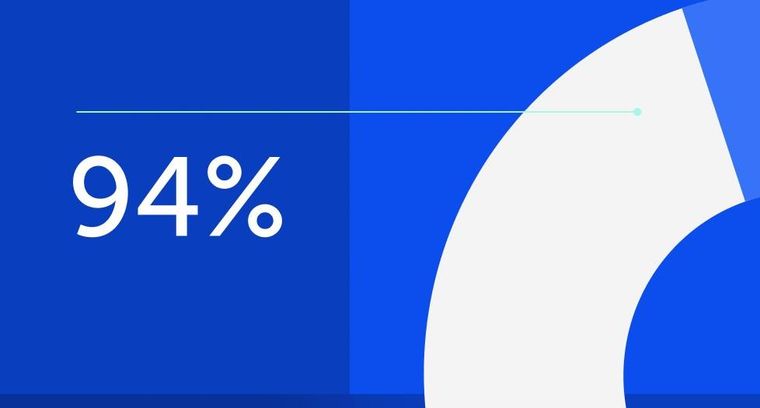
94% of researchers rate our articles as excellent or good
Learn more about the work of our research integrity team to safeguard the quality of each article we publish.
Find out more
MINI REVIEW article
Front. Cell. Neurosci., 05 March 2025
Sec. Cellular Neuropathology
Volume 19 - 2025 | https://doi.org/10.3389/fncel.2025.1547495
This article is part of the Research TopicReviews in Cellular NeuropathologyView all 4 articles
As a transmembrane protein, DPP6 modulates the function and properties of ion channels, playing a crucial role in various tissues, particularly in the brain. DPP6 interacts with potassium channel Kv4.2 (KCND2), enhancing its membrane expression and channel kinetics. Potassium ion channels are critical in progressing action potential formation and synaptic plasticity. Therefore, dysfunction of DPP6 can lead to significant health consequences. Abnormal DPP6 expression has been identified in several diseases, such as amyotrophic lateral sclerosis (ALS), autism spectrum disorder (ASD), spinal bulbar muscular atrophy (SBMA), and idiopathic ventricular fibrillation. Recent research has indicated a connection between DPP6 and Alzheimer’s disease as well. The most common symptoms resulting from DPP6 dysregulation are mental deficiency and muscle wastage. Notably, these symptoms do not always occur at the same time. Besides genetic factors, environmental factors also undoubtedly play a role in diseases related to DPP6 dysregulation. However, it remains unclear how the expression of DPP6 gets regulated. This review aims to summarize the associations between DPP6 and neurological diseases, offering insights as well as proposing hypotheses to elucidate the underlying mechanisms of DPP6 dysregulation.
In mammals, Shal potassium channels (Kv4 channels), including Kv4.1, Kv4.2, and Kv4.3, are transmembrane voltage-gated ion channels characterized by a highly conserved structure. Compared to Kv1, Kv2, and Kv3 channels, Kv4 channels exhibit faster activation and inactivation kinetics, enabling precise temporal control of neuronal excitability. DPP6, a key modulator of Kv4 channels, is predominantly expressed in the brain, underscoring its critical role in regulating these ion channels and contributing significantly to processes such as chronic pain modulation and synaptic integration, which are closely associated with a range of neurological disorders (Birnbaum et al., 2004; Hu et al., 2006; Cai et al., 2004).
The DPP6 protein (Dipeptidyl Peptidase Like 6, DPPX) was initially identified in bovine and rat brains as a transmembrane protein, also expressed in human and other mammalian species. The expression of DPP6 varies across tissues, with the highest levels observed in the brain (Wada et al., 1992; Kin et al., 2001). The intracellular N-terminal residue and the large extracellular domain are connected by the transmembrane domain, which functions as a dimer formed by two monomers, each monomer contains an eight-bladed β-propeller domain and an α/β hydrolase domain (Figure 1) (Strop et al., 2004).
Figure 1. (a) The biological unit structure of DPP6 (DPP6 dimer, PDB ID: 1XFD) (Strop et al., 2004). (b) Structure of human Kv4.2-DPP6S complex (PDB ID: 7E8B) (Kise et al., 2021). Images are produced using Molmil, a WebGL Molecular Viewer developed by PDBj (Bekker et al., 2016, 2022; Kinjo et al., 2018).
The most notable feature of DPP6 is its interaction with the Shal type potassium ion channel Kv4. The presence or absence of the transmembrane helical region of DPP6, as an auxiliary subunit of Kv4.2, directly affects the channel efficiency of Kv4.2 (Boyden et al., 2016; Ye et al., 2022). By associating with Kv4 channels, DPP6 significantly enhances the expression of somatodendritic subthreshold A-type K+ currents (ISA), which are characterized by their rapid activation and inactivation within the subthreshold range of membrane potentials. These currents play a crucial role in shaping synaptic integration and plasticity, the fundamental mechanisms underlying learning and memory (Soh and Goldstein, 2008; Kaulin et al., 2009; Nadin and Pfaffinger, 2013). DPP6-KO mice showed impaired learning and memory abilities, as well as the appearance of aging markers in the brain (Lin et al., 2018, 2020). DPP6 is closely related to early neural development, and hippocampal neurons lacking DPP6 exhibit a decrease in dendritic and spine density (Lin et al., 2013). This suggests that DPP6 may be an important yet unexplored link in ion channel related diseases and neurodevelopmental disorders.
Anti-dipeptidyl-peptidase-like protein 6 encephalitis can lead to rapid progressive dementia (RPD), hippocampal atrophy, and a range of symptoms, including diarrhea and weight loss, hyperexcitability (such as myoclonus, tremor, spasticity, and muscle cramps), cognitive dysfunction (such as memory loss and executive dysfunction), and cerebellar ataxia, among others (Esechie et al., 2023). Loss of function of DPP6 is associated with autosomal dominant microcephaly, which can lead to varying degrees of intellectual disability (Liao et al., 2013).
Since dementia is a hallmark symptom of Alzheimer’s disease (AD), DPP6, as a protein closely associated with learning and memory, could be considered one of many candidate proteins involved in the pathogenesis of AD. In addition to exhibiting impaired hippocampal-dependent learning and memory abilities and reduced brain size, DPP6-KO mice display early-onset deposition of Alzheimer’s disease-related proteins, including amyloid β, α-synuclein, and phosphorylated tau. Moreover, aged DPP6-KO mice exhibit structural degeneration, characterized by reduced brain and hippocampal volume, a decreased total number of neurons, and increase in neuronal loss (Lin et al., 2022). Genetic variations in DPP6, including nonsense and frameshift variants as well as intronic SNPs, are associated with frontotemporal dementia (FTD). These mutations may contribute to reduced DPP6 protein levels, likely due to nonsense-mediated mRNA decay and haploinsufficiency (Cacace et al., 2019; Pottier et al., 2019).
Autism spectrum disorder (ASD) is a neurodevelopmental disorder characterized by deficits in social communication, as well as restricted interests and repetitive behaviors. Additionally, a significant number of individuals with ASD exhibit intellectual disabilities (Bartak and Rutter, 1976). Previous microarray and karyotype analyses have revealed DPP6 abnormalities in ASD patients, suggesting a potential link between DPP6 dysfunction and ASD (Marshall et al., 2008). Among patients with Gilles de la Tourette syndrome (TS) who also have neurodevelopmental disorders, clinical studies have shown that 2.9 to 20% of patients have comorbid ASD, which is higher than the general incidence (Huisman-van Dijk et al., 2016). TS is mainly manifested as tic symptoms, which are believed to be related to dopaminergic regulation of the central nervous system, and DPP6 regulates Kv4 properties, providing a possibly related influencing factor at the molecular level (Robertson et al., 2017). Although there are suspicions that this high probability is due to similar symptoms interfering with diagnosis, mutations lead to downregulating DPP6 expression have indeed been occurred in some TS patients (Darrow et al., 2017; Prontera et al., 2014).
Haloperidol is one of the two drugs approved by the FDA for the treatment of Tourette’s syndrome, and symptoms are significantly reduced in children with autism who receive treatment with Haloperidol (Anderson et al., 1989; Remington et al., 2001; Campbell et al., 1978). Haloperidol is a first-generation typical antipsychotic drug widely used worldwide, which works by blocking dopamine D2 receptors in the brain (Seeman and Tallerico, 1998). It has been reported that long-term use of Haloperidol leading to increased expression of Kv4.3 channels may be the reason for the long-term changes in the excitability of dopaminergic neurons (Hahn et al., 2003). However, long-term use of haloperidol may lead to adverse reactions, including delayed onset of motor disorders such as tardive dyskinesia (TD). In a mouse model, long-term treatment of haloperidol resulted in decreased DPP6 expression in the brain, which is considered one of the possible causes of this adverse reaction (Tanaka et al., 2013). This reduction in DPP6 expression might be linked to the up regulation of Kv4 channels, potentially triggering a feedback regulatory mechanism. While the precise relationship between haloperidol and DPP6 remains unclear, and no direct evidence of binding exists, further research is needed to explore whether DPP6 plays a role in haloperidol’s effects on the central nervous system.
Amyotrophic Lateral Sclerosis (ALS) is a neurodegenerative disease primarily affecting motor neurons, characterized by muscle atrophy and loss of function. One in ten patients is a familial case. Several previous GWAS studies in European populations have suggested an association between DPP6 mutations and ALS. However, subsequent similar studies were unable to replicate this result in different populations (Van Es et al., 2008; Fogh et al., 2011; Daoud et al., 2010). Meanwhile, motor neurons reprogrammed from somatic cells of ALS patients using induced pluripotent stem cell (iPSC) models revealed a significant downregulation of DPP6 expression, as demonstrated by qPCR analysis (Sareen et al., 2013).
Similar to ALS, motor neurons in Spinal and Bulbar Muscular Atrophy (SBMA) undergo degenerative death, while Multiple Sclerosis (MS) mainly involves the central nervous system (brain and spinal cord) and has relatively less effect on lower motor neurons (Sahashi et al., 2015). However, these three diseases ultimately lead to motor disorders and muscle atrophy. A study on multiple sclerosis in the Nordic population showed that DPP6 levels were elevated in PBMCs of PrMS patients, although DPP6 is known to be mainly expressed in the brain and spinal cord, and DPP6 downregulation was observed in spinal motor neurons induced by SBMA iPSC differentiation (Brambilla, 2012; Sheila, 2019).
The changes in the expression levels of DPP6 in motor neurons, induced by reprogramming patient-derived cells, support the association of DPP6 with the aforementioned diseases. However, several GWAS studies have produced conflicting results, which may be attributed to the small sample size of rare diseases, the complex involvement of multi-gene interactions in ALS, or environmental factors.
Existing research has mostly focused on the role of DPP6 as a Kv4 helper subunit in the nervous system and heart, while research into its role in skeletal muscle and other tissues is still insufficient. The elevated expression level of DPP6 in PBMCs of PrMS patients suggests that pathological conditions may lead to high expression of DPP6 in other locations, or this ectopic expression itself may be one of the pathogenic factors. In addition to the aforementioned muscle atrophy-related diseases, short stature and low weight are also widely present in patients with DPP6 dysfunction, suggesting that DPP6 may have cross-system functions (Liao et al., 2013).
Cardiomyocytes rely on complex ion channel processes on the membrane to achieve their functions. Among these, Kv4 channels primarily regulate the transient outward potassium current (Ito), which plays a crucial role in the early repolarization of the cardiac action potential and serves as a key determinant of action potential morphology. DPP6, as an auxiliary subunit of Kv4, enhances its surface expression and potently accelerates its gating kinetics, thereby ensuring the normal activity of cardiomyocytes. Among heart-related diseases, Sudden Cardiac Death (SCD) has a high incidence rate and a large social burden (Stecker et al., 2014). Idiopathic vascular fibrosis (IVF) is one of the causes of SCD, and DPP6 mutation is a pathogenic factor of IVF (Viskin, 1990).
A typical risk mutation occurred in a multi-generational family in the Netherlands. In patients carrying the corresponding 7q36 mutation, the mRNA expression level of DPP6 is 22 times higher than that of the control group. This overexpression increases Ito and increases the probability of sudden cardiac death in patients. However, no partial mutations in the DPP6 exon were detected, indicating that this regulatory mutation may occur outside of the exon (Alders et al., 2009; Postema et al., 2011). In contrast to the increase in Ito when DPP6 is overexpressed, a decrease in Ito density is observed when DPP6 is knocked down (Xiao et al., 2013).
This disease risk is believed to be related to Purkinje Fibers (PF). In PF, overexpression of DPP6 markedly increased Ito density and enhanced sensitivity to tetraethylamine (TEA). Previous studies have shown that the sensitivity of Kv4 channels to TEA depends on the presence of DPP6 (Colinas et al., 2008). However, similar Ito enhancement and TEA sensitivity were not observed when DPP6 was overexpressed in ventricular myocytes (VM) (Xiao et al., 2013). This difference may be attributed to variations in cellular structure, Kv4 channels, and the abundance of other regulatory factors in PF compared to VM, which may facilitate a more pronounced regulatory role of DPP6 in PF.
In the heart, NaV1.5, encoded by the SCN5A gene, is the primary sodium channel responsible for generating the sodium current (INa), driving the rapid upstroke of the cardiac action potential (AP). While most of the INa (peak INa) is rapidly inactivated, a small fraction (INa late) persists throughout the AP plateau phase. Beyond its role as an auxiliary subunit of Ito channels, DPP6 has been shown to regulate NaV1.5 by reducing both peak INa and late INa in CHO cells. Furthermore, prolonged QT/QU intervals on an electrocardiogram (ECG) indicate delayed cardiac repolarization and an increased risk of arrhythmias; DPP6 mutations may contribute to these abnormalities, thereby increasing arrhythmia susceptibility (Rossetti et al., 2022).
Therefore, preventing sudden death is one of the important application directions of DPP6 research. In DPP6 haplotype carriers, primary prevention by implanting an ICD (implantable cardiac defibrillator) has been shown to significantly reduce the rate of sudden death in patients who have not undergone IVF. Meanwhile, the research results indicate that the mortality rate and arrhythmia recurrence rate of male patients are still relatively high, therefore further adjustments and optimization of treatment strategies are needed. In addition, infections and unnecessary electric shocks caused by ICD implantation remain pressing concerns. However, studies have suggested that median survival time of patients carrying the DPP6 risk haplotype has significantly increased compared to earlier estimates, which indicates that cascade screening, early diagnosis, and ICD treatment have contributed to mortality reduction (Bergeman et al., 2023; Elmonzer, 2022). Future research could combine genetic screening to develop more accurate risk prediction models for personalized interventions.
In summary, due to the regulatory role of DPP6 on ion channels, mutations of DPP6 are associated with abnormal discharges of excitable cells, which may further disrupt cellular functions and potentially lead to effects at the individual level. As mentioned earlier, DPP6-related diseases and their characteristics are shown in Table 1. It is evident that these conditions involve highly diverse pathogenic mechanisms. However, the symptoms associated with DPP6 mutations mentioned above typically do not manifest simultaneously in individuals carrying the mutation. The interaction between DPP6 and other regulatory factors, such as KCNIP2, FLNC, within different cells plays a crucial role in its functional modulation.
A recent study has revealed that DPP6 is one of the factors contributing to cognitive decline in Parkinson’s disease (Li et al., 2024). Given the strong association of DPP6 with Alzheimer’s disease and frontotemporal dementia, as well as the shared features among ALS, MS, and SBMA, it is worth considering whether these complex neurodegenerative diseases share a common molecular mechanism potentially linked to DPP6 dysfunction.
Interestingly, a study on organ-derived extracellular vesicles in mouse serum showed that DPP6, as an extracellular vesicle protein, exhibits 100% specificity to the brain, meaning it was exclusively detected in brain-derived extracellular vesicles with no peptides found in vesicles from other organs. Furthermore, the content of this protein in the extracellular vesicles of elderly mice is lower than that in young mice (Abdelmohsen et al., 2023). These extracellular vesicles can transport their contents across the blood–brain barrier, suggesting that the DPP6 content in serum extracellular vesicles could serve as a potential biomarker for dementia. This method does not require cerebrospinal fluid and can be easily detected through collecting blood. Another study showed that mutations in the DPP6 gene are more prevalent in the plasma exosomes of patients with neuroblastoma, but its precise role in this disease remains unclear (Degli Esposti et al., 2021).
Currently, research on DPP6 mainly focuses on high-expression tissues, and the study of its effects on other tissue cells is still minimal. However, previous reports have shown that DPP6 regulation is equally important outside of the heart and brain. For example, DPP6 could be one of the genes associated with lipid metabolism regulation, as indicated by lipid mass spectrometry analysis. Alterations in lipid metabolism can lead to cellular apoptosis or necrosis, potentially affecting tissues such as skeletal muscle (Martin et al., 2012).
Considering the role of DPP6 in different tissues and even in extracellular vehicles, it should be afforded necessary attention. Stem cell related technologies can be applied to expand research on DPP6. Specifically, DPP6 mutant patient derived cells and normal human derived cells (e.g., urine epithelial cells) can be collected and reprogrammed to induce differentiation in vitro to obtain different types of cell models including skeletal muscle cells, cardiomyocytes, and nerve cells.
Different samples can be compared using transcriptomics, proteomics, and other methods to analyze the expression and regulation of DPP6 in muscle tissue. Different tissue-specific DPP6 gene knockout models using Cre-loxP and other systems can be utilized to investigate their roles in muscle development and pathological processes at the individual level.
As described in the section on the risk of ventricular fibrillation associated with DPP6 mutations, the effects of DPP6 overexpression are more pronounced in PF than in VM. This suggests the potential existence of a compensatory or feedback inhibition mechanism regulating DPP6 expression in VM. Unraveling this mechanism could provide deeper insights into the pathophysiology of DPP6-related cardiac disorders and inform potential treatment strategies. Similarly, the directed differentiation of iPSCs derived from SBMA, IVF, and ASD patients carrying DPP6 mutations could facilitate the investigation of key cell types, including neurons, PF, and muscle cells. By analyzing differences in gene expression profiles, electrophysiological properties, and other cellular characteristics, we may gain a better understanding of how distinct DPP6 mutations and expression levels contribute to disease pathology, potentially identifying novel therapeutic targets.
While DPP6 is well established as a modulator of ion channels in specific neuronal populations, its functions in non-neuronal cells remain largely unexplored.
In future research, single-cell RNA sequencing or conditional knockout models could be utilized to determine whether DPP6 plays a role in glial cell function, thereby expanding our understanding of its physiological roles beyond synaptic regulation. In addition, the diagnosis of neurological diseases is an important direction for the application of DPP6. The diagnosis of neurological diseases such as AD and PD is difficult due to the difficulty and high risk of extracting cerebrospinal fluid for biomarker detection. When behavioral abnormalities are observed, the damage to the nervous system is usually irreversible. Based on the differential levels of DPP6 in brain derived extracellular vesicles in peripheral blood, it is possible to explore its potential as a biomarker for neurological diseases through clinical research.
X-YD: Writing – original draft. JH: Writing – review & editing. Z-YL: Writing – review & editing.
The author(s) declare that financial support was received for the research, authorship, and/or publication of this article. This work was partially supported by Science and Technology Planning Project of Guangdong Province, China (2023B1212060050, 2023B1212120009).
The authors declare that the research was conducted in the absence of any commercial or financial relationships that could be construed as a potential conflict of interest.
The authors declare that no Generative AI was used in the creation of this manuscript.
All claims expressed in this article are solely those of the authors and do not necessarily represent those of their affiliated organizations, or those of the publisher, the editors and the reviewers. Any product that may be evaluated in this article, or claim that may be made by its manufacturer, is not guaranteed or endorsed by the publisher.
Abdelmohsen, K., Herman, A. B., Carr, A. E., Henry-Smith, C. A., Rossi, M., Meng, Q., et al. (2023). Survey of organ-derived small extracellular vesicles and particles (sEVPs) to identify selective protein markers in mouse serum. J. Extracell. Biol. 2:e106. doi: 10.1002/jex2.106
Alders, M., Koopmann, T. T., Christiaans, I., Postema, P. G., Beekman, L., Tanck, M. W. T., et al. (2009). Haplotype-sharing analysis implicates chromosome 7q36 harboring DPP6 in familial idiopathic ventricular fibrillation. Am. J. Hum. Genet. 84, 468–476. doi: 10.1016/j.ajhg.2009.02.009
Anderson, L. T., Campbell, M., Adams, P., Small, A. M., Perry, R., and Shell, J. (1989). The effects of haloperidol on discrimination learning and behavioral symptoms in autistic children. J. Autism Dev. Disord. 19, 227–239. doi: 10.1007/BF02211843
Bartak, L., and Rutter, M. (1976). Differences between mentally retarded and normally intelligent autistic children. J. Autism Child. Schizophr. 6, 109–120. doi: 10.1007/BF01538054
Bekker, G.-J., Nakamura, H., and Kinjo, A. R. (2016). Molmil: a molecular viewer for the PDB and beyond. J. Cheminform. 8:42. doi: 10.1186/s13321-016-0155-1
Bekker, G., Yokochi, M., Suzuki, H., Ikegawa, Y., Iwata, T., Kudou, T., et al. (2022). Protein data Bank Japan: celebrating our 20th anniversary during a global pandemic as the Asian hub of three dimensional macromolecular structural data. Protein Sci. 31, 173–186. doi: 10.1002/pro.4211
Bergeman, A. T., Hoeksema, W. F., Van Der Ree, M. H., Boersma, L. V. A., Yap, S.-C., Verheul, L. M., et al. (2023). Outcomes in Dutch DPP6 risk haplotype for familial idiopathic ventricular fibrillation: a focused update. Neth. Heart J. 31, 309–314. doi: 10.1007/s12471-023-01792-1
Birnbaum, S. G., Varga, A. W., Yuan, L.-L., Anderson, A. E., Sweatt, J. D., and Schrader, L. A. (2004). Structure and function of Kv4-family transient potassium channels. Physiol. Rev. 84, 803–833. doi: 10.1152/physrev.00039.2003
Boyden, P. A., Dun, W., and Robinson, R. B. (2016). Cardiac Purkinje fibers and arrhythmias; the GK Moe award lecture 2015. Heart Rhythm. 13, 1172–1181. doi: 10.1016/j.hrthm.2016.01.011
Brambilla, P. (2012). Association between DPP6 polymorphism and the risk of progressive multiple sclerosis in northern and southern Europeans. Neurosci. Lett. 530, 155–160. doi: 10.1016/j.neulet.2012.10.008
Cacace, R., Heeman, B., Van Mossevelde, S., De Roeck, A., Hoogmartens, J., De Rijk, P., et al. (2019). Loss of DPP6 in neurodegenerative dementia: a genetic player in the dysfunction of neuronal excitability. Acta Neuropathol. 137, 901–918. doi: 10.1007/s00401-019-01976-3
Cai, X., Liang, C. W., Muralidharan, S., Kao, J. P. Y., Tang, C.-M., and Thompson, S. M. (2004). Unique roles of SK and Kv4.2 potassium channels in dendritic integration. Neuron 44, 351–364. doi: 10.1016/j.neuron.2004.09.026
Campbell, M., Anderson, L. T., Meier, M., Cohen, I. L., Small, A. M., Samit, C., et al. (1978). A comparison of haloperidol and behavior therapy and their interaction in autistic children. J. Am. Acad. Child Psychiatry 17, 640–655. doi: 10.1016/S0002-7138(09)61017-7
Colinas, O., Pérez-Carretero, F. D., López-López, J. R., and Pérez-García, M. T. (2008). A role for DPPX modulating external TEA sensitivity of Kv4 channels. J. Gen. Physiol. 131, 455–471. doi: 10.1085/jgp.200709912
Daoud, H., Valdmanis, P. N., Dion, P. A., and Rouleau, G. A. (2010). Analysis of DPP6 and FGGY as candidate genes for amyotrophic lateral sclerosis. Amyotroph. Lateral Scler. 11, 389–391. doi: 10.3109/17482960903358857
Darrow, S. M., Grados, M., Sandor, P., Hirschtritt, M. E., Illmann, C., Osiecki, L., et al. (2017). Autism Spectrum symptoms in a Tourette’s disorder sample. J. Am. Acad. Child Adolesc. Psychiatry 56, 610–617.e1. doi: 10.1016/j.jaac.2017.05.002
Degli Esposti, C., Iadarola, B., Maestri, S., Beltrami, C., Lavezzari, D., Morini, M., et al. (2021). Exosomes from plasma of neuroblastoma patients contain doublestranded DNA reflecting the mutational status of parental tumor cells. Int. J. Mol. Sci. 22:3667. doi: 10.3390/ijms22073667
Elmonzer, M. (2022). Genetics of sudden cardiac death and role of dipeptidyl peptidase like 6 gene. Master's thesis, The University of Iowa.
Esechie, A., Thottempudi, N., Patel, C., Shanina, E., and Li, X. (2023). A devastating neurological disorder: anti-dipeptidyl-peptidase-like protein 6 (DPPX) encephalitis causing rapidly progressive dementia. Cureus 15:e51123. doi: 10.7759/cureus.51123
Fogh, I., D’Alfonso, S., Gellera, C., Ratti, A., Cereda, C., Penco, S., et al. (2011). No association of DPP6 with amyotrophic lateral sclerosis in an Italian population. Neurobiol. Aging 32, 966–967. doi: 10.1016/j.neurobiolaging.2009.05.014
Hahn, J., Tse, T. E., and Levitan, E. S. (2003). Long-term K+ Channel-mediated dampening of dopamine neuron excitability by the antipsychotic drug haloperidol. J. Neurosci. 23, 10859–10866. doi: 10.1523/JNEUROSCI.23-34-10859.2003
Hu, H.-J., Carrasquillo, Y., Karim, F., Jung, W. E., Nerbonne, J. M., Schwarz, T. L., et al. (2006). The Kv4.2 Potassium Channel subunit is required for pain plasticity. Neuron 50, 89–100. doi: 10.1016/j.neuron.2006.03.010
Huisman-van Dijk, H. M., Schoot, R. V. D., Rijkeboer, M. M., Mathews, C. A., and Cath, D. C. (2016). The relationship between tics, OC, ADHD and autism symptoms: a cross-disorder symptom analysis in Gilles de la Tourette syndrome patients and family-members. Psychiatry Res. 237, 138–146. doi: 10.1016/j.psychres.2016.01.051
Kaulin, Y. A., De Santiago-Castillo, J. A., Rocha, C. A., Nadal, M. S., Rudy, B., and Covarrubias, M. (2009). The dipeptidyl-peptidase-like protein DPP6 determines the unitary conductance of neuronal Kv4.2 channels. J. Neurosci. 29, 3242–3251. doi: 10.1523/JNEUROSCI.4767-08.2009
Kin, Y., Misumi, Y., and Ikehara, Y. (2001). Biosynthesis and characterization of the brain-specific membrane protein DPPX, a dipeptidyl peptidase IV--related protein. J. Biochem. 129, 289–295. doi: 10.1093/oxfordjournals.jbchem.a002856
Kinjo, A. R., Bekker, G., Wako, H., Endo, S., Tsuchiya, Y., Sato, H., et al. (2018). New tools and functions in data-out activities at protein data Bank Japan (PDBj). Protein Sci. 27, 95–102. doi: 10.1002/pro.3273
Kise, Y., Kasuya, G., Okamoto, H. H., Yamanouchi, D., Kobayashi, K., Kusakizako, T., et al. (2021). Structural basis of gating modulation of Kv4 channel complexes. Nature 599, 158–164. doi: 10.1038/s41586-021-03935-z
Li, C., Hou, Y., Ou, R., Wei, Q., Zhang, L., Liu, K., et al. (2024). GWAS identifies DPP6 as risk gene of cognitive decline in Parkinson’s disease. J. Gerontol. A Biol. Sci. Med. Sci. 79:glae155. doi: 10.1093/gerona/glae155
Liao, C., Fu, F., Li, R., Yang, W., Liao, H., Yan, J., et al. (2013). Loss-of-function variation in the DPP6 gene is associated with autosomal dominant microcephaly and mental retardation. Eur. J. Med. Genet. 56, 484–489. doi: 10.1016/j.ejmg.2013.06.008
Lin, L., Murphy, J. G., Karlsson, R.-M., Petralia, R. S., Gutzmann, J. J., Abebe, D., et al. (2018). DPP6 loss impacts hippocampal synaptic development and induces behavioral impairments in recognition, learning and memory. Front. Cell. Neurosci. 12:84. doi: 10.3389/fncel.2018.00084
Lin, L., Petralia, R. S., Holtzclaw, L., Wang, Y.-X., Abebe, D., and Hoffman, D. A. (2022). Alzheimer’s disease/dementia-associated brain pathology in aging DPP6-KO mice. Neurobiol. Dis. 174:105887. doi: 10.1016/j.nbd.2022.105887
Lin, L., Petralia, R. S., Lake, R., Wang, Y.-X., and Hoffman, D. A. (2020). A novel structure associated with aging is augmented in the DPP6-KO mouse brain. Acta Neuropathol. Commun. 8:197. doi: 10.1186/s40478-020-01065-7
Lin, L., Sun, W., Throesch, B., Kung, F., Decoster, J. T., Berner, C. J., et al. (2013). DPP6 regulation of dendritic morphogenesis impacts hippocampal synaptic development. Nat. Commun. 4:2270. doi: 10.1038/ncomms3270
Marshall, C. R., Noor, A., Vincent, J. B., Lionel, A. C., Feuk, L., Skaug, J., et al. (2008). Structural variation of chromosomes in autism Spectrum disorder. Am. J. Hum. Genet. 82, 477–488. doi: 10.1016/j.ajhg.2007.12.009
Martin, L. J., Kissebah, A. H., and Olivier, M. (2012). Accounting for a quantitative trait locus for plasma triglyceride levels: utilization of variants in multiple genes. PLoS One 7:e34614. doi: 10.1371/journal.pone.0034614
Nadin, B. M., and Pfaffinger, P. J. (2013). A new TASK for dipeptidyl peptidase-like protein 6. PLoS One 8:e60831. doi: 10.1371/journal.pone.0060831
Postema, P. G., Christiaans, I., Hofman, N., Alders, M., Koopmann, T. T., Bezzina, C. R., et al. (2011). Founder mutations in the Netherlands: familial idiopathic ventricular fibrillation and DPP6. Neth. Heart J. 19, 290–296. doi: 10.1007/s12471-011-0102-8
Pottier, C., Ren, Y., Perkerson, R. B., Baker, M., Jenkins, G. D., Van Blitterswijk, M., et al. (2019). Genome-wide analyses as part of the international FTLD-TDP whole-genome sequencing consortium reveals novel disease risk factors and increases support for immune dysfunction in FTLD. Acta Neuropathol. 137, 879–899. doi: 10.1007/s00401-019-01962-9
Prontera, P., Napolioni, V., Ottaviani, V., Rogaia, D., Fusco, C., Augello, B., et al. (2014). DPP6 gene disruption in a family with Gilles de la Tourette syndrome. Neurogenetics 15, 237–242. doi: 10.1007/s10048-014-0418-9
Remington, G., Sloman, L., Konstantareas, M., Parker, K., and Gow, R. (2001). Clomipramine versus haloperidol in the treatment of autistic disorder: a double-blind, placebo-controlled, crossover study. J. Clin. Psychopharmacol. 21, 440–444. doi: 10.1097/00004714-200108000-00012
Robertson, M. M., Eapen, V., Singer, H. S., Martino, D., Scharf, J. M., Paschou, P., et al. (2017). Gilles de la Tourette syndrome. Nat. Rev. Dis. Primers 3, 1–20. doi: 10.1038/nrdp.2016.97
Rossetti, A., Spatjens, R. L. H., Kammerer, S., Stoks, J., Firneburg, R., Seyen, S. R., et al. (2022). An emerging role for DPP6: reciprocal regulation of INa-Ito and implications for arrhythmogenesis. Eur. Heart J. 43:ehac544-2886. doi: 10.1093/eurheartj/ehac544.2886
Sahashi, K., Katsuno, M., Hung, G., Adachi, H., Kondo, N., Nakatsuji, H., et al. (2015). Silencing neuronal mutant androgen receptor in a mouse model of spinal and bulbar muscular atrophy. Hum. Mol. Genet. 24, 5985–5994. doi: 10.1093/hmg/ddv300
Sareen, D., O’Rourke, J. G., Meera, P., Muhammad, A. K. M. G., Grant, S., Simpkinson, M., et al. (2013). Targeting RNA foci in iPSC-derived motor neurons from ALS patients with a C9ORF72 repeat expansion. Sci. Transl. Med. 5:208ra149. doi: 10.1126/scitranslmed.3007529
Seeman, P., and Tallerico, T. (1998). Antipsychotic drugs which elicit little or no Parkinsonism bind more loosely than dopamine to brain D2 receptors, yet occupy high levels of these receptors. Mol. Psychiatry 3, 123–134. doi: 10.1038/sj.mp.4000336
Sheila, M. (2019). Phenotypic and molecular features underlying neurodegeneration of motor neurons derived from spinal and bulbar muscular atrophy patients. Neurobiol. Dis. 124, 1–13. doi: 10.1016/j.nbd.2018.10.019
Soh, H., and Goldstein, S. A. N. (2008). ISA Channel complexes include four subunits each of DPP6 and Kv4.2. J. Biol. Chem. 283, 15072–15077. doi: 10.1074/jbc.M706964200
Stecker, E. C., Reinier, K., Marijon, E., Narayanan, K., Teodorescu, C., Uy-Evanado, A., et al. (2014). Public health burden of sudden cardiac death in the United States. Circ. Arrhythm. Electrophysiol. 7, 212–217. doi: 10.1161/CIRCEP.113.001034
Strop, P., Bankovich, A. J., Hansen, K. C., Christopher Garcia, K., and Brunger, A. T. (2004). Structure of a human A-type Potassium Channel interacting protein DPPX, a member of the dipeptidyl aminopeptidase family. J. Mol. Biol. 343, 1055–1065. doi: 10.1016/j.jmb.2004.09.003
Tanaka, S., Syu, A., Ishiguro, H., Inada, T., Horiuchi, Y., Ishikawa, M., et al. (2013). DPP6 as a candidate gene for neuroleptic-induced tardive dyskinesia. Pharmacogenomics J. 13, 27–34. doi: 10.1038/tpj.2011.36
Van Es, M. A., Van Vught, P. W., Blauw, H. M., Franke, L., Saris, C. G., Van Den Bosch, L., et al. (2008). Genetic variation in DPP6 is associated with susceptibility to amyotrophic lateral sclerosis. Nat. Genet. 40, 29–31. doi: 10.1038/ng.2007.52
Viskin, S. (1990). Idiopathic ventricular fibrillation. Am. Heart J. 120, 661–671. doi: 10.1016/0002-8703(90)90025-S
Wada, K., Yokotani, N., Hunter, C., Doi, K., Wenthold, R. J., and Shimasaki, S. (1992). Differential expression of two distinct forms of mRNA encoding members of a dipeptidyl aminopeptidase family. Proc. Natl. Acad. Sci. USA 89, 197–201. doi: 10.1073/pnas.89.1.197
Xiao, L., Koopmann, T. T., Ördög, B., Postema, P. G., Verkerk, A. O., Iyer, V., et al. (2013). Unique cardiac Purkinje fiber transient outward current β-subunit composition: a potential molecular link to idiopathic ventricular fibrillation. Circ. Res. 112, 1310–1322. doi: 10.1161/CIRCRESAHA.112.300227
Keywords: DPP6, Kv4 channels, ion channel regulation, neurological diseases, cardiovascular disease
Citation: Ding X-Y, Habimana JdD and Li Z-Y (2025) The role of DPP6 dysregulation in neuropathology: from synaptic regulation to disease mechanisms. Front. Cell. Neurosci. 19:1547495. doi: 10.3389/fncel.2025.1547495
Received: 18 December 2024; Accepted: 17 February 2025;
Published: 05 March 2025.
Edited by:
Alessandro Tozzi, University of Perugia, ItalyReviewed by:
Satchal Erramilli, Meso Scale Diagnostics LLC, United StatesCopyright © 2025 Ding, Habimana and Li. This is an open-access article distributed under the terms of the Creative Commons Attribution License (CC BY). The use, distribution or reproduction in other forums is permitted, provided the original author(s) and the copyright owner(s) are credited and that the original publication in this journal is cited, in accordance with accepted academic practice. No use, distribution or reproduction is permitted which does not comply with these terms.
*Correspondence: Zhi-Yuan Li, bGlfemhpeXVhbkBnaWJoLmFjLmNu
Disclaimer: All claims expressed in this article are solely those of the authors and do not necessarily represent those of their affiliated organizations, or those of the publisher, the editors and the reviewers. Any product that may be evaluated in this article or claim that may be made by its manufacturer is not guaranteed or endorsed by the publisher.
Research integrity at Frontiers
Learn more about the work of our research integrity team to safeguard the quality of each article we publish.