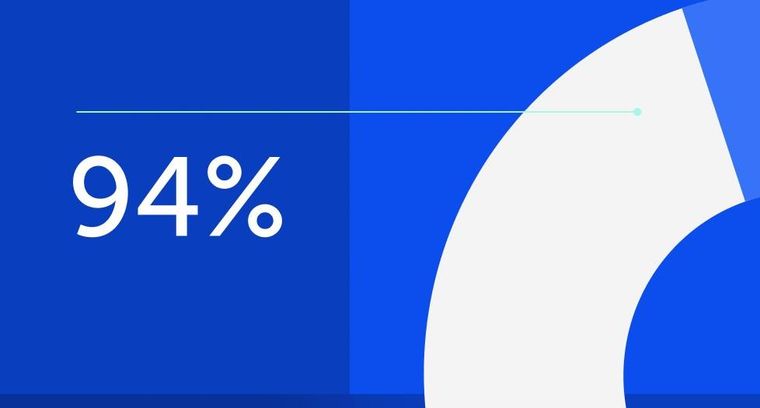
94% of researchers rate our articles as excellent or good
Learn more about the work of our research integrity team to safeguard the quality of each article we publish.
Find out more
MINI REVIEW article
Front. Chem. Biol., 10 April 2024
Sec. Quantitative and Analytical Techniques
Volume 3 - 2024 | https://doi.org/10.3389/fchbi.2024.1360027
The food control of tropane and pyrrolizidine alkaloids has become in recent years in Europe one of the main priorities within the food safety field due to the exponential increase in food alerts related to the concerning levels of these natural toxins in foods. This awareness has led to increasing progress in the research and determination of these compounds. Hence, this mini-review provides a brief and comprehensive overview of the evolution of these food alerts, highlighting the main food products frequently contaminated with these alkaloids and reviewing the analytical advances in their determination within the las 3 years (from 2020 to 2023).
Countless plants belonging to different genera naturally present many phytochemical substances in their composition. Among these substances, the presence of alkaloids can be highlighted. Alkaloids are nitrogenous compounds synthesized as secondary metabolites that act as a defense mechanism of many plants. It is well known that these alkaloids have great pharmacological potential, and therefore their toxicity and applicability in this field have been largely studied (Thawabteh et al., 2019). However, the intake of these alkaloids can have serious health consequences (Casado et al., 2024a). Hence, awareness of the emergence of these compounds as food contaminants has increased significantly in recent years. Particularly, many of the food alerts recently notified in Europe have been related to levels of tropane and pyrrolizidine alkaloids (hereinafter TAs and PAs, respectively) (Figures 1A, B) found above legislated limits in a wide variety of food products (Casado et al., 2022a; González-Gómez et al., 2022a). Hence, within the last 3 years, increasing progress in the research of these compounds as food contaminants has been made. Consequently, the first European regulation to monitor the occurrence of these compounds in food was published in 2020 and 2021 for PAS and TAs, respectively (European Union, 2021; European Union, 2023). Nonetheless, in April 2023, these regulations were revised, updated, and extended for more food products, establishing the regulation currently in force for the control of these contaminants (European Union, 2024). Likewise, recently, a new regulation establishing the methods of sampling and analysis for the control of the levels of plant toxins in food has been published (Commission implementing regulation, 2024).
Figure 1. (A) General chemical structure of pyrrolizidine alkaloids (PAs) including different forms of them (R1 and R2 correspond to different necic acids), (B) general chemical structure of tropane alkaloids (TAs) and the main representative TAs (atropine and scopolamine), and (C) evolution of number of food alerts notified in the European Rapid Alert System for Food and Feed (RASFF) window related to the occurrence of TAs and PAs in different food categories from 2020 to 2023. Data obtained from the RASFF window portal from 1st January 2020 to 30th November 2023.
Accordingly, the aim of this mini-review is to provide an overview of the evolution of the food alerts related to the occurrence of TAs and PAs in Europe within the last 3 years (from 2020 to 2023), highlighting the main food products frequently contaminated with these alkaloids and allowing to get up-to-date on the analytical methodologies developed for their determination in these food matrices within this period. Special emphasis will made on those recent strategies that allow the simultaneous determination of these two types of alkaloids, as well as on those works that have developed novel and sustainable microextraction procedures as emergent approaches in this field. Likewise, challenges and expected future trends are also included. Overall, this review intents to succinctly highlight the importance of these current food safety issue.
Figure 1C shows the evolution of number of food alerts notified in the EU related to the occurrence of TAs and PAs in food products from 2020 to 2023, including the type of product in which these toxins have been detected. It is observed food alerts for PAs are greater than for TAs. Regulation for PAs in Europe started before than that of TAs, so it is possible there has been greater control of these alkaloids in recent years. Moreover, the first regulation for TAs contemplated less variety of products than the current one. Nonetheless, the number of alerts has increased significantly for both toxins in the last year 2023. This may be due to the entry into force of the new EU regulation for these contaminants, requiring more surveillance in a wider variety of foodstuffs (European Union, 2024).
As shown in Figure 1C, the occurrence of these toxins at high levels has primarily been notified for plant-derived products (except honey), since plants are the main source of these alkaloids. Among these plant-derived products, herbs and spices are the products with the highest degree of contamination of both toxins, followed by teas and herbal infusions. Alerts in cereals and bakery products, as well as snacks, have only been reported for the presence of TAs, while alerts in food supplements, honey and pollen exclusively correspond to PAs. In the case of fruits and vegetables, one food alert corresponding to the presence of TAs was reported in 2021, however, in 2023, several alerts have been notified due to the presence of PAs in these products. The main analytical advances carried out in these food categories for the determination of these alkaloids within the period 2020–2023 are reviewed below. It is worth mentioning that liquid chromatography (LC) or its improved variant ultra-high-performance LC (UHPLC) coupled to mass spectrometry (MS) have been mainly used for the analysis using C18 separation columns and electrospray ionization (ESI) in positive ion mode.
Among this food category, food alerts notified in the last years are predominantly linked to high levels of PAs found in oregano and cumin. In contrast, the occurrence of TAs has been basically notified in savory, parsley and peppermint (Rapid Alert System for Food and Feed, 2023). The main contamination pathway of these products with these two alkaloids is during cultivation and harvesting. Frequently, many PAs- and TAs-producing plants grow as weeds among crop fields, so cross-contamination can occur (Casado et al., 2022a; González-Gómez et al., 2022a; Casado et al., 2024a). Likewise, different studies have demonstrated the natural horizontal transfer of these alkaloids through soil as another important contamination route (Nowak et al., 2016; Nowak et al., 2017; Selmar et al., 2019; Chmit et al., 2021; Letsyo et al., 2021; Jiao et al., 2022; Jiao et al., 2023a). On the other hand, in some cases, the occurrence of these toxins could also be due to intentional adulteration by using undisclosed herbs to gain economic advantage. In fact, oregano is one of the most adulterated herbs (Maquet et al., 2021). Due to the high frequency of contamination of these products with TAs and PAs, different authors have developed several analytical procedures for the determination of these alkaloids in aromatic herbs and spices (Kaltner et al., 2020a; Izcara et al., 2020; Kaczyński and Łozowicka, 2020; González-Gómez et al., 2022b; Izcara et al., 2022; González-Gómez et al., 2023a; Peloso et al., 2023). For instance, in the case of TAs, extraction has been performed with acidic water solution (1.1.% HCl) followed by solid-phase extraction (SPE) (González-Gómez et al., 2022b; González-Gómez et al., 2023a). Different novel sorbents based on mesostructured silicas modified with sulfonic groups have been developed for this purpose. The results obtained in these works revealed coriander, basil, fennel and cloves as the products with the highest concentrations of TAs (González-Gómez et al., 2022b; González-Gómez et al., 2023a). For PAs, acidic solvent extraction with 0.05 M H2SO4 followed by SPE with cation-exchange sorbents (e.g., SCX and MCX) has been used as sample treatment (Kaltner et al., 2020a; Peloso et al., 2023). However, besides SPE, other techniques such as QuEChERS have successfully been applied to the analysis of PAs in herbs and spices (Izcara et al., 2020; Kaczyński and Łozowicka, 2020; Izcara et al., 2022). In this context, it is worth highlighting those works which have miniaturized the QuEChERS procedure up to 10 times and applied it to the analysis of oregano and other aromatic herbs using PSA or mesostructured silicas as clean-up sorbents (Izcara et al., 2020; Izcara et al., 2022). This miniaturization has allowed improving the QuEChERS strategy, leading to a more sustainable and green method. Nonetheless, despite the technique employed, PA levels exceeding the maximum limit established by regulation for aromatic herbs have been detected in these works, namely, in oregano, marjoram, cumin, thyme, parsley, savory, dill and chive (Kaltner et al., 2020a; Izcara et al., 2020; Kaczyński and Łozowicka, 2020; Izcara et al., 2022; Peloso et al., 2023).
Within the period reviewed, food alerts have only been reported for the presence of TAs in this type of products. Like herbs and spices, the contamination is mainly due to cross-contamination during cultivation and harvesting or by natural horizontal transfer. However, these contamination routes are also possible in the case of PAs. Therefore, although no PA alerts have been reported in these products, some works have also addressed the determination of PAs in cereal samples, even in some cases simultaneously together with the analysis of TAs (Dzuman et al., 2020; Bessaire et al., 2021; Letsyo et al., 2021; García-Juan et al., 2023; Rollo et al., 2023). Thus, among the different strategies developed for the analysis of TAs and PAs in these food matrices separate or together, the most common sample preparation procedure has been acidic aqueous solvent extraction followed in some cases by cation-exchange SPE or the QuEChERS method (Basle et al., 2020; Dzuman et al., 2020; González-Gómez et al., 2020; Bessaire et al., 2021; Letsyo et al., 2021; Rausch et al., 2021; Vuković et al., 2022; García-Juan et al., 2023; Rollo et al., 2023). Regarding TAs, atropine and scopolamine ranged between 2.6 and 58.80 μg/kg in different cereal-based products (e.g., teff, buckwheat, sorghum, rice, semolina, corn grits and polenta) (Basle et al., 2020; González-Gómez et al., 2020; Rausch et al., 2021; Vuković et al., 2022). In all cases, contamination by atropine was greater than that by scopolamine. Likewise, scopoline, a low molecular weight TA, was detected in a quinoa sample at 38 μg/kg (Bessaire et al., 2021). However, data of low molecular weight TAs in foods is currently very scarce. On the other hand, trace amounts of PAs were found in maize grown on fields previously dominated with a PA-producing plant (Chromolaena odorata) (Letsyo et al., 2021) and 3 PAs were detected in a multigrain baby food sample (García-Juan et al., 2023). Thus, these results suggest the need to also explore the occurrence of PAs in cereal-based products.
These products have also caused many of the food alerts reported for both types of alkaloids. Like herbs and spices, teas and herbal teas are susceptible to contamination due to co-harvesting and natural horizontal transfer. For the determination of these compounds, different strategies have been addressed. On the one hand, some authors have determined the presence of these alkaloids in the dry product (Kwon et al., 2021; Han et al., 2022a; Han et al., 2022b; Jansons et al., 2022; León et al., 2022; Luo et al., 2022; Jiao et al., 2023b; Gumus, 2023; Mateus et al., 2023; Peloso et al., 2023). For PAs, many authors have performed and acidic solvent extraction with H2SO4 (0.05–0.1 M) followed by SPE purification with MCX cartridges or dispersive clean-up with GCB, PSA and C18 sorbents (Kwon et al., 2021; Han et al., 2022a; Han et al., 2022b; Jiao et al., 2023b; Peloso et al., 2023). Likewise, QuEChERS has been used for both PAs and TAs (Han et al., 2022b; Jansons et al., 2022; León et al., 2022; Jiao et al., 2023b; Mateus et al., 2023). Regarding miniaturization, alkaline organic extraction followed by solid-phase microextraction (SPME) using molecularly imprinted fibers with cation-exchange interaction was employed for selective recognition of 10 PAs from scented teas (Luo et al., 2022). On the other hand, other works have directly performed the analysis in the prepared infusion samples (Casado et al., 2022b; Han et al., 2022b; González-Gómez et al., 2022c; Martinello et al., 2022; Rizzo et al., 2023a; González-Gómez et al., 2023b; Fernández-Pintor et al., 2023). Analyzing the infusion seems more suitable to avoid overestimation of the real intake and exposure of consumers to these toxins through these beverages, since transfer rate of these alkaloids during brewing is not always complete (Casado et al., 2023). Accordingly, some recent works have assessed the transfer rate of these alkaloids in addition to estimating their content in the product. In these cases, after preparing the infusions, the aqueous extracts have been purified before chromatographic analysis by using different techniques, such as SPE, salting-out assisted liquid-liquid extraction (SALLE) and µSPEed (Casado et al., 2022b; Han et al., 2022b; González-Gómez et al., 2022c; Martinello et al., 2022; Rizzo et al., 2023a; González-Gómez et al., 2023b; Fernández-Pintor et al., 2023). It is worth highlighting this last technique, which it is an improved high-resolution miniaturized SPE that provides more efficiency and faster procedures at a lower overall cost. This miniaturized approach has proved its green potential for both the analysis of PAs and TAs in infusion samples by minimal use of sample, sorbents and solvents (Casado et al., 2022b; González-Gómez et al., 2022c; González-Gómez et al., 2023b; Fernández-Pintor et al., 2023).
In 2021, an alert related to the contamination of deep-frozen spinach puree with high concentrations (>1,000 μg/kg) of atropine and scopolamine was notified (Rapid Alert System for Food and Feed, 2023). The occurrence of TAs in spinach products can be due to the similarity with Datura innoxia (TA-producing plant) leaves. Likewise, this may also happen in the case of PAs, since the leaves of arugula or rocket are very similar to the leaves of Senecio vulgaris (PA-producing plant). Thus, contamination with toxins due to co-harvesting or natural horizontal transfer is highly possible in vegetables, especially in salad mix. On the other hand, all the alerts related to PAs in vegetables have been reported in 2023, specifically in lovage leaf, chives and borage (Rapid Alert System for Food and Feed, 2023). Nonetheless, it is worth noting that borage is a PA-producing plant, which it is intentionally consumed despite its content in PAs. Regarding the literature, the analysis of these alkaloids in vegetable samples is scarce. For instance, TAs have been determined in spinach-based products using QuEChERS (Castilla-Fernández et al., 2021). Atropine and scopolamine were found in 16 out of 66 samples, with concentrations between 0.02 and 8.19 μg/kg, but only one sample exceed the maximum limit established by the EU. More recently, the miniaturized version of QuEChERS has been applied to the determination of TAs in leafy vegetable samples, achieving a greener procedure with reduced amount of samples, solvents and salts without losing extraction efficiency (González-Gómez et al., 2022d). Mixed leafy vegetables were contaminated in a controlled manner with TA-producing plants simulating a real cross-contamination to assess the efficiency of the method and it was then applied to 18 commercial samples. Atropine was detected in 12 samples, whereas scopolamine was detected only in one, with concentrations of 2.7–3.4 μg/kg. In the case of PAs, they have been determined in borage leaves using solvent extraction with 0.05 M H2SO4 followed by SPE with MCX cartridges (Peloso et al., 2023). As expected, all borage samples presented PAs (mean concentration 530.8 μg/kg), and only in one the content (3410 μg/kg) exceeded the maximum limit (750 μg/kg).
In the last 3 years, food alerts related to these products have only been notified for high levels of PAs found in pollen (Rapid Alert System for Food and Feed, 2023). Nonetheless, within this period, many authors have addressed the determination of TAs and PAs in honey and pollen samples (Gottschalk et al., 2020; He et al., 2020; Moreira et al., 2020; Romera-Torres et al., 2020; Thompson et al., 2020; Bandini and Spisso, 2021; Valese et al., 2021; Schlappack et al., 2022a; Schlappack et al., 2022b; Guo et al., 2022; Jansons et al., 2022; Kowalczyk and Kwiatek, 2022; Kwon et al., 2022; Martinello et al., 2022; Rizzo et al., 2022; Rizzo et al., 2023a; Peloso et al., 2023; Roncada et al., 2023; Casado et al., 2024b). The results achieved in these works have revealed these products are frequently contaminated with these toxins, detecting levels of PAs and TAs in wide range (0.012–3313 μg/kg). In PA- and TA-producing plants, these alkaloids can be present in flower nectar. Consequently, contamination of pollen and honey with these toxins mainly happens during bee forage. Pollen is normally more contaminated than honey since it is closely related to the plant materials. In contrast, the occurrence of PAs and TAs in honey is due to the accidental or deliberate introduction of pollen in this matrix. For the extraction of these toxins from this type of matrices, most authors have first dissolved the samples in 0.05 M H2SO4, since it helps to release the analytes from the matrix and decrease the sample viscosity. Afterwards, for sample purification, different conventional sample preparation procedures have been employed, being SPE the most widely used, followed by QuEChERS (Gottschalk et al., 2020; He et al., 2020; Moreira et al., 2020; Romera-Torres et al., 2020; Schlappack et al., 2022a; Schlappack et al., 2022b; Guo et al., 2022; Jansons et al., 2022; Kowalczyk and Kwiatek, 2022; Kwon et al., 2022; Martinello et al., 2022; Peloso et al., 2023; Roncada et al., 2023). For SPE, cartridges with cation-exchange sorbents (e.g., MCX, SCX) have been preferred. Accordingly, some authors have developed and applied different sulfonated halloysite nanotubes as novel cation-exchange SPE sorbents for this purpose (Schlappack et al., 2022a; Schlappack et al., 2022b). On the other hand, liquid-liquid extraction (LLE) and SALLE have also been explored for the extraction of TAs and PAs, respectively (Thompson et al., 2020; Rizzo et al., 2022; Rizzo et al., 2023a). Regarding miniaturization, a recent work proposed the green simultaneous microextraction of TAs and PAs from honey by µSPEed® in just 3 min (Casado et al., 2024b). Nonetheless, some authors have avoided sample purification by directly dissolving the sample in water o aqueous ammonium hydroxide and injecting it into the chromatographic system (Bandini and Spisso, 2021; Valese et al., 2021). However, this leads to higher matrix interferences that can damage the ionization source in mass spectrometry detection. Likewise, this does not allow preconcentration, what may hinder achieving the sensitivity set by legislation for the analysis of these compounds.
There have not been many food alerts in this category within the last 3 years, only 2 in 2023 regarding the occurrence of PAs in Tribulus terrestris and Ginkgo biloba extracts (Rapid Alert System for Food and Feed, 2023). In this period, some authors have proposed strategies for the analysis of PAs in plant-based and honey-derived food supplements (Kaltner et al., 2020b; Picron et al., 2020; Chen et al., 2021; Rizzo et al., 2023a; Rizzo et al., 2023b). In these works, a first solvent extraction with acidic aqueous solution with 0.05 M H2SO4 has been performed followed by a purification step by SPE with cation-exchange sorbents or SALLE prior to chromatographic analysis. Nonetheless, Chen et al. (2021) carried a different approach by performing first an aqueous solvent extraction of the samples with 0.1% formic acid followed by basification of the extract and LLE with dichloromethane. The organic phase was then recovered, evaporated and re-dissolved in methanol:water (1:1, v/v) for direct analysis in real-time coupled to MS (DART-MS) (Chen et al., 2021). Nonetheless, a smaller number of PAs were assessed in the samples compared to the previous works with chromatographic separation.
In 2022, only 3 alerts were reported for TAs in this type of products, specifically in corn tortilla chips (Rapid Alert System for Food and Feed, 2023). However, no works analyzing this type of snack have been found in the literature. Nonetheless, TAs have recently been determined in corn puffs (popular extruded snacks made of cornmeal) by QuEChERS (Stojanović et al., 2021). Only atropine was quantified in 22% of the samples analyzed, but although scopolamine was not quantified, it was also detected in 4 samples. It is worth mentioning that authors highlighted the importance of knowing whether TAs were lost during the extrusion process. The effect of food processing on these toxins is also a relevant issue scarcely studied (Casado et al., 2023). Accordingly, another research has evaluated the thermal degradation of TAs in gluten-free corn breadsticks under different conditions (Vera-Baquero et al., 2022). The results obtained indicated degradation of TAs can be up to 65% during the baking process depending on the conditions used. Likewise, atropine and scopolamine have been detected in popcorn samples in a range of 5.3–28.0 and 2.1–6.3 μg/kg, respectively (Vuković et al., 2022). In the case of PAs, they have been analyzed in honey-based candies and snacks (honey breakfast cereals, honey/cereal bars and gingerbreads) (Picron et al., 2020). The extraction was performed with 0.05 M H2SO4 followed by SPE with SCX cartridges. Only one-third of the snacks were contaminated, with a maximum level of 0.36 μg/kg, and all the cereal bars were free of PAs. Regarding candies, 54% of the samples showed PA contamination. Nonetheless, the authors mainly attributed the occurrence of PAs in the snacks and candies to the presence of honey as ingredient.
Table 1 summarizes all the analytical methods developed in the last 3 years that addressed the simultaneous determination of TAs and PAs. These works have mainly been developed for food matrices with high probability of being contaminated with both toxins because of their similar contamination pathways (i.e., herbs and spices, teas and herbal infusions, cereals, honey and pollen). Among the sample preparation techniques, QuEChERS has been the most widely used, since is a very effective procedure when it is necessary to simultaneously extract many analytes of different nature. Nonetheless, other techniques such as SPE, dispersive SPE, solid-liquid extraction or µSPEed have also been applied (Table 1). Regarding the analytical technique, all works have used LC or UHPLC coupled to MS. Accordingly, different analyzers have been used, being the most common the triple quadrupole and those based on the combination of quadrupole and linear ion trap (e.g., Q-Orbitrap or Q-TRAP). Likewise, the ionization source has been in all cases ESI in positive ion mode. For the chromatographic separation different types of C18 columns have been used at a temperature range between 30–50°C. In general, the analysis time in most works ranged between 15 and 20 min. However, in those works using LC instead of UHPLC the analysis time is generally higher, exceeding 1 h in some cases (Table 1). In contrast, it is worth highlighting the work of Dzuman et al. (2020), which achieved the separation of 54 compounds in 22 min (Dzuman et al., 2020).
Table 1. Analytical methods published for the simultaneous determination of tropane and pyrrolizidine alkaloids in food samples (2020–2023).
Currently, the occurrence of natural toxins is a food safety topic of great relevance. In recent years, the number of alerts notified for TAs and PAs has increased. However, this may be a consequence of greater food control. To date, the strategies carried out for the determination of these alkaloids are mainly based on acid solvent extraction followed by SPE purification with cation-exchange sorbents, or the QuEChERS procedure. However, future works should make efforts to develop green procedures by employing miniaturized alternatives of these techniques to improve sustainability and optimize resources, including the simultaneous analysis of these two types of alkaloids. Likewise, future works should focus on the effect of food processing on these alkaloids, since it is a field scarcely explored. Thus, the evaluation of degradation/transformation products constitutes a research line with interesting future challenges.
NC: Conceptualization, Data curation, Formal Analysis, Funding acquisition, Investigation, Methodology, Project administration, Resources, Software, Visualization, Writing–original draft, Writing–review and editing. JG: Conceptualization, Data curation, Formal Analysis, Funding acquisition, Investigation, Methodology, Project administration, Resources, Software, Visualization, Writing–original draft, Writing–review and editing. SM-Z: Supervision, Visualization, Writing–review and editing. IS: Funding acquisition, Project administration, Resources, Supervision, Writing–review and editing.
The author(s) declare that financial support was received for the research, authorship, and/or publication of this article. This research was funded by MCIN/AEI/10.13039/501100011033/FEDER, UE, grant number PID 2022-137278OB-I00 (EVALKALIM-II) and by the call Proyectos de Impulso a la Investigación para Jóvenes Doctores de la Universidad Rey Juan Calos, projects PROCESALK (ref. M2984) and ALKASENS (ref. M2987).
The authors declare that the research was conducted in the absence of any commercial or financial relationships that could be construed as a potential conflict of interest.
All claims expressed in this article are solely those of the authors and do not necessarily represent those of their affiliated organizations, or those of the publisher, the editors and the reviewers. Any product that may be evaluated in this article, or claim that may be made by its manufacturer, is not guaranteed or endorsed by the publisher.
Bandini, T. B., and Spisso, B. F. (2021). Development and validation of an LC-HRMS method for the determination of pyrrolizidine alkaloids and quinolones in honey employing a simple alkaline sample dilution. J. Food Meas. Charact. 15, 4758–4770. doi:10.1007/s11694-021-01048-9
Basle, Q., Mujahid, C., and Bessaire, T. (2020). Application of a streamlined LC-MS/MS methodology for the determination of atropine and scopolamine in cereals from Asian and African countries. Food Addit. Contam. Part A 37 (10), 1744–1754. doi:10.1080/19440049.2020.1800828
Bessaire, T., Ernest, M., Christinat, N., Carrères, B., Panchaud, A., and Badoud, F. (2021). High resolution mass spectrometry workflow for the analysis of food contaminants: application to plant toxins, mycotoxins and phytoestrogens in plant-based ingredients. Food Addit. Contam. Part A 38, 978–996. doi:10.1080/19440049.2021.1902575
Casado, N., Casado-Hidalgo, G., González-Gómez, L., Morante-Zarcero, S., and Sierra, I. (2023). Insight into the impact of food processing and culinary preparations on the stability and content of plant alkaloids considered as natural food contaminants. Appl. Sci. 13 (3), 1704. doi:10.3390/app13031704
Casado, N., Fernández-Pintor, B., Morante-Zarcero, S., and Sierra, I. (2022b). Quick and green microextraction of pyrrolizidine alkaloids from infusions of mallow, calendula, and Hibiscus flowers using ultrahigh-performance liquid chromatography coupled to tandem mass spectrometry analysis. J. Agric. Food Chem. 70, 7826–7841. doi:10.1021/acs.jafc.2c02186
Casado, N., Gañán, J., Morante-Zarcero, S., and Sierra, I. (2024a). “Occurrence of pyrrolizidines and other alkaloids of plant origin in foods,” in Encyclopedia of food safety. Editor G. W. Smithers (Elsevier, Academic Press), 1, 518–528. doi:10.1016/B978-0-12-822521-9.00184-2
Casado, N., Morante-Zarcero, S., and Sierra, I. (2022a). The concerning food safety issue of pyrrolizidine alkaloids: an overview. Trends Food Sci. Technol. 120, 123–139. doi:10.1016/j.tifs.2022.01.007
Casado, N., Morante-Zarcero, S., and Sierra, I. (2024b). Miniaturized analytical strategy based on μ SPEed for monitoring the occurrence of pyrrolizidine and tropane alkaloids in honey. J. Agric. Food Chem. 72, 819–832. (in press). doi:10.1021/acs.jafc.3c04805
Castilla-Fernández, D., Moreno-González, D., García-Reyes, J. F., Ballesteros, E., and Molina-Díaz, A. (2021). Determination of atropine and scopolamine in spinach-based products contaminated with genus Datura by UHPLC–MS/MS. Food Chem. 347, 129020. doi:10.1016/j.foodchem.2021.129020
Chen, Y., Li, L., Xiong, F., Xie, Y., Xiong, A., Wang, Z., et al. (2021). Rapid identification and determination of pyrrolizidine alkaloids in herbal and food samples via direct analysis in real-time mass spectrometry. Food Chem. 334, 127472. doi:10.1016/j.foodchem.2020.127472
Chmit, M. S., Horn, G., Dübecke, A., and Beuerle, T. (2021). Pyrrolizidine alkaloids in the food chain: is horizontal transfer of natural products of relevance? Foods 10 (8), 1827. doi:10.3390/foods10081827
Commission implementing regulation (2024). Commission implementing regulation (EU) 2023/2783 of 14 December 2023 laying down the methods of sampling and analysis for the control of the levels of plant toxins in food and repealing Regulation (EU) 2015/705.
Dzuman, Z., Jonatova, P., Stranska-Zachariasova, M., Prusova, N., Brabenec, O., Novakova, A., et al. (2020). Development of a new LC-MS method for accurate and sensitive determination of 33 pyrrolizidine and 21 tropane alkaloids in plant-based food matrices. Anal. Bioanal. Chem. 412, 7155–7167. doi:10.1007/s00216-020-02848-6
European Union (2021). Commission regulation (EU) 2021/1408 of 27 August 2021 amending Regulation (EC) No 1881/2006 as regards maximum levels of tropane alkaloids in certain foodstuffs. Off. J. Eur. Union, 1–4.
European Union (2023). Commission Regulation (EU) 2020/2040 of 11 December 2020 amending Regulation (EC) No 1881/2006 as regards maximum levels of pyrrolizidine alkaloids in certain foodstuffs (Text with EEA relevance) (OJ L 420 14.12.2020, p. 1). Available online: http://data.europa.eu/eli/reg/2020/2040/oj (accessed on December 13, 2023).
European Union (2024). Commission regulation (EU) 2023/915 of 25 April 2023 on maximum levels for certain contaminants in food and repealing Regulation (EC) No 1881/2006.
Fernández-Pintor, B., Casado, N., Morante-Zarcero, S., and Sierra, I. (2023). Evaluation of the thermal stability and transfer rate of pyrrolizidine alkaloids during the brewing of herbal infusions contaminated with Echium vulgare and Senecio vulgaris weeds. Food control. 153, 109926. doi:10.1016/j.foodcont.2023.109926
García-Juan, A., León, N., Armenta, S., and Pardo, O. (2023). Development and validation of an analytical method for the simultaneous determination of 12 ergot, 2 tropane, and 28 pyrrolizidine alkaloids in cereal-based food by LC-MS/MS. Food Res. Int. 174, 113614. doi:10.1016/j.foodres.2023.113614
González-Gómez, L., Gañán, J., Morante-Zarcero, S., Pérez-Quintanilla, D., and Sierra, I. (2020). Sulfonic acid-functionalized SBA-15 as strong cation-exchange sorbent for Solid-Phase Extraction of atropine and scopolamine in gluten-free grains and flours. Foods 9 (12), 1854. doi:10.3390/foods9121854
González-Gómez, L., Gañán, J., Morante-Zarcero, S., Pérez-Quintanilla, D., and Sierra, I. (2022b). Mesostructured silicas as cation-exchange sorbents in packed or dispersive solid phase extraction for the determination of tropane alkaloids in culinary aromatics herbs by HPLC-MS/MS. Toxins 14 (3), 218. doi:10.3390/toxins14030218
González-Gómez, L., Gañán, J., Morante-Zarcero, S., Pérez-Quintanilla, D., and Sierra, I. (2023a). Atropine and scopolamine occurrence in spices and fennel infusions. Food control. 146, 109555. doi:10.1016/j.foodcont.2022.109555
González-Gómez, L., Morante-Zarcero, S., Pereira, J. A., Câmara, J. S., and Sierra, I. (2022d). Improved analytical approach for determination of tropane alkaloids in leafy vegetables based on µ-QuEChERS combined with HPLC-MS/MS. Toxins 14 (10), 650. doi:10.3390/toxins14100650
González-Gómez, L., Morante-Zarcero, S., Pereira, J. A., Câmara, J. S., and Sierra, I. (2023b). Evaluation of tropane alkaloids in teas and herbal infusions: effect of brewing time and temperature on atropine and scopolamine content. Toxins 15 (6), 362. doi:10.3390/toxins15060362
González-Gómez, L., Morante-Zarcero, S., Pérez-Quintanilla, D., and Sierra, I. (2022a). Occurrence and chemistry of tropane alkaloids in foods, with a focus on sample analysis methods: a review on recent trends and technological advances. Foods 11 (3), 407. doi:10.3390/foods11030407
González-Gómez, L., Pereira, J. A., Morante-Zarcero, S., Câmara, J. S., and Sierra, I. (2022c). Green extraction approach based on μSPEed® followed by HPLC-MS/MS for the determination of atropine and scopolamine in tea and herbal tea infusions. Food Chem. 394, 133512. doi:10.1016/j.foodchem.2022.133512
Gottschalk, C., Kaltner, F., Zimmermann, M., Korten, R., Morris, O., Schwaiger, K., et al. (2020). Spread of Jacobaea vulgaris and occurrence of pyrrolizidine alkaloids in regionally produced honeys from Northern Germany: inter-and intra-site variations and risk assessment for special consumer groups. Toxins 12, 441. doi:10.3390/toxins12070441
Gumus, Z. P. (2023). Assessment of toxic pyrrolizidine and tropane alkaloids in herbal teas and culinary herbs using LC-Q-ToF/MS. Foods 12 (19), 3572. doi:10.3390/foods12193572
Guo, Q., Yang, Y., Li, J., Shao, B., and Zhang, J. (2022). Screening for plant toxins in honey and herbal beverage by ultrahigh-performance liquid chromatography-ion mobility-quadrupole time of flight mass spectrometry. Am. J. Anal. Chem. 13, 108–134. doi:10.4236/ajac.2022.133009
Han, H., Jiang, C., Wang, C., Lu, Y., Wang, Z., Chai, Y., et al. (2022b). Dissipation pattern and conversion of pyrrolizidine alkaloids (PAs) and pyrrolizidine alkaloid N-oxides (PANOs) during tea manufacturing and brewing. Food Chem. 390, 133183. doi:10.1016/j.foodchem.2022.133183
Han, H., Jiang, C., Wang, C., Wang, Z., Chai, Y., Zhang, X., et al. (2022a). Development, optimization, validation and application of ultra high performance liquid chromatography tandem mass spectrometry for the analysis of pyrrolizidine alkaloids and pyrrolizidine alkaloid N-oxides in teas and weeds. Food control. 132, 108518. doi:10.1016/j.foodcont.2021.108518
He, Y., Zhu, L., Ma, J., Wong, L., Zhao, Z., Ye, Y., et al. (2020). Comprehensive investigation and risk study on pyrrolizidine alkaloid contamination in Chinese retail honey. Environ. Pollut. 267, 115542. doi:10.1016/j.envpol.2020.115542
Izcara, S., Casado, N., Morante-Zarcero, S., Pérez-Quintanilla, D., and Sierra, I. (2022). Miniaturized and modified QuEChERS method with mesostructured silica as clean-up sorbent for pyrrolizidine alkaloids determination in aromatic herbs. Food Chem. 380, 132189. doi:10.1016/j.foodchem.2022.132189
Izcara, S., Casado, N., Morante-Zarcero, S., and Sierra, I. (2020). A miniaturized QuEChERS method combined with ultrahigh liquid chromatography coupled to tandem mass spectrometry for the analysis of pyrrolizidine alkaloids in oregano samples. Foods 9, 1319. doi:10.3390/foods9091319
Jansons, M., Fedorenko, D., Pavlenko, R., Berzina, Z., and Bartkevics, V. (2022). Nanoflow liquid chromatography mass spectrometry method for quantitative analysis and target ion screening of pyrrolizidine alkaloids in honey, tea, herbal tinctures, and milk. J. Chromatogr. A 1676, 463269. doi:10.1016/j.chroma.2022.463269
Jiao, W., Shen, T., Wang, L., Zhu, L., Li, Q. X., Wang, C., et al. (2022). Source and route of pyrrolizidine alkaloid contamination in tea samples. J. Vis. Exp. JoVE (187), e64375. doi:10.3791/64375
Jiao, W., Zhu, L., Li, Q. X., Shi, T., Zhang, Z., Wu, X., et al. (2023a). Pyrrolizidine alkaloids in tea (camellia sinensis L.) from weeds through weed–soil–tea transfer and risk assessment of tea intake. J. Agric. Food Chem. 71, 19045–19053. doi:10.1021/acs.jafc.3c04339
Jiao, W., Zhu, L., Li, Q. X., Shi, T., Zhang, Z., Wu, X., et al. (2023b). Pyrrolizidine alkaloids in tea (camellia sinensis L.) from weeds through weed–soil–tea transfer and risk assessment of tea intake. J. Agric. Food Chem. 71, 19045–19053. doi:10.1021/acs.jafc.3c04339
Kaczyński, P., and Łozowicka, B. (2020). A novel approach for fast and simple determination pyrrolizidine alkaloids in herbs by ultrasound-assisted dispersive solid phase extraction method coupled to liquid chromatography–tandem mass spectrometry. J. Pharm. Biomed. Analysis 187, 113351. doi:10.1016/j.jpba.2020.113351
Kaltner, F., Kukula, V., and Gottschalk, C. (2020b). Screening of food supplements for toxic pyrrolizidine alkaloids. J. Consumer Prot. Food Saf. 15, 237–243. doi:10.1007/s00003-020-01296-9
Kaltner, F., Rychlik, M., Gareis, M., and Gottschalk, C. (2020a). Occurrence and risk assessment of pyrrolizidine alkaloids in spices and culinary herbs from various geographical origins. Toxins 12, 155. doi:10.3390/toxins12030155
Kowalczyk, E., and Kwiatek, K. (2022). Simultaneous determination of pyrrolizidine and tropane alkaloids in honey by liquid chromatography–mass spectrometry. J. Veterinary Res. 66 (2), 235–243. doi:10.2478/jvetres-2022-0032
Kwon, Y., Gu, Y., and Jeong, Y. (2022). Evaluation of pyrrolizidine alkaloids in Korean commercial honeys and bee pollens. Food Sci. Technol. Res. 28 (2), 123–132. doi:10.3136/fstr.FSTR-D-21-00221
Kwon, Y., Koo, Y., and Jeong, Y. (2021). Determination of pyrrolizidine alkaloids in teas using liquid chromatography–tandem mass spectrometry combined with rapid-easy extraction. Foods 10, 2250. doi:10.3390/foods10102250
León, N., Miralles, P., Yusà, V., and Coscollà, C. (2022). A green analytical method for the simultaneous determination of 30 tropane and pyrrolizidine alkaloids and their N-oxides in teas and herbs for infusions by LC-Q-Orbitrap HRMS. J. Chromatogr. A 1666, 462835. doi:10.1016/j.chroma.2022.462835
Letsyo, E., Adams, Z. S., Dzikunoo, J., and Asante-Donyinah, D. (2021). Uptake and accumulation of pyrrolizidine alkaloids in the tissues of maize (Zea mays L.) plants from the soil of a 4-year-old Chromolaena odorata dominated fallow farmland. Chemosphere 270, 128669. doi:10.1016/j.chemosphere.2020.128669
Luo, Z., Chen, X., Ma, Y., Yang, F., He, N., Yu, L., et al. (2022). Multi-template imprinted solid-phase microextraction coupled with UPLC-Q-TOF-MS for simultaneous monitoring of ten hepatotoxic pyrrolizidine alkaloids in scented tea. Front. Chem. 10, 1048467. doi:10.3389/fchem.2022.1048467
Maquet, A., Lievens, A., Paracchini, V., Kaklamanos, G., De La Calle Guntinas, M. B., Garlant, L., et al. (2021). Results of an EU wide coordinated control plan to establish the prevalence of fraudulent practices in the marketing of herbs and spices, EUR 30877 EN. Luxembourg: Publications Office of the European Union. 978-92-76-42979-1. doi:10.2760/309557
Martinello, M., Manzinello, C., Gallina, A., and Mutinelli, F. (2022). In-house validation and application of UHPLC-MS/MS method for the quantification of pyrrolizidine and tropane alkaloids in commercial honey bee-collected pollen, teas and herbal infusions purchased on Italian market in 2019–2020 referring to recent European Union regulations. Int. J. Food Sci. Technol. 57, 7505–7516. doi:10.1111/ijfs.15567
Mateus, A. R. S., Crisafulli, C., Vilhena, M., Barros, S. C., Pena, A., and Sanches Silva, A. (2023). The bright and dark sides of herbal infusions: assessment of antioxidant capacity and determination of tropane alkaloids. Toxins 15 (4), 245. doi:10.3390/toxins15040245
Moreira, R., Fernandes, F., Valentão, P., Pereira, D. M., and Andrade, P. B. (2020). Echium plantagineum L. honey: search of pyrrolizidine alkaloids and polyphenols, anti-inflammatory potential and cytotoxicity. Food Chem. 328, 127169. doi:10.1016/j.foodchem.2020.127169
Nowak, M., Wittke, C., Lederer, I., Klier, B., Kleinwächter, M., and Selmar, D. (2016). Interspecific transfer of pyrrolizidine alkaloids: an unconsidered source of contaminations of phytopharmaceuticals and plant derived commodities. Food Chem. 213, 163–168. doi:10.1016/j.foodchem.2016.06.069
Nowak, M., Yahyazadeh, M., Lewerenz, L., and Selmar, D. (2017). Horizontal natural product transfer: a so far unconsidered source of contamination of medicinal plants. Med. Plants Environ. Challenges, 215–225. doi:10.1007/978-3-319-68717-9_12
Peloso, M., Minkoumba Sonfack, G., Paduano, S., De Martino, M., De Santis, B., and Caprai, E. (2023). Pyrrolizidine alkaloids in food on the Italian market. Molecules 28 (14), 5346. doi:10.3390/molecules28145346
Picron, J. F., Herman, M., Van Hoeck, E., and Goscinny, S. (2020). Monitoring of pyrrolizidine alkaloids in beehive products and derivatives on the Belgian market. Environ. Sci. Pollut. Res. 27 (6), 5693–5708. doi:10.1007/s11356-019-04499-2
Rapid Alert System for Food and Feed (2023). Rapid alert system for food and feed (RASFF) window. Available online: https://webgate.ec.europa.eu/rasff-window/screen/search (accessed on December 7, 2023).
Rausch, A. K., Brockmeyer, R., and Schwerdtle, T. (2021). Development, validation, and application of a multi-method for the determination of mycotoxins, plant growth regulators, tropane alkaloids, and pesticides in cereals by two-dimensional liquid chromatography tandem mass spectrometry. Anal. Bioanal. Chem. 413, 3041–3054. doi:10.1007/s00216-021-03239-1
Rizzo, S., Celano, R., Campone, L., Rastrelli, L., and Piccinelli, A. L. (2022). Salting-out Assisted Liquid-Liquid Extraction for the rapid and simple simultaneous analysis of pyrrolizidine alkaloids and related N-oxides in honey and pollen. J. Food Compos. Analysis 108, 104457. doi:10.1016/j.jfca.2022.104457
Rizzo, S., Celano, R., Piccinelli, A. L., Russo, M., and Rastrelli, L. (2023b). Target screening method for the quantitative determination of 118 pyrrolizidine alkaloids in food supplements, herbal infusions, honey and teas by liquid chromatography coupled to quadrupole orbitrap mass spectrometry. Food Chem. 423, 136306. doi:10.1016/j.foodchem.2023.136306
Rizzo, S., Celano, R., Piccinelli, A. L., Serio, S., Russo, M., and Rastrelli, L. (2023a). An analytical platform for the screening and identification of pyrrolizidine alkaloids in food matrices with high risk of contamination. Food Chem. 406, 135058. doi:10.1016/j.foodchem.2022.135058
Rollo, E., Catellani, D., Dall'Asta, C., and Suman, M. (2023). QuEChERS method combined to liquid chromatography high-resolution mass spectrometry for the accurate and sensitive simultaneous determination of pyrrolizidine and tropane alkaloids in cereals and spices. J. Mass Spectrom. e4969. doi:10.1002/jms.4969
Romera-Torres, A., Romero-González, R., Martínez Vidal, J. L., and Garrido Frenich, A. (2020). Comprehensive tropane alkaloids analysis and retrospective screening of contaminants in honey samples using liquid chromatography-high resolution mass spectrometry (Orbitrap). Food Res. Int. 133, 109130. doi:10.1016/j.foodres.2020.109130
Roncada, P., Isani, G., Peloso, M., Dalmonte, T., Bonan, S., and Caprai, E. (2023). Pyrrolizidine alkaloids from monofloral and multifloral Italian honey. Int. J. Environ. Res. Public Health 20 (7), 5410. doi:10.3390/ijerph20075410
Schlappack, T., Rainer, M., Weinberger, N., and Bonn, G. K. (2022a). Sulfonated halloysite nanotubes as a novel cation exchange material for solid phase extraction of toxic pyrrolizidine alkaloids. Anal. Methods 14, 2689–2697. doi:10.1039/D2AY00614F
Schlappack, T., Weidacher, N., Huck, C. W., Bonn, G. K., and Rainer, M. (2022b). Effective solid phase extraction of toxic pyrrolizidine alkaloids from honey with reusable organosilyl-sulfonated halloysite nanotubes. Separations 9, 270. doi:10.3390/separations9100270
Selmar, D., Wittke, C., Beck-von Wolffersdorff, I., Klier, B., Lewerenz, L., Kleinwächter, M., et al. (2019). Transfer of pyrrolizidine alkaloids between living plants: a disregarded source of contaminations. Environ. Pollut. 248, 456–461. doi:10.1016/j.envpol.2019.02.026
Stojanović, T., Vuković, G., Petrović, A., Konstantinović, B., Puvača, N., Marinković, D., et al. (2021). Determination of tropane alkaloids in corn puffs by the LC-MS/MS. Zb. Matice Srp. za Prir. nauke 141, 69–80. doi:10.2298/ZMSPN2141069S
Thawabteh, A., Juma, S., Bader, M., Karaman, D., Scrano, L., Bufo, S. A., et al. (2019). The biological activity of natural alkaloids against herbivores, cancerous cells and pathogens. Toxins 11 (11), 656. doi:10.3390/toxins11110656
Thompson, T. S., van den Heever, J. P., and Limanowka, R. E. (2020). Hyoscyamine and scopolamine in honey by HILIC–ESI-MS/MS. Chromatographia 83 (5), 683–689. doi:10.1007/s10337-020-03880-5
Valese, A. C., Daguer, H., Muller, C. M. O., Molognoni, L., da Luz, C. F. P., de Barcellos Falkenberg, D., et al. (2021). Quantification of pyrrolizidine alkaloids in Senecio brasiliensis, beehive pollen, and honey by LC-MS/MS. J. Environ. Sci. Health, Part B 56, 685–694. doi:10.1080/03601234.2021.1943257
Vera-Baquero, F. L., Morante-Zarcero, S., and Sierra, I. (2022). Evaluation of thermal degradation of tropane and opium alkaloids in gluten-free corn breadsticks samples contaminated with stramonium seeds and baked with poppy seeds under different conditions. Foods 11 (15), 2196. doi:10.3390/foods11152196
Keywords: natural toxins, alkaloids, food safety, RASFF, food analysis, sample preparation, chromatographic analysis, mass spectrometry
Citation: Casado N, Gañán J, Morante-Zarcero S and Sierra I (2024) Recent food alerts and analytical advances related to the contamination of tropane and pyrrolizidine alkaloids in food. Front. Chem. Biol 3:1360027. doi: 10.3389/fchbi.2024.1360027
Received: 22 December 2023; Accepted: 27 March 2024;
Published: 10 April 2024.
Edited by:
Emmanouil Tsochatzis, European Food Safety Authority (EFSA), ItalyReviewed by:
Mostafa Alilou, Universität Innsbruck, AustriaCopyright © 2024 Casado, Gañán, Morante-Zarcero and Sierra. This is an open-access article distributed under the terms of the Creative Commons Attribution License (CC BY). The use, distribution or reproduction in other forums is permitted, provided the original author(s) and the copyright owner(s) are credited and that the original publication in this journal is cited, in accordance with accepted academic practice. No use, distribution or reproduction is permitted which does not comply with these terms.
*Correspondence: Isabel Sierra, aXNhYmVsLnNpZXJyYUB1cmpjLmVz; Sonia Morante-Zarcero, c29uaWEubW9yYW50ZUB1cmpjLmVz
Disclaimer: All claims expressed in this article are solely those of the authors and do not necessarily represent those of their affiliated organizations, or those of the publisher, the editors and the reviewers. Any product that may be evaluated in this article or claim that may be made by its manufacturer is not guaranteed or endorsed by the publisher.
Research integrity at Frontiers
Learn more about the work of our research integrity team to safeguard the quality of each article we publish.