photo-thermal water evaporation and photocatalysis reduced the concentration of the dye in the water body, reducing the negative environmental impact of the concentrated effluent.
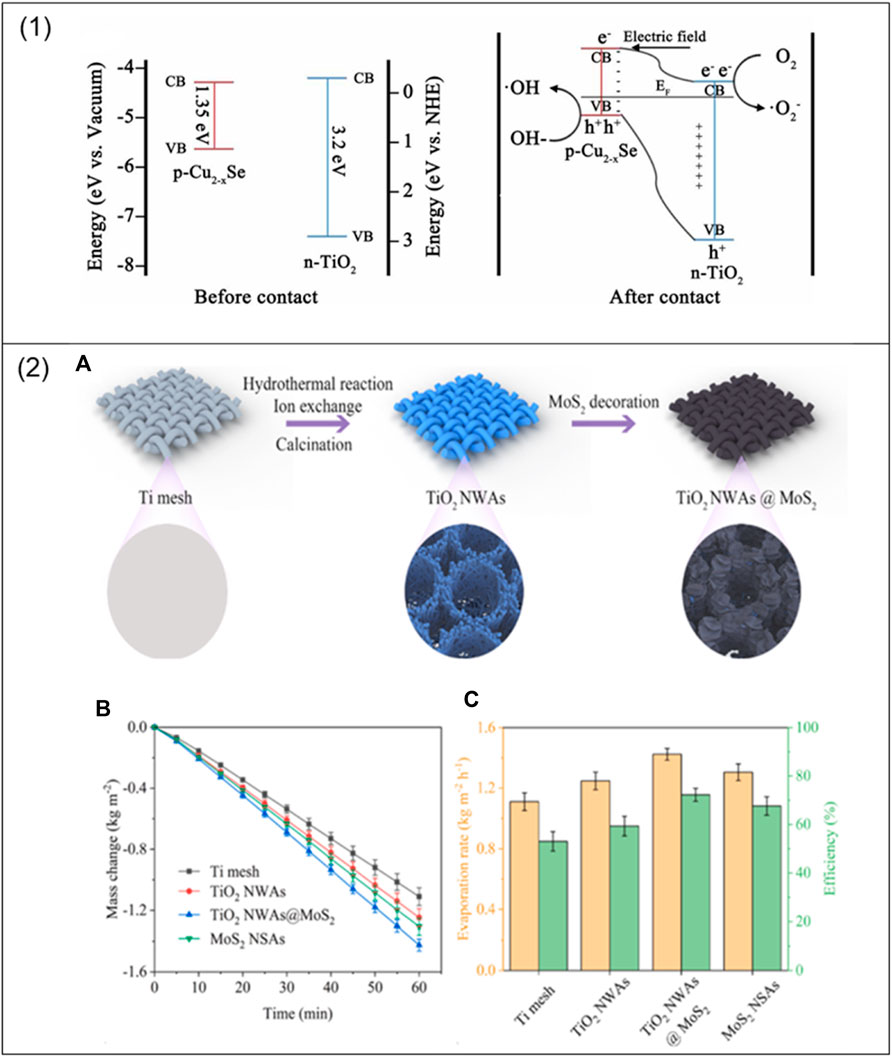
FIGURE 9. (1) Energy band structure diagrams of Cu2-xSe and TiO2 before and after contact. (CB: conduction band, VB: valence band, NHE: normal hydrogen electrode) (Ren et al. 2019); Reproduced with permission. Copyright 2019 IWA publishing. (2) (A) Descriptive illustration of the preparation process of TiO2 NWAs @ MoS2. (B, C) Comparative results of mass change, evaporation rates, and efficiency of different samples (Yuan et al., 2021); Reproduced with permission. Copyright 2021 Elsevier.
Solar absorbers with a broader wavelength were also designed using a multistage ultrathin MoS2 nanosheet decorated with TiO2 nanowires (TiO2@MoS2) by microstructure engineering (Figure 9(2)(A)). This combination amplified the photo-absorption capacity with an absorption of 96.5% and evaporation rate of 1.42 Kg m−2 h−1 (Figures 9(2)(B, C)) (Yuan et al., 2021), respectively. To broaden the light absorption of solar absorbers, Sun et al. (2020) co-workers developed an effective hydrogel-based evaporator by assembling MCNT-TiO2-SiO2-TiN. They showed that this evaporator had low thermal conductivity and high light-to-heat conversion capacity for interfacial evaporation. Due to these properties, the microporous hydrogel-based evaporator had 77.39% evaporation efficiency with a 1.853 Kg m−2 h−1 evaporation rate. In addition, Tian et al. (2022) fabricated TiO2-loaded CuO nanowire-covered Cu foam (TiO2-CuO-Cufoam) simultaneously, demonstrating a high solar evaporation efficiency of 86.6% with about 80.0% VOCs removal efficiency under 1 Sun irradiation. Yuan et al. (2021) presented a hierarchical 1D/2D TiO2 @ MoS2 core–shell network on a Ti mesh, which had efficient solar-driven interfacial water evaporation and pollutant removal with a high light absorption of 96.5% and a water evaporation rate of 1.42 kg m−2 h−1 under 1 Sun.
The clear benefits of this type of connection include increased energy and water productivity without affecting the ecological footprint. Furthermore, multifunctional photo-thermal materials may increase solar energy usage and conversion efficiency through suitable hybrid applications. Despite their high absorption properties, the preparation of this hybrid material is complex and expensive. Table 2 summarizes the various studies on hybridized TiO2 photo-thermal materials projecting comparative solar conversion efficiency.
3.4 TiO2 in solar stills - a virtual practice
A solar still is a simple and low-productive water purification technique used in rural areas. Traditional solar stills have low efficiency due to insufficient light absorption, evaporation, and condensation with large heat dissipation. Recently, micro/nanotechnology has gained attention in improving the performance of solar stills. Many researchers reported studies to improve the performance of solar stills. For instance, a group of researchers investigated the effect of NPs mixed with black/reduced paint on solar stills and found that their efficiency increased by 12.18% (Sain and Kumawat, 2015). Elango et al. (2015) provided a comparative study of solar stills with and without nanofluids and reported a performance increase of 18.63%.
Recently, Shanmugan et al. (2020) prepared a hybrid solar still coated with TiO2 mixed with Cr2O3 on a basin liner. The experiment compared the performance between with or without nanolayer composition on a copper sheet at a constant water depth in the presence of sunlight. A thermodynamic analysis of the heat and mass transfer of newly designed single-slope single-basin solar stills was tested with some assumptions. The presence of a nanolayer (Cr2O3-TiO2) increased the basin temperature during sunshine hours and in the summer and winter. Using a nanolayer coating, a maximum daily yield of 6.1 kg m−2 hr−1 and 4.1 kg m−2 hr−1 was achieved, with efficiency of 57.16% and 36.69% in the summer and winter, respectively (Figures 10A, B). Similar investigations were carried out studying the performance of TiO2 at variable water depths and concentrations of nanomaterials with/without black paint under active and passive solar stills (Parikh et al., 2021) (Kabeel et al., 2019). A comparative study of TiO2 NPs with others, such as Ag and Au, revealed better performance in terms of economic and exergoeconomic factors (Kabeel et al., 2019).
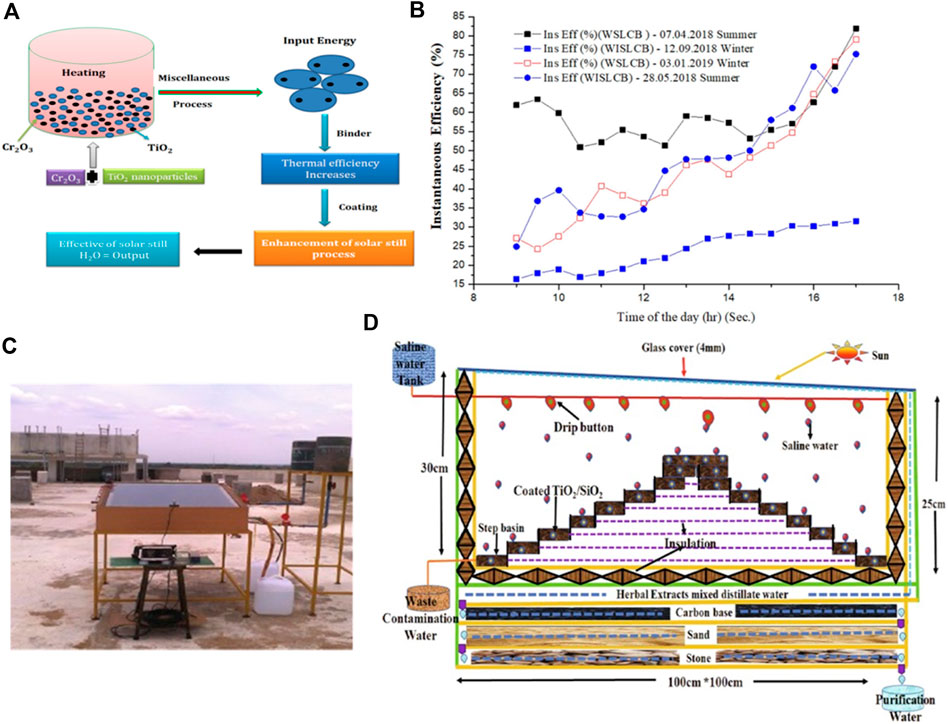
FIGURE 10. (A) Schematic preparation of TiO2 NPs and Cr2O3 composite coating of a solar still. (B) Variation in absorption efficiency during sunshine hours with and without a nanolayer coating. (WSLCB—with solar liquid coating basin area, WISLCB—without solar liquid coating basin area) (Shanmugan et al., 2020); Reproduced with permission. Copyright 2020 Elsevier. SiO2/TiO2 nanolayer-coated steeped basin solar still (SBSS). (C) Experimental setup view of an SBSS. (D) Schematic diagram of SBSS (Gandhi et al., 2022); Reproduced with permission. Copyright 2022 Elsevier.
Recently, a study reported a natural distiller in the form of an advanced running steeped solar basin coated with a SiO2/TiO2 nanolayer with an effective thermal conductive ratio of 30% (Figures 10C, D). This nano-coated solar basin exhibited a thermal efficiency of 40.241%, with a collected water outlet of about 5.893 kg m−3 day−1. Moreover, herbal extracts added to the system helped regulate the temperature and high-purity water collection with a view of providing microbe-free drinking water to the masses (Gandhi et al., 2022).
4 Conclusions and future aspects
In the past few decades, solar-driven evaporation techniques have undergone significant advancement. Here, we reviewed the recent improvements made to high-performance TiO2-based photo-thermal materials. For efficient solar water evaporation, various light absorbing materials such as black/reduced TiO2 NPs, TiO2 synthesized nanostructures with plasmonic materials, carbon, and other semiconductors, and TiO2 with different structural engineering methods have been summarized. Each absorbing material has its own mechanism, absorbing capacity, stability, strengths, and limitations. All of these methods effectively enhanced solar water evaporation rates and conversion efficiency. Notably, black/reduced TiO2 NPs exhibited excellent solar evaporation efficiency.
Moreover, hybrid structures and multifunctional photo-thermal materials may promote solar evaporation and conversion efficiency. Although there has been significant progress in TiO2-based materials over the last few decades, research on TiO2 materials related to evaporation is still in the early stages. To further increase performance, some challenges need to be overcome.
First, despite high evaporation rates and efficiency (approximately 90%), due to changing environmental conditions such as temperature, humidity, wind, and lack of standard setup, the method used to determine parameters and specific calculations for efficiency needs to be investigated further. Second, studies of the structural properties and fundamental dynamic kinetics of newly designed materials are still limited. It should be noted that water transportation from any surface is based on its pore structure and surface properties like hydrophobicity and thermal diffusion. In addition to experimental observations, simulation is another powerful tool that can be used to optimize the fundamental behavior, the kinetics of light, water, and vapor, and thermal diffusion. Third, less attention has been paid to the long-term stability and durability of light-absorbing materials in different water sources such as industrial water, seawater, and river water. To avoid this problem, structural integration and pore engineering should be introduced to establish highly stable, recyclable, and compatible solar-absorbing materials. Fourth, VOCs present in the water will evaporate and condense on the absorbing surface, causing fouling and clogging. Accordingly, a robust photo-thermal structure appropriately designed will surpass the evaporation of organic components. Overall, the use of modified TiO2 (capable of light absorption in the entire solar spectrum) is one of the most promising strategies, and further research focusing on the engineering of SWIE is required.
Author contributions
All authors listed have made a substantial, direct, and intellectual contribution to the work and approved it for publication.
Conflict of interest
The authors declare that the research was conducted in the absence of any commercial or financial relationships that could be construed as a potential conflict of interest.
Publisher’s note
All claims expressed in this article are solely those of the authors and do not necessarily represent those of their affiliated organizations, or those of the publisher, the editors, and the reviewers. Any product that may be evaluated in this article, or claim that may be made by its manufacturer, is not guaranteed, or endorsed by the publisher.
References
Awazu, K., Fujimaki, M., Rockstuhl, C., Tominaga, J., Murakami, H., Ohki, Y., et al. (2008). A plasmonic photocatalyst consisting of silver nanoparticles embedded in titanium dioxide. J. Am. Chem. Soc. 130 (5), 1676–1680. doi:10.1021/ja076503n
Aydin, K., Ferry, V. E., Briggs, R. M., and Atwater, H. A. (2011). Broadband polarization-independent resonant light absorption using ultrathin plasmonic super absorbers. Nat. Commun. 2 (1), 517–7. doi:10.1038/ncomms1528
Bae, K., Kang, G., Cho, S. K., Park, W., Kim, K., and Padilla, W. J. (2015). Flexible thin-film black gold membranes with ultrabroadband plasmonic nanofocusing for efficient solar vapour generation. Nat. Commun. 6, 10103. doi:10.1038/ncomms10103
Bai, H., Zhao, T., and Cao, M. (2020). Interfacial solar evaporation for water production: From structure design to reliable performance. Mol. Syst. Des. Eng. 5 (2), 419–432. doi:10.1039/C9ME00166B
Basavarajappa, P. S., Patil, S. B., Ganganagappa, N., Reddy, K. R., Raghu, A. V., and Reddy, C. V. (2020). Recent progress in metal-doped TiO2, non-metal doped/codoped TiO2 and TiO2 nanostructured hybrids for enhanced photocatalysis. Int. J. Hydrogen Energy 45, 7764–7778. doi:10.1016/j.ijhydene.2019.07.241
Cao, S., Jiang, Q., Wu, X., Ghim, D., Gholami Derami, H., Chou, P. I., et al. (2019). Advances in solar evaporator materials for freshwater generation. J. Mat. Chem. A Mat. 7 (42), 24092–24123. doi:10.1039/c9ta06034k
Carp, O., Huisman, C. L., and Reller, A. (2004). Photoinduced reactivity of titanium dioxide, 32, 33–177. doi:10.1016/j.progsolidstchem.2004.08.001
Chen, C., Kuang, Y., and Hu, L. (2019). Challenges and opportunities for solar evaporation. Joule 3 (3), 683–718. doi:10.1016/j.joule.2018.12.023
Chen, H., Shao, L., Ming, T., Sun, Z., Zhao, C., Yang, B., et al. (2010a). Understanding the photothermal conversion efficiency of gold nanocrystals. Small 6 (20), 2272–2280. doi:10.1002/smll.201001109
Chen, K. Y., Lai, W. W. P., Wang, H. J., Lin, C. C., Chen, C. W., and Lin, A. Y. C. (2021). Clean water generation through a multifunctional activated carbon-TiO2interfacial solar distillation system. RSC Adv. 11 (37), 23036–23044. doi:10.1039/d1ra02185k
Chen, X., Liu, L., Yu, P. Y., and Mao, S. S. (2011). Increasing solar absorption for photocatalysis with black hydrogenated titanium dioxide nanocrystals. Science 331 (6018), 746–750. doi:10.1126/science.1200448
Chen, X., Meng, C., Wang, Y., Zhao, Q., Li, Y., Chen, X.-M., et al. (2020). Laser-synthesized rutile TiO2 with abundant oxygen vacancies for enhanced solar water evaporation. ACS Sustain. Chem. Eng. 8 (2), 1095–1101. doi:10.1021/acssuschemeng.9b05952
Chou, C. H., and Chen, F. C. (2014). Plasmonic nanostructures for light trapping in organic photovoltaic devices. Nanoscale 6 (15), 8444–8458. doi:10.1039/c4nr02191f
Ding, T., Zhou, Y., Ong, W. L., and Ho, G. W. (2021). Hybrid solar-driven interfacial evaporation systems: Beyond water production towards high solar energy utilization. Mater. Today 42, 178–191. doi:10.1016/j.mattod.2020.10.022
Dong, B. Q., Liu, X. H., Zhan, T. R., Jiang, L. P., Yin, H. W., Liu, F., et al. (2010). Structural coloration and photonic pseudogap in natural random close-packing photonic structures. Opt. Express 18 (14), 14430. doi:10.1364/oe.18.014430
Dong, Y., Tan, Y., Wang, K., Cai, Y., Li, J., Sonne, C., et al. (2022). Reviewing wood-based solar-driven interfacial evaporators for desalination. Water Res. 223, 119011. doi:10.1016/j.watres.2022.119011
Elango, T., Kannan, A., and Kalidasa Murugavel, K. (2015). Performance study on single basin single slope solar still with different water nanofluids. Desalination 360, 45–51. doi:10.1016/j.desal.2015.01.004
Fan, C., Chen, C., Wang, J., Fu, X., Ren, Z., Qian, G., et al. (2015). Black hydroxylated titanium dioxide prepared via ultrasonication with enhanced photocatalytic activity. Sci. Rep. 5 (1), 11712. doi:10.1038/srep11712
Gandhi, A. M., Shanmugan, S., Kumar, R., Elsheikh, A. H., Sharifpur, M., Bewoor, A. K., et al. (2022). SiO2/TiO2 nanolayer synergistically trigger thermal absorption inflammatory responses materials for performance improvement of stepped basin solar still natural distiller. Sustain. Energy Technol. Assessments 52, 101974. doi:10.1016/j.seta.2022.101974
Gao, M., Zhu, L., Peh, C. K., and Ho, G. W. (2019). Solar absorber material and system designs for photothermal water vaporization towards clean water and energy production. Energy Environ. Sci. 12 (3), 841–864. doi:10.1039/C8EE01146J
Geng, Y., Zhang, K., Yang, K., Ying, P., Hu, L., Ding, J., et al. (2019). Constructing hierarchical carbon framework and quantifying water transfer for novel solar evaporation configuration. Carbon 155, 25–33. doi:10.1016/j.carbon.2019.08.055
Ghim, D., Jiang, Q., Cao, S., Singamaneni, S., and Jun, Y. S. (2018). Mechanically interlocked 1T/2H phases of MoS2 nanosheets for solar thermal water purification. Nano Energy 53, 949–957. doi:10.1016/j.nanoen.2018.09.038
Guan, W., Guo, Y., and Yu, G. (2021). Carbon materials for solar water evaporation and desalination. Small 17 (48), 2007176. doi:10.1002/smll.202007176
Guo, Z., Wang, G., Ming, X., Mei, T., Wang, J., Li, J., et al. (2018). PEGylated self-growth MoS2 on a cotton cloth substrate for high-efficiency solar energy utilization. ACS Appl. Mat. Interfaces 10 (29), 24583–24589. doi:10.1021/acsami.8b08019
Gurbatov, S. O., Modin, E., Puzikov, V., Tonkaev, P., Storozhenko, D., Sergeev, A., et al. (2021). Black Au-decorated TiO2Produced via laser ablation in liquid. ACS Appl. Mat. Interfaces 13 (5), 6522–6531. doi:10.1021/acsami.0c20463
Han, X., Ding, S., Hu, H., and Wang, S. (2022). Recent advances in structural regulation and optimization of high-performance solar-driven interfacial evaporation systems. J. Mat. Chem. A Mat. 10 (36), 18509–18541. doi:10.1039/D2TA05666F
He, F., Wu, X., Gao, J., and Wang, Z. (2021). Solar-driven interfacial evaporation toward clean water production: Burgeoning materials, concepts and technologies. J. Mat. Chem. A Mat. 9 (48), 27121–27139. doi:10.1039/D1TA08886F
Herzog, J. B., Knight, M. W., and Natelson, D. (2014). Thermoplasmonics: Quantifying plasmonic heating in single nanowires. Nano Lett. 14 (2), 499–503. doi:10.1021/nl403510u
Hessel, C. M., Pattani, V. P., Rasch, M., Panthani, M. G., Koo, B., Tunnell, J. W., et al. (2011). Copper selenide nanocrystals for photothermal therapy. Nano Lett. 11 (6), 2560–2566. doi:10.1021/nl201400z
Hsieh, Y. Y., and Lin, T. F. (2003). Evaporation heat transfer and pressure drop of refrigerant R-410A flow in a vertical plate heat exchanger. J. Heat. Transf. 125 (5), 852–857. doi:10.1115/1.1518498
Hua, Z., Li, B., Li, L., Yin, X., Chen, K., and Wang, W. (2017). Designing a novel photothermal material of hierarchical microstructured copper phosphate for solar evaporation enhancement. J. Phys. Chem. C 121 (1), 60–69. doi:10.1021/acs.jpcc.6b08975
Huang, J., He, Y., Chen, M., Jiang, B., and Huang, Y. (2017b). Solar evaporation enhancement by a compound film based on Au@TiO2 core–shell nanoparticles. Sol. Energy 155, 1225–1232. doi:10.1016/j.solener.2017.07.070
Huang, J., He, Y., Wang, L., Huang, Y., and Jiang, B. (2017a). Bifunctional Au@TiO2 core–shell nanoparticle films for clean water generation by photocatalysis and solar evaporation. Energy Convers. Manag. 132, 452–459. doi:10.1016/j.enconman.2016.11.053
Huang, J., Hu, Y., Bai, Y., He, Y., and Zhu, J. (2020). Novel solar membrane distillation enabled by a PDMS/CNT/PVDF membrane with localized heating. Desalination 489, 114529. doi:10.1016/j.desal.2020.114529
Ito, Y., Tanabe, Y., Han, J., Fujita, T., Tanigaki, K., and Chen, M. (2015). Multifunctional porous graphene for high-efficiency steam generation by heat localization. Adv. Mat. 27 (29), 4302–4307. doi:10.1002/adma.201501832
Jiang, F., Li, Y., Zhang, Y., Gong, A., Dai, J., Hitz, E., et al. (2017). Wood-based nanotechnologies toward sustainability. Adv. Mat. 30 (1), 1703453. doi:10.1002/adma.201703453
Kabeel, A. E., Sathyamurthy, R., Sharshir, S. W., Muthumanokar, A., Panchal, H., Prakash, N., et al. (2019). Effect of water depth on a novel absorber plate of pyramid solar still coated with TiO2 nano black paint. J. Clean. Prod. 213, 185–191. doi:10.1016/j.jclepro.2018.12.185
Kamat, P. V. (2008). Quantum dot solar cells. Semiconductor nanocrystals as light harvesters. J. Phys. Chem. C 112 (48), 18737–18753. doi:10.1021/jp806791s
Kanakaraju, D., anak Kutiang, F. D., Lim, Y. C., and Goh, P. S. (2022). Recent progress of Ag/TiO2 photocatalyst for wastewater treatment: Doping, co-doping, and green materials functionalization. Appl. Mater. Today 27, 101500. doi:10.1016/j.apmt.2022.101500
Kim, S., Tahir, Z., Rashid, M. U., Jang, J. I., and Kim, Y. S. (2021). Highly efficient solar vapor generation via a simple morphological alteration of TiO2Films grown on a glassy carbon foam. ACS Appl. Mat. Interfaces 13 (43), 50911–50919. doi:10.1021/acsami.1c14247
Lalisse, A., Tessier, G., Plain, J., and Baffou, G. (2015). Quantifying the efficiency of plasmonic materials for near-field enhancement and photothermal conversion. J. Phys. Chem. C 119 (45), 25518–25528. doi:10.1021/acs.jpcc.5b09294
Lei, W., Khan, S., Chen, L., Suzuki, N., Terashima, C., Liu, K., et al. (2021). Hierarchical structures hydrogel evaporator and superhydrophilic water collect device for efficient solar steam evaporation. Nano Res. 14 (4), 1135–1140. doi:10.1007/s12274-020-3162-5
Li, C., Goswami, Y., and Stefanakos, E. (2013). Solar assisted sea water desalination: A review. Renew. Sustain. Energy Rev. 19, 136–163. doi:10.1016/j.rser.2012.04.059
Li, H., He, Y., Liu, Z., Huang, Y., and Jiang, B. (2017b). Synchronous steam generation and heat collection in a broadband Ag@TiO2 core–shell nanoparticle-based receiver. Appl. Therm. Eng. 121, 617–627. doi:10.1016/j.applthermaleng.2017.04.102
Li, H., He, Y., Liu, Z., Jiang, B., and Huang, Y. (2017a). A flexible thin-film membrane with broadband Ag@TiO2 nanoparticle for high-efficiency solar evaporation enhancement. Energy 139, 210–219. doi:10.1016/j.energy.2017.07.180
Li, J., Jing, Y., Xing, G., Liu, M., Cui, Y., Sun, H., et al. (2022). Solar-driven interfacial evaporation for water treatment: Advanced research progress and challenges. J. Mat. Chem. A Mat. 10 (36), 18470–18489. doi:10.1039/D2TA03321F
Li, R., Zhang, L., Shi, L., and Wang, P. (2017). MXene Ti3C2: An Effective 2D light-to-heat conversion material. ACS Nano, 11 (4), 3752–3759. doi:10.1021/acsnano.6b08415
Liu, G., Xu, J., and Wang, K. (2017). Solar water evaporation by black photothermal sheets. Nano Energy 41, 269–284. doi:10.1016/j.nanoen.2017.09.005
Liu, H., Zhang, X., Hong, Z., Pu, Z., Yao, Q., Shi, J., et al. (2017). A bioinspired capillary-driven pump for solar vapor generation. Nano Energy 42, 115–121. doi:10.1016/j.nanoen.2017.10.039
Liu, K. K., Jiang, Q., Tadepalli, S., Raliya, R., Biswas, P., Naik, R. R., et al. (2017). Wood-graphene oxide composite for highly efficient solar steam generation and desalination. ACS Appl. Mat. Interfaces 9 (8), 7675–7681. doi:10.1021/acsami.7b01307
Liu, L., Zhong, K., Munro, T., Alvarado, S., Cote, R., Creten, S., et al. (2015). Wideband fluorescence-based thermometry by neural network recognition: Photothermal application with 10 ns time resolution. J. Appl. Phys. 118 (18), 184906. doi:10.1063/1.4935277
Liu, X., Cheng, H., Guo, Z., Zhan, Q., Qian, J., and Wang, X. (2018). Bifunctional, moth-eye-like nanostructured black titania nanocomposites for solar-driven clean water generation. ACS Appl. Mat. Interfaces 10 (46), 39661–39669. doi:10.1021/acsami.8b13374
Liu, X., Hou, B., Wang, G., Cui, Z., Zhu, X., and Wang, X. (2018). Black titania/graphene oxide nanocomposite films with excellent photothermal property for solar steam generation. J. Mat. Res. 33 (6), 674–684. doi:10.1557/jmr.2018.25
Liu, Y., and Huang, C. Z. (2013). Screening sensitive nanosensors via the investigation of shape-dependent localized surface plasmon resonance of single Ag nanoparticles. Nanoscale 5 (16), 7458–7466. doi:10.1039/c3nr01952g
Liu, Y., Lou, J., Ni, M., Song, C., Wu, J., Dasgupta, N. P., et al. (2016). Bioinspired bifunctional membrane for efficient clean water generation. ACS Appl. Mat. Interfaces 8 (1), 772–779. doi:10.1021/acsami.5b09996
Liu, Y., Song, H., Bei, Z., Zhou, L., Zhao, C., Ooi, B. S., et al. (2021). Ultra-thin dark amorphous TiOx hollow nanotubes for full spectrum solar energy harvesting and conversion. Nano Energy 84, 105872. doi:10.1016/j.nanoen.2021.105872
Liu, Y., Wang, S., Zheng, P., Ding, M., and Yang, G. (2020). One-step synthesis of Ag-decorated Ti3+-doped TiO2 nanosheets with improved photocatalytic properties via deflagration method. Mater. Lett. 261, 127016. doi:10.1016/j.matlet.2019.127016
Liu, Z. H., Guan, H. Y., and Wang, G. S. (2014). Performance optimization study on an integrated solar desalination system with multi-stage evaporation/heat recovery processes. Energy 76, 1001–1010. doi:10.1016/j.energy.2014.09.017
Luo, Y.-Q., Liu, L., Zhao, L. R., Ju, J., and Yao, X. (2022). Advanced solar desalination on superwetting surfaces. J. Mat. Chem. A Mat. 10 (37), 19348–19366. doi:10.1039/D2TA03255D
Meng, X., Xu, W., Li, Z., Yang, J., Zhao, J., Zou, X., et al. (2020). Coupling of hierarchical Al2O3/TiO2 nanofibers into 3D photothermal aerogels toward simultaneous water evaporation and purification. Adv. Fiber Mat. 2 (2), 93–104. doi:10.1007/s42765-020-00029-9
Ming, X., Guo, A., Wang, G., and Wang, X. (2018). Two-dimensional defective tungsten oxide nanosheets as high performance photo-absorbers for efficient solar steam generation. Sol. Energy Mater. Sol. Cells 185, 333–341. doi:10.1016/j.solmat.2018.05.049
Naldoni, A., Allieta, M., Santangelo, S., Marelli, M., Fabbri, F., Cappelli, S., et al. (2012). Effect of nature and location of defects on bandgap narrowing in black TiO 2 nanoparticles. J. Am. Chem. Soc. 134 (18), 7600–7603. doi:10.1021/ja3012676
Namboorimadathil Backer, S., Ramachandran, A. M., Venugopal, A. A., Mohamed, A. P., Asok, A., and Pillai, S. (2020). Clean water from air utilizing black TiO2-based photothermal nanocomposite sheets. ACS Appl. Nano Mat. 3 (7), 6827–6835. doi:10.1021/acsanm.0c01207
Pan, X., Yang, M. Q., Fu, X., Zhang, N., and Xu, Y. J. (2013). Defective TiO2 with oxygen vacancies: Synthesis, properties and photocatalytic applications. Nanoscale 5 (9), 3601–3614. doi:10.1039/c3nr00476g
Parikh, R., Patdiwala, U., Parikh, S., Panchal, H., and Sadasivuni, K. K. (2021). Performance enhancement using TiO2 nano particles in solar still at variable water depth. Int. J. Ambient Energy 43, 4037–4044. doi:10.1080/01430750.2021.1873853
Pelaez, M., Nolan, N. T., Pillai, S. C., Seery, M. K., Falaras, P., Kontos, A. G., et al. (2012). A review on the visible light active titanium dioxide photocatalysts for environmental applications. Appl. Catal. B Environ. 125, 331–349. doi:10.1016/j.apcatb.2012.05.036
Ren, P., and Yang, X. (2019). Bifunctional Cu2-xSe-decorated hierarchical TiO2 nanotube mesh with solar water evaporation and photodegradation effects for clean water generation. Water Supply 19 (7), 2001–2008. doi:10.2166/ws.2019.076
Ren, P., Li, J., Zhang, X., and Yang, X. (2020). Highly efficient solar water evaporation of TiO2@TiN hyperbranched nanowires-carbonized wood hierarchical photothermal conversion material. Mater. Today Energy 18, 100546. doi:10.1016/j.mtener.2020.100546
Sain, M. K., and Kumawat, G. (2015). Performance enhancement of single slope solar still using. NANO- Part. Mix. BLACK PAINT’ 1 (1), 55–65.
Shang, W., and Deng, T. (2016). Solar steam generation: Steam by thermal concentration. Nat. Energy 1 (9), 16133. doi:10.1038/nenergy.2016.133
Shanmugan, S., Essa, F., Gorjian, S., Kabeel, A., Sathyamurthy, R., and Muthu Manokar, A. (2020). Experimental study on single slope single basin solar still using TiO2 nano layer for natural clean water invention. J. Energy Storage 30, 101522. doi:10.1016/j.est.2020.101522
Shayegan, Z., Lee, C.-S., and Haghighat, F. (2018). TiO2 photocatalyst for removal of volatile organic compounds in gas phase – a review. Chem. Eng. J. 334, 2408–2439. doi:10.1016/j.cej.2017.09.153
Sheng, M., Yang, Y., Bin, X., Zhao, S., Pan, C., Nawaz, F., et al. (2021). Recent advanced self-propelling salt-blocking technologies for passive solar-driven interfacial evaporation desalination systems. Nano Energy 89, 106468. doi:10.1016/j.nanoen.2021.106468
Shi, L., Huang, J., and He, Y. (2017). Recyclable purification-evaporation systems based on Fe3O4@TiO2 nanoparticles. Energy Procedia 142, 356–361. doi:10.1016/j.egypro.2017.12.056
Shi, L., Wang, Y., Zhang, L., and Wang, P. (2017). Rational design of a bi-layered reduced graphene oxide film on polystyrene foam for solar-driven interfacial water evaporation. J. Mat. Chem. A Mat. 5 (31), 16212–16219. doi:10.1039/c6ta09810j
Sinhamahapatra, A., Jeon, J.-P., and Yu, J.-S. (2015). A new approach to prepare highly active and stable black titania for visible light-assisted hydrogen production. Energy Environ. Sci. 8 (12), 3539–3544. doi:10.1039/C5EE02443A
Søndergaard, T., Novikov, S. M., Holmgaard, T., Eriksen, R. L., Beermann, J., Han, Z., et al. (2012). Plasmonic black gold by adiabatic nanofocusing and absorption of light in ultra-sharp convex grooves. Nat. Commun. 3, 969. doi:10.1038/ncomms1976
Su, C. Y., Lu, A. Y., Xu, Y., Chen, F. R., Khlobystov, A. N., and Li, L. J. (2011). High-quality thin graphene films from fast electrochemical exfoliation. ACS Nano 5 (3), 2332–2339. doi:10.1021/nn200025p
Sun, Z., Li, Z., Li, W., and Bian, F. (2020). Mesoporous cellulose/TiO2/SiO2/TiN-based nanocomposite hydrogels for efficient solar steam evaporation: Low thermal conductivity and high light-heat conversion. Cellulose 27 (1), 481–491. doi:10.1007/s10570-019-02823-0
Tian, Y., Yang, H., Wu, S., Gong, B., Xu, C., Yan, J., et al. (2022). High-performance water purification and desalination by solar-driven interfacial evaporation and photocatalytic VOC decomposition enabled by hierarchical TiO 2 -CuO nanoarchitecture. Int. J. Energy Res. 46 (2), 1313–1326. doi:10.1002/er.7249
Tom, R. T., Nair, A. S., Singh, N., Aslam, M., Nagendra, C. L., Philip, R., et al. (2003). Freely dispersible Au@TiO2, Au@ZrO2, Ag@TiO2, and Ag@ZrO2 core-shell nanoparticles: One-step synthesis, characterization, spectroscopy, and optical limiting properties. Langmuir 19 (8), 3439–3445. doi:10.1021/la0266435
Tudu, B. K., Gupta, V., Kumar, A., and Sinhamahapatra, A. (2020). Freshwater production via efficient oil-water separation and solar-assisted water evaporation using black titanium oxide nanoparticles. J. Colloid Interface Sci. 566, 183–193. doi:10.1016/j.jcis.2020.01.079
Ullattil, S. G., Narendranath, S. B., Pillai, S. C., and Periyat, P. (2018). Black TiO2 nanomaterials: A review of recent advances. Chem. Eng. J. 343, 708–736. doi:10.1016/j.cej.2018.01.069
Wang, B., Shen, S., and Mao, S. S. (2017). Black TiO2 for solar hydrogen conversion. J. Materiomics 3 (2), 96–111. doi:10.1016/j.jmat.2017.02.001
Wang, J., Li, Y., Deng, L., Wei, N., Weng, Y., Dong, S., et al. (2017a). High-performance photothermal conversion of narrow-bandgap Ti2O3 nanoparticles. Adv. Mater. 29 (3), 1603730. doi:10.1002/adma.201603730
Wang, J., Li, Y., Deng, L., Wei, N., Weng, Y., Dong, S., et al. (2017b). High-performance photothermal conversion of narrow-bandgap Ti2O3 nanoparticles. Adv. Mat. 29 (3), 1603730. doi:10.1002/adma.201603730
Wang, P., Zhang, J., Li, C., and Jin, Y. (2019a). A ternary Pt/Au/TiO2-decorated plasmonic wood carbon for high-efficiency interfacial solar steam generation and photodegradation of tetracycline. ChemSusChem 12, 467–472. doi:10.1002/cssc.201802485
Wang, Q., Qiu, L., Jia, Y., Chang, Y., Tan, X., Yang, L., et al. (2019b). Design of carbon loaded porous TiO2 foams by the hydrothermal-assisted annealing carbonization of fruit residue for solar-driven water evaporation. Sol. Energy Mater. Sol. Cells 202, 110116. doi:10.1016/j.solmat.2019.110116
Wang, X., Li, Z., Wu, Y., Guo, H., Zhang, X., Yang, Y., et al. (2021). Construction of a three-dimensional interpenetrating network sponge for high-efficiency and cavity-enhanced solar-driven wastewater treatment. ACS Appl. Mat. Interfaces 13 (9), 10902–10915. doi:10.1021/acsami.0c21690
Wang, X., He, Y., Liu, X., Cheng, G., and Zhu, J. (2017d). Solar steam generation through bio-inspired interface heating of broadband-absorbing plasmonic membranes. Appl. Energy 195, 414–425. doi:10.1016/j.apenergy.2017.03.080
Wang, Z., Liu, Y., Tao, P., Shen, Q., Yi, N., Zhang, F., et al. (2014). Bio-inspired evaporation through plasmonic film of nanoparticles at the air-water interface. Small 10 (16), 3234–3239. doi:10.1002/smll.201401071
Wu, D., Qu, D., Jiang, W., Chen, G., An, L., Zhuang, C., et al. (2019). Self-floating nanostructured Ni-NiO:X/Ni foam for solar thermal water evaporation. J. Mat. Chem. A Mat. 7 (14), 8485–8490. doi:10.1039/c9ta00529c
Wu, X., Chen, G. Y., Owens, G., Chu, D., and Xu, H. (2019). Photothermal materials: A key platform enabling highly efficient water evaporation driven by solar energy. Mater. Today Energy 12 (4), 277–296. doi:10.1016/j.mtener.2019.02.001
Xue, C., Hu, S., Chang, Q., Li, N., Wang, Y., Liu, W., et al. (2018). Air–water interface solar heating using titanium gauze coated with reduced TiO2 nanotubes. J. Mat. Sci. 53 (13), 9742–9754. doi:10.1007/s10853-018-2293-5
Xue, C., Huang, R., Xue, R., Chang, Q., Li, N., Zhang, J., et al. (2022). Design of ultrathin TiO2 nanosheets coated Ti plate for enhanced interfacial solar driven water evaporation performance. J. Alloys Compd. 909, 164843. doi:10.1016/j.jallcom.2022.164843
Xue, C., Li, D., Li, Y., Li, N., Zhang, F., Wang, Y., et al. (2019). 3D-carbon dots decorated black TiO2 nanotube Array@Ti foam with enhanced photothermal and photocatalytic activities. Ceram. Int. 45 (14), 17512–17520. doi:10.1016/j.ceramint.2019.05.313
Yan, J., Su, Q., Xiao, W., Wu, Z., Chen, L., Tang, L., et al. (2022). A review of nanofiber membranes for solar interface evaporation. Desalination 531, 115686. doi:10.1016/j.desal.2022.115686
Yang, X. C., Dong, Z. W., Liu, H. X., Xu, J. X., and Qian, S. X. (2009). Effects of thermal treatment on the third-order optical nonlinearity and ultrafast dynamics of Ag nanoparticles embedded in silicate glasses. Chem. Phys. Lett. 475 (4–6), 256–259. doi:10.1016/j.cplett.2009.05.055
Yang, X., Yang, Y., Fu, L., Zou, M., Li, Z., Cao, A., et al. (2018). An ultrathin flexible 2D membrane based on single-walled nanotube–MoS2 hybrid film for high-performance solar steam generation. Adv. Funct. Mat. 28 (3), 1704505–1704509. doi:10.1002/adfm.201704505
Yang, Y., Zhao, H., Yin, Z., Zhao, J., Yin, X., Li, N., et al. (2018). A general salt-resistant hydrophilic/hydrophobic nanoporous double layer design for efficient and stable solar water evaporation distillation. Mat. Horiz. 5 (6), 1143–1150. doi:10.1039/c8mh00386f
Yao, J., Zheng, Z., and Yang, G. (2018). Layered tin monoselenide as advanced photothermal conversion materials for efficient solar energy-driven water evaporation. Nanoscale 10 (6), 2876–2886. doi:10.1039/c7nr09229f
Ye, E., and Li, Z. (2022). Photothermal nanomaterials. The Royal Society of Chemistry (Nanoscience & Nanotechnology Series. doi:10.1039/9781839165177
Ye, M., Jia, J., Wu, Z., Qian, C., Chen, R., O'Brien, P. G., et al. (2016). Synthesis of black TiOxNanoparticles by Mg reduction of TiO2Nanocrystals and their application for solar water evaporation. Adv. Energy Mat. 7, 1601811. doi:10.1002/aenm.201601811
Ying, P., Li, M., Yu, F., Geng, Y., Zhang, L., He, J., et al. (2020). Band gap engineering in an efficient solar-driven interfacial evaporation system. ACS Appl. Mat. Interfaces 12 (29), 32880–32887. doi:10.1021/acsami.0c09965
Yuan, B., Meng, L., Zhang, C., Yang, L., Bai, L., Yang, H., et al. (2021). Enhancement of pollutant degradation and solar-driven water evaporation by architecting hierarchical 1D/2D TiO2 @ MoS2 core–shell networks. Appl. Surf. Sci. 570, 151143. doi:10.1016/j.apsusc.2021.151143
Zada, I., Zhang, W., Sun, P., Imtiaz, M., Iqbal, N., Ghani, U., et al. (2020). Superior photothermal black TiO2 with random size distribution as flexible film for efficient solar steam generation. Appl. Mater. Today 20, 100669. doi:10.1016/j.apmt.2020.100669
Zechang, W., Wang, J., Guo, S., and Tan, S. C. (2022). Towards highly salt-rejecting solar interfacial evaporation: Photothermal materials selection, structural designs, and energy management. Nano Res. Energy 1, e9120014. doi:10.26599/NRE.2022.9120014
Zhang, L., Tang, B., Wu, J., Li, R., and Wang, P. (2015). Hydrophobic light-to-heat conversion membranes with self-healing ability for interfacial solar heating. Adv. Mat. 27 (33), 4889–4894. doi:10.1002/adma.201502362
Zhao, D., Duan, H., Yu, S., Zhang, Y., He, J., Quan, X., et al. (2015). Enhancing localized evaporation through separated light absorbing centers and scattering centers. Sci. Rep. 5, 17276. doi:10.1038/srep17276
Zheng, P., Tang, J., Zhou, Z., Gong, L., Yang, H., Jia, X., et al. (2020). Ultrafast synthesis of defective black TiO2 via one-step NaN3 deflagration for high-efficiency solar water evaporation. Surfaces Interfaces 22, 100901. doi:10.1016/j.surfin.2020.100901
Zhou, L., Tan, Y., Ji, D., Zhu, B., Zhang, P., Xu, J., et al. (2016). Self-assembly of highly efficient, broadband plasmonic absorbers for solar steam generation. Sci. Adv. 2 (4), e1501227. doi:10.1126/sciadv.1501227
Zhu, G., Xu, J., Zhao, W., and Huang, F. (2016). Constructing black titania with unique nanocage structure for solar desalination. ACS Appl. Mater. Interfaces, 8 (46), 31716–31721. doi:10.1021/acsami.6b11466
Zhu, L., Gao, M., Peh, C. K. N., and Ho, G. W. (2018). Solar-driven photothermal nanostructured materials designs and prerequisites for evaporation and catalysis applications. Mat. Horiz. 5 (3), 323–343. doi:10.1039/c7mh01064h
Keywords: photothermal material, solar water interfacial evaporation, TiO2, water purification, renewable energy sources
Citation: Srishti , Khandelwal K, Kumar A and Sinhamahapatra A (2022) Progress on TiO2-based materials for solar water interfacial evaporation. Front. Chem. Eng. 4:1046019. doi: 10.3389/fceng.2022.1046019
Received: 16 September 2022; Accepted: 28 October 2022;
Published: 18 November 2022.
Edited by:
Mikhail Zheludkevich, Helmholtz Centre for Materials and Coastal Research (HZG), GermanyReviewed by:
Rastko Vasilic, University of Belgrade, SerbiaFernando Graniero Echeverrigaray, State University of Campinas, Brazil
Copyright © 2022 Srishti, Khandelwal, Kumar and Sinhamahapatra. This is an open-access article distributed under the terms of the Creative Commons Attribution License (CC BY). The use, distribution or reproduction in other forums is permitted, provided the original author(s) and the copyright owner(s) are credited and that the original publication in this journal is cited, in accordance with accepted academic practice. No use, distribution or reproduction is permitted which does not comply with these terms.
*Correspondence: Apurba Sinhamahapatra, apurba@iitism.ac.in
†These authors have contributed equally to this work and share first authorship