- Faculty of Science, School of Life and Environmental Sciences, Sydney Institute of Agriculture, The University of Sydney, Sydney, NSW, Australia
There are hundreds of species of Agaves found globally in natural and anthropogenic systems. Agaves are used to produce fibres, alcoholic beverages like tequila, and in biofuel production. The objectives of this study were to assess the research available into Agave planting density and to use PRISMA (Preferred Reporting Items for Systematic Reviews and Meta-Analyses) to suggest an optimum planting density for the highest dry aboveground productivity. Background research into Agave planting densities found little data on the effect of planting density on biomass production, with most studies focusing on other independent variables affecting productivity. There were 13 data points included in the analysis. The meta-analysis suggested that the optimal planting density of Agave is approximately 2,600 plants ha−1, which provides optimal dry aboveground biomass of 28.8 Mg ha−1 yr−1. These findings provide a framework for further experimentation in Australian conditions using a Nelder design density experiment to ground-truth the meta-analysis.
Introduction
Agaves can be found in many arid and semi-arid regions worldwide as part of both anthropogenic and natural landscapes. The Agavaceae family is a culturally, environmentally, and economically significant family, with a geographic centre of origin in Mexico. This monocot has spread through semi-arid to arid regions from Central America to South America (Good-Avila et al., 2006). Agaves are a resilient species with the ability to survive in dry landscapes and low-quality soils. Their ability to survive in adverse climates has resulted in Agave being used for beverages, food, fibre, shelter, ornamentals, and soil stabilisation (Garcia-Moya et al., 2011).
Agaves are most notably known globally for their use in the popular alcoholic beverage tequila. Tequila is made exclusively using Agave tequilana Weber cv. azul and must also be produced in the Mexican state, Jalisco, and some surrounding areas. A. tequilana is protected by a geographic indicator (GI). If Agave is produced from a species other than A. tequilana or outside Jalisco’s growing regions, the spirit is called mezcal (Bowen, 2015; Subedi, 2017). Interest in Agave for use as a bioenergy has increased in recent years because of its predicted resilience to impacts of climate change (Yan et al., 2020). The predicted impacts of climate change are 62% lower for Agave than corn and 30% lower than sugarcane, which are two other primary bioenergy feedstocks. The ability for Agave to survive in marginal semi-arid landscapes reduces the impact on other agricultural production systems. This will become increasingly important as agricultural production is under pressure with a changing climate and a higher demand for agricultural commodities with an increasing population. The resilience of Agave species in arid and semi-arid landscapes also provides an opportunity to sequester carbon on land unfit for agricultural production (Kant, 2010).
The Agavaceae family is relatively young, 20–26 million years old, and has more than 200 recognised species (García-Mendoza, 2002; Good-Avila et al., 2006). Agaves take between 6 and 12 years to mature and can grow from a few centimetres to over 4 m depending on the species. (Gentry, 2004; Sandoval et al., 2012). Agave plants can produce aboveground dry weight biomass between 10 and 40 Mg ha−1 yr−1, with A. salmiana and A. mapisaga being the most productive species (Nobel et al., 1992; Li et al., 2012). Agaves can reproduce sexually, with seeds, and asexually through aerial bulbils and basal shoots and rhizomes, but mainly reproduce asexually as they only flower once a lifetime when fully mature (Arizaga and Ezcurra, 2002). When fully mature Agave produce a flower stalk, technically called the poll, which can be 4.5–12 m in height (Smith, 1929).
Agave americana was the first plant to be shown to undergo Crassulacean acid metabolism (CAM) photosynthesis (Garcia-Moya et al., 2011). CAM photosynthesis is a photosynthetic pathway that allows plants to regulate their use of water and carbon dioxide. CAM photosynthesis is abundant in nature, with an estimated 20,000 species found terrestrially and aquatically and in 7% of vascular plants (Andrade et al., 2007; Osmond et al., 2008; Ruiz and Estrella-Ruiz, 2008). CAM plants take in CO2 at night and then sequester it during the following day cycle (Winter and Smith, 1996). By splitting the process into day and night cycles, CAM photosynthesis allows stomata to be tightly closed during the heat of the day and significantly reduces water loss compared to other photosynthetic pathways. Plants have control over their gas exchange through their stomata during CAM rhythms, allowing carbon sequestration into malate overnight. The benefit of CAM photosynthesis is that plants can be highly productive under heat and water stress.
Competition can be divided into intraspecific and interspecific competition, with intraspecific competition having the most significant form of competition because of shared resources. Intraspecific competition affects communities and can result in asymmetric growth between individuals or symmetrical growth negatively impacting the community. When competition is asymmetric, an individual removes a disproportionate amount of resource and is larger than other individuals. In symmetric competition all individuals are equally affected. In a study on tree plantations by Yang et al. (2019), found that competition pressure of neighbouring trees was crucial in determining tree growth and biomass partitioning. According to Chu et al. (2008), the relationship between body size and density is key to ecology. The understanding of how planting density affects yield has changed over time. The ‘Law Of Constant Final Yield’ explains that the relationship between density and yield within a single species increases proportionally and then levels off and remains constant but this law is not always satisfied, as there can be exceptions when higher densities result in a decline in yield (see Figure 1). (Farazdaghi and Harris, 1968). Chu et al. (2008) reported that the greatest biomass was found at intermediate densities because of resource competition. There have been no previous field experiments on the effect of planting density on Agave yield. The significance of the analysis undertaken in this study is that it provides a baseline for future research.
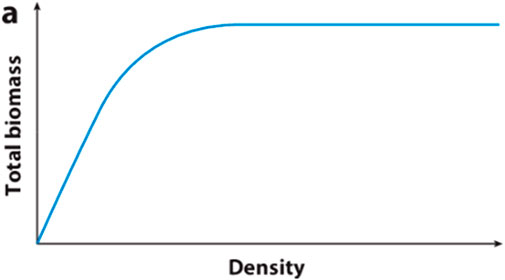
FIGURE 1. Graph showing the law of constant final yield (Weiner and Freckleton, 2010).
The focus of previous studies has generally been on the net CO2 uptake of CAM plants, resulting in limited background knowledge on planting density. The significance of this research is that this is the first systematic literature review on the effect of planting density on dry aboveground biomass of Agave. The only previous study investigating the relationship between planting density and biomass in Agave species was undertaken by Nobel (1988). This study used a model based on ray-tracing techniques to determine what leaf area index (LAI) led to the highest productivity. This systematic literature review takes a different approach by collating previous meta-data from all available literature to suggest an optimum planting density range for future research. The model estimated the highest productivity for A. tequilana and A. fourcroydes to be between an LAI of 4–8 or a density of between 2,500 and 3,400 plants per hectare. After reaching a peak at this density, the productivity flattened out and remained the same for higher planting densities, which is consistent with the “Law Of Constant Final Yield” but not in line with the results of Chu et al. (2008), who suggested a yield decrease after an optimum planting density. The estimated productivity at densities of 2,500–3,400 plants ha−1 is slightly above 20 Mg ha−1 yr−1 for A. tequilana and slightly below 20 Mg ha−1 yr−1 for A. fourcroydes. Nobel (1988) suggested that the productivity could be as high as 44 Mg ha−1 yr−1 for A. tequilana if the water index was increased through greater rainfall or introducing irrigation. Further background research into Agave planting densities revealed little research into the effect of planting density on productivity, other than Nobel (1988), which gave predictive insight into the topic but had no follow-up ground-truthing. Smith (1929) found that the planting density of Agave was dependent on abiotic factors like soil fertility and advocated for higher planting densities in poorer soil quality as plants will not grow as large. Planting densities can range from 1,500 plants ha−1 in good soils to 3,500 plants ha−1 in poorer soils, although these densities may have been recommended for greater row access when harvesting sisal fibre (Smith, 1929).
To find the optimum planting density, this study aims to assess the available research on Agave species, which report planting densities and yield and then recommend an optimum planting density for greatest productivity from available research using meta-analysis. The relationship is hypothesised to be quadratic with an optimum planting density for productivity. Based on the findings of Nobel (1988), the hypothesised optimum density is 2,500–3,400 plants per hectare for Agave, which will equate to aboveground dry biomass of approximately 21 Mg ha−1 yr−1.
Methodology
The methodology followed for this systematic literature review was PRISMA (Preferred Reporting Items for Systematic Reviews and Meta-Analyses). The process is visualised in Figure 2. Background research on the topic was conducted ’by reviewing peer reviewed literature. This is the first systematic literature review that examines the relationship between crop planting density and dry aboveground productivity. The advantage of conducting a systematic review is that it provides baseline data for future research into planting density and yield. Furthermore, outcomes such as optimal planting density may be adopted by the Agave industry. From this background research, it was discovered that there were few studies that recorded both density and productivity as aboveground biomass. To build a table of Agave planting densities and aboveground biomass, all available data was collated. Initially, there was insufficient evidence for agave density trials, so other commonly cultivated CAM plants were included (Table 1). These other CAM plants were Opuntia ficus-indica (prickly pear), Aloe, and Ananas comosus (pineapple). Journal articles that did not have sufficient evidence were not included in Figure 1. It was uncertain initially if productivity would be tonnes per hectare per year or tonnes per hectare, so both were left in the table.
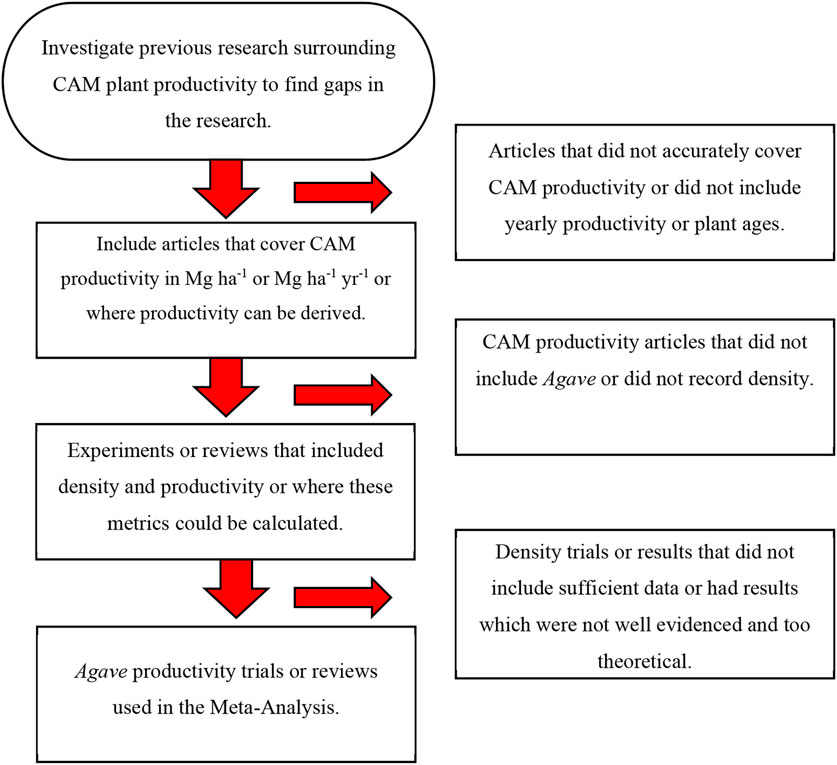
FIGURE 2. Flowchart showing the research process, sorting through relevant articles to find relevant and reliable sources.
The CAM articles of species other than Agave were later removed from the meta-analysis, as sufficient evidence was found in Agave research articles and the results were determined to not be relevant to the study. Productivity per hectare per year was the chosen productivity measurement, so data that could not be transformed into a yearly productivity measurement was removed. Articles that did not record density were also removed.
The data was then evaluated on reliability, as some of the data was theoretical and provided yields that were not physically possible in the field. For example, Nobel et al. (1992) had a yield of 40 Mg ha−1 yr−1 because the experiment only had a few plants in a plot, and they could grow much larger than in scaled production. There were three groups of 12–15 plants in this experiment, meaning the density of 2076.92 plants ha−1 was not representative of the small-scale experiment. Some smaller Agaves were also removed, such as A. deserti and A. cantala, as they skewed the data too much because of their significantly lower yields. Only one data point was included from Smith (1929) because two of the points were expected yields, instead of actual yields, and very different at the same density. Another paper that was not included was Davis et al. (2017), which had A. americana aboveground dry biomass yields ranging from 2.0 to 9.3 Mg ha−1 yr−1 at densities of 2,500 plants ha−1. The yields for the experiment were low because of infestation by the Agave snout weevil (Scyphophorus acupunctatus), which is a major threat to Agave crops in America. This pest has increased survivability in irrigated crops. The data points that were included in the analysis are displayed in Table 2 with their reference, density, and productivity. Table 3 provides more in-depth background for each region with climatic and environmental conditions.
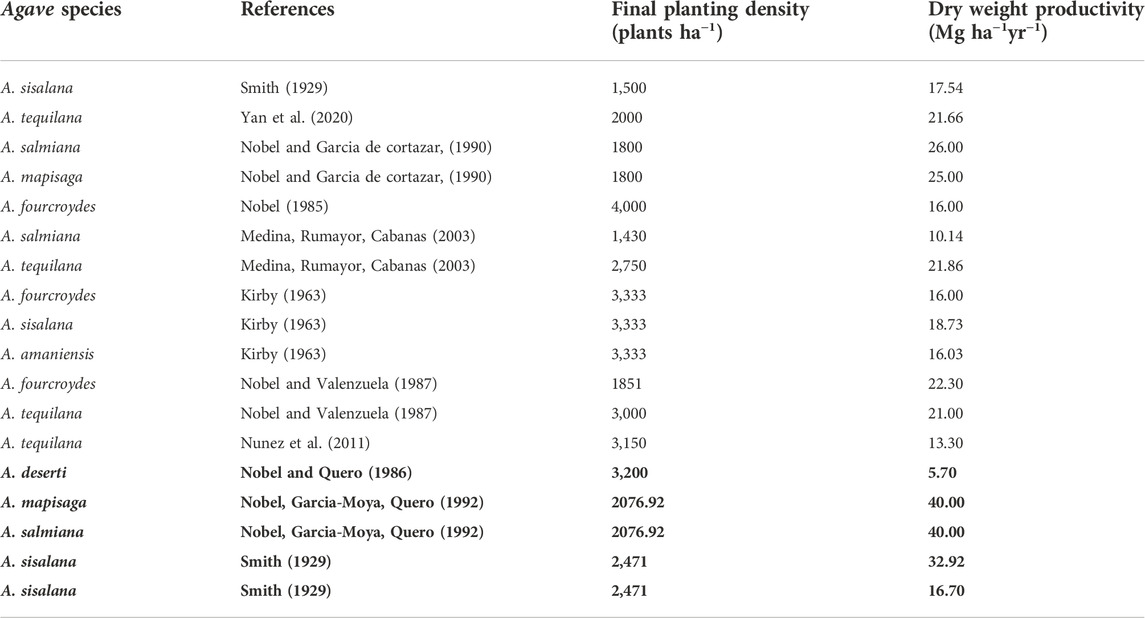
TABLE 2. Dataset used in the meta-analysis. The results in bold were not used in the final meta-analysis. The table is in order of planting density.
A study by Nunez et al. (2011), referenced multiple Agave species but gave productivity as “mescal feedstock” instead of individually. The only species referenced individually was Agave tequilana, from which productivity was derived from tables in the study on cost of planting. Productivity was not directly related to the density in the study. The planting density was 3,150 plants ha−1 and had a productivity of 13.3 Mg ha−1 yr−1.
Data from Kirby (1963) and Smith (1929) needed transformation, as they were measurements of leaf fibre and not aboveground dry biomass, as well as being recorded in pounds (lbs). Using a harvest index from Nobel (1988), a coefficient was calculated to be 6.12, the conversion factor for fibre from total aboveground dry biomass. The data from the two studies also needed to be converted from imperial measurements to metric. Yan et al. (2020) recorded aboveground fresh biomass. To convert the recorded fresh weight from Yan et al. (2020), an assumption of 85% of fresh weight was used to convert to dry weight (Li et al., 2012; Corbin et al., 2015). The referenced fresh weight of the A. tequilana was 144 Mg ha−1 yr−1 and this was converted to 22 Mg ha−1 yr−1 dry weight. Other data required no modification because tonnes per hectare was calculated by total productivity in Mg ha−1 divided by plant age to find Mg ha−1 yr−1. Table 1 shows all the included data points in the literature review and Table 2 shows the data points included in the meta-analysis.
Data analysis was undertaken using the nlme package in R (Pinheiro J et al., 2021). A linear mixed model was fitted to the data (Table 1) with planting density as a fixed effect and the study ID (Researcher) and Agave species (CAM_Plant) included as random effects to account for variation in genetic, management, and environmental difference between the studies and species. The relationship between yield and density was modelled as a second order polynomial. Both linear and quadratic models were fitted to the data and the models were compared using a likelihood ratio test and the Akaike Information Criteria (AIC). Approximate variance explained by the model terms were estimated using the MuMIn package in R (Bartoń, 2022), model residuals were checked using a Q-Q plot and standardised residual plots and were determined to follow a reasonably homogenous normal distribution.
Results
Table 1 includes the final dataset collated from the meta-analysis, including the data from Nobel and Quero (1986), which was excluded because of the small sample size and Nobel et al. (1992), which was excluded because A. deserti is significantly smaller than other species of Agave. The excluded data are highlighted in Table 2.
Table 4 shows the four A. tequilana and A. fourcroydes that achieved a productivity equal to or greater than 21 Mg ha−1 yr−1. The densities of these Agaves ranged from 1851 plants ha−1 to 3,000 plants ha−1.

TABLE 4. Data points that achieved a productivity greater than or equal to 21 Mg ha−1 yr−1, as Nobel (1988) predicted would be the maximum for A. tequilana and A. fourcroydes. A. salmiana and A. mapisaga data were excluded because they were not included in the Nobel’s ray-tracing model.
The second order polynomial model was highly significant (X2 = 33.890, df = 2, p = 4.373 × 10−8) (Figure 3). The model residuals were reasonably normally distributed (Figure 4). The AIC was lower in the quadratic model compared to the linear model (quadratic model 13.40 and linear model 31.66) indicating that the quadratic model is the better model. The estimated optimal planting density is 2,600 plants/ha with a predicted yield of 28.8 Mg ha−1 yr−1. The planting density explained approximately 48.7% of the variation in the dataset and the two random effects, CAM species and Study ID, together explained 50.9% of the variation in the model.
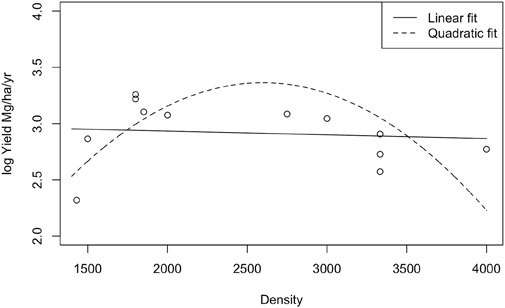
FIGURE 3. Fitted linear and quadratic models. The planting density was a significant predictor of yield in the quadratic model (X2 = 33.890, df = 2, p = 4.373 × 10−8).
Discussion
Nobel (1988) developed a model based on ray-tracing techniques to calculate what leaf area index (LAI) resulted in the highest productivity. The model was used to estimate the productivity of Agaves at different positions in a canopy. This model suggested an optimum planting density between 2,500 and 3,400 plants ha−1 for A. tequilana and A. fourcroydes to achieve a productivity of approximately 21 Mg ha−1 yr−1. The meta-analysis from this study predicted an optimal density of 2,600 plants ha−1 that produced a dry aboveground biomass of 28.8 Mg ha−1 yr−1. Therefore, this meta-analysis provides evidence for the optimum Agave density speculated by Nobel (1988) as the density falls within the range of the model. The predicted productivity in this meta-analysis is higher, although Nobel (1988) also stated that with proper irrigation and watering, the productivity of A. tequilana could be as high as 44 Mg ha−1 yr−1, which is nearly the productivity of sugarcane. A. tequilana, A. fourcroydes, A. salmiana, and A. mapisaga were the species that achieved maximum productivity over 21 Mg ha−1 yr−1, the predicted maximum by Nobel (1988). Table 4 excludes A. salmiana and A. mapisaga, which had productivities of 26 Mg ha−1 yr−1 and 25 Mg ha−1 yr−1, respectively. Out of the four points in Table 4 that have a productivity greater than or equal to 21 Mg ha−1 yr−1, only two are within the density range (2,500–3,400) Nobel (1988) calculated. Therefore, further experimentation is needed to see why A. tequilana and A. fourcroydes crops were outside of the modelled optimum density achieved the predicted 21 Mg ha−1 yr−1.
The “Law Of Constant Final Yield”, suggested that yield increases proportionally with increasing density and then levels off and remains constant. Farazdaghi and Harris (1968) found that the law may not always be satisfied and that at higher densities, yield may decline. The meta-analysis in this systematic literature review supports the idea that yield decreases above the optimum density, and the graph showed a decrease in yield at higher densities. The decline found in this meta-analysis differs from the results of Nobel (1988) and the “Law of Constant Final Yield” in which biomass increases to a flat asymptote. However, more data is needed at density extremes to sufficiently determine if the data fits to confirm the shape of the curve. A reason for yield reducing at higher densities may be because of resource competition, such as light, nutrients and water. For example, reduced competition between plants may be why the smaller planting trial by Nobel et al. (1992) with three groups of 12–15 plants ha−1 achieved a productivity of 40 Mg ha−1 yr−1. Chu et al. (2008) found that the greatest biomass was found at intermediate planting densities and that as abiotic stress increased, the yield-biomass relationship shifted from linear to humped.
The importance for studying a species such as Agave is that with predicted climate impacts, Agave is suggested to be more resilient to these impacts (Yan et al., 2011), enabling future production of bioenergy. Agave also has a less significant impact on arable land needed for agricultural production, as it can survive in marginal, semi-arid landscapes. Given as much of 40% of land on Earth is arid and semi-arid, Agave production provides a unique opportunity to sequester carbon on land unfit for agricultural production (Garcia-Moya et al., 2011). Understanding how to efficiently produce Agave in the future is crucial for this reason.
Limitations
The meta-analysis undertaken was limited by the type of research used in the analysis. Many studies were not researching density as the independent variable but were testing other variables, such as soil type or acidity (Nobel, 1985). Some of the biomass estimates used in the analysis were from papers studying wild Agave and not cultivated species. The number of plants in these was influenced by external factors, such as the sale of young plants, forage, and removal for production of alcoholic beverages (Martínez-Salvador et al., 2005). The power of the meta-analysis was limited because of the small sample size of available data.
Different species of Agave sp. have a range of sizes, as mature plants can range from a few centimetres to 4 m depending on the species (Davis et al., 2011). A. deserti was excluded because it was smaller than the other species as it produced 5.7 Mg ha−1 yr−1, while the next lowest producer was 10 Mg ha−1 yr−1. Nobel (1988) found that smaller Agave species, like A. sisalana, can be planted at much higher densities, up to 10,000 plants ha−1, although optimum densities are between 4,000 and 5,000 plants ha−1. Kirby (1963) also noted high planting densities with approximately 4,000 plants per hectare, providing satisfactory results in Tanganyika, present-day Tanzania, suggesting densities of 10,000 plants ha−1 on productive land. Therefore, further investigation is required to determine the size planting density interaction.
Different species also reached maturity at different ages; for example, A. fourcroydes takes approximately ten years to mature, and A. tequilana and A. sisalana take approximately seven years to mature (Nobel, 1988). The use of Mg ha−1 yr−1 limited the reliability of the results in the meta-analysis as some plants grew slower than others, but this unit of measurement was necessary as not all plant ages were recorded, limiting the use of Mg ha−1. The analysis is limited by the research focus area of the referenced data points. There is no literature with a specific field experiment that focuses on the planting density and productivity.
Future research—An Australian perspective and case study
The meta-analysis highlights opportunity for further experimentation opportunities to ground truth the model through further field experiments. There have been no previous systematic literature reviews exploring the relationship between density and aboveground biomass for a crop. This study also provides further evidence for the ‘Law of Constant Final Yield’ and resource constraints at higher densities. The use of multiple Agave species and varying cultivation techniques limited the accuracy of the meta-analysis. However, as more data on multiple species becomes available, it will be possible to determine whether a general model can be applied to all species of Agave sp.
In an Australian context, Agave tequilana would be used in a field experiment because it is the best-studied species of Agave in Australia (Subedi, 2017). Densities of previous studies range from 1,430 to 7,400 plants per hectare would be planted, and it would then be possible to confirm whether optimum planting densities agree with Nobel (1988) calculated using ray-tracing models (2,500 and 3,400 plants ha−1) and the results from this meta-analysis which suggests that the best planting density for Agave is 2,600 plants ha−1.
Jalisco, Mexico, is the only place tequila can be produced. The monthly mean daily air temperature extremes measured by Pimienta-Barrios et al. (2001), was 9–22°C at night and 33–41°C during the day. Holtum et al. (2011) and Holtum and Chambers (2010), suggested that the climatic conditions and soil types within the Atherton Tablelands, Mareeba, Mackay, Ayr, Rockhampton, and Childers districts are similar to those where A. tequilana is grown commercially in Mexico and would be potential growing regions for Agave. Agave cultivation was trialled in Ayr, Queensland, where there was a trial site of A. tequilana. Agave is also grown in Narrabri, New South Wales, by the Black Snake Distillery, where a crop of A. americana is grown for the production of Agave spirit. The suggested locations for field trials are shown below in Figure 5. Another important consideration for Agave biofuel production is the proximity to processing facilities, such as sugarcane mills. The sugarcane mills are found close to the coast of Queensland, where there are established sugarcane production regions. Without the construction of a processing facility further inland, Agave production would be limited to the coastal regions or higher transport costs would be required. Sugar mills are only required for processing Agave as a biofuel and are not required in the production of beverages such as mezcal or Australian agave spirit.
Research into the potential to produce Agave in Australia is limited and often hypothetical. Subedi (2017) claimed that farmers responded positively to the potential production of A. tequilana in Australia but would want to see the results of the field experiment in Ayr, Queensland, before they include the plant in their crop rotation. A. tequilana has been considered for production on marginal and unproductive land on farms. The research conducted by Yan et al. (2020) was on the 3,500 plant Agave crop planted by Mr Don Chambers in 2009 in Ayr, Queensland. The initial density planted in 2009 was 4,000 plants ha−1 but had to be thinned to 2000 plants ha−1 suggesting that the initial crop was planted too densely.
Most planting density trials are rectangular plots with different spacings between rows where the rows are then compared to find the optimal planting density. The Nelder design is a planting density method that essentially enables multiple density trials in a small area. The benefit of a Nelder planting design for ground-truthing the results of this meta-analysis is that it is more cost-effective compared to other planting designs. Figure 6 shows a Nelder planting design for Eucalyptus dunni in Brazil by Stape and Binkley (2010). This research investigated planting densities from 210 plants ha−1 to 4,400 plants ha−1 on 0.6 ha of land. Compared to a block design, which was only able to test 1,111 plants ha−1 to 2,222 plants ha−1, the Nelder design was much more efficient for testing various planting densities than traditional planting methods. To grow an Agave crop, the same planting density could be used to test from 1,000 plants ha−1 to 8,000 plants ha−1 to find the optimal planting density for A. tequilana in Australia. The data from this proposed field experiment would conclusively determine the best planting density for Australian A. tequilana crops. The study is limited by the time taken for A. tequilana to mature, which takes approximately 5–7 years, although optimum density would be apparent before then.
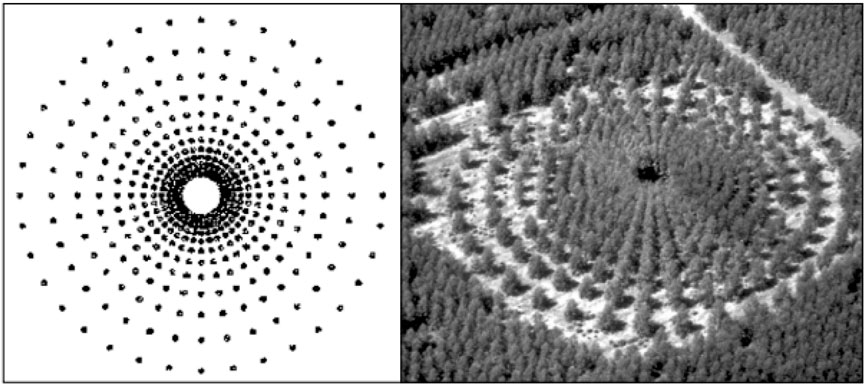
FIGURE 6. Diagramatic representation and photograph of a Nelder planting design with 36 rays and 12 arcs.
In conjunction with the data from the experiment in Ayr, Queensland, the proposed trial will provide farmers on marginal land with confidence to trial A. tequilana on their land to produce maximal biomass. This proposed research will help Australian producers determine an optimum planting density for Agave and provide other benefits such as reduced desertification and increased carbon sequestration.
Conclusion
Agave are an increasingly researched species because of their climate resilience and opportunities in bioenergy production. The meta-analysis in this global systematic literature review revealed an optimum planting density of 2,600 plants ha−1 with a dry aboveground biomass yield of approximately 28.8 Mg ha−1 yr−1. The optimal density is at the lower limit of estimates of previous models of density between 2,500 and 3,400 plants ha−1. Currently the estimates of planting densities are based on a small number of data points. However, the results of the meta-analysis provide a baseline for future research on optimising planting densities of Agave. For example, it is recommended that a Nelder design is used to determine the optimal planting density of A. tequilana to determine the optimal planting density in semi-arid regions in Australia and other potential growing regions across the globe to better inform potential growers.
Data availability statement
The original contributions presented in the study are included in the article/Supplementary Material, further inquiries can be directed to the corresponding author.
Author contributions
WC was the primary author of the manuscript. DT was the supervising author and contributed to the editing. FV assisted with editing and statistics.
Conflict of interest
The authors declare that the research was conducted in the absence of any commercial or financial relationships that could be construed as a potential conflict of interest.
Publisher’s note
All claims expressed in this article are solely those of the authors and do not necessarily represent those of their affiliated organizations, or those of the publisher, the editors and the reviewers. Any product that may be evaluated in this article, or claim that may be made by its manufacturer, is not guaranteed or endorsed by the publisher.
References
Andrade, J. L., De la Barrera, E., García, C. R., Ricalde, M. F., Soto, G. V., and Cervera, J. C. (2007). El metabolismo ácido de las crasuláceas: Diversidad, fisiología ambiental y productividad. Bot. Sci. 81, 37–50. doi:10.17129/botsci.1764
Arizaga, S., and Ezcurra, E. (2002). Propagation mechanisms in Agave macroacantha (Agavaceae), a tropical arid-land succulent rosette. Am. J. Bot. 89 (4), 632–641. doi:10.3732/ajb.89.4.632
Bowen, S. (2015). “Divided spirits,” in Divided spirits (United States: University of California Press).
Chu, C. J., Maestre, F. T., Xiao, S., Weiner, J., Wang, Y. S., Duan, Z. H., et al. (2008). Balance between facilitation and resource competition determines biomass–density relationships in plant populations. Ecol. Lett. 11 (11), 1189–1197. doi:10.1111/j.1461-0248.2008.01228.x
Corbin, K. R., Byrt, C. S., Bauer, S., DeBolt, S., Chambers, D., Holtum, J. A., et al. (2015). Prospecting for energy-rich renewable raw materials: Agave leaf case study. PLoS One 10 (8), e0135382. doi:10.1371/journal.pone.0135382
Davis, S. C., Dohleman, F. G., and Long, S. P. (2011). The global potential for Agave as a biofuel feedstock. Gcb Bioenergy 3 (1), 68–78. doi:10.1111/j.1757-1707.2010.01077.x
Davis, S. C., Kuzmick, E. R., Niechayev, N., and Hunsaker, D. J. (2017). Productivity and water use efficiency of Agave americana in the first field trial as bioenergy feedstock on arid lands. Gcb Bioenergy 9 (2), 314–325. doi:10.1111/gcbb.12324
de Jesús Escalona-Alcázar, F., Escobedo-Arellano, B., Castillo-Félix, B., García-Sandoval, P., Gurrola-Menchaca, L. L., Carrillo-Castillo, C., et al. (2012). “A geologic and geomorphologic analysis of the Zacatecas and Guadalupe quadrangles in order to define hazardous zones associated with the erosion processes,” in Sustainable development-authoritative and leading edge content for environmental management (kalamazoo: western michigan university).
Estrada-Medina, H., Bautista, F., Jiménez-Osornio, J. J. M., González-Iturbe, J. A., and Aguilar Cordero, W. d. J. (2013). Maya and WRB soil classification in yucatan, Mexico: Differences and similarities. Int. Sch. Res. Notices 2013, 634260. doi:10.1155/2013/634260
Farazdaghi, H., and Harris, P. (1968). Plant competition and crop yield. Nature 217 (5125), 289–290. doi:10.1038/217289a0
Garcia-Moya, E., Romero-Manzanares, A., and Nobel, P. (2011). Highlights for agave productivity. Gcb Bioenergy 3 (1), 4–14. doi:10.1111/j.1757-1707.2010.01078.x
García-Mendoza, A. (2002). Distribution of agave (Agavaceae) in méxico. Cactus Succul. J. 74 (4), 177–187.
García-Moya, E., and Nobel, P. S. (1990). Leaf unfolding rates and responses to cuticle damaging for pulque agaves in Mexico, Colegio de Postgraduados, Mexico
Gentry, H. S. (2004). Agaves of continental north America. United States: University of Arizona Press.
Good-Avila, S. V., Souza, V., Gaut, B. S., and Eguiarte, L. E. (2006). Timing and rate of speciation in agave (Agavaceae). Proc. Natl. Acad. Sci. U. S. A. 103 (24), 9124–9129. doi:10.1073/pnas.0603312103
Holtum, J. A., Chambers, D., Morgan, T., and Tan, D. K. (2011). Agave as a biofuel feedstock in Australia. Gcb Bioenergy 3 (1), 58–67. doi:10.1111/j.1757-1707.2010.01083.x
Holtum, J., and Chambers, D. (2010). Feasibility of Agave as a feedstock for biofuel production in Australia. ACT Canberra: Rural Industry Research and Development Corporation.
Juárez-Camarena, M., Auvinet-Guichard, G., and Méndez-Sánchez, E. (2016). Geotechnical zoning of Mexico Valley subsoil. Ing. Investig. Tecnol. 17 (3), 297–308. doi:10.1016/j.riit.2016.07.001
Kant, P. (2010). “Could Agave be the species of choice for climate change mitigation,” in Working paper IGREC-11 (New Delhi: Institute of Green Economy).
Li, H., Foston, M. B., Kumar, R., Samuel, R., Gao, X., Hu, F., et al. (2012). Chemical composition and characterization of cellulose for Agave as a fast-growing, drought-tolerant biofuels feedstock. RSC Adv. 2 (11), 4951–4958. doi:10.1039/c2ra20557b
Martínez-Salvador, M., Valdez-Cepeda, R., Arias, H., Beltrán-Morales, L., Murillo-Amador, B., Troyo-Diéguez, E., et al. (2005). Distribution and density of maguey plants in the arid Zacatecas Plateau, Mexico. J. Arid Environ. 61 (4), 525–534. doi:10.1016/j.jaridenv.2004.10.002
Medina, G., Rumayor, R., Cabañas, C., Luna, F., Ruiz, C., Gallegos, V., et al. (2003). Potencial productivo de especies agrícolas en el estado de Zacatecas. Calera, Zacatecas, México: Instituto Nacional de Investigaciones Forestales, Agrícolas y Pecuarias, Centro de Investigación Regional Norte Centro campo experimental zacatecas.
Nobel, P., García-Moya, E., and Quero, E. (1992). High annual productivity of certain agaves and cacti under cultivation. Plant Cell Environ. 15 (3), 329–335. doi:10.1111/j.1365-3040.1992.tb00981.x
Nobel, P. S. (1988). Environmental biology of agaves and cacti. United Kingdom: Cambrdige University Press.
Nobel, P. S., and Garcia de Cortazar, V. G. (1987). Interception of photosynthetically active radiation and predicted productivity for agave rosettes. Photosynthetica 21 (3), 261–272.
Nobel, P. S. (1985). PAR, water, and temperature limitations on the productivity of cultivated Agave fourcroydes (henequen). J. Appl. Ecol. 22, 157–173. doi:10.2307/2403334
Nobel, P. S. (1984). Productivity of agave deserti: Measurement by dry weight and monthly prediction using physiological responses to environmental parameters. Oecologia 64 (1), 1–7. doi:10.1007/bf00377535
Nobel, P. S., and Quero, E. (1986). Environmental productivity indices for a Chihuahuan desert CAM plant, Agave lechuguilla. Ecology 67 (1), 1–11. doi:10.2307/1938497
Nobel, P. S., and Valenzuela, A. G. (1987). Environmental responses and productivity of the CAM plant, Agave tequilana. Agric. For. Meteorology 39 (4), 319–334. doi:10.1016/0168-1923(87)90024-4
Nunez, H. M., Rodriguez, L. F., and Khanna, M. (2011). Agave for tequila and biofuels: An economic assessment and potential opportunities. Gcb Bioenergy 3 (1), 43–57. doi:10.1111/j.1757-1707.2010.01084.x
Osmond, B., Neales, T., and Stange, G. (2008). Curiosity and context revisited: Crassulacean acid metabolism in the anthropocene. J. Exp. Bot. 59 (7), 1489–1502. doi:10.1093/jxb/ern052
Pimienta-Barrios, E., Robles-Murguia, C., and Nobel, P. S. (2001). Net CO2Uptake for agave tequilana in a warm and a temperate environment 1. Biotropica 33 (2), 312–318. doi:10.1111/j.1744-7429.2001.tb00181.x
Pinheiro, J., Bates, D., DebRoy, S., and D, S. (2021). Linear and nonlinear mixed effects models_. R. package 3, 1–153. Core team.
Ruiz, E. B., and Estrella-Ruiz, J. P. (2008). Manejo sustentable de los recursos naturales guiado por proyectos científicos en la mixteca poblana mexicana. Ecosistemas 17 (2), 3–9.
Sandoval, S. d. C. D., Juárez, M. J. A., and Simpson, J. (2012). Agave tequilana MADS genes show novel expression patterns in meristems, developing bulbils and floral organs. Sex. Plant Reprod. 25 (1), 11–26. doi:10.1007/s00497-011-0176-x
Silva, H., Sagardia, S., Seguel, O., Torres, C., Tapia, C., Franck, N., et al. (2010). Effect of water availability on growth and water use efficiency for biomass and gel production in Aloe Vera (Aloe barbadensis M.). Ind. Crops Prod. 31 (1), 20–27.
SNIARN (2008). Mexico´s state of the environment. Report 2008 Online SNIARN Available at: https://apps1.semarnat.gob.mx:8443/dgeia/informe_2008_ing/03_suelos/cap3_1.html (Accessed, 2022).
Somerville, C., Youngs, H., Taylor, C., Davis, S. C., and Long, S. P. (2010). Feedstocks for lignocellulosic biofuels. science 329 (5993), 790–792. doi:10.1126/science.1189268
Stape, J., and Binkley, D. (2010). Insights from full-rotation Nelder spacing trials with Eucalyptus in São Paulo, Brazil. South. For. a J. For. Sci. 72 (2), 91–98. doi:10.2989/20702620.2010.507031
Subedi, R. (2017). Assessing the viability of growing'Agave Tequilana'as a biofuel feedstock in Queensland, Australia. Int. J. Energy Econ. Policy 7 (4), 172–180.
Weiner, J., and Freckleton, R. P. (2010). Constant final yield. Annu. Rev. Ecol. Evol. Syst. 41, 173–192. doi:10.1146/annurev-ecolsys-102209-144642
Winter, K., and Smith, J. (1996). “An introduction to crassulacean acid metabolism. Biochemical principles and ecological diversity,” in Crassulacean acid metabolism (Berlin, Germany: Springer), 1–13.
Yan, X., Corbin, K. R., Burton, R. A., and Tan, D. K. (2020). Agave: A promising feedstock for biofuels in the water-energy-food-environment (wefe) nexus. J. Clean. Prod. 261, 121283. doi:10.1016/j.jclepro.2020.121283
Yan, X., Tan, D. K., Inderwildi, O. R., Smith, J., and King, D. A. (2011). Life cycle energy and greenhouse gas analysis for agave-derived bioethanol. Energy Environ. Sci. 4 (9), 3110–3121. doi:10.1039/c1ee01107c
Keywords: biofuel, spacing, productivity, tequila, Agave
Citation: Crawford W, Tan DKY and Van Ogtrop F (2022) Optimal planting density of Agave for maximising aboveground biomass: A systematic literature review. Front. Chem. Eng. 4:1039675. doi: 10.3389/fceng.2022.1039675
Received: 08 September 2022; Accepted: 22 November 2022;
Published: 02 December 2022.
Edited by:
Kevin M. Van Geem, Ghent University, BelgiumReviewed by:
Naveenkumar Rajendran, University of Wisconsin-Madison, United StatesJuan Gabriel Segovia Hernandez, University of Guanajuato, Mexico
Copyright © 2022 Crawford, Tan and Van Ogtrop. This is an open-access article distributed under the terms of the Creative Commons Attribution License (CC BY). The use, distribution or reproduction in other forums is permitted, provided the original author(s) and the copyright owner(s) are credited and that the original publication in this journal is cited, in accordance with accepted academic practice. No use, distribution or reproduction is permitted which does not comply with these terms.
*Correspondence: William Crawford, wdcra17@gmail.com