- 1Radiopharmaceutical Sciences, Centro de Ciências e Tecnologias Nucleares, Instituto Superior Técnico, Universidade de Lisboa, Bobadela, Portugal
- 2Centro de Química Estrutural, Departamento de Engenharia Química, Institute of Molecular Sciences, Instituto Superior Técnico, Universidade de Lisboa, Lisboa, Portugal
Cancer inflicts a tremendous burden on modern society, and more effective and selective treatments need to be developed. Metallodrugs have been extensively explored as alternatives to current chemotherapeutic regimens that suffer from shortcomings such as severe side effects and acquired resistance. Hydrazide-hydrazones are a family of organic molecules containing the hydrazone functionality attached to a carbonyl group (−C=N−NH−CO). These molecules are extremely versatile and can be obtained from modular reactions between hydrazides and aldehydes or ketones, of which a large library is commercially available. Hydrazide-hydrazones show numerous therapeutic effects, and typically, their metal complexes exhibit increased potency. This review aims to highlight the potential of hydrazide-hydrazone metal complexes in the field of anticancer research. It focuses on the literature published in the last 10 years (2013-2023) and describes their biological effects, their antiproliferative activity and their mechanisms of action. It is organized by metal ion, including Cu, Ru, Pt, Pd, V, other d-block, lanthanide, and Ga and Sn ions. It highlights the diversity of molecules being developed and can be used as a guide for developing new hydrazide–hydrazones as efficient anticancer agents.
1 Introduction
The prevalence of cancer is increasing worldwide. As life expectancy continues to rise, so does the number of cancer patients, which is not only associated with age but also with lifestyle. There is therefore a need to try to develop strategies to improve the patients’ condition. The mitigation of cancer often involves a myriad of treatments, chemotherapy included. There are several compounds in clinical use, and since the serendipitous discovery of the anticancer effect of cisplatin in the 1960s (Rosenberg, Vancamp, Trosko and Mansour, 1969), different metal complexes have been designed and tested for similar effects. In this respect, many researchers are developing new, effective organic molecules that can act as good metal ions’ chelators and functional groups like carbonyl and imine are some of the features used for this purpose. This review focuses on the development of new complexes bearing hydrazide-hydrazone ligands coordinated to different metal cations over the last decade, covering works published between 2013 and 2023, with some already appearing in early 2024. Despite the enormous amount of work done on imine-containing compounds, known as Schiff bases, hydrazide-hydrazones (HH), in which a carbonyl group is attached directly to the hydrazone function (Figure 1), account for only a modest percentage, as a search on the Web of Science Core Collection shows. Nevertheless, several examples can be found in the literature, and in this review we will present them according to the metal ion present in the complex.
Several commonly prescribed drugs contain a hydrazide-hydrazone functionality, highlighting the bioactivity of this type of molecules. Examples include isoniazide, an antibiotic used to treat tuberculosis, and nifuroxazide, an oral antibiotic used to treat colitis and diarrhoea. Hydrazide hydrazones are easy to synthesise and isolate in pure form. Furthermore, in the design of these molecules, it is relatively easy to change lipophilicity, total charge and planarity, by proper choice of the main core and/or substituents. This, together with the presence of different heteroatoms, gives these structures a tremendous capacity to coordinate metal cations, leading to a diversity of geometries, as well as preventing ligand degradation, with high potential for exploration in the anticancer field. Hydrazide-hydrazone containing compounds can act as anticancer agents by sequestering the iron cation, which is essential for normal cellular function (Brogyányi et al., 2022; Hruskova et al., 2016; Ibrahim and O'Sullivan, 2020; Mackova et al., 2012; Sung, Kerimoglu, Ooi and Tomat, 2022) or by participating in different cellular pathways involved in cell death. Although in a few cases, the complexes show lower anticancer activity than the corresponding ligand precursors (de Almeida et al., 2019; Hosny, Hassan, Mahmoud and Abdel-Rhman, 2019), in general, cytotoxicity is enhanced upon formation of the metal complex, as this review will showcase.
Despite the biological relevance of hydrazide-hydrazones only a few reviews have been published on their biological activity. In 2007 the biological activity of hydrazine was reviewed (Rollas and Küçükgüzel, 2007) and included many hydrazide-hydrazones, but excluded their metal complexes. The anti-tubercular activity of HH isoniazid derivatives was reviewed in 2017 (Hu et al., 2017). Popiołek has two reviews covering the research published between 2010-2016 and 2017-2021 on their antimicrobial activity (Popiołek, 2017; 2021). Tchekalarova and co-workers reviewed their anticonvulsant properties, focusing on animal studies (Angelova, Karabeliov, Andreeva-Gateva and Tchekalarova, 2016). Angelova and co-workers reviewed the literature on hydrazide-hydrazones capable of inhibiting InhA (a carrier protein reductase), resulting in antimycobacterial properties (Teneva, Simeonova, Valcheva and Angelova, 2023). The antibacterial activity of quinoline hydrazide-hydrazone derivatives, and their metal complexes has recently been reviewed by Verma et al. (Verma, Lal, Narang and Sudhakar, 2023). However, to the best of our knowledge, their anticancer activity has not been reviewed.
In this review each section is devoted to a metal ion or series of metal ions, but in papers where metal complexes with other metals were also tested, these are sometimes included in the section for the metal that gave the best results.
2 Copper complexes
The metal ion that undoubtedly stands out in the number of publications with HH ligands is copper. The vast majority of reports on their anticancer application are with this metal cation, and although the +2 oxidation state predominates, there are some examples of complexes with Cu(I) or mixed valence complexes.
Due to the importance and the biological role of isoniazid, a considerable number of publications describe complexes with HH in which a 2-acetylpyridine is present. In 2013, the synthesis and characterization of two Cu(II) complexes bearing benzoyl and thiophene hydrazones with 2-acetylpyridine (Cu-1, Figure 2) were reported (Alagesan, Bhuvanesh and Dharmaraj, 2013). The complexes bind BSA and DNA, and are able to cleave supercoiled DNA, even in the presence of ROS scavengers. They exhibited half-maximal inhibitory concentrations (IC50) below 5 µM in the cancer cell lines tested: Ehrlich ascites carcinoma (EAC), Dalton’s ascites lymphoma (DAL), human cervical cancer (HeLa) and pancreatic cancer (PANC1), and above 50 µM in a non-cancerous cell line (NIH 3T3). The complexes showed selectivity towards different types of cancer cells, suggesting that they have potential for anti-cancer drug development.
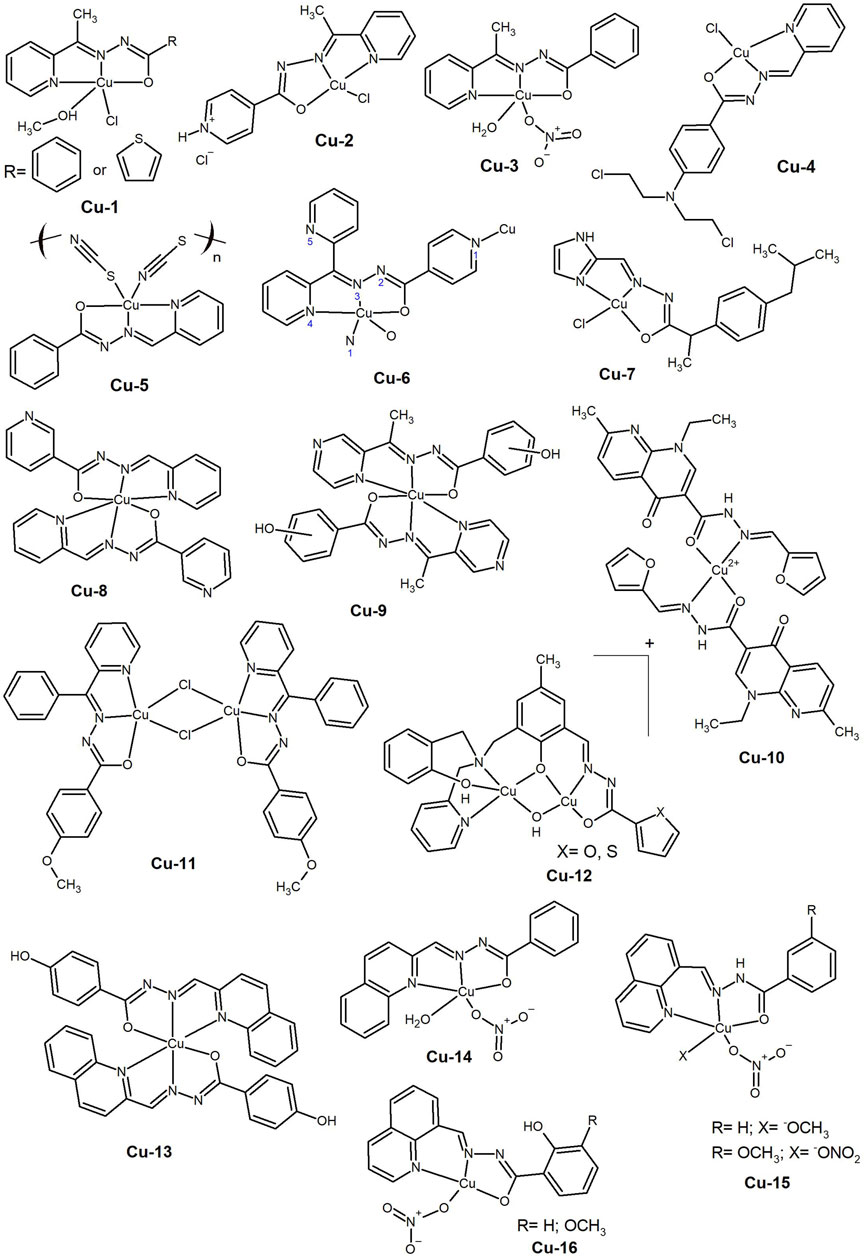
Figure 2. Copper(II) complexes bearing 2-acetylpyridine based hydrazide-hydrazone ligands (Cu-1 to polymeric Cu-6) and a hydrazide-hydrazone with a combination with the nonsteroidal anti-inflammatory drug ibuprofen (Cu-7). Copper(II) complexes with 2:1 ligand-to-metal ratios (Cu-8 to Cu-10) and dinuclear composition (Cu-11 and Cu-12) as well as bearing quinoline based hydrazide-hydrazone ligands (Cu-13 to Cu-16).
The wide range of cancer cells affected by this family of compounds has been further investigated by Firmino et al. (2016). The N,N,O metal chelator 2-pyridinecarboxaldehydeisonicotinoyl hydrazone (HPCIH) and its derivatives 2-acetylpyridine-(HAPIH), 2-pyridineformamide-(HPAmIH) and pyrazineformamide-(HPzAmIH) were used in the synthesis of four Cu(II) complexes. In the complexes [Cu(HPCIH)Cl2] and [Cu(HAPIH)Cl2] the hydrazones coordinate as zwitterions, whereas in [Cu(HPAmIH)Cl2] and [Cu(HPzAmIH)Cl2] neutral ligands are bound to the metal centre, demonstrating the diversity of structures made possible by these ligands. In general, the Cu(II) complexes presented better cytotoxic activity than the corresponding free ligands in the human cancer cell lines OVCAR-8 (ovarian), SF-295 (glioblastoma multiforme) and HCT-116 (colon adenocarcinoma), with [Cu(HAPIH)Cl2] (Cu-2, Figure 2) showing IC50 values in the submicromolar range, comparable to the reference drug, doxorubicin. Although the authors demonstrated the importance of coordination to the Cu(II) centre for the production of ROS, there is no clear evidence as to why the complexes showed these results in such different cancer cell lines, while leaving healthy cells unaffected.
The role of the metal centre in ROS-mediated cellular processes has been demonstrated by Cu(II) complexes. The synthesis of three Cu(II) complexes of acetylpyridine benzoyl hydrazone (HL) with different stoichiometries and coordination modes was reported in 2016 (Deng, Gou, Chen, Fu and Deng, 2016). The complexes were [Cu(L)(NO3)(H2O)]·H2O, [Cu(L)2], and [Cu(L)(HL)]·(NO3)(Sas) (Sas= salicylic acid), in which the L− is the enolate and HL is the keto resonance form. All complexes exhibited anti-proliferative effects on HeLa, human lung carcinoma (A549 and cisplatin-resistant A549cisR), and human breast cancer (MCF-7) cell lines, but showed no selectivity as IC50 values for the non-cancerous WI-38 cell line were in the same concentration range. The IC50 values obtained for [Cu(L)(NO3)(H2O)]·H2O (Cu-3, Figure 2) (ca. 1–2 μM) were slightly lower than those of the 2:1 ligand-to-metal ratio complexes. This complex showed a strong capacity to promote apoptosis through the intrinsic ROS-mediated mitochondrial pathway and to inhibit the activity of the prosurvival Bcl-2 family of proteins.
In addition to the deregulation of apoptotic-related proteins, ROS production can often disrupt the function of other cellular components. In 2017, [Y. L. Yang et al., (2016)] carried out the synthesis of benzaldehyde nitrogen mustard-2-pyridine carboxylic acid hydrazone and its Cu(II) complex (Cu-4, Figure 2). The complex showed much higher anticancer activity than the free ligand (10 times higher), suppressing the growth of liver HEPG2 and HCT-116 cancer cell lines (IC50 ca. 3–5 μM). Both compounds induced ROS generation with upregulation of caspase-8 and Bax and downregulation of Bcl-2 proteins, leading to cell death by apoptosis and cell cycle arrest in S phase. The authors also identified changes in the lysosomal membrane permeability (LMP) following exposure to the compounds.
To be effective, the complexes must be taken up by the cells. The synthesis of Cu(II), Ni(II) and Co(III) complexes with the Schiff base ligands N′-(pyridine-2-ylmethylene)benzohydrazide, 2-(2-hydroxybenzylidene)hydrazine-1-carboxamide and 2-(pyridin-2-ylmethylene)hydrazine-1-carboxamide was reported by Fekri and co-workers (Fekri, Salehi, Asadi and Kubicki, 2019). The complexes showed enhanced anticancer activity compared to the corresponding ligands, exhibiting dose-dependent anti-proliferative activity in both the AGS gastric adenocarcinoma and SW174 colorectal cancer cell lines, with the Cu(II) complex being the most active of the series (Cu-5, Figure 2). The authors explained the increased anticancer activity of the complexes by Tweedy’s chelation theory, stating that the reduction in polarity and increase in lipophilic character compared to the free ligands, makes it easier for the complexes to cross biological membranes. Interestingly, some of the Cu-complexes were further evaluated and overexpression of the Bcl-2 protein, and apoptosis with cell cycle arrest at the G1/S checkpoint were observed in MCF-7 cells (Asghariazar et al., 2023).
Three novel Cu(II) complexes with the tridentate ligand di(2-pyridil) ketone 1-adamantoyl hydrazine, with either halogens or with isothiocyanate as co-ligands were synthesised (Rodic et al., 2016). The Cu(II) complexes exhibited high cytotoxic activity in the cancer cell lines HeLa, LS174, A549, K562 and MDA-MB-231, with IC50 values ranging from 0.5 to 3.2 µM. The complexes induced apoptosis through a caspase-3 dependent mechanism, causing chromatin condensation, nuclear fragmentation and changes in the cellular morphology. Cu(II) complexes also inhibited the angiogenesis process in vascular endothelial cells. In the same year, the synthesis of Cu(II) and Co(II) complexes with N′,N′-4-bis(2-pyridyl)succinohydrazide and N′,N′-4-bis(2-hydroxybenzylidene)succinohydrazide was reported (Golla, Adhikary, Mondal, Tomar and Konar, 2016). The complexes formed either 1D chains or double helical structures with antiferromagnetic exchange interactions or antiferromagnetic spin canting through supramolecular H-bonding, respectively. The complexes interacted with DNA and exhibited nuclease activity in a ROS-dependent process. The authors reported that the Cu(II) complexes presented cytotoxicity against HeLa (IC50 = 24.42 and 35.73 µM, respectively), A549 (IC50 = 133.85 and 388.1 µM) and MDA-MB-231 (IC50 = 72.67 and 80.95 µM) cancer cells, despite showing considerably high IC50 values. However, Annexin and PI staining assays showed induction of apoptosis, corroborating the cytotoxicity. Deng et al. (2016) also prepared a dipyridyl ketone isonicotinoyl hydrazone and its Cu(II) complex (Cu-6, Figure 2). They reported a 1D zigzag polymeric structure in the solid-state, which hydrolysed to the positively charged monomeric form in aqueous solution. The complex showed high anticancer activity against Bel-7402, HeLa and MCF-7 cells, including a drug-resistant strain of the latter (IC50 = 1.5–4.1 µM), being significantly more active than the free ligand. The complex induced the production of ROS leading to a caspase-dependent apoptosis mechanism of cell death.
The examples above demonstrate that Cu-complexes with high antiproliferative activity can be obtained using HH ligands based on 2-acetylpyridine. This activity seems to be linked to cell death processes mediated by ROS. However, only in vitro studies have been conducted, and additional research is required to investigate the molecular mechanism of action, as well as to evaluate safety, efficacy, and delivery in vivo.
The use of pharmaceutically active molecules as components of HH ligands seems to be an interesting strategy, as proposed by (Kaur, Chikate, Bandyopadhyay, Basu and Chikate, 2020), with the synthesis of HHs combining aldehydes of pyridine and imidazole with non-steroidal anti-inflammatory drugs (NSAIDs) and their corresponding Cu(II) complexes. The complexes show a square planar geometry and the ligands behave as bidentate in diclofenac-hydrazone with [Cu(L)2] stoichiometry and as tridentate in ibuprofen-hydrazone conjugates with [Cu(L)Cl] stoichiometry. The compounds revealed moderate cytotoxic activity against lung (A549) and colon (HTC-116) cancer cell lines, with the lowest IC50 values being determined in the triple negative breast cancer cell line (MDA-MB-231) (IC50 = 3.4–6.6 µM). The conjugate ibuprofen-imidazole hydrazone and its Cu(II) complex showed the best set of results for the anticancer activity (Cu-7, Figure 2), demonstrating the advantage of the 1:1 stoichiometry.
Nevertheless, several authors have investigated complexes with a ligand-to-metal ratio of 2:1 and achieved promising results. The synthesis of (E)-N'-(1-(pyridin-2-yl)ethylidene) nicotinohydrazide and its Cu(II), Mn(II), Co(II) and Cd(II) complexes was reported in 2017 (Shen et al., 2017). These complexes exhibited antiproliferative activity on various human cancer cell lines, including lung (A549), gastric (BGC823) and oesophageal (Eca109), in a concentration-dependent manner (IC50 6.5–18.3 µM). The Cu(II) complex (Cu-8, Figure 2) was the most cytotoxic of the series. It promoted apoptosis, increased the levels of the pro-apoptotic proteins p53 and Bax, and decreased the levels of Bcl-2 in the oesophageal cell line.
The synthesis of (E)-x-hydroxy-N’-(1-(pyrazin-2-yl) ethylidene)benzohydrazides, where x corresponds to the position of the hydroxyl group in the benzoyl ring, and their corresponding Cu(II) complexes (Cu-9, Figure 2) was reported in 2019 by [P. Yang et al. (2019)]. The complex with the hydroxyl group in the ortho position revealed poor solubility in water and DMSO and the authors did not present any biological data for it. The other two complexes bind readily to calf thymus DNA (ctDNA) through intercalative and minor groove binding modes and exhibit oxidative cleavage of supercoiled plasmid DNA (pUC19) in the presence of ascorbic acid as an activator. Cell viability studies showed high cytotoxicity towards the human cervical cancer cell line HeLa (IC50 ca. 11 μM), and low toxicity towards the normal mouse fibroblast cell line L929. The effects of the complexes on the cell cycle of HeLa and L929 cells were studied by fluorescence imaging using Hoechst 33342 and PI staining, showing that both complexes induced cell death mainly by apoptosis. Despite the good results, the authors did not present safety assays and in vivo cancer model studies that would have made a strong contribution to the field.
In 2022 Chennam et al. (2022) synthesized a Cu(II) complex with the ligand ((1-ethyl-Nʹ-furan-2-yl)-methylene)-1,4-dihydro-7-methyl-4-oxo-1,8-napthyridine-3-carbohydrazide (Cu-10, Figure 2), which is able to bind BSA and act as a DNA intercalator, as well as exhibiting radical scavenging activity. This complex also presented anti-proliferative activity in the cancer cell lines HeLa (IC50 = 26 µM) and MCF-7 (IC50 = 42 µM), inducing morphological changes such as nuclear swelling, cytoplasmic blebbing and late apoptosis. Although relevant, the IC50 values of these complexes are considerably higher that the ones presented in this review for related compounds.
The use of multinuclear structures can also provide complexes with anticancer potential. Recently, the synthesis of two mononuclear complexes of Co(II) and Ni(II) and a Cl-bridged dinuclear Cu(II) complex of 2-benzoylpyridine-4-methoxybenzoyl hydrazine was reported (Kanchanadevi et al., 2021). The complexes showed the ability to act as ctDNA intercalators and to bind BSA. In addition, all complexes showed better antioxidant activity than ascorbic acid in the DPPH assay. The anticancer activity was evaluated in the MCF-7 and A549 cell lines, with the Cu(II) complex (Cu-11, Figure 2) presenting IC50 values (10–20 µM) identical to those of doxorubicin and cisplatin. It should be noted that the dinuclear structure of the Cu-complex may not be kept in solution and further speciation studies should be carried out to determine the active species, as well as the mechanisms involved in cell death.
The presence of more than one metal cation in the complex structure is also possible with the use of a single ligand as shown by Rada et al. (2019). The authors reported the synthesis of two dinuclear Cu(II) complexes bearing a novel hydrazide ligand and a µ–hydroxo bridge between the metal centres (Cu-12, Figure 2). The ligand precursors showed hydrolysis in a 10% (v/v) DMSO medium but coordination to Cu(II) prevented this phenomenon. Furthermore, the authors reported that the dinuclear mono-positive complexes predominate in such a medium, and are the active species. Both complexes showed high affinity for BSA, binding to this protein in a reversible manner. Meanwhile, the nuclease activity demonstrated by the complexes, proved to be irreversible. The complexes displayed antiproliferative activity against the human colon cancer cell lines HCT116 and HT29, as well as against the human breast cancer cell line MDA-MB-231 and the murine melanoma cell line B16F10, with IC50 values below 2 µM. Overall, these are very interesting compounds that should be further studied.
Another nitrogen-containing fragment found in several HH molecules in the literature is quinoline. In fact, larger aromatic systems show good ADME properties as they can easily bind to the hydrophobic pockets of albumins, which are the main drug transporters in blood. In 2016, Du et al. (2016) synthesized (E)-4-hydroxy-N'-(quinolin-2-ylmethylene)benzohydrazide and its Cu(II), Co(II), and Ni(II) complexes in the form of [M(L)2]. Only the Cu(II) complex (Cu-13, Figure 2) showed antiproliferative activity against the tested cancer cells (HeLa, A549 and A549cisR), but with an IC50 value of the same magnitude in the normal lung fibroblasts (WI-38), in the low micromolar range. The studies showed the involvement of ROS and activation of caspase-7 and caspase-3, leading to mitochondrial dysfunction and apoptosis. More recently, the synthesis of a Cu(II) complex with a new Schiff base derived from quinoline-2-carbaldehyde and benzoylhydrazide (Cu-14, Figure 2) was described (Biswas et al., 2021). The complex is able to bind to BSA and HSA and shows intercalative and groove binding to ctDNA with a low binding constant. It presented a low IC50 value in the human skin carcinoma A431 cell line, making it a strong candidate for further investigation. This work represents a departure from the previous substitution position used in the compounds developed by Hu et al. (2017). The mononuclear complexes were prepared with quinoline-8-carbaldehyde and benzoylhydrazide or 3-methoxybenzoylhydrazide (Cu-15, Figure 2). Both complexes showed higher interactions with the hydrophobic residues of HSA at pH 7.5 than at pH 4.7, suggesting that they can be readily released in the acidic tumor microenvironment. In addition, the growth inhibitory potency against the HeLa cell line was in the low micromolar range, slightly lower than that of cisplatin. Further cell studies showed that the complexes could induce G2 phase cell cycle arrest in HeLa cells, ultimately leading to apoptosis through the mitochondria-mediated pathway. The synthesis of two copper(II) complexes with 8-quinolinecarbaldehyde o-vanilloylhydrazone and 8-quinolinecarbaldehyde salicylhydrazone (Cu-16, Figure 2) had been reported by the same group (Hu et al., 2016) in 2016, which were capable of inducing mitochondrial dysfunction. Both complexes exhibited enhanced cytotoxicity against hepatocellular BEL-7402, Hep-G2, lung NCI-H460, gastric MGC80-3 and HeLa tumor cells (IC50 between 7 and 30 µM), compared to free ligands, copper(II) salt, and cisplatin. They also showed selectivity towards cancer cells, as MGC80-3 cells were more sensitive to these two complexes than the normal liver cells. Cytotoxicity and mechanism of action studies suggested that the complexes could induce MGC80-3 cell cycle arrest in G1 phase, induced by limiting the supply of cyclins D1 and E1 and inhibiting the activity of G1-phase promoting cyclin-Cdk complexes, leading to cell apoptosis via the activation of the Bcl-2 protein. Thus, clearly the use of a quinolone moiety in HH ligands results in Cu(II) complexes with relevant in vitro cytotoxicity that needs further evaluation.
As mentioned above, there may be some advantages in using larger aromatic systems to increase biological interactions (involved in transport and uptake). The synthesis of Cu(II) complexes bearing 2-oxo-1,2-dihydrobenzo[h]quinoline-3-carbaldehyde HH (Cu-17, Figure 3) was carried out by Ramachandran et al. (2018). The aroyl moiety had a hydroxyl substituent in the–ortho, –meta, or –para positions and the interaction with DNA was favoured by the latter two positions, since in this case the OH was not involved in intramolecular hydrogen bonding. The presence of an additional phenyl ring in the quinoline moiety significantly increased the cytotoxicity, even against platinum-resistant ovarian cancer cells, C13* (IC50 < 5 µM). The complexes showed nuclease activity only in the presence of reducing agents and the authors suggested that these compounds could act as DNA intercalators.
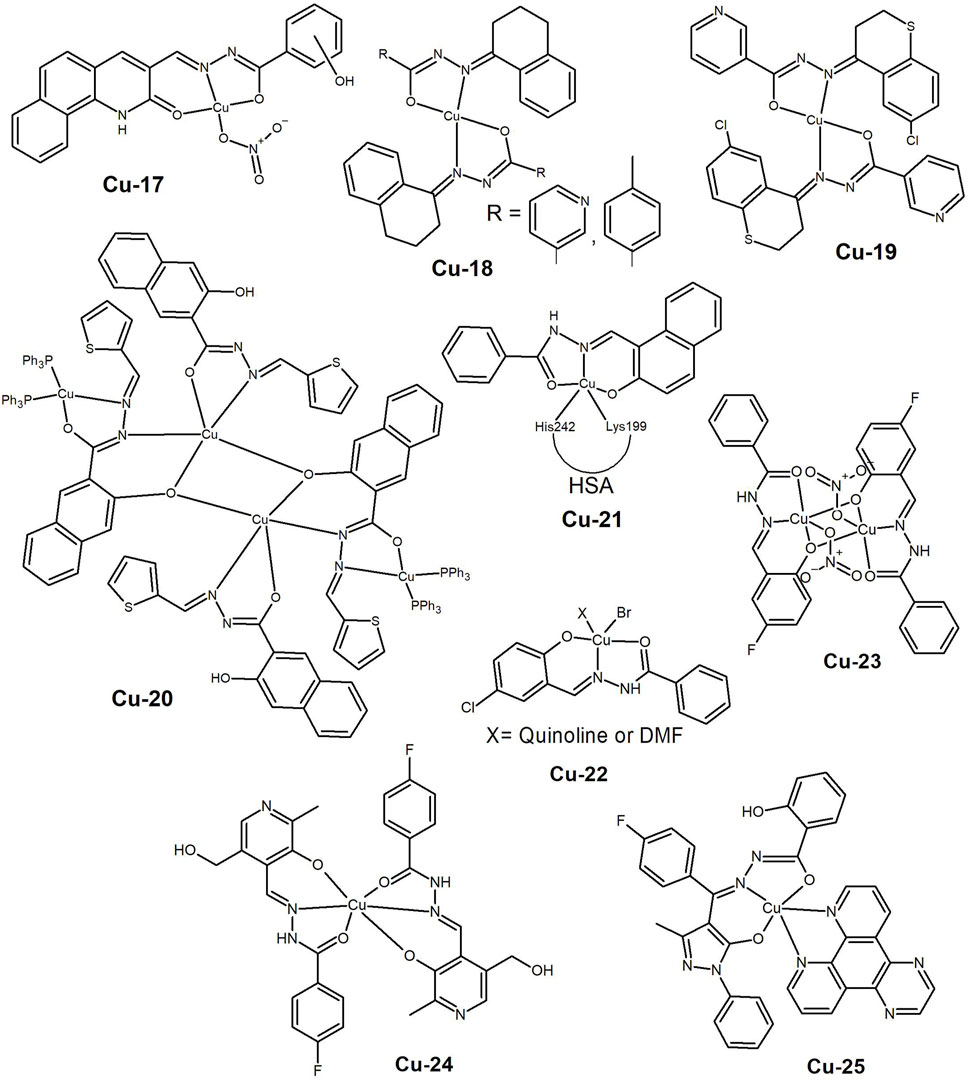
Figure 3. Copper(II) complexes with hydrazide-hydrazone ligands of different heterocycle rings (Cu-17 to Cu-21) and bearing hydrazide-hydrazone ligands and halogen atoms in different positions of the complex (Cu-22 to Cu-25).
In 2021, Devi et al. (2021) reported the synthesis of HH obtained from the condensation of α–tetralone with hydrazide derivatives (nicotinic acid, benzoic acid, p–toluic acid and isonicotinic acid) and their Cu(II), Co(II), Ni(II) and Zn(II) complexes. All compounds were active against the three cancer cell lines tested (A549, Hela and MCF-7, IC50 < 20 µM), but the Cu(II) complexes bearing the nicotinic and methyl benzoic hydrazones presented the highest activity (Cu-18, Figure 3). In addition, the compounds showed some selectivity towards cancer cells, being significantly less toxic to the normal rat skeletal myoblast L6 cell line. Next, the authors considered complexes of the same metal ions and similar ligands, replacing the α–tetralone with 6-chlorothiochroman-4-one (Devi, Kumar, Kumar, Jindal and Poornachandra, 2022). Also in this study, the Cu(II) complex derived from nicotinic hydrazide was found to be the most cytotoxic (Cu-19, Figure 3), with activity in the low micromolar range against lung A549, prostate DU145 and colorectal SW620 cancer cell lines. The complex leads to ROS generation and induction of apoptosis through mitochondrial depolarization.
Although nitrogen-containing rings are commonly used, there are also some interesting examples of compounds bearing the naphthalene ring. In 2019, Anu et al. (2019) carried out the synthesis of a mixed valence Cu(I)/Cu(II) complex bearing a ligand derived from the condensation of thiophene-2-carboxaldehyde with 3-hydroxy-2-naphthoic hydrazide (Cu-20, Figure 3). The structure, which contains four ligands and four metal ions, consists of a central di-µ-O formed by the hydroxyl groups of two of the ligands bridging two Cu(II) centres, while the hydrazide-hydrazone moieties bind the Cu(I) centres, each containing also two PPh3 of the metal precursor. The other two ligands bind to the central Cu(II) centres via the hydrazide-hydrazone moieties. The complex binds BSA and interacts with ctDNA in an intercalative mode. The cytotoxic activity of the complex was tested in the A549 and in the MCF-7 cancer cell lines and its IC50 values are comparable to those obtained for cisplatin.
However, the disadvantage of using compounds with large aromatic systems is that this often leads to poor aqueous solubility. One way to circumvent this problem, while maintaining the integrity of the complex, is to exploit the transport ability of albumins. The synthesis of Cu(II) complexes of a 2-hydroxy-1-naphthaldehydebenzoyl hydrazone with pyridine, imidazole or indazole as co-ligands was reported in 2015 (Gou et al., 2015). Upon binding of the complexes to HSA, the co-ligands were substituted by amino acid residues at the hydrophobic cavity of the protein (Cu-21, Figure 3). The complexes showed enhanced cytotoxicity in MCF-7 cells with cell cycle arrest in the G2/M phase by targeting cyclin-dependent kinase 1 (CDK1). In addition, the complexes induced the production of ROS, leading to cell apoptosis through a mitochondrial mediated pathway.
In addition to nitrogen-containing ligands and larger aromatic systems, promising results have also been obtained with molecules such as salicylaldehyde and its derivatives. These compounds readily react with carboxylic acid hydrazides to form bi- or tridentate ligands, capable of coordinating a variety of metal cations. Two (E)-N′-(5-chloro-2-hydroxybenzylidene)benzohydrazide Schiff base Cu(II) complexes (Cu-22, Figure 3), one with a quinoline as co-ligand and the other with a DMF molecule were reported in 2017 by Gou et al. (2017). The authors described the development of folic acid functionalized HSA nanoparticle carriers, which enhanced the anticancer activity and selectivity of the complexes towards Bel-7402 liver cancer cells by 2 – 3 fold, providing another example of the importance of this transport biomolecule and targeting strategies to deliver the compounds and increase selectivity.
The incorporation of halogen atoms, particularly fluorine, is a common strategy in the design of pharmaceutical drugs. This was done in the work of Jiang et al. (2020) who described dinuclear Cu(II) complexes with halogen-substituted 2-hydroxybenzylidene benzohydrazides. The three complexes revealed high anticancer activity in the tested cell lines (Bel-7402, HeLa, MCF-7 and MGC-803, IC50 ≤ 5 µM), but also an anti-proliferative effect on the normal lung fibroblasts (WI-38). The cytotoxicity of the complexes followed the order F>Cl>Br, and the F-substituted complex (Cu-23, Figure 3) induced mitochondria-mediated apoptotic cell death in HeLa cells. The authors also reported that all complexes bind to HSA in the hydrophobic cavity of subdomain IIA. The same strategy was followed by Kavitha et al. (2021) who reported a new Schiff base, 4-fluoro-N-((3-hydroxy-5-(hydroxymethyl)-2-methylpyridin-4-yl)methylene)benzohydrazide, and its Ni(II), Cu(II) and Zn(II) complexes. The complexes interacted with ctDNA in an intercalative mode and were able to cleave supercoiled pBR322 DNA to its nicked form in the absence of any oxidant. The Cu(II) complex (Cu-24, Figure 3) showed moderate cytotoxicity in the HeLa and MCF-7 cancer cell lines, but a good inhibitory effect on the A549 lung cancer cell line. In 2019, Wang et al. (2019) reported mixed Cu(II) complexes bearing the novel ligand N-(1-phenyl-3-methyl-4-(4-fluorobenzoyl)-5-pyrazolone)-2-salicylidene hydrazide, and 2,2-bipyridine (bipy), 1,10-phenanthroline (phen) or dipyrido[3,2-d:2,3-f]quinoxaline (dpq) as co-ligands. The complexes were able to interact with DNA and showed IC50 values in the low µM range in the human cervical cancer HeLa cells and in the human oesophageal cancer Eca109 cells. The Cu(II) complex with dpq as co-ligand (Cu-25, Figure 3) induced apoptosis in a dose-dependent manner in the Eca109 cells.
These works demonstrate the role of fluoride in enhancing the activity of complexes probably through intermolecular interactions. The use of N,N-containing co-ligands has also been shown to be advantageous. Larger systems with nitrogen present outside the coordination sphere of the metal centre are likely to contribute to overall biological activity, as demonstrated in the latter study.
Sutradhar et al. (2017) reported four mixed ligand Cu(II) complexes of the formula [Cu(L)(X)]2, where L refers to the dianionic form of (5-bromo-2-hydroxybenzylidene)-2-hydroxybenzohydrazide and X to monodenatate N-ligands, namely, pyrazole, pyridine, imidazole and 3-pyridinecarbonitrile. Only the Cu(II) complex bearing the 3-pyridinecarbonitrile presented reasonable IC50 values (<30 µM) against the tested cancer cell lines (A2780, HCT116, MCF-7 and A549). The cell death mechanism induced by this complex was proposed to follow apoptosis with evidence of chromatin condensation and nuclear fragmentation. Further studies are needed to evaluate its full potential.
The use of simple building blocks as starting materials for ligands’s synthesis remains an efficient strategy in drug design. This rationale was followed by Ji et al. (2018) in 2018, when they used salicylaldehyde isonicotinoyl hydrazone as a Cu(II) ionophore to target the redox homeostasis of cancer cells. The Cu(II) complex formed (Cu-26, Figure 4) preferentially kills HepG2 cells over human umbilical vein endothelial cells (HUVEC). The ligand also showed higher cytotoxicity and better selectivity than clioquinol, a copper ionophore in clinical trials, and in the presence of Cu(II) it induced apoptosis in HepG2 cells in a dose-dependent manner through a mitochondria-mediated pathway. Although the use of ionophores based on HH ligands is a promising strategy to target cancer cells, the biological effect is clearly due to the formation of a Cu(II)-complex with the ligand.
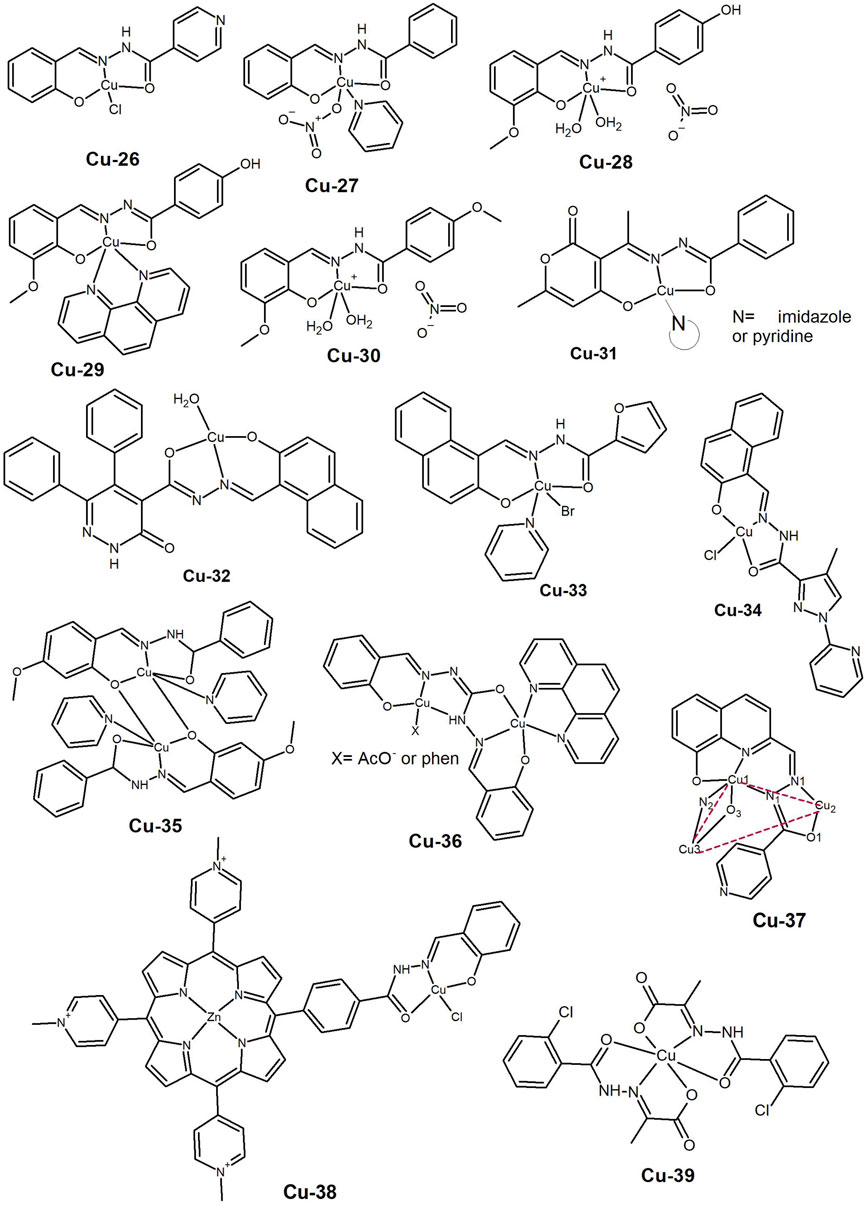
Figure 4. Copper(II) complexes bearing salicylaldehyde (Cu-26 to Cu-30), dehydroacetic acid benzoyl (Cu-31) and naphthoyl (Cu-32 to Cu-34) based hydrazide-hydrazone ligands. Copper(II) complexes with multinuclear aggregates (Cu-35 to Cu-37), conjugated with a Zn-porphyrin (Cu-38) or bearing an aliphatic hydrazone ligand (Cu-39).
In the same year, Mo et al. (2018) reported the synthesis of Cu(II) complexes bearing simple hydrazine-hydrazone ligands, with and without pyridine as co-ligand. The complexes exhibited good cytotoxic profiles against the tested human cancer cell lines (Bel-7402, MCF-7, A549 and WI-38), including the cisplatin-resistant lung A549cisR line, with the ternary complexes showing lower IC50 values (< 6.5 µM) than the corresponding complexes without pyridine. The complexes interacted with DNA and induced morphological changes in the MCF-7 cells. The complex with the best results, Cu-27 (Figure 4), induced DNA damage leading to a ROS-mediated mitochondrial dysfunction apoptosis pathway. The authors provided evidence for the stability of the ternary complexes. Therefore, the improved cytotoxic profile is likely due to the enhanced lipophilicity of these complexes, resulting in better cellular uptake.
This synthetic route was also chosen by Burgos-Lopez et al. (2019), who reported the synthesis of a new Cu(II) complex bearing a tridentate N-acylhydrazone derived from 4-hydroxy-benzohydrazide and 2-hydroxy-3-methoxybenzaldehyde (Cu-28, Figure 4). The ligand binds as a mono-anion and a NO3− acts as a counter-ion. The complex presented anticancer activity in the cancer cell lines A549, MCF-7 and MDA-MB-231, MG-63 (bone) and Jurkat (leukemia) (IC50 ≤ 12 µM). The authors later reported the improved cytotoxicity of the complex when bipy or phen were used as co-ligands in ternary complexes (Burgos-Lopez et al., 2022). The amido-iminol tautomerism of the hydrazone ligand was clearly demonstrated as it coordinates through its ONO donor atoms as monoanionic in the cationic complex [Cu(HL)(bipy)](NO3), but as dianionic in the neutral [Cu(L)(phen)] complex. The ternary complexes exhibited increased cytotoxicity against the same cancer cell lines (MG-63, MCF-7, MDA-MB-231 and A549) compared to the free ligand and cisplatin. Furthermore, the phen-containing complex (Cu-29, Figure 4) demonstrated lower IC50 values than the bipy complex. The potential of this family of compounds was further supported by the synthesis of the Cu(II) complex bearing a similar ligand. In this case, the 4-methoxy-benzohydrazide was used instead of the 4-hydroxy-benzohydrazide (Cu-30, Figure 4) (Balsa et al., 2021). The complex showed a lower IC50 value than cisplatin in the MCF-7, MDA-MB-231, A549, MG-3, HT-29 and L929 cancer cell lines. In addition, the cytotoxicity of the complex was observed in both monolayer (2D) and spheroids (3D) derived from human breast cancer cells. The complex increased the intracellular ROS levels, and exhibited equal cytotoxicity in the non-tumoral breast MCF10 cell line, indicating no selectivity.
The strategy of using N-heterocycles as co-ligands has been widely explored by various research groups in the preparation of hydrazide-hydrazone complexes. Cu(II) complexes of dehydroacetic acid benzoyl hydrazone with different co-ligands were recently reported (Elsayed, Elnabky, di Biase and El-Hendawy, 2022). The compounds were tested on three human cancer cell lines (MCF-7, HepG2 and HCT116 (colon)), and on normal lung fibroblasts (WI38). The IC50 values were approximately 30 µM. The complexes with imidazole and pyridine (Cu-31, Figure 4) showed better results than cisplatin, inducing DNA fragmentation, cell death by apoptosis and cell cycle arrest at the G2/M phase.
While the use of smaller and simpler molecules has shown promising results, some authors are exploring the use of larger less explored aromatic systems. These can offer different properties to the complex and target alternative pathways for cell death. Abdelrahman et al. (2022) reported the synthesis of complexes with different metal ions that bear the ligand 5,6-diphenyl-4-carboxylicacid[1-(2-hydroxynaphthalene)methylidene]-hydrazide-3(2H)-pyridazinone. The Cu(II) complex (Cu-32, Figure 4), and the free ligand showed IC50 values comparable to cisplatin in the liver Hep-G2 cancer cell line. Thus, it appears that coordination to Cu(II) does not enhance the activity to the ligand. Computational studies suggest that the compounds target the enzyme VEGFR-2, resulting in DNA damage and programmed cell death. Further studies are needed to evaluate the potential of the compounds.
The naphthoyl moiety was also chosen by Wu et al. (2023) to construct ligands with hydrazide-hydrazones bearing different substituents. The copper complex containing furanyl as a substituent and pyridine as a co-ligand (Cu-33, Figure 4) showed cytotoxicity on A549 lung cancer cells, even in the 3D spherical model. A wound healing assay showed that the complex had anti-metastatic activity, while flow cytometry and fluorescence imaging showed cell cycle arrest at the S phase in a mitochondria-mediated apoptotic cell death pathway. The conjugation of the naphthoyl moiety with aromatic heterocyclic rings yields favorable biological outcomes, albeit with a multitude of modes of action. Our group (Ribeiro et al., 2017) disclosed that a Cu(II) complex (Cu-34, Figure 4) presented micromolar IC50 values against prostate and breast cancer cells by disrupting vital physiological elements involved in membrane potential and cellular homeostasis, such as P, K and Ca.
Like 2-acetylpyridine, salicylaldehyde can also form copper complexes in multinuclear systems resulting in a variety of structures that may affect the properties of the compounds. In 2017, Cindric et al. (2017) carried out the synthesis of Cu(II) complexes using ligands containing 2-hydroxy-benzaldehyde benzhydrazide derivatives and with N-donor co-ligands such as pyridine, 4,4′-bipyridine and 1,10-phenanthroline. All complexes exhibited higher cytotoxicity than the free ligands in the THP-1 (leukaemia, IC50 < 1 µM) and HepG2 (liver, IC50 2–13 µM) cancer cells. Additionaly, Cu(II) complexes with pyridine as a co-ligand (Cu-35, Figure 4) presented lower IC50 values than the reference compound, Staurosporine. Patel et al. (2019) also reported the synthesis of tetranuclear phenoxy-bridged Cu(II) complexes bearing the acetic acid (2-hydroxy-3-methoxy-benzylidene)-hydrazide ligand. The complexes exhibited an antiproliferative effect on IMR32 (neuroblastoma), MCF-7, HepG2 and L132 (lung) cell lines with IC50 values ca. 30 μM. The authors proposed that this is related to their ability to interfere with the normal content of cellular Bax, Bcl-2, caspase-9, and p53 proteins, probably due to copper’s redox activity.
In 2019, Parsekar et al. (2019) synthesised 1,5-bis(salicylidene)carbohydrazide and its dinuclear Cu(II) complexes, with phenanthroline as a co-ligand (Cu-36, Figure 4). The compounds effectively catalysed the hydrolytic cleavage of supercoiled pUC19 DNA, and exhibited relevant cytotoxicity (IC50 < 9 µM) towards A549 and MCF-7 cell lines, while being relatively less toxic towards the keratinocyte HaCaT normal cell line. The results of Annexin-V/PI dual staining suggest the induction of an apoptotic pathway for the anticancer activity of these complexes. Flow cytometry experiments on cell cycle showed a significant increase in the G2/M phase in both A549 and MCF-7 cell lines caused by the two compounds. However, the complexes were found to have different effects on ROS levels in different cell lines. In A549 cells, the complexes increased ROS levels, while in MCF-7 cells they acted as scavengers or inhibitors of ROS. The following year the same authors reported the cytotoxic effect of the complexes on the HeLa cancer cell line, along with an extensive study on the compounds’ interactions with HSA (Parsekar et al., 2020). Clearly there is potential for these compounds to be used in cancer therapy, but in vivo studies are necessary to evaluate their safety and therapeutic index.
Recently, our group reported (Ribeiro et al., 2023) on a series of Cu(II) complexes that bear 8-hydroxyquinoline HH ligands. Solution equilibrium studies revealed a monomer-trimer equilibrium in aqueous solution in the micromolar conditions of the biological assays. Theoretical studies confirmed the thermodynamically favoured di-anionic character of the hydrazones. The complexes exhibited greater activity against melanoma (A-375) cells, than lung (A549) cancer cells, with IC50 values in the low µM range in both cases. Among the complexes, Cu-37 (Figure 4) which bears the isoniazid derivative showed greater promise due to its ability to induce ROS formation, DNA double-strand breaks and apoptosis-mediated cell death.
One of the main difficulties reported in the literature concerns the low aqueous solubility exhibited by this type of complexes. Therefore, efforts have been made to develop strategies to overcome this drawback. In 2018, Feng and Liu (Feng and Liu, 2018) coupled salicyloylhydrazone to Zn(II) porphyrins and coordinated them to Cu(II) (Cu-38, Figure 4). The resulting complexes are water-soluble and interact with ctDNA through partial intercalation. The complexes presented moderate cytotoxicity against A549 and HepG2 cancer cell lines, with an IC50 range of 12–35 µM. However, a much higher concentration (>200 µM) was required to induce cell death in the non-cancerous Hs 578Bst cells, indicating selectivity towards cancer cells. This work presents an alternative method for achieving water solubility that warrants further exploration.
While aromatic compounds are more commonly used, there are instances where aliphatic aldehydes have been used as starting materials. For example, Hegde et al. (2017) reported the synthesis of ethyl 2-(2-(2-chlorobenzoyl)hydrazono)propanoate and its Cu, Co(II), Ni(II) and Zn(II) complexes. The Cu(II) complex (Cu-39, Figure 4) demonstrated the ability to cleave pBR322 DNA and exhibited good anticancer activity (IC50 1.5–55.4 µM) in the cancer cell lines KB (oral), A431 (skin), Mia-Pa-Ca (pancreas), and MCF-7, while having much higher IC50 values in the normal VERO cell line.
Overall, the reported studies demonstrate that Cu complexes based on hydrazide-hydrazones exhibit promising in vitro anticancer activity in cell lines of different origins. They sometimes show selectivity towards cancer cells. The studies suggest that the complexes impact the cell’s redox status, disrupt the mitochondria-mediated pathway and kill cancer cells by programmed apoptosis. The use of a 1:1 stoichiometry has been found to be advantageous, likely due to increased uptake compared to complexes with a 2:1 stoichiometry. However, there is still a lack of comparative studies. The presence of co-ligands also appears to enhance the anti-proliferative effect of the complexes, but their prevalence in biological media needs to be evaluated to determine the active species. The cellular uptake of the compound is facilitated by lower polarity and increased lipophilicity, as evidenced for the NN-heterocycle complexes and complexes derived from HH ligands with large aromatic systems. Additional cell mechanistic evaluation and in vivo studies are necessary to fully assess their potential for chemotherapy.
3 Ruthenium complexes
Ruthenium is the metal ion that has been more extensively studied in anticancer research (Lee, Kim and Nam, 2020), following platinum. Its complexes possess favourable characteristics not only for cancer treatment, with molecules with targeting ability being currently developed (Liu, Lai, Xiong, Chen and Chen, 2019), but also for diagnosis and photodynamic therapy (PDT) (Lin, Zhao, Bo, Hao and Wang, 2018).
Ruthenium is a metal cation that typically adopts an octahedral geometry and many developed ruthenium complexes possess co-ligands such as phosphines. Neutrality is often achieved with anions such as chloride or PF6. Cytotoxic ruthenium complexes are found in both +2 and +3 oxidation states. In 2017, Jayanthi et al. (2017) conducted a study to elucidate the impact of the Ru valence by synthesising [RuIII(HL)Cl2(PPh3)2] (Ru-1, Figure 5) and [RuII(L)(CO)(PPh3)2] (Ru-2, Figure 5) complexes, where HL is the thiophene-2-carboxylic acid (2-hydroxyl-benzylidene)-hydrazide ligand. The trivalent complex revealed strong interactions with BSA and DNA and cytotoxicity against breast cancer cell lines MCF-7 and MDA-MB-453, probably due to the presence of a non-coordinated phenol. Both complexes were able to cleave supercoiled DNA, even at low concentrations without any oxidant or reductant. The complexes induced cell death through ROS generation, as confirmed by a significant increase in lactate dehydrogenase and nitric oxide content in the culture medium, leading to the disruption of the cellular membrane.
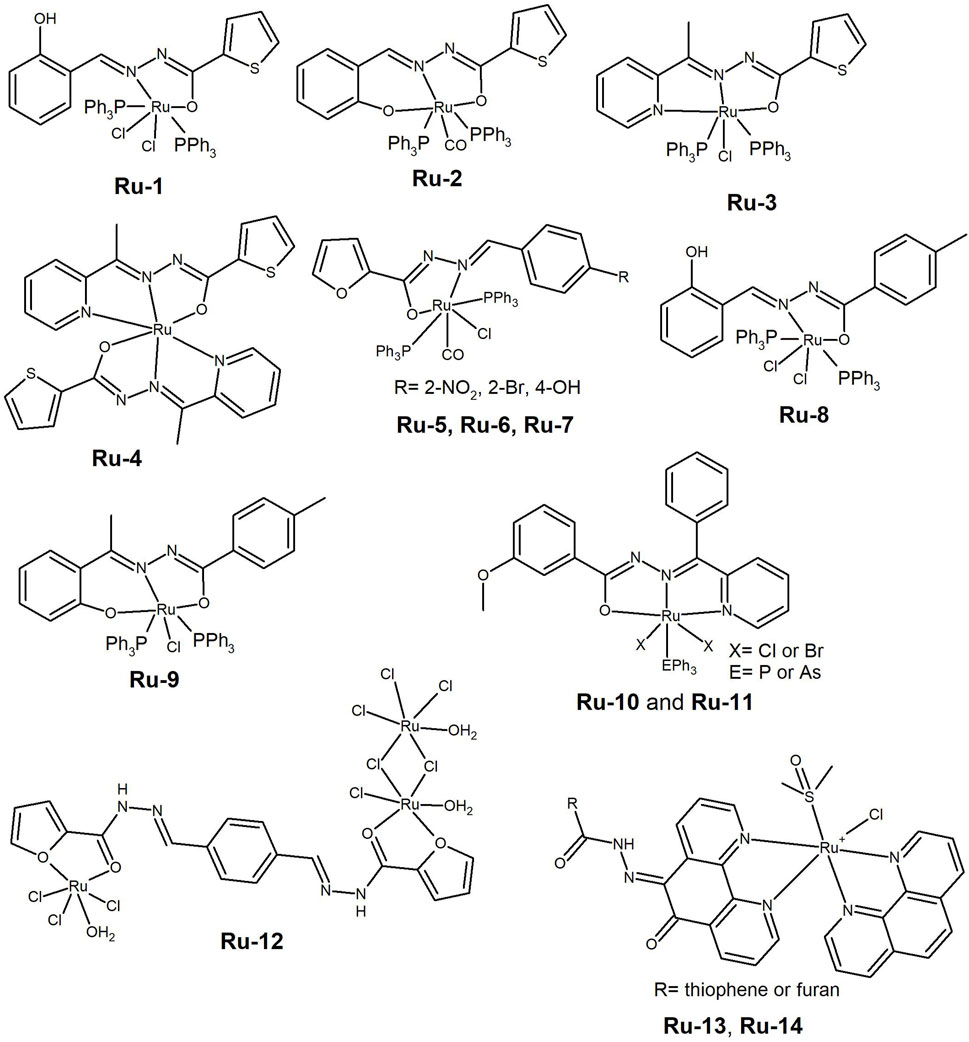
Figure 5. Ruthenium complexes bearing thiophene or furan derived hydrazones (Ru-1 to Ru-7) and salicylaldehyde derivatives with triphenylphosphine and chloride co-ligands (Ru-8 and Ru-9). Ru(III) complexes of (E)-3-methoxy-N'-(phenyl(pyridin-2-yl) methylene) benzohydrazide containing PPh3 (Ru-10) or AsPh3 (Ru-11). Ruthenium(III) complex able to form nano-particles (Ru-12) and ruthenium(II) complexes used as photodynamic therapy agents (Ru-13 and Ru-14).
In addition to oxidation state, another evaluated feature is the stoichiometry of the complexes. In 2019, Eswaran et al. (2019) reported an improved cytotoxic profile for the 2:1 ligand-to-metal ratio, when compared to 1:1, on analogous Ru(II) complexes on cervical (HeLa) and breast (MCF-7) cancer cell lines. The HH ligand used was thiophene-2-carboxylic acid (1-pyridine-2-yl-ethylidene)-hydrazide (Ru-3 and Ru-4, Figure 5). Both complexes have the ability to bind to albumin and DNA, with cleavage ability towards the later. Similar results were disclosed by Mohanraj et al. (2016) for analogous Ru(II) complexes, in which the hydrazide-hydrazone ligands contained a furan moiety and different benzaldehyde derivatives were used instead of pyridine (Ru-5, Ru-6 and Ru-7, Figure 5). The complexes exhibited strong radical-scavenging activity and acted as good DNA cleaving agents. They also showed good anti-proliferative activity against HeLa and MCF-7 cancer cells, with IC50 values in the few tenths of micromolar range. The results indicate that the pyridine derivatives have a better cytotoxic profile than the substituted benzene derivatives (Ru-5, Ru-6 and Ru-7). The additional binding of the pyridine N, probably increases the complex stability.
Paramagnetic Ru(III) complexes containing hydrazone derivatives, Cl and PPh3 (Maikoo et al., 2020) were developed and screened for their cytotoxicity, but showed low potency. Nevertheless, they were found to be good DNA groove binders as shown by Electron Paramagnetic Resonance spectroscopy. In 2015, Jayanthi et al. (2015) synthesised and characterized ruthenium(III) aroylhydrazone complexes [RuCl2(PPh3)2(HL)] (Ru-8, Figure 5), where L= salicylaldehyde p-toluic acid hydrazone and [RuCl(PPh3)2(L′)] (Ru-9, Figure 5), where L’= 2-hydroxy-acetophenone p-toluic acid hydrazone. The complexes bind to BSA and act as DNA intercalators. They showed IC50 values below 15 µM in the A549 cancer cell line, and cell death was most likely induced through a ROS-mediated apoptosis mechanism. The binding ability and cytotoxicity of Ru-8 were higher than those of Ru-9, possibly due to the availability of a non-coordinated but hydrogen-bonded hydroxyl group of the phenolic moiety, which may favour enhanced interactions with biomolecules and thus a better performance. A series of Ru(III) complexes containing (E)-3-methoxy-N'-(phenyl(pyridin-2-yl) methylene) benzohydrazide and either PPh3 (Ru-10, Figure 5) or AsPh3 (Ru-11, Figure 5) were developed (S. Kanchanadevi, Fronczek and Mahalingam, 2021). The complexes were found to interact with albumin and DNA, with the phosphine analogues showing better binding affinity than the arsine ones. The study found that the binding affinity is affected by the nature and size of the co-ligands in the complex. The newly developed Ru(III) complexes demonstrated cytotoxic activity (IC50 10–22 µM) against breast (MCF-7) and lung (A549) cancer cell lines.
Abouzayed and co-workers reported the superior cytotoxicity of a Ru(III) complex of the tetradentate ligand resulting from the condensation of 2-furoic acid hydrazide with terephthaldehyde (Ru-12, Figure 5) compared to complexes with divalent metal ions Co(II), Ni(II), Cu(II), and Pd(II) in the Hep-G2 liver cancer cell line (Abouzayed, Emam and Abouel-Enein, 2020). The authors hypothesized that the higher cytotoxicity and antioxidant activity of the Ru(III) complex could be attributed to its higher lipophilicity and nano-sized particles. However, all compounds presented a poor cytotoxic profile under the analysed conditions.
Polypyridyl ligands with large delocalised aromatic systems have been used to develop metal complexes for photodynamic therapy. In 2021, Silva-Caldeira et al. (2021) synthesised Ru(II) complexes of general formula [RuCl(DMSO)(phen)(L)]PF6 (Ru-13 and Ru-14, Figure 5) in which L is a NN-heterocycle containing a hydrazone. The ligand coordinates the metal ion through the NN-heterocycle, not the HH moiety. The complexes showed moderate cytotoxic activity against leukaemia K562 cells in the dark, that was significantly increased upon exposure to UV-light, with IC50 values decreasing from approximately 50 μM to 18 µM. Therefore, the complexes have potential to be explored as PDT agents. It is worth noting that the presence of phenanthroline moieties did not enhance the DNA interaction, as the complexes only exhibited moderate DNA affinity.
The family of organometallic arene-capped ruthenium complexes is one of the most widely explored for their anticancer activity. Ru-complexes containing hydrazide-hydrazone ligands have been reported, however, the high lipophilicity associated with these complexes has sometimes precluded the in vivo studies necessary to advance to clinical trials. Ruthenium(II) arene benzhydrazones with the general formula [(η6-arene)Ru(L)Cl], where L is a monobasic bidentate substituted 9-anthraldehyde benzhydrazone derivative (Ru-15, Figure 6) were reported (Subarkhan and Ramesh, 2016). The complexes’ were found to be biocompatible based on a haemolysis assay with human erythrocytes. All complexes presented lC50 values in the low micromolar range (<30 µM) against HeLa, MDA-MB-231 and Hep-G2 cancer cells. The analysis revealed that DNA fragmentation and apoptosis were involved in the cell death mechanisms. Almost simultaneously, the same authors (Subarkhan, Ramesh and Liu, 2016) reported similar [(η6-arene)Ru(L)Cl] complexes, where L is a substituted indole-3-carboxaldehyde benzhydrazone derivative (Ru-16, Figure 6), showing anticancer activity in the same cancer cell lines, with slightly lower IC50 values. The Ru-complexes induced apoptosis in the MDA-MB-231 cells, through mitochondria-mediated pathways. This was achieved by upregulating the p53 and Bax proteins, while downregulating the activity of the pro-survival Bcl-2 protein.
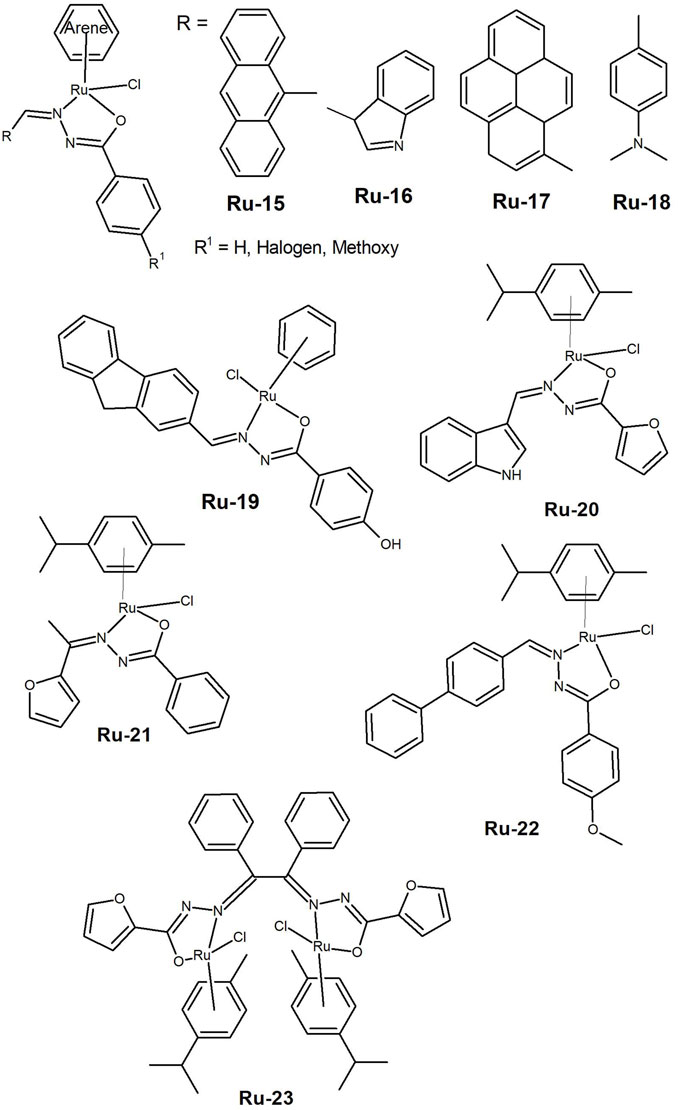
Figure 6. Organometallic arene capped ruthenium(II) complexes bearing hydrazones with different aromatic substituents (Ru-15 to Ru-19) and half-sandwich Ru(II)-p-cymene complexes (Ru-20 to Ru-23).
In 2018 results were presented for Ru(II) complexes of the same type, but with 1-pyrene-carboxaldehyde benzhydrazone derivatives (Ru-17, Figure 6) (Raj Kumar, Ramesh and Małecki, 2018). The authors reported that complexes containing a p-cymene moiety exhibited IC50 values ranging from 12 to 19 µM. Additionally, the unsubstituted p-cymene complex was found to suppress cell growth in lung A549 cancer cells at the G0/G1 phase. Balaji et al. (2020) later presented a series of Ru(II) complexes with the general formula [(η6-benzene)Ru(L)Cl] and [(η6-p-cymene)Ru(L)Cl], where L is a dimethylaminobenzhydrazone (Ru-18, Figure 6). The complexes’ cytotoxicity was tested against A549, LoVo (colon adenocarcinoma), and HuH-7 (hepato cellular carcinoma) cells, as well as noncancerous 16HBE (human lung bronchial epithelium) cells. The IC50 values determined for the complexes were comparable to those of cisplatin and the unsubstituted p-cymene Ru(II) complex showed some selectivity towards cancer cells. The cancer cells underwent apoptosis, and A549 cells were arrested in S phase, which was associated with cytotoxicity.
Recently, a family of ruthenium(II), rhodium(III), and iridium(III) complexes with the general formula [(arene)M(L)Cl] (arene: benzene, p-cymene or Cp* and L a bidentate substituted benzoyl hydrazone)] was synthesized (Sawkmie et al., 2023). The complex [(benzene)Ru(κ2N∩O-L3)Cl] (Ru-19, Figure 6) exhibited an IC50 of 4.99 µM in the HeLa cancer cell line and the authors suggested to continue the cytotoxicity studies.
Half-sandwich Ru(II)-p-cymene complexes were reported by Haribabu et al. (2020) that used a furan-2-carbohydrazide and an indole-3-carboxaldehyde derivative, which showed anticancer activity in the A549 (IC50= 23.4 µM) and HeLa (IC50= 12.9 µM) cancer cell lines (Ru-20, Figure 6). Recently, Małecki’s group (Prabaharan, Rengan, Umapathy, Arockiam and Malecki, 2022) demonstrated good cytotoxicity results for a related family of compounds that also contain furan derivatives (Ru-21, Figure 6). The complexes induced apoptosis and exhibited some selectivity, as evidenced by the higher IC50 values observed for the non-cancerous L132 lung cells. Similar compounds were explored by Arunachalam et al. (2022). The Ru(II)-p-cymene complex with the methoxy-substituted biphenyl benzhydrazone (Ru-22, Figure 6) showed cytotoxicity against A549, MDA-MB-231, and HEPG2 cancer cells. The authors atributed this observation to the hydrophobic nature of the p-cymene moiety and the substituent effects of the ligand. Staining tests indicated that the complex induces apoptosis in cancer cells, as confirmed by ROS generation and mitochondrial membrane potential (MMP) assays. Recently, Monika and Ramesh (Monika and Ramesh, 2023) presented a dinuclear ruthenium(II) complex (Ru-23, Figure 6) that exhibited greater activity in inhibiting the proliferation of the A549 and HeLa cancer cells compared to cisplatin. The study showed cellular death through apoptosis as evidenced by morphological changes in the cells. Given the positive outcomes achieved with ruthenium complexes, it is crucial to develop systems that enable progression to in vivo tests.
4 Platinum and palladium
While platinum-based complexes have been the most successful in the field of anticancer treatment, there has been limited investment in their complexes with hydrazide-hydrazone ligands. Nevertheless, there are some examples in the literature, and promising results have been reported both in vitro and in vivo.
In 2018, (Deng et al., 2018), synthesised tetracoordinated Pt(II) complexes with hydrazones by condensation of 2-hydroxy-1-naphthaldehyde with substituted benzohydrazides. The Pt(II) complexes had the general formula [Pt(Ln)(DMSO)Cl] n= 1–5 (Pt-1 to Pt-5, Figure 7) and showed moderate cytotoxicity against six human tumor cell lines (MGC80-3, SK-OV-3, A549, NCI-H460, HeLa and MDA-MB-231) with IC50 values ranging from 4.38 to 25.16 µM. Cell death occurred by apoptosis, which was triggered either by the inhibition of telomerase activity or by mitochondrial dysfunction due to increased ROS production. The compounds deserve further evaluation as some of these are cell lines of aggressive cancers and selectivity and drug resistance should be assessed.
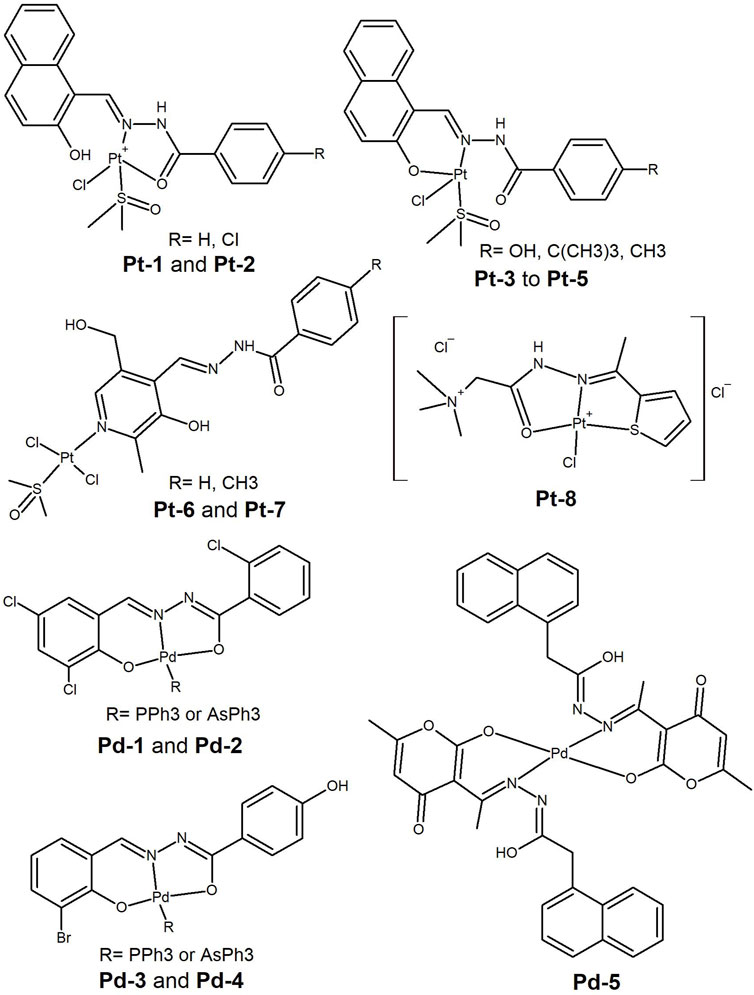
Figure 7. Platinum(II) and Palladium(II) complexes bearing hydrazide-hydrazone ligands having cytotoxicity in different tumoral cell lines.
Pt(II) favours square planar geometries and HH ligands may bind as bidentate, leaving free groups for binding to endogenous metal ions or for intermolecular interactions with targets. Interestingly, Pt(II) complexes with HH ligands derived from vitamin B6 and benzohydrazide derivatives (Pt-6 and Pt-7, Figure 7) were resported (Qi et al., 2022), in which the HH ligand binds as monodentate. The Pt(II) ion binds to the pyridinic-N, while the benzoyl hydrazone leaves a potential ONO donor set free for intracellular metal ion coordination. The complexes showed high cytotoxic activity against lung (A549) and ovarian (SK-OV-3) cancer cell lines (IC50 ca. 1 μM) and presented high efficacy even on cisplatin resistant subtypes. The authors suggest that the high activity of the complexes is due to increased lipid solubility, which leads to lysosomal accumulation and damage. The mechanism of cell death was determined to be apoptosis, as suggested by ROS accumulation and changes in mitochondrial membrane permeability. The authors also reported an in vivo study that demonstrated a high inhibitory effect (81.5%) for one of the Pt(II) complexes on A549 xenograft tumors in nude mice, without affecting the animals’ body weight. These are promising compounds as multidrug resistance is one of the biggest challenges in cancer research.
Recently, platinum(II), palladium(II), gold(III), and ruthenium(III) complexes bearing new Girard-T reagent ligands were synthesised (Al-Radadi and El-Gamil, 2023). The complexes presented either square planar or octahedral geometries around the metal cations. The Pt(II) complex with a thiophene moiety (Pt-8, Figure 7) showed a comparable IC50 value (1.66 µM) to the reference drug, 5-fluorouracil, in the MCF-7 cancer cell line.
Alongside platinum, palladium complexes are strong candidates for the development of anticancer drugs. Two Pd(II) complexes bearing 2-chloro-benzoic acid (3,5-dichloro-2-hydroxy-benzylidene)-hydrazide ligand and triphenyl phosphine (Pd-1, Figure 7) or triphenyl arsine (Pd-2, Figure 7) as co-ligands were reported (G. Ayyannan et al., 2020). The complexes bind BSA and act as ctDNA intercalators. In addition, the complexes displayed moderate cytotoxicity (IC50 > 20 µM) against HeLa and MCF-7 cancer cell lines. The phosphine complex exhibited better efficacy than the arsine-containing complex. Similar conclusions were previously reported by the authors in a study done with Pd(II) complexes Pd-3 and Pd-4 (Figure 7), bearing 4-hydoxy-benzoic acid (5-bromo-2-hydroxy-benzylidene)-hydrazide ligand (Ayyannan et al., 2016). The IC50 values for these complexes were higher in the same cell lines, but in the same concentration range and again higher than for cisplatin.
Encapsulation of the metal complexes in micelles is one strategy used to improve bioavailability. A series of six transition metal complexes (Co(II), Ni(II), Cu(II), Zn(II), Pd(II) and Cd(II)) with a 2-(naphthalene-1-yl) acetic acid hydrazone ligand were reported by Ahmad et al. (2021) The complexes were enclosed on a cetyltrimethylammonium bromide ionic surfactant, in high micellar concentration. The Pd(II) complex (Pd-5, Figure 7) was the best performing metal complex, showing cytotoxicity against HeLa cervical cancer cells at 30 µM concentration, comparable to the reference drug Doxorubicin (showing 97% growth inhibition).
Overall, the development of platinum and palladium complexes of HH ligands is in its early stages, leaving ample room for further exploration.
5 Vanadium complexes
One element that has received considerable attention since its biological activity was first recognized is vanadium, which also exhibits a rich chemistry in aqueous environments (Costa Pessoa, 2015; Rehder, 2015). Therefore, its complexes have been the subject of intensive research in the anticancer field (Kioseoglou, Petanidis, Gabriel and Salifoglou, 2015). Vanadium has several oxidation states, but in biological systems, it is mainly found in the +4 or +5 valence.
In 2014, Nair et al. (2014) reported the anticancer properties and apoptose-inducing ability of two oxidovanadium(IV) complexes with benzoylpyridine nicotinoyl hydrazone (V-1, Figure 8) and 2-hydroxy-4-methoxybenzaldehyde nicotinoyl hydrazone (V-2, Figure 8), in human papillomavirus (HPV) positive cervical cancer cell lines, HeLa and SiHa. The complexes induced DNA condensation and loss of mitochondrial membrane potential, leading to apoptosis. Complex V-1 showed anticancer activity in HPV-positive SiHa cells (IC50= 50 µM) by increasing the p53 expression. The cytosolic p53 probably interacts with pro-apoptotic mitochondrial BAK proteins leading to cell death. Although the authors presented a number of interesting mechanistic cell studies, that included gene expression, the need for such a high concentration for the anti-proliferative effect requires further in vivo safety studies to fully evaluate the potential of the V-complex for the treatment of HPV-positive cervical cancers.
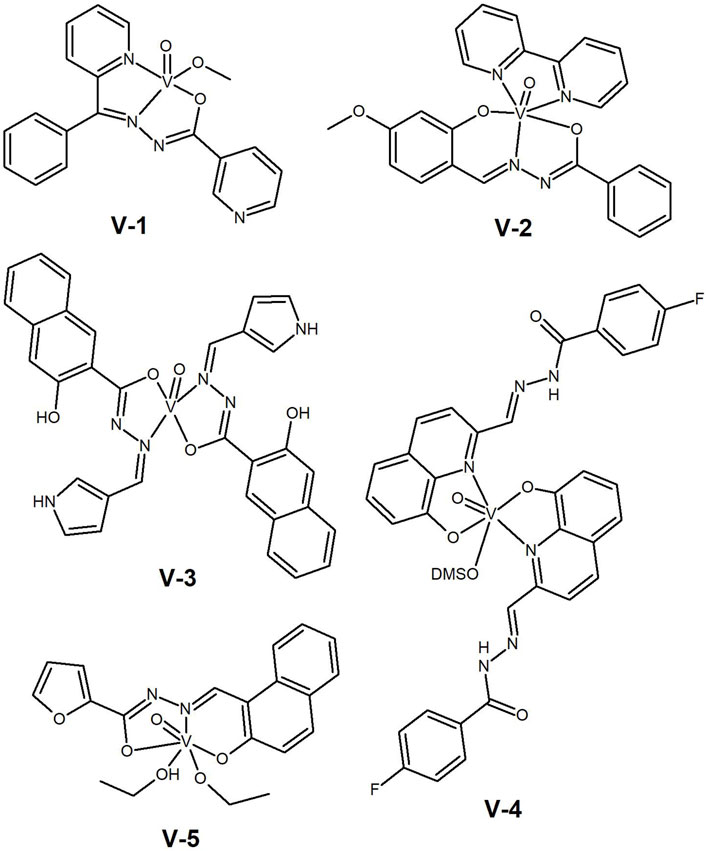
Figure 8. Vanadium(IV) (V1–V4) and vanadium(V) (V-5) with aromatic hydrazide-hydrazones tested as anti-cancer agents.
However, it is not always possible to identify this correlation with p53 expression. In 2019, our group (Ribeiro et al., 2019) reported on a series of V(IV)O, Cu(II) and Zn(II) complexes of naphthoylhydrazones bearing pyrrole, furan and thiophene moieties. IC50 values against p53 wild-type and p53 depleted colon cancer cells (HCT-116) were found to be similar. All complexes interacted with BSA, mainly through π–π stacking as disclosed by docking studies, demonstrating their ability to be transported by this protein. Although the complexes lacked cancer cells selectivity, the V(IV)O complex with the pyrrole derivative (V-3, Figure 8) showed promising results in lung (H1299, IC50= 7.0 µM), breast (MCF-7, IC50= 0.73 µM) and colon (HCT-116, IC50= 1.12 µM) cancer cells. These are relevant results and strategies to overcome the low selectivity are being developed.
More recently, our group reported a study on the solution speciation of vanadium(IV) complexes bearing HH-ligands with an 8-hydoxyquinoline moiety (Ribeiro et al., 2022). Interestingly, different stoichiometries and coordination modes were found for these ligands. The V(IV)O complex bearing two F-substituted ligands (V-4, Figure 8) showed the best anti-proliferative results against the human melanoma A375 cell line (IC50= 2.4 µM). The cell death mechanism involves activation of caspase 3/7 and apoptosis.
The use of hydrazide-hydrazones containing different types of heterocycles had already been reported by Dash et al. in 2015, but for vanadium(V) complexes (Dash et al., 2015). The reported ligands were quite different from each other, containing either furan, thiophene or pyridine residues and sharing only the HH functionality. The V(V) complexes interacted with DNA via minor groove binding and cleaved this biomolecule in a photolytic process involving singlet oxygen and hydroxyl radicals. The cytotoxicity of the complexes was evaluated against HeLa cancer cells and the results compared well with standard drugs. The highest cytotoxicity (IC50 ca. 10 μM) was found for the furan-containing V(V) complex (V-5, Figure 8), which the authors correlated with the destabilizing effect on DNA.
The rich chemistry of vanadium allows the formation of multinuclear complexes that may contain bridging (µ–) elements. This is the case of the complexes reported by Patra et al. (2017), with the general formula [VV2O3(HL)2], where the ligands correspond to the dianionic form of 2-hydroxybenzoylhydrazones of acetylacetone (V-6, Figure 9) and benzoylacetone (V-7, Figure 9). The complexes presented anticancer activity against SiHa cervical cancer cells, with IC50 values <30 μM, inducing apoptosis, and acting as DNA intercalators.
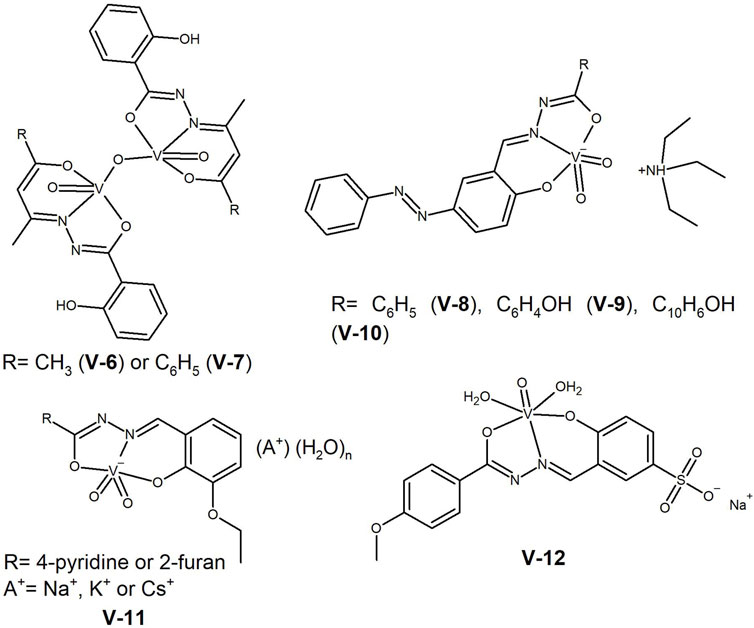
Figure 9. Oxidovanadium(V) complexes with a µ-O bridge between the two metal centres (V-6 and V-7), dioxido-vanadium(V) complexes (V-8 to V-11) and water-soluble vanadium(V) complex (V-12) with anti-cancer potential.
In 2019 Mohanty et al. (2019) reported on the synthesis of dioxidovanadium(V) complexes, with the general formula (HNEt3)[VO2(L)]. The ligands L are condensation products of 5-(arylazo)-salicylaldehyde with benzoic hydrazide (V-8, Figure 9), salicylic hydrazide (V-9, Figure 9) and 3-hydroxy-2-naphthoic hydrazide (V-10, Figure 9). The complexes exhibited IC50 values between 4.7 and 7.48 µM in the human cervical (HeLa) and human colon (HT-29) cancer cell lines, and a 10-fold higher value in the non-cancerous human epidermal keratinocyte cells (HaCaT). Confocal microscopy analysis revealed the shrunken morphology of HT-29 cells with condensed chromatin bodies, nuclear blebbing and fragmentation, indicative of apoptosis.
With the aim of synthetisizing water-soluble compounds, Sahu et al. (2021) reported the synthesis of five anionic dioxidovanadium(V) complexes, [VO2(L)A(H2O)n] (V-11, Figure 9), with pyridine-4-carboxylic acid (3-ethoxy-2-hydroxybenzylidene) hydrazide and furan-2-carboxylic acid (3-ethoxy-2-hydroxybenzylidene) hydrazide ligands, using different alkali metals (A = Na+, K+, Cs+) as counterions. The authors reported that an optimal hydrophobicity is essential for high cytotoxicity. The complexes were more cytotoxic against colon (HT-29), breast (MCF-7), and cervical (HeLa) cancer cell lines (IC50 range 5–40 µM) than against the non-cancerous NIH-3T3 cells, showing some selectivity towards cancer cells. They interacted with BSA and DNA and showed potential as antimetastatic agents against HT-29 cells.
One strategy to improve the aqueous solubility of oxidovanadium(IV) complexes, can be the use of hydrophilic substituents. This was followed by Adam et al. in their 4-hydroxy-3-((2-(4-methoxybenzoyl)hydrazineylidene)methyl) benzene sodium sulfonate ligand (Adam, Shaaban, Khalifa, Alhasani and El-Metwaly, 2021). The corresponding [VO(L)]·½H2O (V-12, Figure 9) interacted with ctDNA and showed promising results in in vitro anticancer assays. The complex exhibited IC50 values in the colon (HCT-116), breast (MCF-7) and liver (Hep-G2) cancer cell lines comparable to the reference drug, vinblastine, in the 10–20 µM range.
A limited number of interesting studies have been reported with this versatile metal ion, but it is clear that the field is still underexplored.
6 Metal complexes with other d-block ions
Researchers engaged in the discovery of new anticancer metallodrugs can choose from many elements in the d-block of the Periodic Table and several complexes of these elements can be found in the literature.
Dioxido complexes are common with molybdenum(VI) and in 2021, Vineetha et al. (2021) presented the synthesis of new dioxidomolybdenum(VI) complexes containing 3-ethoxysalicylaldehyde benzoylhydrazone (Mo-1, Figure 10). The complexes acted as DNA intercalators and cleaved supercoiled DNA to its nicked form, acting as nucleases. They exhibited low cytotoxicity against breast MCF-7 cancer cells (IC50 ca. 70 μM), which the authors attributed to DNA damage and apoptosis, but both lack corroboration.
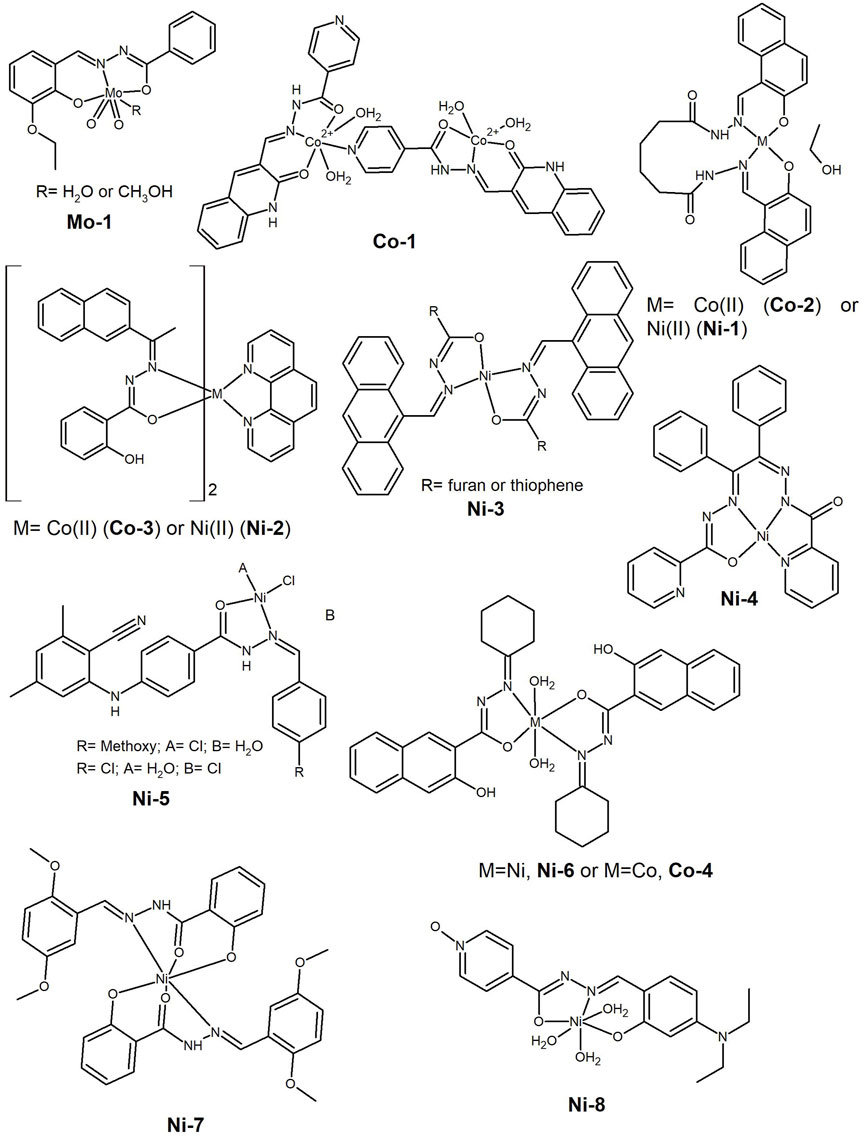
Figure 10. Dioxidomolybdenum(VI) complex presenting cytotoxicity against breast MCF-7 cancer cells (Mo-1). Cobalt(II) and nickel(II) complexes tested as anti-cancer agents against several cancer cell lines.
The researchers’ attention is often focused on the solubility issue and early in the last decade, Raja et al. (2012) synthesized a new water-soluble ligand-bridged cobalt(II) coordination polymer (Co-1, Figure 10) by reacting the ligand, 2-oxo-1,2-dihydroquinoline-3-carbaldehyde (isonicotinic) hydrazone with [Co(NO3)2]·6H2O. Notably, the complex was found to be not only water-soluble but also stable in this medium, with the polymeric composition most likely to be the active species in the biological activities. In addition to its radical scavenging activity, the complex binds BSA and acts as ctDNA intercalator. The complex shows anticancer activity in HeLa, Hep G2, human laryngeal epithelial carcinoma cells (HEp-2) and human skin cancer cells (A431).
Often papers report on complexes of different metal ions and attempt to establish a correlation with their cytotoxic activity. Generally, when Cu(II) is tested, its complexes show the best results, as observed in the literature cited in Section 2, but this is not always the case. In 2017, the synthesis of Mn(II), Co(II), Ni(II) and Cu(II) complexes with N1,N6-bis((2-hydroxynaphthalin-1-yl)methinyl))adipohydrazone, acting as dibasic tetradentate ligand were reported (Gaber, Khedr and Elsharkawy, 2017). Overall, the complexes exhibited potent anticancer activity against the Hep-G2 cell line (IC50 <15 µM) and the activity increased in the order Cu(II) < Mn(II) < Co(II) (Co-2, Figure 10) < Ni(II) (Ni-1, Figure 10). In fact, these two elements, Co(II) and Ni(II), appear together in some works, and in the same year, Li et al. (2017) presented the synthesis of two 1,10-phenanthroline (phen) ternary complexes, [Ni(L)2(phen)]·CH3CN (Ni-2, Figure 10) and [Co(L)2(phen)]·CH3CN (Co-3, Figure 10), where L is the monobasic form of 2-acetonaphthone salicylhydrazone. The complexes bind BSA and act as intercalators with herring sperm DNA, in addition to possessing radical scavenging activity. The authors suggest that the DNA/BSA binding activities, as well as the cytotoxicity, can be tuned by changing the ligands and the geometry in these nickel and cobalt systems, highlighting the important role of the synergistic planarity of the aroylhydrazone and the diimine co-ligand, as well as the cationic nature of the complex in the biological activities. Nevertheless, the in vitro cytotoxic evaluation of the complexes showed that Ni-2 has only a moderate ability to inhibit the growth of HeLa (IC50= 35 µM) and A549 (IC50= 29 µM) tumor cells, however, without significantly affecting non-cancerous NIH-3T3 cells.
Indeed, in recent years there has been increasing interest in Ni(II) complexes for anticancer applications, and the hydrazide-hydrazone ligands have good chelating properties for this metal centre. Complexes have been reported as promising agents, some comparable to their Cu(II) analogues, as reported by Neethu et al. (2021) for a series of Ni(II) (Ni-3, Figure 10) and Cu(II) complexes bearing anthracenyl hydrazone ligands. The complexes showed anti-proliferative activity in the colon HCT-15 cancer cells and negligible toxicity towards non-cancerous L929 skin cells, thus demonstrating selectivity. The Cu(II) complexes proved to be only slightly more cytotoxic than the Ni(II) and all exhibited IC50 values in the range of 14–28 µM. Flow cytometric analysis showed that they induced apoptotic cell death through a ROS-dependent mechanism.
In 2020, Ay et al. (2020) reported the synthesis and characterization of a mononuclear Ni(II) complex with a picolinohydrazide derivative (Ni-4, Figure 10), which showed a low IC50 value on the A549 lung cancer cell line (4 µM). The cell death mechanism proceeds via apoptosis, with a decrease in the expression of Snail2 and an increase in E-cadherin, important biomolecules in the metastatic process (Hu et al., 2019). A wound-healing assay also showed the ability of the complex to inhibit cell motility, indicating the potential of this complex for an anti-metastatic application.
A series of square-planar Ni(II) complexes bearing N′-arylidene-4-((3-cyano-4,6-dimethylpyridine-2-yl)amino)-benzohydrazide derivatives in which the benzaldehyde has different substituents at the para position, were reported in the same year (Abumelha et al., 2020). An extensive molecular operating environment (MOE) docking simulation was carried out to evaluate the inhibition of the 3s7s protein, an important aromatase in breast cancer. The computational studies identified the methoxy, chloride and nitro substituted compounds as the most potent inhibitors. The in vitro anticancer screening against the MCF-7 breast cancer cell line showed higher potency for the methoxy and chloride substituted complexes (Ni-5, Figure 10). This study highlighted docking simulations as an important tool in predicting the compounds’ activity. With the accelerated development of technology, an increase in computational studies is also expected in this area. Some of the authors were involved in a similar study but using a green ball milling strategy to prepare 2:1 ligand-to-metal octahedral Cd(II) and Zn(II) complexes bearing 2 N′-benzylidene-3-hydroxy-2-naphthohydrazide ligands. The ligand and respective complexes showed activity against various microbes, free radicals and the carcinoma cell line HCT-116 (Alzahrani et al., 2020). In fact, the following year, the same MOE docking strategy was used to evaluate the Ni(II), Co(II) and Cu(II) complexes of the new N′-cyclohexylidene-3-hydroxy-2-naphthohydrazide ligand with the general formula [M(HL)2] (Alkhamis et al., 2021). The Ni(II) complex (Ni-6, Figure 10) showed excellent antioxidant activity against the liver cancer cell line (Hep-G2), while the Co(II) complex (Co-4, Figure 10) showed cytotoxic activity comparable to the reference drug 5-fluorouracil (5-FU).
Recently, the synthesis of (E)-N’-(2,5-dimethoxybenzylidene)-2-hydroxybenzohydrazide and its Co(II), Ni(II) and Zn(II) metal complexes was reported (Patil, Javarappa, Kumar and Naik, 2022). The Ni(II) complex (Ni-7, Figure 10) showed antiproliferative activity against MCF-7 and HeLa cancer cells (IC50 > 10 μM). In 2023 the quantitative structure activity relationship (QSAR) and molecular orbital properties of Cu(II), Ni(II) and Zn(II) complexes of a new isonicotinoyl N-oxide hydrazone were reported (Anitha et al., 2023). The authors used the in vitro CTG method to investigate the antiproliferative activity of the complexes against MCF-7 cancer cells, with the Ni(II) complex (Ni-8, Figure 10) exhibiting an IC50 value of 1.07 µM. So, clearly Ni(II) complexes of HHs deserve further investigation and development.
Hydrazide-hydrazones are also good chelators for the endogenous iron cation, and a few complexes of this element have shown anticancer activity. Stevanović et al. (2021) while designing a new series of metal complexes of Girard’s reagents hydrazones, showed the antiproliferative effect of the isothiocyanato Fe(III) complex with (E)-N,N,N-trimethyl-2-oxo-2-(2-(1-(pyridin-2-yl)ethylidene)hydrazinyl)ethan-1-aminium chloride, [Fe(L)(NCS)3] (Fe-1, Figure 11), in the cervical cancer (HeLa, IC50= 37.63 µM) and melanoma (A375, IC50= 22.98 µM) cell lines. Fe-1 arrested the HeLa cell cycle in the subG1 phase, and it also showed a protective effect against the generation of oxidative stress induced by hydrogen peroxide in the normal keratinocyte cell line HaCaT.
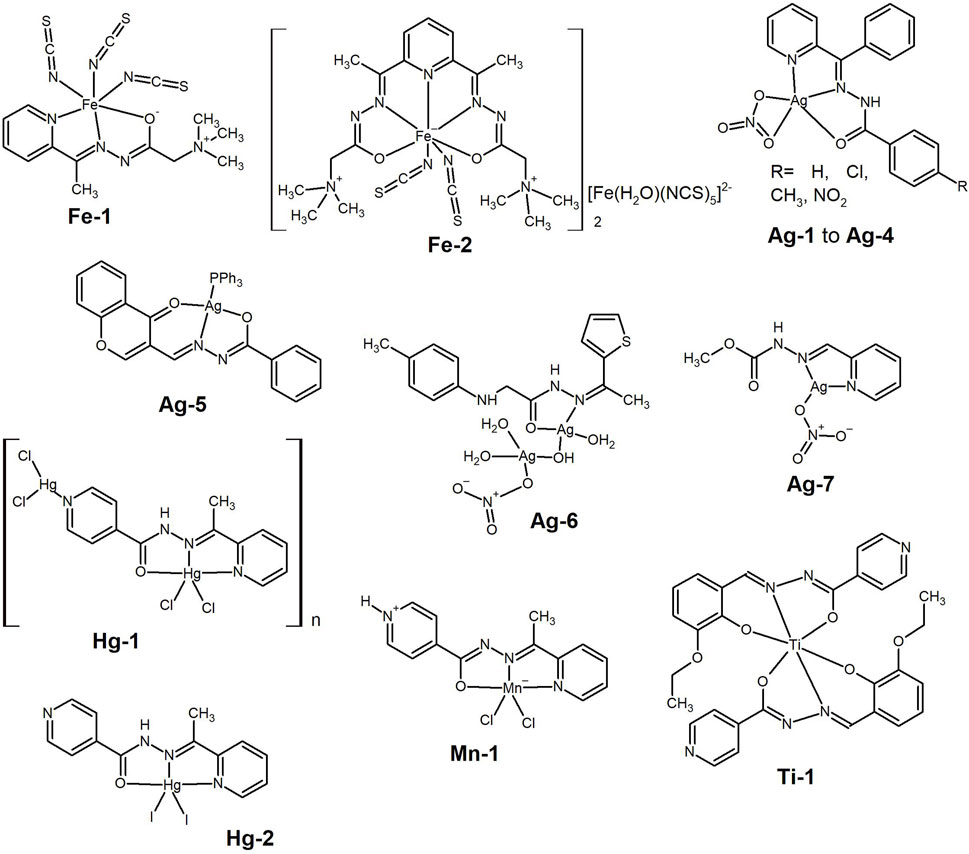
Figure 11. Iron(III) complexes bearing hydrazide-hydrazone and isothiocyanato ligands presenting anti-cancer activity. Silver (I) complexes bearing hydrazide-hydrazone studied for the anti-cancer activity. Mercury(II) and manganese(II) complexes bearing pyridine-hydrazide-hydrazone and halogen ligands, which were tested as anti-cancer agents. Titanium(IV) hydrazone complex tested against HeLa cancer cell line.
Anđelković et al. (2017) reported on Fe(III)-complexes built from 2,2′-[2,6-pyridinediylbis(ethylidyne-1-hydrazinyl-2-ylidene)]bis[N,N,N-trimethyl-2-oxoethanaminium] dichloride. They reported a superior cytotoxic activity of the positively charged complex when the counterion was [Fe(H2O)(NCS)5]2− (Fe-2, Figure 11), compared to that with only the free isothiocyanate anion. Further studies are needed to understand the mechanism of action of these Fe(III)-complexes.
A few studies have also demonstrated the anticancer potential of silver(I) complexes of hydrazide hydrazones, and Santos et al. (2018) synthesised complexes with general the general formula [Ag(L)(NO3)], where L are 2-benzoylpyridine hydrazones (Ag-1 to Ag-4, Figure 11). The complexes interact with HSA and DNA, although covalent binding of silver(I) to DNA is unlikely to occur. The complexes are cytotoxic to B16F10 metastatic murine melanoma cells (IC50 ca. 2 μM), with higher concentrations required to kill non-cancerous murine Melan-A melanocyte cells, demonstrating the selectivity of the complexes towards cancer cells.
A series of metal complexes derived from 3-formylchromone and benzohydrazide were prepared by Elsayed et al. (2015). The Ag(I) complex containing PPh3 as co-ligand (Ag-5, Figure 11) showed higher cytotoxicity than cisplatin in the human breast cancer (MDA-MB-231) and ovarian cancer (OVCAR-8) cell lines and much higher than all other complexes [Ni(II), Zn(II), Pd(II) and Pt(II)], with IC50 values of ca. 1 μM.
Recently, a new family of metal complexes with the ligand N-(1-(thiophene-2-yl)ethylidene)-2-(p-tolylamino)acetohydrazide were reported (Hassan, Rizk, Khidr and Aly, 2024). The Co(II), Zn(II) and Ru(III) complexes are mononuclear, whereas the Ag(I) complex (Ag-6, Figure 11) is proposed to be dinuclear. Among the tested compounds, complex Ag-6 showed the highest anti-proliferative activity against MCF-7 cancer cell line, with IC50= 98 μM, which is too high to be considered relevant. Ganesh Babu’s group also showed that a simple Ag(I) complex (Ag-7, Figure 11) exhibited anti-proliferative activity against the MCF-7 cancer cell line (IC50= 57.45 µM) (Elankathirselvan, Paunkumar, Srinivasan, Premkumar and Babu, 2024), but again this is a quite high value and the study focused mostly on the synthesis of Ag-nanoparticles.
There are even some examples in the literature of metal complexes of elements traditionally associated with general toxicity, such as some cadmium complexes mentioned above and those presented by Demir et al. (2021) derived from mercury(II) and manganese(II). The synthesis of Hg(II) (Hg-1 and Hg-2, Figure 11) and Mn(II) (Mn-1, Figure 11) pincer-type complexes with N′-(1-(pyridin-2-yl)ethylidene) isonicotinohydrazide ligand was reported. The neutral ligand coordinates the metal cations in different tautomeric forms, presenting a tridentate coordination mode through the 2-pyridyl and imine nitrogen atoms, and the carbonyl oxygen atom. Interestingly the most active compounds are the Hg(II)-complexes with IC50 values in the range 2.56–9.90 μM μM against the Huh7 cell line. The Mn(II)-complex is not cytotoxic towards all the three cell lines with relatively high IC50 values. The Hg-complexes inhibit cell growth and induce apoptosis by interacting with DNA and inducing p53 expression. Given the known toxicity of Hg, which easily binds to sulphur in proteins, the complex stability should be evaluated in biological media prior to further studies related with in vivo toxicity.
Late last year, Rupam Dinda’s group showed that Ti(IV)-hydrazone complexes are hydrolytically stable and tested them as anticancer agents (Sahu et al., 2023) against HeLa, A549 and NIH-3T3 cell lines. Among the three complexes the pyridine derivative (Ti-1, Figure 11) showed the best anti-proliferative activity against the HeLa cancer cell line, with IC50 = 11.6 µM. Confocal microscopy and flow cytometry studies indicated apoptosis as the mode of cell death.
These studies highlight the variety of metal cations being used in the development of hydrazone-hydrazide complexes for cancer therapy, with some showing relevant in vitro results.
7 Lanthanide complexes
The elements of the lanthanide series have also been investigated in the development of new metallodrugs. Lanthanides typically have coordination numbers higher than 6, and are therefore, capable of accommodating multiple ligand molecules within their sphere. In the middle of the last decade, Xu et al. (2015) reported the synthesis and characterization of [Ce(L)2(H2O)4](NO3)3·4H2O (Ln-1, Figure 12) and [Sm(L)2(NO3)2](NO3)·C2H5OH (Ln-2, Figure 12), where L is the 2-acetylpyridine nicotinohydrazone ligand. The complexes showed good cytotoxic results against human pancreatic (PATU8988), colorectal (LoVo) and gastric (SGC7901) cancer cell lines (IC50 values ranging from 9 to 57 µM), with both complexes showing lower IC50 values in the latter cancer cell line and also lower than that measured for the free ligand. The complexes induced apoptosis and morphological changes were observed in the tested cancer cells. A few years later, Xie and co-workers (Xie et al., 2017) published the synthesis of Ln(III) complexes of the type [Ln(HL)2(NO3)(CH3OH)2]·CH3OH (Ln-3, Figure 12), where (HL)= (E)-N’-[1-(2-pyridinyl)ethylidene] isonicotinohydrazone. The La and Ce exhibited significant cytotoxic activity against three human cancer cell lines (lung, A549—IC50 = 11.5 µM—and gastric, BCG823—IC50 = 13.3 µM—and SGC7901—IC50 = 15.6 µM). Analysis suggested that the Ln(III) complexes could induce apoptosis in A549 cells by increasing the expression levels of the pro-apoptotic proteins caspase-3 and Bax, while decreasing the expression levels of the anti-apoptotic protein Bcl-2. The ability to regulate the expression levels of these proteins seems to be closely related to the mechanism of action of complexes containing a lanthanide element, but this has also been also shown for Cu(II)-complexes (see Section 2).
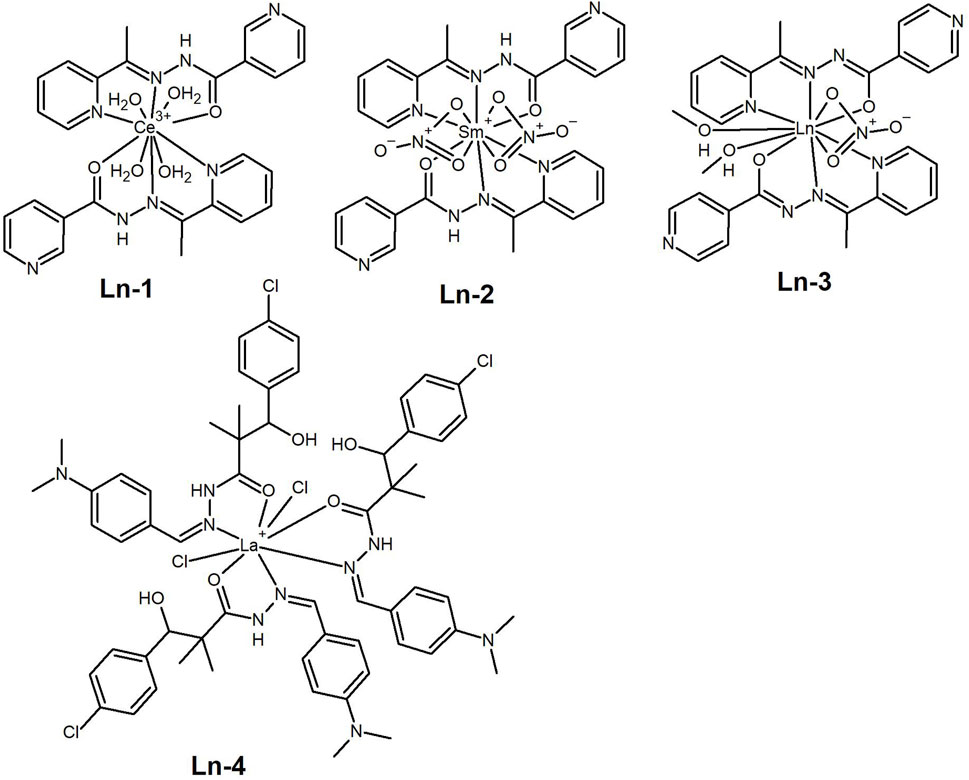
Figure 12. Complexes of metal ions belonging to the f-block using hydrazide-hydrazone ligands tested as anti-cancer agents presenting cytotoxicity.
A complex with the formula [La(HL)3Cl2]·Cl·7H2O (Ln-4, Figure 12), where HL represents the new 3-(4-chlorophenyl)-N’-(4-(dimethylamino)benzylidene)-3-hydroxy-2,2-dimethylpropanehydrazide ligand was recently reported (Aboelmagd et al., 2021) in a study that also included Cu(II) and Ni(II) complexes. This complex, as well as the others, showed selectivity towards human colon cancer cells HCT-116 when compared to the non-cancerous HEK-293 cells. However, the IC50 value determined for Ln-4, despite being the lowest in the series, is quite high (0.154 mM). Based on docking calculations obtained with other compounds in the study, the authors propose a mechanism of action by inhibition of CDK8-CYCC kinase (an important protein in cell cycle progression), but this clearly lacks experimental corroboration.
Overall, the number of studies reported for lanthanide complexes are quite limited and given the high coordination numbers of lanthanides and the high chelating ability of HH ligands, these should be further explored.
8 Gallium and tin
Some elements outside the d-block of the Periodic Table have also attracted the interest of researchers in the field of anticancer research. For example, Firmino et al. (2019) reported on the synthesis of gallium(III) complexes with 2-acetylpyridine (Ga-1, Figure 13) and 2-pyridineformamide (Ga-2, Figure 13) isonicotinoyl hydrazones. The hypothesis behind the use of gallium is that Ga(III) complexes can be used as Fe(III) mimics since the cancer cells have high iron requirement due to their rapid proliferation. The hydrazones and their Ga(III) complexes were tested against MCF-7, HL-60 (leukaemia), HCT-116 (colon cancer) and PC3 (prostate cancer) tumor cell lines and non-malignant human embryonic kidney 293 (HEK-293) cells. The Ga-1 complex showed high toxicity against HCT-116 cells (IC50= 0.4 µM) but low toxicity in the HEK-293 cell line. This complex showed pro-apoptotic potential and the ability to inhibit the cell cycle by increasing subdiploid DNA content.
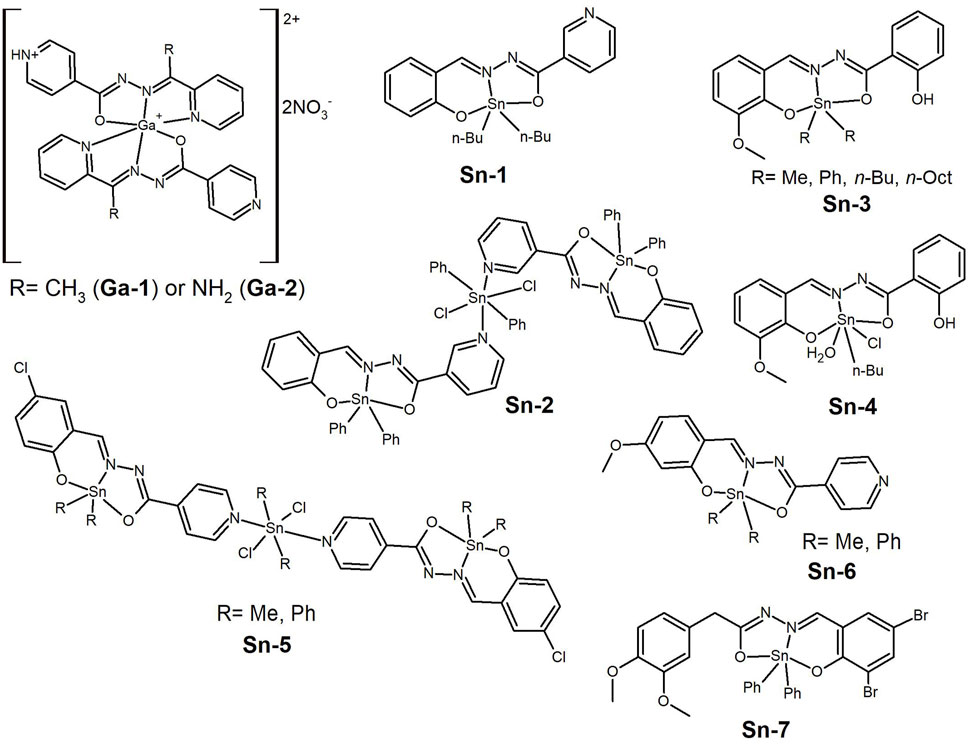
Figure 13. Gallium(III) complexes tested in several cancer cell lines, showing high toxicity against colorectal carcinoma. Organotin(IV) complexes tested as anti-cancer agents, which were able to bind BSA.
Another element that has been explored in this context is tin(IV). The synthesis of two organotin(IV) compounds from salicylaldehyde nicotinoyl hydrazone and the corresponding dialkyltin(IV) precursor (Sn-1 and Sn-2, Figure 13) were reported by Xu et al. (2016). The complexes bind to BSA, and show in vitro cytotoxicity against three human cisplatin-resistant tumor cell lines (lung, cervical and breast) with very high activity (IC50 values ranging from 0.85 to 2.41 µM). Almost simultaneously, Hong et al. (2016) reported the synthesis of five new mono- or di-organotin(IV) complexes with 2-hydroxy-N’-[(2-hydroxy-3-methoxyphenyl) methylidene]-benzohydrazone ligand. The ligand binds the tin cation as an ONO tridentate chelate and while the di-organotin(IV) complexes have the general formula [(organo)2Sn(L)] (Sn-3, Figure 13), the n-Bu forms a complex with the formula [(n-Bu)SnCl(H2O)(L)(CH3CH2OH)] (Sn-4, Figure 13). The complexes bind to BSA and act as ctDNA intercalators. In vitro cytotoxicity screening showed that all compounds exhibited good activity against three cisplatin-resistant human cancer cell lines: A549cisR, HeLacisR and MCF-7cisR cell lines, with IC50 values as low as 0.4 µM. This is significant both because of the submicromolar IC50 values and the activity against cisplatin-resistant cancer cells.
Later (Liu et al., 2017), the group reported four organotin(IV) complexes, this time having 5-chlorosalicylaldehyde-isonicotinoyl hydrazone (Sn-5, Figure 13) and 2-hydroxy-4-methoxybenzaldehyde-isonicotinoyl hydrazone (Sn-6, Figure 13) ligands. The complexes interacted with the BSA and DNA biomolecules in the same way as the previous ones. All of them showed cytotoxicity against lung A549 (IC50 1.53–10.50 µM) and cervical HeLa (IC50 1.25–15.16 µM) cancer cell lines, with the mononuclear diphenyl complex being the most potent against HeLa cancer cells (Sn-6, with R= Phenyl, Figure 13).
The importance of increased aromaticity and consequent lipophilicity in the anti-proliferative activity was again demonstrated by the group of Jai Devi (Kumar et al., 2023). The authors reported a series of diorganotin(IV) complexes with halogenated hydrazones, with the di-bromo derivative showing the best anti-proliferative results in the A549, MCF-7 and HCT-116 cancer cell lines tested. The performance of the diphenyltin(IV) complex (Sn-7, Figure 13) was comparable to that of the reference drug Paclitaxel, with an IC50 value of around 4 µM. So, tin is clearly an element to take into account in the development of new chemotherapeutic-based HH ligands.
9 Conclusion and future remarks
Despite recent progress, cancer remains the second leading cause of death worldwide. Given the severe side effects and acquired resistance of several tumors to the current chemotherapeutic regimens, there is an urgent need for further development and research into alternative mechanisms for targeting and killing cancer cells. This has led bioinorganic researchers to develop new metallodrugs with different properties, aiming at higher efficacy and selectivity towards different types of cancer.
Hydrazide-hydrazones can be readily obtained by condensation of commercially abundant hydrazides and aldehydes or ketones, providing a huge library of different molecules to screen. Although this review has not focused on their structural versatility, by having different functionalities and tautomers, HHs bind metal ions with different chelating groups and denticity, yielding complexes with different properties and biological effects. Structure–activity relationships based on systematic studies are lacking and preclude the establishment of improved drug design.
In general, it is observed that the metal complexes show higher anticancer activity than the corresponding ligands, which are usually also bioactive. Copper is the metal ion that has been most extensively explored in the context of coordination to HH molecules, but many other metal cations have been used, from transition to late transition metals and lanthanides, with very interesting results. Most studies report in vitro cytotoxicity studies on human lung adenocarcinoma (A549), human cervical cancer (HeLa) and human breast cancer (MCF-7 and MDA-MB-231) cell lines, which represent cancer types with high incidence or mortality rates. Authors typically do not compare their systems with others published in the literature, which prevents further advances in the field. Additionally, the lipophilic/hydrophilic balance affects the solubility in biological media and the drug uptake, and this should be further investigated to obtain correlations that will allow the design of compounds with more suitable properties. Alternatively, the development of carrier systems with properties that can circumvent this aspect should be considered. Another important issue is that few studies evaluate the speciation in aqueous solution under physiological conditions to determine the active species. In addition, many studies report only a preliminary screening with IC50 values and no evaluation of the mechanism of action or biological targets. However, and in general, most studies with hydrazide-hydrazone metal complexes seem to point to the involvement of ROS and apoptosis as the mode of cell death. Downregulation of the pro-survival Bcl-2 and upregulation of the caspase and BAX protein families have been demonstrated in several studies. However, there is a lack of in vivo studies that are essential to select hits for drug development.
Overall, hydrazide-hydrazones are a very interesting and versatile ligand system that deserves further development, as they and their metal complexes show high potential for the development of new anticancer agents.
Author contributions
NR: Writing–review and editing, Writing–original draft, Methodology, Investigation, Data curation. IC: Writing–review and editing, Validation, Supervision, Funding acquisition, Formal Analysis, Conceptualization.
Funding
The author(s) declare that financial support was received for the research, authorship, and/or publication of this article. This work was partially funded by Fundação para a Ciência e a Tecnologia (FCT) through projects UIDB/00100/2020 (https://doi.org/10.54499/UIDB/00100/2020), UIDP/00100/2020 (https://doi.org/10.54499/UIDP/00100/2020) and LA/P/0056/2020, and PTDC/QUI-QIN/0586/2020.
Conflict of interest
The authors declare that the research was conducted in the absence of any commercial or financial relationships that could be construed as a potential conflict of interest.
The author(s) declared that they were an editorial board member of Frontiers, at the time of submission. This had no impact on the peer review process and the final decision.
Publisher’s note
All claims expressed in this article are solely those of the authors and do not necessarily represent those of their affiliated organizations, or those of the publisher, the editors and the reviewers. Any product that may be evaluated in this article, or claim that may be made by its manufacturer, is not guaranteed or endorsed by the publisher.
Abbreviations
ADME, absorption, distribution, metabolism, and excretion studies; AsPh3, triphenylarsine; bipy, 2, 2′-bipyridine; BSA, bovine serum albumin; DMF, dimethylformamide; DMSO, dimethyl sulfoxide; DNA, deoxyribonucleic acid; DPPH, 2,2-diphenyl-1-picrylhydrazyl radical; HH, hydrazide-hydrazone; HSA, human serum albumin; IC50, half-maximal inhibitory concentration; phen, 1,10-phenanthroline; PPh3, triphenylphosphine; PTD, photodynamic therapy; ROS, reactive oxygen species.
References
Abdelrahman, M. S. A., Omar, F. M., Saleh, A. A., and El-ghamry, M. A. (2022). Synthesis, molecular modeling, and docking studies of a new pyridazinone-acid hydrazone ligand, and its nano metal complexes. Spectroscopy, thermal analysis, electrical properties, DNA cleavage, antitumor, and antimicrobial activities. J. Mol. Struct. 1251, 131947. doi:10.1016/j.molstruc.2021.131947
Aboelmagd, A., El Rayes, S. M., Gomaa, M. S., Fathalla, W., Ali, I. A. I., Nafie, M. S., et al. (2021). Synthesis and cytotoxic activity of novel metal complexes derived from methyl-3-(4-chlorophenyl)-3-hydroxy-2,2-dimethylpropanoate as potential CDK8 kinase inhibitors. ACS Omega 6 (8), 5244–5254. doi:10.1021/acsomega.0c05263
Abouzayed, F. I., Emam, S. M., and Abouel-Enein, S. A. (2020). Synthesis, characterization and biological activity of nano-sized Co(II), Ni(II), Cu(II), Pd(II) and Ru(III) complexes of tetradentate hydrazone ligand. J. Mol. Struct. 1216, 128314. doi:10.1016/j.molstruc.2020.128314
Abumelha, H. M., Al-Fahemi, J. H., Althagafi, I., Bayazeed, A. A., Al-Ahmed, Z. A., Khedr, A. M., et al. (2020). Deliberate-characterization for Ni(II)-Schiff base complexes: promising in-vitro anticancer feature that matched MOE docking-approach. J. Inorg. Organomet. Polym. Mater. 30 (9), 3277–3293. doi:10.1007/s10904-020-01503-y
Adam, M. S. S., Shaaban, S., Khalifa, M. E., Alhasani, M., and El-Metwaly, N. (2021). New Cu(II) and VO(II)-O,N,O-aroylhydrazone complexes: biological evaluation, catalytic performance, ctDNA interaction, DFT, pharmacophore, and docking simulation. J. Mol. Liq. 335, 116554. doi:10.1016/j.molliq.2021.116554
Ahmad, F., Alkahtani, M. D. F., Taj, M. B., Alnajeebi, A. M., Alzahrani, S. O., Babteen, N. A., et al. (2021). Synthesis of new naphthyl aceto hydrazone-based metal complexes: micellar interactions, DNA binding, antimicrobial, and cancer inhibition studies. Molecules 26 (4), 1044. Accessed. doi:10.3390/molecules26041044
Alagesan, M., Bhuvanesh, N. S. P., and Dharmaraj, N. (2013). Potentially cytotoxic new copper(ii) hydrazone complexes: synthesis, crystal structure and biological properties. Dalton Trans. 42 (19), 7210–7223. doi:10.1039/C3DT50371B
Alkhamis, K., Alkhatib, F., Alsoliemy, A., Alrefaei, A. F., Katouah, H. A., Osman, H. E., et al. (2021). Elucidation for coordination features of hydrazide ligand under influence of variable anions in bivalent transition metal salts; green synthesis, biological activity confirmed by in-silico approaches. J. Mol. Struct. 1238, 130410. doi:10.1016/j.molstruc.2021.130410
Al-Radadi, N. S., and El-Gamil, M. M. (2023). Synthesis, spectroscopic, molecular docking, theoretical calculations, DNA-binding, and anticancer activity studies of gold (III), platinum (II), palladium (II), and ruthenium (III) complexes with Girard-T reagent. Appl. Organomet. Chem. 37 (11), e7241. doi:10.1002/aoc.7241
Alzahrani, S., Morad, M., Bayazeed, A., Aljohani, M. M., Alkhatib, F., Shah, R., et al. (2020). Ball milling approach to prepare new Cd(II) and Zn(II) complexes; characterization, crystal packing, cyclic voltammetry and MOE-docking agrees with biological assay. J. Mol. Struct. 1218, 128473. doi:10.1016/j.molstruc.2020.128473
Anđelković, K., Milenković, M. R., Pevec, A., Turel, I., Matić, I. Z., Vujčić, M., et al. (2017). Synthesis, characterization and crystal structures of two pentagonal-bipyramidal Fe(III) complexes with dihydrazone of 2,6-diacetylpyridine and Girard's T reagent. Anticancer properties of various metal complexes of the same ligand. J. Inorg. Biochem. 174, 137–149. doi:10.1016/j.jinorgbio.2017.06.011
Angelova, V., Karabeliov, V., Andreeva-Gateva, P. A., and Tchekalarova, J. (2016). Recent developments of hydrazide/hydrazone derivatives and their analogs as anticonvulsant agents in animal models. Drug Dev. Res. 77 (7), 379–392. doi:10.1002/ddr.21329
Anitha, N., Jhansi Lakshmi, P., Mudavath, R., and Sarala Devi, C. (2023). Experimental and theoretical studies on structural aspects of transition metal complexes of isonicotinoyl N-oxide hydrazone: their DNA-binding interaction and cytotoxicity. Appl. Organomet. Chem. 37 (12), e7289. doi:10.1002/aoc.7289
Anu, D., Naveen, P., VijayaPandiyan, B., Frampton, C. S., and Kaveri, M. V. (2019). An unexpected mixed valence tetranuclear copper(I/II) complex: synthesis, structural characterization, DNA/protein binding, antioxidant and anticancer properties. Polyhedron 167, 137–150. doi:10.1016/j.poly.2019.04.021
Arunachalam, A., Rengan, R., Umapathy, D., and Arockiam, A. J. V. (2022). Impact of biphenyl benzhydrazone-incorporated arene Ru(II) complexes on cytotoxicity and the cancer cell death mechanism. Organometallics 41, 2474–2486. doi:10.1021/acs.organomet.2c00290
Asghariazar, V., Amini, M., Pirdel, Z., Fekri, R., Asadi, A., Nejati-Koshki, K., et al. (2023). The Schiff base hydrazine copper(II) complexes induce apoptosis by P53 overexpression and prevent cell migration through protease-independent pathways. Med. Oncol. 40 (9), 271. doi:10.1007/s12032-023-02150-2
Ay, B., Şahin, O., Saygıdeğer Demir, B., Saygideger, Y., López-de-Luzuriaga, J. M., Mahmoudi, G., et al. (2020). Antitumor effects of novel nickel–hydrazone complexes in lung cancer cells. New J. Chem. 44 (21), 9064–9072. doi:10.1039/D0NJ00921K
Ayyannan, G., Mohanraj, M., Gopiraman, M., Uthayamalar, R., Raja, G., Bhuvanesh, N., et al. (2020). New Palladium(II) complexes with ONO chelated hydrazone ligand: synthesis, characterization, DNA/BSA interaction, antioxidant and cytotoxicity. Inorg. Chim. Acta 512, 119868. doi:10.1016/j.ica.2020.119868
Ayyannan, G., Mohanraj, M., Raja, G., Bhuvanesh, N., Nandhakumar, R., and Jayabalakrishnan, C. (2016). New palladium(II) hydrazone complexes: synthesis, structure and biological evaluation. J. Photochem. Photobiol. B Biol. 163, 1–13. doi:10.1016/j.jphotobiol.2016.08.003
Balaji, S., Mohamed Subarkhan, M. K., Ramesh, R., Wang, H., and Semeril, D. (2020). Synthesis and structure of arene Ru(II) N∧O-chelating complexes: in vitro cytotoxicity and cancer cell death mechanism. Organometallics 39 (8), 1366–1375. doi:10.1021/acs.organomet.0c00092
Balsa, L. M., Ferraresi-Curotto, V., Lavecchia, M. J., Echeverria, G. A., Piro, O. E., Garcia-Tojal, J., et al. (2021). Anticancer activity of a new copper(ii) complex with a hydrazone ligand. Structural and spectroscopic characterization, computational simulations and cell mechanistic studies on 2D and 3D breast cancer cell models. Dalton Trans. 50 (28), 9812–9826. doi:10.1039/d1dt00869b
Biswas, N., Saha, S., Biswas, B. K., Chowdhury, M., Rahaman, A., Junghare, V., et al. (2021). The DNA- and protein-binding properties and cytotoxicity of a new copper(II) hydrazone Schiff base complex. J. Coord. Chem. 74 (9-10), 1482–1504. doi:10.1080/00958972.2021.1913128
Brogyányi, T., Kaplánek, R., Kejík, Z., Hosnedlová, B., Antonyová, V., Abramenko, N., et al. (2022). Azulene hydrazide-hydrazones for selective targeting of pancreatic cancer cells. Biomed. Pharmacother. 155, 113736. doi:10.1016/j.biopha.2022.113736
Burgos-Lopez, Y., Balsa, L. M., Piro, O. E., Leon, I. E., Garcia-Tojal, J., Echeverria, G. A., et al. (2022). Tridentate acylhydrazone copper(II) complexes with heterocyclic bases as coligands. Synthesis, spectroscopic studies, crystal structure and cytotoxicity assays. Polyhedron 213, 115621. doi:10.1016/j.poly.2021.115621
Burgos-Lopez, Y., Del Pla, J., Balsa, L. M., Leon, I. E., Echeverria, G. A., Piro, O. E., et al. (2019). Synthesis, crystal structure and cytotoxicity assays of a copper(II) nitrate complex with a tridentate ONO acylhydrazone ligand. Spectroscopic and theoretical studies of the complex and its ligand. Inorg. Chim. Acta 487, 31–40. doi:10.1016/j.ica.2018.11.039
Chennam, K. P., Ravi, M., Boinala, A., Sudeepa, K., Ravinder, P., and Sarala Devi, C. (2022). Spectroanalytical, computational, DNA/BSA binding and in vitro cytotoxic activity studies of new transition metal complexes of novel aryl hydrazone. J. Mol. Struct. 1252, 132126. doi:10.1016/j.molstruc.2021.132126
Cindric, M., Bjelopetrovic, A., Pavlovic, G., Damjanovic, V., Lovric, J., Matkovic-Calogovic, D., et al. (2017). Copper(II) complexes with benzhydrazone-related ligands: synthesis, structural studies and cytotoxicity assay. New J. Chem. 41 (6), 2425–2435. doi:10.1039/c6nj03827a
Costa Pessoa, J. (2015). Thirty years through vanadium chemistry. J. Inorg. Biochem. 147, 4–24. doi:10.1016/j.jinorgbio.2015.03.004
Dash, S. P., Panda, A. K., Pasayat, S., Dinda, R., Biswas, A., Tiekink, E. R. T., et al. (2015). Oxidovanadium(v) complexes of aroylhydrazones incorporating heterocycles: synthesis, characterization and study of DNA binding, photo-induced DNA cleavage and cytotoxic activities. RSC Adv. 5 (64), 51852–51867. doi:10.1039/C4RA14369H
de Almeida, P., Pereira, T. M., Kummerle, A. E., Guedes, G. P., Silva, H., de Oliveira, L. L., et al. (2019). New Ru(II)-DMSO complexes containing coumarin-N-acylhydrazone hybrids: synthesis, X-ray structures, cytotoxicity and antimicrobial activities. Polyhedron 171, 20–31. doi:10.1016/j.poly.2019.06.053
Deng, J. G., Chen, W., and Deng, H. (2016). Synthesis of dipyridyl ketone isonicotinoyl hydrazone copper(II) complex: structure, anticancer activity and anticancer mechanism. J. Fluoresc. 26 (6), 1987–1996. doi:10.1007/s10895-016-1892-2
Deng, J. G., Gou, Y., Chen, W., Fu, X., and Deng, H. (2016). The Cu/ligand stoichiometry effect on the coordination behavior of aroyl hydrazone with copper(II): structure, anticancer activity and anticancer mechanism. Bioorg. Med. Chem. 24 (10), 2190–2198. doi:10.1016/j.bmc.2016.03.033
Deng, J. G., Wang, J., Khan, M., Yu, P., Yang, F., and Liang, H. (2018). Structure and biological properties of five Pt(II) complexes as potential anticancer agents. J. Inorg. Biochem. 185, 10–16. doi:10.1016/j.jinorgbio.2018.04.017
Devi, J., Kumar, S., Kumar, D., Jindal, D. K., and Poornachandra, Y. (2022). Synthesis, characterization, in vitro antimicrobial and cytotoxic evaluation of Co(II), Ni(II), Cu(II) and Zn(II) complexes derived from bidentate hydrazones. Res. Chem. Intermed. 48 (1), 423–455. doi:10.1007/s11164-021-04602-8
Devi, J., Sharma, S., Kumar, S., Jindal, D. K., Dutta, P. P., and Kumar, D. (2021). Transition metal (II) complexes of hydrazones derived from tetralone: synthesis, spectral characterization, in vitro antimicrobial and cytotoxic studies. Res. Chem. Intermed. 47 (6), 2433–2467. doi:10.1007/s11164-021-04413-x
Du, Y. P., Chen, W., Fu, X., Deng, H., and Deng, J. G. (2016). Synthesis and biological evaluation of heterocyclic hydrazone transition metal complexes as potential anticancer agents. RSC Adv. 6 (111), 109718–109725. doi:10.1039/c6ra23477a
Elankathirselvan, K., Paunkumar, P., Srinivasan, K., Premkumar, T., and Babu, S. G. (2024). Green synthesis and structural and cytotoxic properties of a new silver(I) complex: solid-state, single-source precursor of Ag and Ag2O NPs. J. Mol. Struct. 1301, 137298. doi:10.1016/j.molstruc.2023.137298
Elsayed, S. A., Butler, I. S., Claude, B. J., and Mostafa, S. I. (2015). Synthesis, characterization and anticancer activity of 3-formylchromone benzoylhydrazone metal complexes. Transit. Metal. Chem. 40 (2), 179–187. doi:10.1007/s11243-014-9904-z
Elsayed, S. A., Elnabky, I. M., di Biase, A., and El-Hendawy, A. M. (2022). New mixed ligand copper(II) hydrazone-based complexes: synthesis, characterization, crystal structure, DNA/RNA/BSA binding, in vitro anticancer, apoptotic activity, and cell cycle analysis. Appl. Organomet. Chem. 36 (1). doi:10.1002/aoc.6481
Eswaran, J., Sankar, N. K., Bhuvanesh, N. S. P., and Velusamy, K. M. (2019). Ruthenium hydrazone complexes with 1:1 and 1:2 metal-ligand stoichiometry: a comparison of biomolecular interactions and in vitro cytotoxicities. Transit. Metall. Chem. 44 (4), 369–382. doi:10.1007/s11243-018-00303-1
Eswaran, J., Sivalingam, K., Viswanatha, V. P., Nattamai, S. B., and Nallasamy, D. (2015). Synthesis, characterization, DNA/protein binding and in vitro cytotoxic evaluation of new Ru(III) complexes containing aroylhydrazone ligands: does hydrogen bonding influence the coordination behavior of hydrazones? Inorganica Chim. Acta 429, 148–159. doi:10.1016/j.ica.2015.01.045
Fekri, R., Salehi, M., Asadi, A., and Kubicki, M. (2019). Synthesis, characterization, anticancer and antibacterial evaluation of Schiff base ligands derived from hydrazone and their transition metal complexes. Inorg. Chim. Acta 484, 245–254. doi:10.1016/j.ica.2018.09.022
Feng, X. X., and Liu, J. C. (2018). DNA binding and in vitro anticarcinogenic activity of a series of newfashioned Cu(II)-complexes based on tricationic metalloporphyrin salicyloylhydrazone ligands. J. Inorg. Biochem. 178, 1–8. doi:10.1016/j.jinorgbio.2017.09.024
Firmino, G. d. S. S., André, S. C., Hastenreiter, Z., Campos, V. K., Abdel-Salam, M. A. L., de Souza-Fagundes, E. M., et al. (2019). In vitro assessment of the cytotoxicity of Gallium(III) complexes with Isoniazid-Derived Hydrazones: effects on clonogenic survival of HCT-116 cells. Inorg. Chim. Acta 497, 119079. doi:10.1016/j.ica.2019.119079
Firmino, G. S. S., de Souza, M. V. N., Pessoa, C., Lourenco, M. C. S., Resende, J., and Lessa, J. A. (2016). Synthesis and evaluation of copper(II) complexes with isoniazid-derived hydrazones as anticancer and antitubercular agents. Biometals 29 (6), 953–963. doi:10.1007/s10534-016-9968-7
Gaber, M., Khedr, A. M., and Elsharkawy, M. (2017). Characterization and thermal studies of nano-synthesized Mn(II), Co(II), Ni(II) and Cu(II) complexes with adipohydrazone ligand as new promising antimicrobial and antitumor agents. Appl. Organomet. Chem. 31 (12). doi:10.1002/aoc.3885
Golla, U., Adhikary, A., Mondal, A. K., Tomar, R. S., and Konar, S. (2016). Synthesis, structure, magnetic and biological activity studies of bis-hydrazone derived Cu(II) and Co(II) coordination compounds. Dalton Trans. 45 (29), 11849–11863. doi:10.1039/c6dt01496h
Gou, Y., Qi, J. X., Ajayi, J. P., Zhang, Y., Zhou, Z. P., Wu, X. Y., et al. (2015). Developing anticancer copper(II) pro-drugs based on the nature of cancer cells and the human serum albumin carrier IIA subdomain. Mol. Pharm. 12 (10), 3597–3609. doi:10.1021/acs.molpharmaceut.5b00314
Gou, Y., Zhang, Y., Zhang, Z., Wang, J., Zhou, Z., Liang, H., et al. (2017). Design of an anticancer copper(II) prodrug based on the Lys199 residue of the active targeting human serum albumin nanoparticle carrier. Mol. Pharm. 14 (6), 1861–1873. doi:10.1021/acs.molpharmaceut.6b01074
Haribabu, J., Srividya, S., Umapathi, R., Gayathri, D., Venkatesu, P., Bhuvanesh, N., et al. (2020). Enhanced anticancer activity of half-sandwich Ru(II)-p-cymene complex bearing heterocyclic hydrazone ligand. Inorg. Chem. Commun. 119, 108054. doi:10.1016/j.inoche.2020.108054
Hassan, S. S., Rizk, N. M. H., Khidr, M. A., and Aly, S. A. (2024). Antibacterial, antifungal, and anticancer screening of some nano mono Co(II), Zn(II), Ru(III), and Ag(I) binuclear metal complexes of new hydrazone ligand. Appl. Organomet. Chem. 38 (1), e7298. doi:10.1002/aoc.7298
Hegde, D., Dodamani, S., Kumbar, V., Jalalpure, S., and Gudasi, K. B. (2017). Synthesis, crystal structure, DNA interaction and anticancer evaluation of pyruvic acid derived hydrazone and its transition metal complexes. Appl. Organomet. Chem. 31 (12). doi:10.1002/aoc.3851
Hong, M., Chang, G. L., Li, R., and Niu, M. J. (2016). Anti-proliferative activity and DNA/BSA interactions of five mono- or di-organotin(IV) compounds derived from 2-hydroxy-N '- (2-hydroxy-3-methoxyphenyl)methylidene -benzohydrazone. New J. Chem. 40 (9), 7889–7900. doi:10.1039/c6nj00525j
Hosny, N. M., Hassan, N. Y., Mahmoud, H. M., and Abdel-Rhman, M. H. (2019). Synthesis, characterization and cytotoxicity of new 2-isonicotinoyl-N-phenylhydrazine-1-carbothioamide and its metal complexes. Appl. Organomet. Chem. 33 (8), e4998. doi:10.1002/aoc.4998
Hruskova, K., Potuckova, E., Hergeselova, T., Liptakova, L., Haskova, P., Mingas, P., et al. (2016). Aroylhydrazone iron chelators: tuning antioxidant and antiproliferative properties by hydrazide modifications. Eur. J. Med. Chem. 120, 97–110. doi:10.1016/j.ejmech.2016.05.015
Hu, K., Li, F. Y., Zhang, Z., and Liang, F. P. (2017). Synthesis of two potential anticancer copper(II) complex drugs: their crystal structure, human serum albumin/DNA binding and anticancer mechanism. New J. Chem. 41 (5), 2062–2072. doi:10.1039/c6nj02483a
Hu, K., Zhou, G., Zhang, Z., Li, F., Li, J., and Liang, F. (2016). Two hydrazone copper(ii) complexes: synthesis, crystal structure, cytotoxicity, and action mechanism. RSC Adv. 6 (42), 36077–36084. doi:10.1039/C6RA03478K
Hu, Y., Zheng, Y., Dai, M., Wu, J., Yu, B., Zhang, H., et al. (2019). Snail2 induced E-cadherin suppression and metastasis in lung carcinoma facilitated by G9a and HDACs. Cell. Adhesion Migr. 13 (1), 284–291. doi:10.1080/19336918.2019.1638689
Hu, Y.-Q., Zhang, S., Zhao, F., Gao, C., Feng, L.-S., Lv, Z.-S., et al. (2017). Isoniazid derivatives and their anti-tubercular activity. Eur. J. Med. Chem. 133, 255–267. doi:10.1016/j.ejmech.2017.04.002
Ibrahim, O., and O'Sullivan, J. (2020). Iron chelators in cancer therapy. Biometals 33 (4-5), 201–215. doi:10.1007/s10534-020-00243-3
Jayanthi, E., Venkataramana, M., Neethu, S., Bhuvanesh, N. S. P., and Dharmaraj, N. (2017). Biomolecular interaction and in vitro cytotoxicity of ruthenium complexes containing heterocyclic hydrazone. Is methanol a non-innocent solvent to influence the oxidation state of the metal and ligation of hydrazone? Polyhedron 132, 39–52. doi:10.1016/j.poly.2017.04.026
Ji, Y., Dai, F., and Zhou, B. (2018). Designing salicylaldehyde isonicotinoyl hydrazones as Cu(II) ionophores with tunable chelation and release of copper for hitting redox Achilles heel of cancer cells. Free Rad. Free Radic. Biol. Med. 129, 215–226. doi:10.1016/j.freeradbiomed.2018.09.017
Jiang, S. S., Ni, H. H., Liu, F., Gu, S. S., Yu, P., and Gou, Y. (2020). Binuclear Schiff base copper(II) complexes: syntheses, crystal structures, HSA interaction and anti-cancer properties. Inorg. Chim. Acta 499, 119186. doi:10.1016/j.ica.2019.119186
Kanchanadevi, S., Fronczek, F. R., Immanuel David, C., Nandhakumar, R., and Mahalingam, V. (2021). Investigation of DNA/BSA binding and cytotoxic properties of new Co(II), Ni(II) and Cu(II) hydrazone complexes. Inorg. Chim. Acta 526, 120536. doi:10.1016/j.ica.2021.120536
Kanchanadevi, S., Fronczek, F. R., and Mahalingam, V. (2021). Ruthenium(III) hydrazone complexes with triphenylphosphine/triphenylarsine co-ligands: synthesis, DNA/BSA binding, antioxidative and cytotoxic activity. Inorg. Chim. Acta 526, 120532. doi:10.1016/j.ica.2021.120532
Kaur, J., Chikate, T., Bandyopadhyay, P., Basu, S., and Chikate, R. (2020). Cu(II) complexes of hydrazones–NSAID conjugates: synthesis, characterization and anticancer activity. J. Coord. Chem. 73 (23), 3186–3202. doi:10.1080/00958972.2020.1843160
Kavitha, R., Reddy, C. V. R., and Sireesha, B. (2021). Synthesis, spectroscopic and biological activity evaluation of Ni(II), Cu(II) and Zn(II) complexes of schiff base derived from pyridoxal and 4-fluorobenzohydrazide. Nucleosides, Nucleotides Nucleic Acids 40 (8), 845–866. doi:10.1080/15257770.2021.1961271
Kioseoglou, E., Petanidis, S., Gabriel, C., and Salifoglou, A. (2015). The chemistry and biology of vanadium compounds in cancer therapeutics. Coord. Chem. Rev. 301-302, 87–105. doi:10.1016/j.ccr.2015.03.010
Kumar, N., Asija, S., Deswal, Y., Kumar, D., Kumar Jindal, D., Kumar, L., et al. (2023). Insighting the inhibitory potential of new diorganotin(IV) complexes derived from tridentate ligands: synthesis, structural elucidation and biological evaluation. Inorg. Chem. Commun. 154, 110953. doi:10.1016/j.inoche.2023.110953
Lee, S. Y., Kim, C. Y., and Nam, T. G. (2020). Ruthenium complexes as anticancer agents: a brief history and perspectives. Drug Des. Dev. Ther. 14 (1177-8881 (Electronic)), 5375–5392. doi:10.2147/DDDT.S275007
Li, Y., Yang, Z., Zhou, M., and Li, Y. (2017). Synthesis and crystal structure of new monometallic Ni(ii) and Co(ii) complexes with an asymmetrical aroylhydrazone: effects of the complexes on DNA/protein binding property, molecular docking, and in vitro anticancer activity. RSC Adv. 7 (78), 49404–49422. doi:10.1039/C7RA10283F
Lin, K., Zhao, Z.-Z., Bo, H.-B., Hao, X.-J., and Wang, J.-Q. (2018). Applications of ruthenium complex in tumor diagnosis and therapy. Front. Pharmacol. 9, 1323. doi:10.3389/fphar.2018.01323
Liu, J., Lai, H., Xiong, Z., Chen, B., and Chen, T. (2019). Functionalization and cancer-targeting design of ruthenium complexes for precise cancer therapy. Chem. Commun. 55 (67), 9904–9914. doi:10.1039/C9CC04098F
Liu, K., Yan, H., Chang, G. L., Li, Z., Niu, M. J., and Hong, M. (2017). Organotin(IV) complexes derived from hydrazone Schiff base: synthesis, crystal structure, in vitro cytotoxicity and DNA/BSA interactions. Inorg. Chim. Acta 464, 137–146. doi:10.1016/j.ica.2017.05.017
Mackova, E., Hruskova, K., Bendova, P., Vavrova, A., Jansova, H., Haskova, P., et al. (2012). Methyl and ethyl ketone analogs of salicylaldehyde isonicotinoyl hydrazone: novel iron chelators with selective antiproliferative action. Chem. Biol. Interact. 197 (2-3), 69–79. doi:10.1016/j.cbi.2012.03.010
Maikoo, S., Dingle, L. M. K., Chakraborty, A., Xulu, B., Edkins, A. L., and Booysen, I. N. (2020). Synthetic, characterization and cytotoxic studies of ruthenium complexes with Schiff bases encompassing biologically relevant moieties. Polyhedron 184, 114569. doi:10.1016/j.poly.2020.114569
Mo, Q. Y., Deng, J. G., Liu, Y. N., Huang, G. D., Li, Z. W., Yu, P., et al. (2018). Mixed-ligand Cu(II) hydrazone complexes designed to enhance anticancer activity. Eur. J. Med. Chem. 156, 368–380. doi:10.1016/j.ejmech.2018.07.022
Mohamed Subarkhan, M. K., Ramesh, R., and Liu, Y. (2016). Synthesis and molecular structure of arene ruthenium(II) benzhydrazone complexes: impact of substitution at the chelating ligand and arene moiety on antiproliferative activity. New J. Chem. 40 (11), 9813–9823. doi:10.1039/c6nj01936f
Mohanraj, M., Ayyannan, G., Raja, G., and Jayabalakrishnan, C. (2016). Synthesis, characterization, DNA binding, DNA cleavage, antioxidant and in vitro cytotoxicity studies of ruthenium(II) complexes containing hydrazone ligands. J. Coord. Chem. 69 (23), 3545–3559. doi:10.1080/00958972.2016.1235700
Mohanty, M., Maurya, S. K., Banerjee, A., Patra, S. A., Maurya, M. R., Crochet, A., et al. (2019). In vitro cytotoxicity and catalytic evaluation of dioxidovanadium(V) complexes in an azohydrazone ligand environment. New J. Chem. 43 (45), 17680–17695. doi:10.1039/c9nj01815h
Monika, S., and Ramesh, R. (2023). New dinuclear arene Ru(ii) benzilbis(furoylhydrazone) complexes: synthesis, structure, and anticancer activity. New J. Chem. 47 (33), 15622–15630. doi:10.1039/D3NJ02869K
Nair, R. S., Kuriakose, M., Somasundaram, V., Shenoi, V., Kurup, M. P., and Srinivas, P. (2014). The molecular response of vanadium complexes of nicotinoyl hydrazone in cervical cancers—a possible interference with HPV oncogenic markers. Life Sci. 116 (2), 90–97. doi:10.1016/j.lfs.2014.09.011
Neethu, K. S., Sivaselvam, S., Theetharappan, M., Ranjitha, J., Bhuvanesh, N. S. P., Ponpandian, N., et al. (2021). In vitro evaluations of biomolecular interactions, antioxidant and anticancer activities of Nickel(II) and Copper(II) complexes with 1:2 coordination of anthracenyl hydrazone ligands. Inorg. Chim. Acta 524, 120419. doi:10.1016/j.ica.2021.120419
Parsekar, S. U., Singh, M., Mishra, D. P., Antharjanam, P. K. S., Koley, A. P., and Kumar, M. (2019). Efficient hydrolytic cleavage of DNA and antiproliferative effect on human cancer cells by two dinuclear Cu(II) complexes containing a carbohydrazone ligand and 1,10-phenanthroline as a coligand. J. Biol. Inorg. Chem. 24 (3), 343–363. doi:10.1007/s00775-019-01651-8
Parsekar, S. U., Velankanni, P., Sridhar, S., Haldar, P., Mate, N. A., Banerjee, A., et al. (2020). Protein binding studies with human serum albumin, molecular docking and in vitro cytotoxicity studies using HeLa cervical carcinoma cells of Cu(ii)/Zn(ii) complexes containing a carbohydrazone ligand. Dalton Trans. 49 (9), 2947–2965. doi:10.1039/C9DT04656A
Patel, S. K., Patel, R. N., Singh, Y., Singh, Y. P., Kumhar, D., Jadeja, R. N., et al. (2019). Three new tetranuclear phenoxy-bridged metal(II) complexes: synthesis, structural variation, cryomagnetic properties, DFT study and antiprolifirative properties. Polyhedron 161, 198–212. doi:10.1016/j.poly.2019.01.006
Patil, P. R., Javarappa, R., Kumar, A. C., and Naik, N. (2022). Synthesis of Co(II), Ni(II) and Zn(II) metal complexes derived from a hydrazone schiff base: electrochemical behavior and comprehensive biological studies. ChemistrySelect 7 (35). doi:10.1002/slct.202200752
Patra, D., Paul, S., Majumder, I., Sepay, N., Bera, S., Kundu, R., et al. (2017). Exploring the effect of substituent in the hydrazone ligand of a family of mu-oxidodivanadium(v) hydrazone complexes on structure, DNA binding and anticancer activity. Dalton Trans. 46 (46), 16276–16293. doi:10.1039/c7dt03585c
Popiołek, Ł. (2017). Hydrazide–hydrazones as potential antimicrobial agents: overview of the literature since 2010. Med. Chem. Res. 26 (2), 287–301. doi:10.1007/s00044-016-1756-y
Popiołek, Ł. (2021). Updated information on antimicrobial activity of hydrazide–hydrazones. Int. J. Mol. Sci. 22 (17), 9389. doi:10.3390/ijms22179389
Prabaharan, R., Rengan, R., Umapathy, D., Arockiam, A. J. V., and Malecki, J. G. (2022). Assessment of antiproliferative activity of new half-sandwich arene Ru (II) furylbenzhydrazone complexes. Appl. Organomet. Chem. 36 (2). doi:10.1002/aoc.6512
Qi, J., Zheng, Y., Li, B., Wei, L., Li, J., Xu, X., et al. (2022). Mechanism of vitamin B6 benzoyl hydrazone platinum(II) complexes overcomes multidrug resistance in lung cancer. Eur. J. Med. Chem. 237, 114415. doi:10.1016/j.ejmech.2022.114415
Rada, J. P., Bastos, B. S. M., Anselmino, L., Franco, C. H. J., Lanznaster, M., Diniz, R., et al. (2019). Binucleating hydrazonic ligands and their μ-hydroxodicopper(II) complexes as promising structural motifs for enhanced antitumor activity. Inorg. Chem. 58 (13), 8800–8819. doi:10.1021/acs.inorgchem.9b01195
Raja, D. S., Bhuvanesh, N. S. P., and Natarajan, K. (2012). A novel water soluble ligand bridged cobalt(II) coordination polymer of 2-oxo-1,2-dihydroquinoline-3-carbaldehyde (isonicotinic) hydrazone: evaluation of the DNA binding, protein interaction, radical scavenging and anticancer activity. Dalton Trans. 41 (15), 4365–4377. doi:10.1039/c2dt12274j
Raj Kumar, R., Ramesh, R., and Małecki, J. G. (2018). Synthesis and structure of arene ruthenium(II) benzhydrazone complexes: antiproliferative activity, apoptosis induction and cell cycle analysis. J. Organomet. Chem. 862, 95–104. doi:10.1016/j.jorganchem.2018.03.013
Ramachandran, E., Gandin, V., Bertani, R., Sgarbossa, P., Natarajan, K., Bhuvanesh, N. S. P., et al. (2018). Synthesis, characterization and cytotoxic activity of novel copper(II) complexes with aroylhydrazone derivatives of 2-Oxo-1,2-dihydrobenzo h quinoline-3-carbaldehyde. J. Inorg. Biochem. 182, 18–28. doi:10.1016/j.jinorgbio.2018.01.016
Rehder, D. (2015). The role of vanadium in biology. Metallomics 7 (5), 730–742. doi:10.1039/C4MT00304G
Ribeiro, N., Bulut, I., Pósa, V., Sergi, B., Sciortino, G., Pessoa, J. C., et al. (2022). Solution chemical properties and anticancer potential of 8-hydroxyquinoline hydrazones and their oxidovanadium(IV) complexes. J. Inorg. Biochem. 235, 111932. doi:10.1016/j.jinorgbio.2022.111932
Ribeiro, N., Bulut, I., Sergi, B., Pósa, V., Spengler, G., Sciortino, G., et al. (2023). Promising anticancer agents based on 8-hydroxyquinoline hydrazone copper(II) complexes. Front. Chem. 11, 1106349. doi:10.3389/fchem.2023.1106349
Ribeiro, N., Galvao, A., Gomes, C., Ramos, H., Pinheiro, R., Saraiva, L., et al. (2019). Naphthoylhydrazones: coordination to metal ions and biological screening. New J. Chem. 43 (45), 17801–17818. doi:10.1039/c9nj01816f
Ribeiro, N., Roy, S., Butenko, N., Cavaco, I., Pinheiro, T., Alho, I., et al. (2017). New Cu(II) complexes with pyrazolyl derived Schiff base ligands: synthesis and biological evaluation. J. Inorg. Biochem. 174, 63–75. doi:10.1016/j.jinorgbio.2017.05.011
Rodic, M. V., Leovac, V. M., Jovanović, L. S., Spasojevic, V., Joksovic, M. D., Stanojkovic, T., et al. (2016). Synthesis, characterization, cytotoxicity and antiangiogenic activity of copper(II) complexes with 1-adamantoyl hydrazone bearing pyridine rings. Eur. J. Med. Chem. 115, 75–81. doi:10.1016/j.ejmech.2016.03.003
Rollas, S., and Küçükgüzel, S. G. (2007). Biological activities of hydrazone derivatives. Molecules 12 (8), 1910–1939. Accessed. doi:10.3390/12081910
Rosenberg, B., Vancamp, L., Trosko, J. E., and Mansour, V. H. (1969). Platinum compounds: a new class of potent antitumour agents. Nature 222 (5191), 385–386. doi:10.1038/222385a0
Sahu, G., Banerjee, A., Samanta, R., Mohanty, M., Lima, S., Tiekink, E. R. T., et al. (2021). Water-soluble dioxidovanadium(V) complexes of aroylhydrazones: DNA/BSA interactions, hydrophobicity, and cell-selective anticancer potential. Inorg. Chem. 60 (20), 15291–15309. doi:10.1021/acs.inorgchem.1c01899
Sahu, G., Sahu, K., Patra, S. A., Mohapatra, D., Khangar, R., Sengupta, S., et al. (2023). Hydrolytically stable TiIV–Hydrazone-Based metallodrugs: protein interaction and anticancer potential. ACS Appl. Bio Mat. 6 (12), 5360–5371. doi:10.1021/acsabm.3c00629
Santos, A. F., Ferreira, I. P., Pinheiro, C. B., Santos, V. G., Lopes, M. T. P., Teixeira, L. R., et al. (2018). [Ag(L)NO3] complexes with 2-benzoylpyridine-derived hydrazones: cytotoxic activity and interaction with biomolecules. ACS Omega 3 (6), 7027–7035. doi:10.1021/acsomega.8b00533
Sawkmie, M., Bhattacharyya, M., Banothu, V., Kaminsky, W., Gannon, P. M., Majaw, S., et al. (2023). Ruthenium, rhodium, and iridium complexes featuring fluorenyl benzohydrazone derivatives: synthesis and preliminary investigation of their anticancer and antibacterial activity. J. Mol. Struct. 1291, 135994. doi:10.1016/j.molstruc.2023.135994
Saygıdeğer Demir, B., Mahmoudi, G., Sezan, A., Derinoz, E., Nas, E., Saygideger, Y., et al. (2021). Evaluation of the antitumor activity of a series of the pincer-type metallocomplexes produced from isonicotinohydrazide derivative. J. Inorg. Biochem. 223, 111525. doi:10.1016/j.jinorgbio.2021.111525
Shen, S. S., Chen, H., Zhu, T. F., Ma, X. Q., Xu, J., Zhu, W. J., et al. (2017). Synthesis, characterization and anticancer activities of transition metal complexes with a nicotinohydrazone ligand. Oncol. Lett. 13 (5), 3169–3176. doi:10.3892/ol.2017.5857
Silva-Caldeira, P. P., Oliveira Junior, A. C. A. d., and Pereira-Maia, E. C. (2021). Photocytotoxic activity of ruthenium(II) complexes with phenanthroline-hydrazone ligands. Molecules 26 (7), 2084. doi:10.3390/molecules26072084
Stevanović, N., Mazzeo, P. P., Bacchi, A., Matić, I. Z., Đorđić Crnogorac, M., Stanojković, T., et al. (2021). Synthesis, characterization, antimicrobial and cytotoxic activity and DNA-binding properties of d-metal complexes with hydrazones of Girard’s T and P reagents. J. Biol. Inorg. Chem. 26 (8), 863–880. doi:10.1007/s00775-021-01893-5
Subarkhan, M. K. M., and Ramesh, R. (2016). Ruthenium(II) arene complexes containing benzhydrazone ligands: synthesis, structure and antiproliferative activity. Inorg. Chem. Front. 3 (10), 1245–1255. doi:10.1039/c6qi00197a
Sung, Y.-S., Kerimoglu, B., Ooi, A., and Tomat, E. (2022). Aroylhydrazone glycoconjugate prochelators exploit glucose transporter 1 (GLUT1) to target iron in cancer cells. ACS Med. Chem. Lett. 13 (9), 1452–1458. doi:10.1021/acsmedchemlett.2c00250
Sutradhar, M., Roy Barman, T., Roy Barman, T., Fernandes, A. R., Paradinha, F., Roma-Rodrigues, C., et al. (2017). Mixed ligand aroylhydrazone and N-donor heterocyclic Lewis base Cu(II) complexes as potential antiproliferative agents. J. Inorg. Biochem. 175, 267–275. doi:10.1016/j.jinorgbio.2017.07.034
Teneva, Y., Simeonova, R., Valcheva, V., and Angelova, V. T. (2023). Recent advances in anti-tuberculosis drug discovery based on hydrazide–hydrazone and thiadiazole derivatives targeting InhA. Pharmaceuticals 16 (4), 484. doi:10.3390/ph16040484
Verma, S., Lal, S., Narang, R., and Sudhakar, K. (2023). Quinoline hydrazide/hydrazone derivatives: recent insights on antibacterial activity and mechanism of action. ChemMedChem 18 (5), e202200571. doi:10.1002/cmdc.202200571
Vineetha, M. C., Joy, F., Krishna P, M., Vinod, T. P., Venkataraman, S. K., Agarwal, A. K., et al. (2021). Novel dioxidomolybdenum complexes containing ONO chelators: synthesis, physicochemical properties, crystal structures, Hirshfeld surface analysis, DNA binding/cleavage studies, docking, and in vitro cytotoxicity. Appl. Organomet. Chem. 35 (9). doi:10.1002/aoc.6334
Wang, J., Xu, G. C., Zhang, Y. P., Luo, H. Y., Li, J. Y., Zhang, L., et al. (2019). Copper(II) complexes with 4-acyl pyrazolone derivatives and diimine coligands: synthesis, structural characterization, DNA binding and antitumor activity. New J. Chem. 43 (6), 2529–2539. doi:10.1039/c8nj02695e
Wu, Y. R., Hou, L. X., Lan, J. F., Yang, F., Huang, G. J., Liu, W., et al. (2023). Mixed-ligand copper(II) hydrazone complexes: synthesis, structure, and anti-lung cancer properties. J. Mol. Struct. 1279, 134986. doi:10.1016/j.molstruc.2023.134986
Xie, J., Shen, S., Chen, R., Xu, J., Dong, K., Huang, J., et al. (2017). Synthesis, characterization and antitumor activity of Ln(III) complexes with hydrazone Schiff base derived from 2-acetylpyridine and isonicotinohydrazone. Oncol. Lett. 13, 4413–4419. doi:10.3892/ol.2017.6018
Xu, L. D., Hong, M., Yang, Y. G., Cui, J. C., and Li, C. Z. (2016). Synthesis, structural characterization, in vitro cytotoxicities, and BSA interaction of di-organotin(IV) complexes derived from salicylaldehyde nicotinoyl hydrazone. J. Coord. Chem. 69 (17), 2598–2609. doi:10.1080/00958972.2016.1217408
Xu, Z. Q., Mao, X. J., Jia, L., Xu, J., Zhu, T. F., Cai, H. X., et al. (2015). Synthesis, characterization and anticancer activities of two lanthanide(III) complexes with a nicotinohydrazone ligand. J. Mol. Struct. 1102, 86–90. doi:10.1016/j.molstruc.2015.07.047
Yang, P., Zhang, D. D., Wang, Z. Z., Liu, H. Z., Shi, Q. S., and Xie, X. B. (2019). Copper(ii) complexes with NNO ligands: synthesis, crystal structures, DNA cleavage, and anticancer activities. Dalton Trans. 48 (48), 17925–17935. doi:10.1039/c9dt03746b
Yang, Y. L., Li, C. P., Fu, Y., Liu, Y. X., Zhang, Y., Zhang, Y. F., et al. (2016). Redox cycling of a copper complex with benzaldehyde nitrogen mustard-2-pyridine carboxylic acid hydrazone contributes to its enhanced antitumor activity, but no change in the mechanism of action occurs after chelation. Oncol. Rep. 35 (3), 1636–1644. doi:10.3892/or.2015.4530
Keywords: metallodrugs, anticancer, hydrazides, hydrazones, Schiff bases
Citation: Ribeiro N and Correia I (2024) A review of hydrazide-hydrazone metal complexes’ antitumor potential. Front. Chem. Biol 3:1398873. doi: 10.3389/fchbi.2024.1398873
Received: 10 March 2024; Accepted: 10 April 2024;
Published: 25 April 2024.
Edited by:
Magdalena Malik, Wrocław University of Science and Technology, PolandCopyright © 2024 Ribeiro and Correia. This is an open-access article distributed under the terms of the Creative Commons Attribution License (CC BY). The use, distribution or reproduction in other forums is permitted, provided the original author(s) and the copyright owner(s) are credited and that the original publication in this journal is cited, in accordance with accepted academic practice. No use, distribution or reproduction is permitted which does not comply with these terms.
*Correspondence: Nádia Ribeiro, bmFkaWEucmliZWlyb0B0ZWNuaWNvLnVsaXNib2EucHQ=